ABSTRACT
Convalescent plasma has been extensively tested during the COVID-19 pandemic as a transfusion product. Similarly, monoclonal antibodies have been largely administered either intravenously or intramuscularly. Nevertheless, when used against a respiratory pathogen, respiratory delivery is preferable to maximize the amount of antibody that reaches the entry door in order to prevent sustained viral multiplication. In this narrative review, we review the different types of inhalation device and summarize evidence from animal models and early clinical trials supporting the respiratory delivery (for either prophylactic or therapeutic purposes) of convalescent plasma or monoclonal antibodies (either full antibodies, single-chain variable fragments, or camelid-derived monoclonal heavy-chain only antibodies). Preliminary evidences from animal models suggest similar safety and noninferior efficacy, but efficacy evaluation from clinical trials is still limited.
Introduction
While the mucosal (especially nasal) route is being increasingly investigated for COVID-19 vaccination, very few studies have reported intranasal (i.n.) delivery of passive immunotherapies. Respiratory delivery presents less logistical burden, offering a needle- and nurse-free delivery, requiring lower antibody doses (which facilitates scaling up of deployment), enabling self-administration at home, and not requiring refrigeration (which is relevant in low-and-middle-income countries).Citation1,Citation2
Intranasal delivery of passive immunotherapies against human pathogens has been scarcely used in the past. In the setting of influenza virus (including highly pathogenic avian influenza), administration of broadly-neutralizing IgGCitation3,Citation4 or IgACitation5 through i.n. and aerosol routes in mice showed higher prophylactic protection as compared to systemic routes, and controlled the advanced stage infection with a much-reduced dose. All of this translates into higher chances of viral clearance and lower chances of treatment-emergent resistance.Citation6 In comparison to parenteral treatment, inhalation of IgG’s gave better protection and a greater therapeutic response in pneumonia models using the influenza virus.Citation7,Citation8 Nasal delivery of viral vectors encoding anti-influenza IgG has also been tested successfully in mice.Citation9 More limited experiences have been reported for monkeypox virusCitation10 and respiratory syncytial virus.Citation11 Again, no clinical study has been run for those pathogens.
All the monoclonal antibodies (mAb) targeting the SARS-CoV-2 Spike protein and emergency-use authorized since the beginning of the COVID-19 pandemic have been of the IgG1 subtype: the levels of IgG antibodies after intravenous infusion are 200–500 times lower in the mucosal compartments than in serum.Citation4 This results in the need for administration of high doses of IgG (i.e., up to 8 g) to achieve antiviral activity in respiratory tissues. Despite such high doses, the antiviral effect is still marginal in the respiratory tract compared to the placebo controls.Citation5 On the contrary IgMCitation12 and IgA1Citation13 assemble into multimers via the J-chain, facilitating mucosal transcytosis, and can be nebulized:Citation14 of interest, IgA represents the majority of SARS-CoV-2-neutralizing antibodies in vivo.Citation15
Inhalation devices
In addition to drop inoculation, three different types of inhalation devices are commonly used ().Citation16 Nebulizers (jet, ultrasonic, and vibrating mesh) use a liquid solution of the medicine to produce aerosol droplets and are used to deliver them to airways and lungs: they are cheap but prolonged storage of proteins in liquid solutions causes deamination, hydrolysis, and aggregation,Citation17–19 which can be minimized by the use of surfactant.Citation18 Human immunoglobulin preparations were successfully deposited into the airways as well as the alveoli of treated lungs when nebulized with eFlow® in both rats and non-human primates (NHP) studies.Citation14
Figure 1. Potential approaches for respiratory delivery of passive immunotherapeutics against COVID-19.
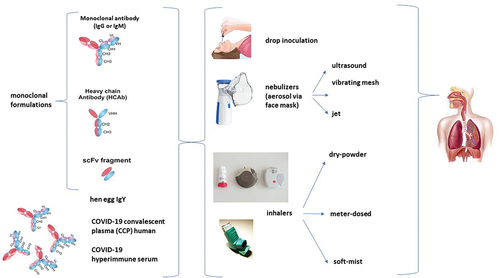
Dry powder inhalers (DPIs) are propellant-free devices that deliver dry powder (solid) aerosol to the lungs, which comes with shelf-life advantages. Unfortunately, pure antibody solutions significantly agglomerate during spray drying,Citation20 requiring excipients such as trehalose or Tween 20 surfactant.Citation21–23 The recent phase 1 double-blind, randomized clinical trial (RCT) on inhaled IL-13 mAb supports the development of inhaled antibodies as a viable future therapy option for parenteral mAbs. VR942 (an anti-IL-13 mAb) represents a first-in-class for DPI-delivered mAbs.Citation24
Finally, metered-dose inhalers (MDIs) create fixed-dose aerosolsCitation25 but come with the limitation of requiring lipophilic propellers, which hydrophilic biologics cannot solve,Citation26 thus limiting their dose range.Citation27,Citation28
Search strategy
On June 20, 2023, we searched PubMed for English-language literature published after December 1, 2019, using the query “SARS-CoV-2 AND (intranasal OR nasal OR inhaled OR aerosol OR spray OR inhalable) AND (“monoclonal antibody” OR “convalescent plasma” or “immunoglobulins” or “nanobodies” or “scFv”).”
Results
Monoclonal antibodies (mAb)
Human mAb
Most studies reported protective efficacy of single dose administered via nasal inoculation within a few hours after SARS-CoV-2 challenge in K18-hACE2 mice (IgM-14,Citation29 F61 and H121Citation30) or golden (Syrian) hamster model (STI-2020 and STI-5041,Citation31 1249A8 ± 1213H7,Citation32 58G6Citation33). A few studies also tested prophylactic models in mice (IgM-14,Citation29 DZIF-10cCitation34).
While doses were in the range of 2–5 mg/kg of body weight, the lowest dose was achieved using an IgM (0.044 mg/kg of IgM-14), a mAb engineered from COV2–14 which persisted 168 h in the lungs.Citation29
Delivery modes alternative to drop inoculation have been also investigated in animal models. At a single 5 mg/kg nebulized dose, the peak HB27 concentration in mice’s pulmonary epithelial lining fluid reached 857.8 μg/mL, 670-fold higher than the PRNT90 value of 1.28 μg/mL, and was maintained above PRNT90 over 240 h.Citation35 Dual administration of WKS13 mAb in particles below 5 mm (by 2-fluid nozzle) or above 10 mm (by ultrasonic nozzle) to the lung and nasal cavity of hamsters, respectively, was effective in offering prophylactic protection against the SARS-CoV-2 Delta variant.Citation36
Only two clinical trials have been reported so far. In a phase 1 clinical trial, a single nasal spray of 35B5 conveyed 24-h effective in vitro protection against SARS-CoV-2 VOCs, including the Alpha, Beta, Delta, or Omicron variants in 30 healthy volunteers.Citation37 In a single-blind placebo-controlled RCT of post-exposure prophylaxis in healthy adult workers, the anti-Spike mAb SA58 reduced laboratory-confirmed symptomatic COVID-19 from 2.8 to 1.04 per 100 person-days (61% efficacy).Citation38
Lumen Bioscience has recently reported exploiting its spirulina-based oral delivery platform for i.n. delivery of its anti-Spike mAb cocktail LMN-301.Citation39 The edible photosynthetic cyanobacterium Arthrospira platensis (spirulina) can be engineered to express the mAbs and dry spirulina can be used to encapsulate the mAbs.Citation40,Citation41
Single chain variable fragments (scFv)
Being devoid of immunoglobulin Fc, scFv antibodies are not at risk for inducing antibody-dependent enhancement (ADE) of viral infection, and because of their rapid production at large scale in non-mammalian expression systems, they could be developed at a relatively lower cost compared with mAbs. Moreover, because of their smaller size (about 28 kDa vs 150 kDa of mAbs) and lower complexity (no glycosylation), they are particularly stable and suitable for topical use in non-hospital settings. For example, the first human scFv antibody, brolucizumab, was approved in 2019 for topical treatment of exudative age-related macular degeneration by the FDA, thus paving the regulatory pathway for this class of molecules,Citation42 while the anti-CD3 mAb foralumab has been used i.n. in COVID-19.Citation43 I.n. administration (37 μg/nostril) of scFv76 2 h before and 4 h after infection, completely blocked infection by luciferase-expressing D614G S-pseudotyped virus in hACE2-expressing transgenic mice, as deduced by the loss of luminescent signal; the same was true when 35 μg/nostril were administered to hamsters 2 h before and once daily for the 2 consecutive days post-infection.Citation44 Furthermore, nebulized scFv76 antibodies can be effectively delivered to the lung down to the alveolar space, as shown by immunohistochemistry.
Camelid-derived monoclonal heavy-chain only antibodies (HcAbs)
Camelids (camels, dromedaries, alpacas and llamas) produce antibodies devoid of light chainsCitation45 and highly thermostable, named heavy-chain only antibodies (HcAbs), and weighing approximately 90 kDa. The variable domain on each heavy chain (VHH or nanobody, 15 kDa) is longer, acting like an extended hand, able to reach buried sites the short-handed classical antibodies can’t.Citation46 However, being nanobodies of camelid origin, they require sophisticated humanization procedures to avoid immunogenic responses, potentially hampering their full development: e.g., nanobodies can be humanized by fusing them to human Fc regions.
Small, single-domain VHH nanobodies, specific for SARS-CoV-2, were proposed for respiratory delivery as an alternative to systemic mAbs: e.g., 50 µg of PiN-21 treated infection in Syrian hamsters when administered immediately or 6 h post-infection via aerosol generated with a vibrating mesh nebulizers.Citation47 A bispecific nanobody dimer (2–3-Fc), manufactured by fusing aSA3-Fc to aRBD-2, a previously identified broad-spectrum nanobody targeting an RBD epitope distinct from aSA3, when prophylactically inoculated with nasal drops drastically reduced viral RNA loads and completely eliminated infectious Omicron particles in the trachea and lungs of hamsters at three low doses of 0.5 mg/kg, 2–3-Fc.Citation48 Nasal drop inoculation of K-874A, effectively inhibited SARS-CoV-2 titers in the infected lungs of Syrian hamsters without causing weight loss or cytokine induction.Citation49 I.n. drop inoculation of alpaca-derived Nb22 exhibited protection against the SARS-CoV-2 Delta variant in post-exposure prophylaxis (PEP) and pre-exposure prophylaxis (PrEP). Of note, i.n. Nb22 also demonstrated high efficacy against the SARS-CoV-2 Delta for 7 days when administered as single-dose prophylaxis, and exhibited long-lasting retention in the respiratory system for >1 month when administered by four doses.Citation50
VHH-Fc ABS-VIR-001 (a trispecific VHH fused to the Fc of human IgG1), thermostable at 45°C for up to 4 weeks, prevented SARS-CoV-2 infection and death when provided as an i.n. drop inoculation prophylaxis in a humanized ACE-2 mouse model. In addition, ABS-VIR-001 post-exposure treatment was shown to greatly reduce viral loads by as much as 50-fold.Citation51 In mice-adapted COVID-19 severe model, intranasal drop inoculation of three alpaca-derived nanobodies fused to Fcγ1 (aVHH-11-Fc, aVHH-13-Fc and aVHH-14-Fc) effectively protected mice from lethal challenges with Delta, BA.1 and BA.2, and reduced viral loads in both the upper and lower respiratory tracts.Citation52
Polyclonal immunoglobulins
No study has been reported yet deploying i.n. the two most common human polyclonal immunoglobulin formulations, namely standard IVIG and hyperimmune serum.
We instead have studies on polyclonal immunoglobulins generated in commercial hens (IgY), which are concentrated in their eggs to 50–100 mg/egg within 2–3 weeks after vaccination (the yearly yield per hen can be escalated up to 5 times higher when using specific-pathogen-free (SPF) hens). IgY antibodies have shown good thermal stability and can be stored stably in a liquid state at 2–8’C for 6 months, or at room temperature for 2 weeks.Citation2 Strikingly, egg production has already been scaled up, making low-cost and large-scale IgY antibodies feasible. Nasal drop inoculation of broadly protective IgY antibody against SARS-CoV-2 showed a residence time of 2–4 hCitation53 and conferred significant protection against SARS-CoV-2 infection in hACE2-transgenic mice,Citation54 ratsCitation55 and hamsters.Citation56 A double-blind, placebo-controlled, phase 1 RCT (NCT04567810) evaluating single-ascending and multiple doses of anti-SARS-CoV-2 RBD IgYadministered i.n. for 14 days in 48 healthy adults also demonstrated an excellent safety and tolerability profile, and no evidence of systemic absorption.Citation55
COVID-19 convalescent plasma (CCP)
Wouters et al tested i.n. drop inoculation of 25 μL CCP/nostril (i.e., 0.01–0.06 mg anti-RBD antibodies/kg) in wild-type Syrian golden hamsters exposed the day after to infected littermates. Animals received CCP for 4 more days after challenge and were sacrificed on the last day: the authors found that 40% of CCP treated hamsters were fully protected and 40% had significantly reduced viral loads. The effect was dose-dependent because high-titer CCP from a vaccinated donor was more effective than low-titer CCP from a donation prior to vaccine rollout. I.n. administration of human CCP resulted in a reactive (immune) response in hamster lungs, whose contribution compared to viral neutralization could not be discerned.Citation57
Notably, respiratory delivery of human CCP for therapeutic purposes has not been attempted so far.
Discussion
Passive immunotherapies are not the only protein biologics considered for COVID-19. Other biologics considered for respiratory delivery include ACE2 decoysCitation58 and exosomes from convalescent human immune cells,Citation59 but passive immunotherapies remain the most tested and reliable antiviral.
The preliminary findings we reviewed here support clinical safety and efficacy of passive immunotherapies delivered to the respiratory tract. Overall, preliminary findings reviewed in this manuscript support that intranasal delivery of passive immunotherapies is as safe asintravenous or intramuscular administration, Blood derivatives other than immunoglobulins have been administered mucosally in the past. E.g., human platelet lysates are used to treat conjunctivitis and keratitis due to either Sjogren syndrome or graft-versus-host disease. As such, excellent safety is expected from i.n. administration of passive immunotherapeutics. In regard to efficacy, clinical trials are still very limited in number:Citation37,Citation38,Citation55 given the widespread availability of oral small-molecule antivirals, proving superior efficacy at preventing disease progression in head-to-head RCTs will likely be needed to implement further development by pharma manufacturers. Discriminating the relative contribution to viral eradication of the local inflammatory reaction against xenogeneic human proteins that are invariably observed in immunocompetent animal models will provide informative insights.
As per any antiviral approach, delivery of a prophylactic regiment as opposed to a therapeutic regiment comes with obvious differences. In particular, modification of mAb half-life (as achieved, e.g., for the Evusheld™ mAb cocktailCitation60 has not been attempted so far during intranasal delivery: even if they would be engineered, mucus clearance is likely to remain a major barrier to the actual half-life of mAbs delivered in the respiratory tract. As such pre-exposure prophylaxis with respiratory delivery is likely to require repeated and very frequent administrations. Although intranasal delivery comes with a much comfortable administration than other parenteral routes, is the authors’ opinion that intranasal delivery of passive immunotherapeutics will likely only have a role as for post-exposure prophylaxis or early treatment of COVID-19 outpatients. In particular, it could prove useful for the frail immunocompromised patients who cannot tolerate the side effects or the pharmacokinetic interactions of small-molecule antivirals.
Disclosure statement
No potential conflict of interest was reported by the author(s).
Additional information
Funding
References
- Chavda VP, Baviskar KP, Vaghela DA, Raut SS, Bedse AP. Nasal sprays for treating COVID-19: a scientific note. Pharmacol Rep. 2023;75(2):249–6. doi:10.1007/s43440-023-00463-7.
- Parray HA, Shukla S, Perween R, Khatri R, Shrivastava T, Singh V, Murugavelu P, Ahmed S, Samal S, Sharma C, et al. Inhalation monoclonal antibody therapy: a new way to treat and manage respiratory infections. Appl Microbiol Biotechnol. 2021;105(16–17):6315–32. doi:10.1007/s00253-021-11488-4.
- Ibañez LI, De Filette M, Hultberg A, Verrips T, Temperton N, Weiss RA, Vandevelde W, Schepens B, Vanlandschoot P, Saelens X, et al. Nanobodies with in vitro neutralizing activity protect mice against H5N1 influenza virus infection. J Infect Dis. 2011;203(8):1063–72. doi:10.1093/infdis/jiq168.
- He F, Kumar SR, Syed Khader SM, Tan Y, Prabakaran M, Kwang J. Effective intranasal therapeutics and prophylactics with monoclonal antibody against lethal infection of H7N7 influenza virus. Antiviral Res. 2013;100(1):207–14. doi:10.1016/j.antiviral.2013.08.003.
- Ye J, Shao H, Hickman D, Angel M, Xu K, Cai Y, Song H, Fouchier RAM, Qin A, Perez DR, et al. Intranasal delivery of an IgA monoclonal antibody effective against sublethal H5N1 influenza virus infection in mice. Clin Vaccine Immunol. 2010;17(9):1363–70. doi:10.1128/CVI.00002-10.
- Casadevall A, Focosi D. SARS-CoV-2 variants resistant to monoclonal antibodies in immunocompromised patients is a public health concern. J Clin Invest. 2023;133(6). doi:10.1172/JCI168603.
- Leyva-Grado VH, Tan GS, Leon PE, Yondola M, Palese P. Direct administration in the respiratory tract improves efficacy of broadly neutralizing anti-influenza virus monoclonal antibodies. Antimicrob Agents Chemother. 2015;59(7):4162–72. doi:10.1128/AAC.00290-15.
- Sun T, Wang Y, Zou P, Wang Q, Liu J, Liu W, Huang J, Wu F. M2e-specific antibodies protect against influenza PR8 virus in an isotype and route dependent manner. J Med Virol. 2023;95:e28721. doi:10.1002/jmv.28721.
- Michelini Z, Minkoff JM, Yang J, Negri D, Cara A, Hanson BJ, Salvatore M. Integrase-defective lentiviral vectors for delivery of monoclonal antibodies against influenza. Viruses. 2020;12(12):12. doi:10.3390/v12121460.
- Mucker EM, Wollen-Roberts SE, Kimmel A, Shamblin J, Sampey D, Hooper JW, Geisbert T. Intranasal monkeypox marmoset model: prophylactic antibody treatment provides benefit against severe monkeypox virus disease. PLoS Negl Trop Dis. 2018;12(6):e0006581. doi:10.1371/journal.pntd.0006581.
- Jacque E, Chottin C, Laubreton D, Nogre M, Ferret C, de Marcos S, Baptista L, Drajac C, Mondon P, De Romeuf C, et al. Hyper-enriched anti-RSV immunoglobulins nasally administered: a promising approach for respiratory syncytial virus prophylaxis. Front Immunol. 2021;12:683902. doi:10.3389/fimmu.2021.683902.
- Li Y, Wang G, Li N, Wang Y, Zhu Q, Chu H, Wu W, Tan Y, Yu F, Su X-D, et al. Structural insights into immunoglobulin M. Science. 2020;367(6481):1014–17. doi:10.1126/science.aaz5425.
- Kumar N, Arthur CP, Ciferri C, Matsumoto ML. Structure of the secretory immunoglobulin a core. Science. 2020;367(6481):1008–14. doi:10.1126/science.aaz5807.
- Vonarburg C, Loetscher M, Spycher MO, Kropf A, Illi M, Salmon S, Roberts S, Steinfuehrer K, Campbell I, Koernig S, et al. Topical application of nebulized human IgG, IgA and IgAM in the lungs of rats and non-human primates. Respir Res. 2019;20(1):99. doi:10.1186/s12931-019-1057-3.
- Wang Z, Lorenzi JCC, Muecksch F, Finkin S, Viant C, Gaebler C, Cipolla M, Hoffmann HH, Oliveira TY, Oren DA, et al. Enhanced SARS-CoV-2 neutralization by dimeric IgA. Sci Transl Med. 2020;13(577):33288661.
- Moroni-Zentgraf P, Usmani OS, Halpin DMG. Inhalation devices. Can Resp J. 2018;2018:5642074. doi:10.1155/2018/5642074.
- Kane C, O’Neil K, Conk M, Picha K. Inhalation delivery of protein therapeutics. Inflamm Allergy Drug Targets. 2013;12(2):81–7. doi:10.2174/1871528111312020002.
- Respaud R, Marchand D, Parent C, Pelat T, Thullier P, Tournamille JF, Viaud-Massuard M-C, Diot P, Si-Tahar M, Vecellio L, et al. Effect of formulation on the stability and aerosol performance of a nebulized antibody. MAbs. 2014;6(5):1347–55. doi:10.4161/mabs.29938.
- Mayor A, Thibert B, Huille S, Respaud R, Audat H, Heuzé-Vourc’h N. Inhaled antibodies: formulations require specific development to overcome instability due to nebulization. Drug Deliv Transl Res. 2021;11(4):1625–33. doi:10.1007/s13346-021-00967-w.
- Faghihi H, Najafabadi AR, Vatanara A. Optimization and characterization of spray-dried IgG formulations: a design of experiment approach. DARU. 2017;25:22. doi:10.1186/s40199-017-0187-8.
- Cleland JL, Jones AJ. Stable formulations of recombinant human growth hormone and interferon-gamma for microencapsulation in biodegradable microspheres. Pharm Res. 1996;13(10):1464–75. doi:10.1023/A:1016063109373.
- Mumenthaler M, Hsu CC, Pearlman R. Feasibility study on spray-drying protein pharmaceuticals: recombinant human growth hormone and tissue-type plasminogen activator. Pharm Res. 1994;11(1):12–20. doi:10.1023/A:1018929224005.
- Jayasundera M, Adhikari B, Adhikari R, Aldred P. The effects of proteins and low molecular weight surfactants on spray drying of model sugar-rich foods: powder production and characterisation. J Food Eng. 2011;104(2):259–71. doi:10.1016/j.jfoodeng.2010.12.017.
- Burgess G, Boyce M, Jones M, Larsson L, Main MJ, Morgan F, Phillips P, Scrimgeour A, Strimenopoulou F, Vajjah P, et al. Randomized study of the safety and pharmacodynamics of inhaled interleukin-13 monoclonal antibody fragment VR942. EBioMedicine. 2018;35:67–75. doi:10.1016/j.ebiom.2018.07.035.
- Ibrahim M, Verma R, Garcia-Contreras L. Inhalation drug delivery devices: technology update. Med Devices (Auckland, NZ). 2015;8:131–9. doi:10.2147/MDER.S48888.
- Liao YH, Brown MB, Jones SA, Nazir T, Martin GP. The effects of polyvinyl alcohol on the in vitro stability and delivery of spray-dried protein particles from surfactant-free HFA 134a-based pressurised metered dose inhalers. Int J Pharm. 2005;304(1–2):29–39. doi:10.1016/j.ijpharm.2005.07.013.
- Fathe K, Ferrati S, Moraga-Espinoza D, Yazdi A, Smyth HD. Inhaled biologics: from preclinical to product approval. Curr Pharm Des. 2016;22(17):2501–21. doi:10.2174/1381612822666160210142910.
- Liang W, Pan HW, Vllasaliu D, Lam JKW. Pulmonary delivery of biological drugs. Pharmaceutics. 2020;12(11):12. doi:10.3390/pharmaceutics12111025.
- Ku Z, Xie X, Hinton PR, Liu X, Ye X, Muruato AE, Ng DC, Biswas S, Zou J, Liu Y, et al. Nasal delivery of an IgM offers broad protection from SARS-CoV-2 variants. Nature. 2021;595(7869):718–23. doi:10.1038/s41586-021-03673-2.
- Lu J, Yin Q, Pei R, Zhang Q, Qu Y, Pan Y, Sun L, Gao D, Liang C, Yang J, et al. Nasal delivery of broadly neutralizing antibodies protects mice from lethal challenge with SARS-CoV-2 delta and omicron variants. Virol Sin. 2022;37(2):238–47. doi:10.1016/j.virs.2022.02.005.
- Cao X, Maruyama J, Zhou H, Fu Y, Kerwin L, Powers C, Sattler RA, Manning JT, Singh A, Lim R, et al. Unbiased approach to identify and assess efficacy of human SARS-CoV-2 neutralizing antibodies. Sci Rep. 2022;12(1):15517. doi:10.1038/s41598-022-19780-7.
- Piepenbrink MS, Park JG, Deshpande A, Loos A, Ye C, Basu M, Sarkar S, Khalil AM, Chauvin D, Woo J, et al. Potent universal beta-coronavirus therapeutic activity mediated by direct respiratory administration of a Spike S2 domain-specific human neutralizing monoclonal antibody. PLoS Pathog. 2022;18(7):e1010691. doi:10.1371/journal.ppat.1010691.
- Zhang X, Zhang H, Li T, Chen S, Luo F, Zhou J, Zheng P, Song S, Wu Y, Jin T, et al. A potent neutralizing antibody provides protection against SARS-CoV-2 Omicron and Delta variants via nasal delivery. Sig Transduct Target Ther. 2022;7:301. doi:10.1038/s41392-022-01135-3.
- Halwe S, Kupke A, Vanshylla K, Liberta F, Gruell H, Zehner M, Rohde C, Krähling V, Gellhorn Serra M, Kreer C, et al. Intranasal administration of a monoclonal neutralizing antibody protects mice against SARS-CoV-2 infection. Viruses. 2021;13(8):1498. doi:10.3390/v13081498.
- Jia J, Yin Z, Zhang X, Li H, Meng D, Liu Q, Wang H, Han M, Suo S, Liu Y, et al. Feasibility studies of nebulized SARS-CoV-2 neutralizing antibody in mice and cynomolgus monkeys. Pharm Res. 2022;39(9):2191–201. doi:10.1007/s11095-022-03340-9.
- Seow HC, Cai JP, Pan HW, Luo C, Wen K, Situ J, Wang K, Cao H, Leung SWS, Yuan S, et al. Neutralisation of SARS-CoV-2 by monoclonal antibody through dual targeting powder formulation. J Control Release. 2023;358:128–41. doi:10.1016/j.jconrel.2023.04.029.
- Lin Y, Yue S, Yang Y, Yang S, Pan Z, Yang X, Gao L, Zhou J, Li Z, Hu L, et al. Nasal spray of neutralizing monoclonal antibody 35B5 confers potential prophylaxis against severe acute respiratory syndrome coronavirus 2 variants of concern: a small-scale clinical trial. Clin Infect Dis. 2023;76:e336–e41. doi:10.1093/cid/ciac448.
- Song R, Zeng G, Yu J, Meng X, Chen X, Li J, et al. Post-exposure prophylaxis with SA58 (anti-SARS-COV-2 monoclonal antibody) nasal spray for the prevention of symptomatic COVID-19 in healthy adult workers: a randomized, single-blind, placebo-controlled clinical study. Emerging Microbes Infect. 2023:2212806. doi:10.1080/22221751.2023.2212806.
- BARDA-funded Intranasal Antibody Cocktail for COVID-19 Shows Promise in a Preclinical Study. [accessed 2023 Jun 6]. https://www.lumen.bio/news/barda-funded-intranasal-antibody-cocktail-covid-19-shows-promise-preclinical-study.
- Jester BW, Zhao H, Gewe M, Adame T, Perruzza L, Bolick DT, Agosti J, Khuong N, Kuestner R, Gamble C, et al. Development of spirulina for the manufacture and oral delivery of protein therapeutics. Nat Biotechnol. 2022;40(6):956–64. doi:10.1038/s41587-022-01249-7.
- Tabakh H, Jester BW, Zhao H, Kuestner R, Khuong N, Shanitta C, Takeuchi R, Roberts J. Protocol for the transformation and engineering of edible algae Arthrospira platensis to generate heterologous protein-expressing strains. STAR Protocols. 2023;4:102087. doi:10.1016/j.xpro.2023.102087.
- Markham A. Brolucizumab: first approval. Drugs. 2019;79:1997–2000. doi:10.1007/s40265-019-01231-9.
- GM T, Gauthier CD, Murphy L, Lanser TB, Paul A, Matos KTF, Mangani D, Izzy S, Rezende RM, Healy BC, et al. Nasal administration of anti-CD3 mAb (foralumab) downregulates NKG7 and increases TGFB1 and GIMAP7 expression in T cells in subjects with COVID-19. Proc Natl Acad Sci USA. 2023;120:e2220272120.
- Minenkova O, Santapaola D, Milazzo FM, Anastasi AM, Battistuzzi G, Chiapparino C, Rosi A, Gritti G, Borleri G, Rambaldi A, et al. Human inhalable antibody fragments neutralizing SARS-CoV-2 variants for COVID-19 therapy. Mol Ther. 2022;30(5):1979–93. doi:10.1016/j.ymthe.2022.02.013.
- Hamers-Casterman C, Atarhouch T, Muyldermans S, Robinson G, Hammers C, Songa EB, Bendahman N, Hammers R. Naturally occurring antibodies devoid of light chains. Nature. 1993;363:446–8. doi:10.1038/363446a0.
- Muyldermans S, Cambillau C, Wyns L. Recognition of antigens by single-domain antibody fragments: the superfluous luxury of paired domains. Trends Biochem Sci. 2001;26(4):230–5. doi:10.1016/S0968-0004(01)01790-X.
- Nambulli S, Xiang Y, Tilston-Lunel NL, Rennick LJ, Sang Z, Klimstra WB, Reed DS, Crossland NA, Shi Y, Duprex WP, et al. Inhalable nanobody (PiN-21) prevents and treats SARS-CoV-2 infections in Syrian hamsters at ultra-low doses. Sci Adv. 2021;7. doi:10.1126/sciadv.abh0319.
- Ma H, Zhang X, Zeng W, Zhou J, Chi X, Chen S, Zheng P, Wang M, Wu Y, Zhao D, et al. A bispecific nanobody dimer broadly neutralizes SARS-CoV-1 & 2 variants of concern and offers substantial protection against Omicron via low-dose intranasal administration. Cell Discovery. 2022;8(1):132. doi:10.1038/s41421-022-00497-w.
- Haga K, Takai-Todaka R, Matsumura Y, Song C, Takano T, Tojo T, Nagami A, Ishida Y, Masaki H, Tsuchiya M, et al. Nasal delivery of single-domain antibody improves symptoms of SARS-CoV-2 infection in an animal model. PLoS Pathog. 2021;17(10):e1009542. doi:10.1371/journal.ppat.1009542.
- Wu X, Wang Y, Cheng L, Ni F, Zhu L, Ma S, Huang B, Ji M, Hu H, Li Y, et al. Short-term instantaneous prophylaxis and efficient treatment against SARS-CoV-2 in hACE2 mice conferred by an intranasal nanobody (Nb22). Front Immunol. 2022;13:865401. doi:10.3389/fimmu.2022.865401.
- Titong A, Gallolu Kankanamalage S, Dong J, Huang B, Spadoni N, Wang B, Wright M, Pham KLJ, Le AH, Liu Y, et al. First-in-class trispecific VHH-Fc based antibody with potent prophylactic and therapeutic efficacy against SARS-CoV-2 and variants. Sci Rep. 2022;12(1):4163. doi:10.1038/s41598-022-07952-4.
- Han Q, Wang S, Wang Z, Zhang C, Wang X, Feng N, Wang T, Zhao Y, Chi H, Yan F, et al. Nanobodies with cross-neutralizing activity provide prominent therapeutic efficacy in mild and severe COVID-19 rodent models. Virol Sin. 2023. doi:10.1016/j.virs.2023.07.003.
- Shen H, Cai Y, Zhang H, Wu J, Ye L, Yang P, Lin X, Jiang S, Liao M. Anti-SARS-CoV-2 IgY isolated from egg yolks of hens immunized with inactivated SARS-CoV-2 for immunoprophylaxis of COVID-19. Virol Sin. 2021;36:1080–2. doi:10.1007/s12250-021-00371-1.
- Fan W, Sun S, Zhang N, Zhang Y, Jiao P, Wang J, Gao GF, Liu W, Bi Y, Yang L, et al. Nasal delivery of thermostable and broadly neutralizing antibodies protects mice against SARS-CoV-2 infection. Sig Transduct Target Ther. 2022;7:55. doi:10.1038/s41392-022-00911-5.
- Frumkin LR, Lucas M, Scribner CL, Ortega-Heinly N, Rogers J, Yin G, Hallam TJ, Yam A, Bedard K, Begley R, et al. Egg-derived anti-SARS-CoV-2 immunoglobulin Y (IgY) with broad variant activity as intranasal prophylaxis against COVID-19. Front Immunol. 2022;13:899617. doi:10.3389/fimmu.2022.899617.
- Agurto-Arteaga A, Poma-Acevedo A, Rios-Matos D, Choque-Guevara R, Montesinos-Millán R, Montalván Á, Isasi-Rivas G, Cauna-Orocollo Y, Cauti-Mendoza MDG, Pérez-Martínez N, et al. Preclinical assessment of IgY antibodies against recombinant SARS-CoV-2 RBD protein for prophylaxis and post-infection treatment of COVID-19. Front Immunol. 2022;13:881604. doi:10.3389/fimmu.2022.881604.
- Wouters E, Verbrugghe C, Abdelnabi R, Devloo R, De Clippel D, Jochmans D, De Bleser D, Weynand B, Compernolle V, Neyts J, et al. Intranasal administration of convalescent plasma protects against SARS-CoV-2 infection in hamsters. EBioMedicine. 2023;92:104597. doi:10.1016/j.ebiom.2023.104597.
- Tada T, Minnee J, Landau NR. Vectored immunoprophylaxis and treatment of SARS-CoV-2 infection in a preclinical model. Proc Natl Acad Sci USA. 2023;120:e2303509120. doi:10.1073/pnas.2303509120.
- Gül F, Gonen ZB, Jones OY, Taşlı NP, Zararsız G, Ünal E, Özdarendeli A, Şahin F, Eken A, Yılmaz S, et al. A pilot study for treatment of severe COVID-19 pneumonia by aerosolized formulation of convalescent human immune plasma exosomes (ChipEXO™). Front Immunol. 2022;13:963309. doi:10.3389/fimmu.2022.963309.
- Focosi D, Casadevall A. A critical analysis of the use of cilgavimab plus tixagevimab monoclonal antibody cocktail (Evusheld™) for COVID-19 prophylaxis and treatment. Viruses. 2022;14(9):14. doi:10.3390/v14091999.