ABSTRACT
The continuous evolution of severe acute respiratory syndrome coronavirus 2 (SARS-CoV-2) variants poses a challenge to determine the optimal updated composition of the coronavirus disease 2019 (COVID-19) vaccine. The present study aimed to investigate the immunogenicity of the Delta monovalent vaccine, the Omicron monovalent vaccine, and the Delta and Omicron BA.1 bivalent vaccine. Three COVID-19 vaccines were designed using the heterologous DNA prime-protein boost strategy, with each vaccine containing either Delta receptor-binding domain (RBD) of the spike protein, Omicron RBD, or both Delta and Omicron antigens. Temporal serum antibody binding titers and neutralizing antibody titers induced by the three vaccines in New Zealand White rabbits were analyzed. To further dissect the vaccine elicited antibodies (mAb) responses at the molecular level, a panel of rabbit monoclonal antibodies (RmAbs) was generated by a high-throughput single B cell sorting and discovery pipeline and further comprehensively characterized. The Omicron monovalent vaccine induced higher antibody binding titers and neutralization activities than the Delta and Omicron bivalent vaccine. Four RmAbs with robust neutralization capacity were isolated from rabbits immunized with the Omicron or Delta monovalent vaccine. Notably, 9E11 isolated from the Omicron monovalent vaccine group neutralized all the Omicron subvariants with an IC50 value ranging from 1.5 to 503.6 ng/mL; thus, this vaccine could serve as a prophylactic and therapeutic intervention. Given the increasing incidence of COVID-19 cases due to the Omicron variant, RBD from the Omicron strain could serve as a candidate immunogen that can induce higher neutralization activities against the SARS-CoV-2 Omicron sublineages.
Introduction
The rapid global transmission of severe acute respiratory syndrome coronavirus 2 (SARS-CoV-2) poses an unprecedented global threat to public health. In late 2020, India reported the first case of the B.1.617.2 (Delta) strain of SARS-CoV-2, which was classified as a variant of concern (VOC) on May 11, 2021 by the World Health Organization (WHO) and spread worldwide in only 3 months.Citation1,Citation2 In November 2021, another SARS-CoV-2 VOC, namely the Omicron variant (B.1.1.529), was first identified in Botswana and spread globally. The Omicron variant is unique in that its spike glycoprotein carries over 30 mutations.Citation3–7 The Omicron variant has continuously evolved since the end of 2021, leading to the rapid, simultaneous evolution of multiple sublineages. Different from other VOCs, the Omicron variant and its sublineages exhibit increased transmissibility and immune evasion from the neutralizing antibodies (nAbs) generated through previous infection or vaccination and have caused numerous reinfections and breakthrough infections. The expanding sublineages of the Omicron variant, such as BA.2, BA.2.12.1, BA.2.75, BA.4, and BA.5, have been the source of a series of viral infection peaks; these sublineages are similar to but spread faster than the BA.2 lineage.Citation8–13 The circulating SARS-CoV-2 strain has become more diverse because of the BA.4/BA.5 and BA.2.75 subvariants, resulting in several new subvariants such as BA.4.6, BF.7, BQ.1, and BQ.1.1 (derived from BA.4/BA.5) as well as BA.2.75.2 (derived from BA.2.75). These new subvariants are now becoming more prevalent and could be the next significantly dominating Omicron subvariant.Citation14,Citation15
Coronavirus disease 2019 (COVID-19) vaccine is a safe and critical intervention to afford high levels of protection against the severe and fatal disease caused by SARS-CoV-2 infection. However, the continuous and remarkable evolution of SARS-CoV-2, exemplified by Omicron sublineages, has raised serious concerns regarding whether the COVID-19 vaccines developed using the ancestral strain sequence could reduce vaccine effectiveness against COVID-19 caused by the new subvariants. Currently, several bivalent vaccines directed against the ancestral strain and the Omicron strain such as BA.1 or BA.4/BA.5 have been approved as booster vaccines in the United States.Citation16,Citation17 However, compared to monovalent boosters, bivalent boosters did not appreciably improve neutralization capacity and T cell responses.Citation18,Citation19 Nevertheless, these observations were from the populations vaccinated with COVID-19 vaccine composed of the ancestral strain, and prior immune imprinting might affect the immunogenicity of bivalent boosters.
The immunogenicity of the receptor-binding domain (RBD) immunogen derived from different SARS-CoV-2 variants has not been comprehensively compared. Therefore, in the present study, we investigated the immunogenicity of monovalent Delta RBD, monovalent Omicron BA.1 RBD, and bivalent Delta and Omicron BA.1 RBD by using heterologous DNA prime-protein boost immunization strategy. In this approach, the DNA vaccine is delivered as the priming immunization, followed by a boost with protein antigens as recombinant proteins.Citation20,Citation21
Neutralizing monoclonal antibodies have been increasingly used as passive antiviral reagents in prophylactic and therapeutic interventions and to guide the design of viral vaccines, including COVID-19 vaccines.Citation22–25 However, Omicron sublineages, particularly BA.4/BA.5, BQ.1.1, and XBB, exhibited a high level of immune evasion for the currently authorized therapeutic rabbit monoclonal antibodies (RmAbs).Citation5 Therefore, it is critical to develop potent monoclonal antibodies (mAbs) against the broad-spectrum Omicron subvariants. In our previous study, several potent neutralizing RmAbs were generated by a novel COVID-19 vaccine using the DNA prime-protein boost strategy, and one of these RmAbs could neutralize Omicron subvariants, including BA.1, BA.2, BA.3, and BA.4/BA.5.Citation26–28 To further analyze the nAb responses induced by the three vaccines at the molecular level, neutralizing RmAbs were isolated, and their binding capacity, neutralization potency, binding affinity, and germline genetic modification were comprehensively characterized.
Materials and methods
Construction of the DNA vaccine
The SARS-CoV-2 DNA vaccine comprising the RBD domain and DNA bullet preparation was constructed as previously described.Citation29 The pcDNA3.4 expression vector was used to clone the codon-optimized Delta RBD and Omicron RBD regions. The Qiagen Plasmid Mega kit (Cat #: 10023) was used to purify the expression construct after its expansion in the Escherichia coli DH5 strain. Next, 100 μL of 100 mg/mL gold powder (Alpha Aesar, Cat #: 39817) precoated with 100 mg/mL spermidine (Sigma, Cat #: S2626) was further coated with 40 μg of the pure expression construct. The process of coating gold with DNA was simplified by gradually adding 200 μL of 2.5 M CaCl2 to the gold powder coated with DNA; the powder was then rinsed in 100% ethanol before loading into bullet tubes mounted on the bullet maker (Scientz Scientific). After drying under slow N2 flow at 0.1 MPa for 10 min, the bullet tubes filled with the gold powder were sliced into DNA bullets.
Protein vaccine preparation
Recombinant SARS-CoV-2 RBD protein (Cat #: 40592-V08B, Sino Biological) and SARS-CoV-2 B.1.617.2 RBD protein (Cat #: 40592-V08H90, Sino Biological) were purchased for immunization. SARS-CoV-2 Delta spike protein (Cat #:40592-V08H91, Sino Biological) and Omicron spike protein (B.1.1.529) (Cat #: 40589-V08B33, Sino Biological) were used to prepare spike-specific probes for single B cell sorting.
SARS-CoV-2 (B.1.1.529) spike protein fused with His tag (Vazyme, Cat #: CG235), SARS-CoV-2 (BA.5) spike protein (Vazyme, Cat #: CG236), and spike proteins of the related variants (BQ.1, BQ.1.1, and XBB.1) were obtained for ELISA. His tag-fused SARS-CoV-2 RBD protein (GenScript, Cat #: Z03479), His tag-fused SARS-CoV-2 B.1.1.529 RBD protein (GenScript, Cat #: Z03730), and the related RBD variants (BA.2 RBD, BA.5 RBD, and Delta RBD) were used for ELISA.
Rabbit immunization strategy
The research protocol was approved by the Ethics Committee of the Nanjing Drum Tower Hospital on Animal Use (Protocol No. 2020AE01120). Nine New Zealand white rabbits were assigned to three groups (three rabbits per group) and immunized with either Delta RBD, Omicron BA.1 RBD, or Delta and Omicron RBD immunogen simultaneously. The heterologous DNA prime-protein boost strategy was used in the animal study. First, DNA immunization with 30 μg Omicron RBD or Delta RBD for monovalent vaccine, or a combination of 15 μg Omicron RBD and 15 μg Delta RBD for bivalent vaccine was administered by DNA bullet under the abdomen On days 0, 7, and 21. All rabbits were then administered a protein booster dose with 100 μg Omicron RBD or Delta RBD (for monovalent vaccine) or a combination of 50 μg Omicron RBD and 50 μg Delta RBD (for bivalent vaccine) on days 35 and 56 through a multipoint subcutaneous injection (S.Q.) on both sides of the spine. Two weeks later, three rabbits with an optimal immune response were intravenously(i.v.) administered 400 μg of the corresponding antigen as the final booster dose. Sera samples during the prime and boost phases were collected on days 0, 28, 42, and 63. The spleens of the rabbits were collected 5 days after the final booster dose.
Serum binding assay
ELISA was performed for determining SARS-CoV-2-specific binding titers for the immunized sera samples.Citation28 Briefly, 96-well binding plates (Corning, Cat. #: 42592) were filled with 100 μL of RBD or spike protein of SARS-CoV-2 variants at 0.5 µg/mL and incubated overnight at 4°C. The plates were blocked with 200 μL of blocking buffer (15% skimmed milk and bovine serum albumin [BSA] in 1× PBS) and rinsed five times with washing buffer containing 1 × PBS supplemented with 0.1% Tween-20 (Sigma, Cat #: P9416). Three-fold serially diluted rabbit serum samples starting at 1:3000 were added to the wells, and the plates were then incubated for 1 h. Subsequently, the plates were incubated with anti-rabbit IgG labeled with horseradish peroxidase (HRP) (Jackson ImmunoResearch, Cat #: 111-035-045) in blocking buffer at 1:10000 dilution for 1 h at 37°C. The plates were incubated with 100 μL of TMB substrate solution (Moss Inc., Cat. #: TMBHK-1000) for 3 min in the dark, followed by washing for five times. Next, 1 M H2SO4 was added to terminate the reaction. The Epoch microplate spectrophotometer (Thermo, USA) was used to determine the optical density (OD) values at 450 nm. Rabbit serum samples before immunization were used as the control, and the reciprocal of the maximum dilution was calculated as the serum titer.
ACE2 receptor blocking assay
Briefly, 96-well plates were coated with human ACE2-Fc (1 μg/mL) at the volume of 100 μL/well and incubated overnight at 4°C. The plates were then blocked with 200 μL of blocking buffer (15% skimmed milk and BSA in 1× PBS) and rinsed five times with washing buffer containing 1× PBS supplemented with 0.1% Tween-20 (Sigma, Cat #: P9416). The B cell culture medium was first diluted at the ratio of 1:2, followed by 3-fold serial dilution, and mixed with 0.1586 μg/mL (final concentration) of Omicron-spike-biotin or Delta-spike-biotin for 1 h at room temperature. For final detection, HRP-conjugated neutravidin (Thermo, Cat #: 31001) at 1:10000 dilution was added for 1 h at room temperature, followed by washing for five times. The plates were then incubated with 100 μL of TMB substrate solution (Thermo Fisher, Cat. #: 34029) for 3 min in the dark. Next, 1 M H2SO4 was added to terminate the reaction. The Epoch microplate spectrophotometer (Thermo, USA) was used to determine the OD values at 450 nm.
Pseudovirus neutralization assay
HEK293-ACE2 cells were seeded on 96-well plates at the density of 2 × 104 cells/well. SARS-CoV-2 pseudoviruses at the titer of 1.3 × 104 TCID50 were then incubated with serially diluted RmAbs for 1 h at 37°C. Cell control and virus control were included as the control groups. The culture medium in the 96-well plate was refreshed at 24 h. Luminescence was measured after 48 h of incubation at 37°C and 5% CO2 by using the luciferase substrate (One-Lite Luciferase Assay System, Vazyme, Cat. #: DD1203–01). Relative light units (RLUs) were measured by the luminescence detection instrument (Infinite E plex, Tecan). After subtracting the background RLUs from the control groups with cells alone, the half-maximal inhibitory concentrations (IC50) of the RmAbs were calculated as the concentration of RmAbs at which the RLUs were reduced by 50% as compared to that for the control wells.Citation30
Construction of fluorescent spike-specific protein probes
SARS-CoV-2 Delta spike protein and Omicron BA.1 spike protein were incubated with EZ-Link NHS-PEG4-Biotin (Thermo Scientific, Cat. #: 21362) for 6 h on ice. The mixture was transferred to a dialysis unit (Thermo Scientific, Cat. #: 69576) and dialyzed overnight at 200 rpm in sterile PBS. The biotin-spike protein and APC streptavidin (BD Pharmingen, Cat. #: 554067) were then incubated for 1 h at room temperature to construct fluorescent spike-specific protein probes. Spike-specific single B cells were then sorted.
Isolation of spike-specific single B cells from rabbit splenocytes
Rabbit spleens were collected on day 7 after the final booster dose. Single splenocytes were produced as a single-cell suspension in PBS with 2% fetal bovine serum and 1 mM EDTA. Single splenocytes were then sorted using the fluorescent spike probes and grown overnight in B cell culture medium (Yurogen Biosystems, Wuhan, China). The splenocytes were then processed using the single B cell SMab® platform (Yurogen Biosystems). By using FACS Aria III (BD Biosciences, USA), single sorted B cells were further sorted into 96-well plates, with one cell per well, and maintained in a specialized rabbit B cell complete medium for 10–14 days at 37°C and 5% CO2.
Generation of RmAbs
Spike-specific B cell clones were identified by screening B cell culture supernatants against RBD or spike protein through ELISA. B cell clones with OD450 values higher than five times the background noise were defined as positive B cell clones. By using RT-PCR, the variable area of the IgG heavy and light chains was retrieved from the top positive clones. The complete heavy and light IgG chains from each clone were co-transfected into HEK293T cells. The recombinant rabbit IgG-containing supernatants from the transfected HEK293T cells were collected, and their capacity to bind with spike and RBD was determined by ELISA. To produce antibodies in HEK293T cells, the variable sections of PCR fragments from the selected clones were cloned into the pcDNA3.4 vector. Protein A chromatography was used to purify recombinant RmAbs for further analysis.
Biolayer interferometry analysis
Biolayer interferometry (BLI) analysis was performed to determine the binding kinetics of RmAbs to the spike proteins of the SARS-CoV-2 variants by using GatorPrime Label-free Bioanalysis instrument (Gator Bio, Palo Alto, CA, USA). Briefly, the protein A probe was spun at 1000 rpm in K buffer. For antibody binding to a height of approximately 1.2 nm, the probe was spun at 1000 rpm while keeping it submerged in a diluted antibody solution. The probe was then spun again at 1000 rpm in K buffer to remove any residual antibodies. Subsequently, the probe was submerged in the diluted antigen solution and spun at 1000 rpm, causing the antibody linked to the probe to bind to various antigen concentrations; the antigen-antibody binding curve was simultaneously monitored. Finally, the probe was submerged in K buffer and spun at 1000 rpm to monitor the antigen-antibody dissociation curve. Following the fitting of the curve, the dissociation constant (KD) value was calculated after the background was removed.Citation27
Statistical analysis
Data were analyzed using GraphPad Prism version 8 software and SPSS version 22.0 software. One-way ANOVA was used to compare the mean values among the three groups. An unpaired t-test was used to compare the mean values between two groups. Pearson’s correlation analysis was used to analyze the correlation between binding capacity and neutralization potency. A p-value of < .05 was considered significant.
Results
Monovalent Delta or Omicron vaccine induced a higher level of antibody response than the bivalent vaccine
To determine the immunogenicity of bivalent and monovalent COVID-19 vaccines, we designed monovalent and bivalent vaccines composed of Delta or Omicron RBD antigen by using the highly immunogenic heterologous DNA prime-protein boost immunization strategy.Citation31 In the first two groups, rabbits were immunized with a DNA vaccine containing either Delta or Omicron RBD region followed by the corresponding protein, while in the third group, the rabbits were immunized with two DNA vaccines containing either Delta or Omicron RBD region and heterologously primed with bivalent protein vaccines containing both Delta and Omicron RBD proteins ().
Figure 1. Humoral responses induced by monovalent Omicron, monovalent Delta, and bivalent Omicron and Delta vaccines. (a) Immunization strategy for the immunization of rabbits. Rabbits received three doses of the DNA vaccine with different antigens on days 0, 7, and 21 and two additional doses of the S1 protein vaccine on days 35 and 49. Sera were collected on days 0, 28, and 56 for immunoassay. (b) Mean serum IgG titer curves of the three groups of rabbits over time. T0: before immunization; T3: day 28 post immunization; T4: day 42 post immunization; T5: day 63 post immunization. (c) Temporal IgG titer of rabbit serum against RBD and spike proteins measured by ELISA in the three animal groups immunized with either Delta, Omicron, or Delta and Omicron. (d) SARS-CoV-2 pseudovirus neutralization assay of rabbit serum at T5 against WT; Delta; and Omicron BA.1, BA.1(R346K), BA.2, BA.4/BA.5, and BA.2.75.
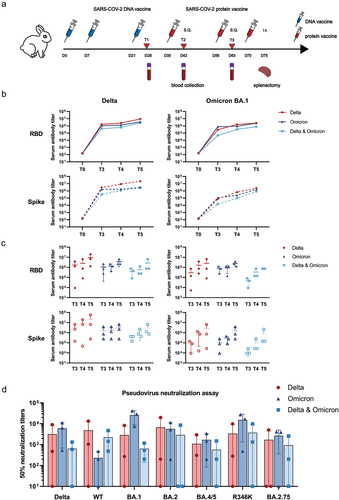
Antibody titers were substantially increased after three immunization sessions with the DNA vaccine and further immunization with booster doses of two protein vaccines (). Among the three vaccine groups, the Delta monovalent vaccine group induced anti-Delta RBD-binding IgG antibodies with a geometric median titer (GMT) of 5.46 × 106 (95% CI: 3.40 × 106-7.53 × 106), whereas the GMT of the anti-Delta RBD-binding IgG in the Omicron monovalent vaccine group was 3.15 × 106 (95% CI: 2.60 × 106-3.70 × 106) and that of the anti-Delta RBD-binding IgG in the bivalent vaccine group was 1.52 × 106 (95% CI: 7.83 × 105-2.25 × 106) (). In contrast, the GMT of the anti-Omicron RBD-binding IgG in the Delta monovalent vaccine group was 7.29 × 105 (95% CI, −4.82 × 104 to 1.51 × 106), whereas the GMT of the anti-Omicron RBD-binding IgG was 2.19 × 106 (95% CI, 1.91 × 106-2.47 × 106) and 7.29 × 105 in the Omicron monovalent vaccine group and the bivalent vaccine group, respectively (). Our findings showed that compared to immunization with Delta or Omicron RBD alone, immunization with the bivalent vaccine did not induce higher serum antibody binding titers as expected (). A similar tendency was noted in the binding assay against Omicron BA.2, BA.3, and BA.5 spike proteins (Figure S1). This indicated that the Delta RBD immunogen induced the highest antibody titer among the three vaccine groups.
We then compared the neutralization potency of serum samples extracted from the three immunization groups against wildtype (WT), Delta, and Omicron BA.1, BA.2, BA.4/BA.5, BA.1(R346K), and BA.2.75 strains (). The bivalent vaccine group showed potent neutralizing activity against the WT strain, with a geometric titer of 1484.11 (1484.11 ± 2208.34) as compared to the Omicron RBD group (98.79 [98.79 ± 237.52]) (p = .03) and the Delta RBD group (1406.13 [1406.13 ± 7339.34]) (p = .483). Furthermore, compared to the Delta monovalent group and the bivalent group, the Omicron monovalent group exhibited significantly higher neutralization capacity against the variants Delta (GMT: Delta monovalent vs. bivalent vs. Omicron monovalent: 349.57 vs. 197.66 vs. 3350.24), Omicron BA.1 (GMT: 280.70 vs. 501.03 vs. 20650.61), BA.2 (GMT: 341.23 vs. 95.07 vs. 2283.70), BA.4/BA.5 (GMT: 208.21 vs. 144.18 vs. 998.01), BA.1(R346K) (GMT: 301.48 vs. 506.38 vs. 6080.64), and BA.2.75 (GMT: 79.58 vs. 172.72 vs. 541.73). The bivalent group also induced substantially lower nAb titers than the Omicron monovalent group against Omicron BA.1, BA.2, BA.5, BA.1(R346K), and BA.2.75 (501.03 vs. 20650.61, 95.07 vs. 2283.70, 144.18 vs. 998.01, 506.38 vs. 6080.64, and 172.72 vs. 541.73, respectively). Thus, our results showed that the Omicron RBD immunogen had strong immunogenicity against the Omicron sublineages but modest immunogenicity against the WT strain.
Isolation of RmAbs from spike-specific B cells
To further characterize the humoral responses induced by the three vaccines at the molecular level, Omicron or Delta spike-specific single B cells from immunized rabbit splenocytes were isolated by flow cytometry (). A total of 1096 Delta spike-positive B cells and 1208 Omicron spike-positive B cells were collected. The B cells were then cultured in the rabbit B cell complete medium for 7 days, and the culture supernatants were collected for selection. We identified 802 positive B cell clones showing potent binding capability with the spike protein, including 397 B cells isolated with the Delta spike probe and 405 B cells isolated with the Omicron spike probe (all OD values > 0.7) (Table S1) (). Next, we examined the cross-reactivity of these positive clones for the Delta and Omicron spike proteins. Of the 288 positive clones, 277 could bind to both Omicron and Delta spike proteins (Table S1). Furthermore, 85 B cell clones were identified as strong ACE2 blockers among the 288 clones (Table S2). Among these 85 strong ACE2 blockers, 21 clones were further selected based on their binding and blocking capability to the Delta and Omicron spike proteins (). Thirteen of the 21 clones with high binding capability were selected based on their binding capacity, ACE2 blocking, and binding affinity. These top 13 clones showed good recognition of the Omicron and Delta spike proteins (), whereas the 1B11, 1H4, and 2C9 clones could not block the ACE2 activity (). BLI analysis showed that the supernatants of clone 9E11 with ACE2 blocking ability showed the most cross-reactive binding affinity to all five SARS-CoV-2 RBD proteins (Table S3). The supernatants from the top 13 clones were also tested for neutralization against Omicron BA.1. Nine of these clones showed high neutralization capacity against BA.1 (). Because clones 18A3 and 17H4 did not appear to bind to the spike proteins of BA.2 and BA.5 (Table S3), the top seven neutralizing RmAb clone candidates were identified as follows: 1H4, 1B12, 1A5, 2C9, 9E11, 9H10, and 10D7.
Figure 2. Isolation of spike-specific single B cells and functional testing of B cell clones. (a) Flow cytometry gating strategy to sort spike-specific B cells from rabbit splenocytes. (b) Summary of sorted single B cell clones, Delta spike-binding clones, Omicron BA.1 spike-binding clones, and RBD/ACE2 blocking clones. (c) Determination of the binding ability of Omicron and Delta spike-specific B cell clones from the culture supernatants by ELISA. (d) Detection of the ACE2 blocking capacity of spike-specific B cell clones from the culture supernatants by blocking ELISA. (e) Neutralization capability of spike-specific B cell clones from the culture supernatants at 50 ng/mL. (f) Venn diagram of the classification of the 21 clones.
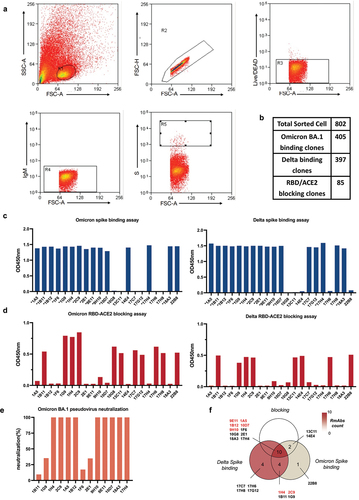
Binding properties of four RBD-specific RmAbs with spike and RBD proteins
We next determined the binding capacity of the isolated RmAbs against the SARS-CoV-2 spike and RBD proteins, which were further divided into three groups. In the first group, three RmAbs, namely 1H4, 2C9, and 9H10, showed a strong binding ability to the spike proteins of WT, Delta, and Omicron sublineages (). In the second group, 1A5, 1B12, and 10D7 bound strongly to WT spike, Delta spike, and the spike proteins of the Omicron sublineages BA.1, BA.2, and BA.3 but bound weakly to spike proteins of the Omicron sublineages BA.5, XBB.1, BQ.1, and BQ.1.1. The third group containing 9E11 showed higher binding to the spike proteins of WT, Delta, BA.1, BA.2, BA.3, and BA.5 than to the spike proteins of BQ.1 and BQ.1.1; however, it did not show binding to the spike protein of XBB.1 (). Intriguingly, RmAbs demonstrated a distinct binding profile with RBD proteins. RmAbs had a similar binding capacity for the RBD of WT, Delta, and BA.1 (); however, 1A5 and 10D7 clones were weakly bound to BA.2 RBD and failed to recognize the RBD proteins of BA.3 and BA.5. Clone 1B12 showed weaker binding to the RBD proteins from BA.2 and BA.5 than to the RBD proteins from WT, Delta, and BA.1 variants and also failed to recognize BA.3 RBD. Nevertheless, 1H4, 2C9, 9H10, and 9E11 clones showed a strong binding capacity to the most tested RBDs from WT, Delta, BA.1, BA.2, and BA.5, except that 9E11 and 9H10 failed to bind to BA.3 RBD (). Regarding binding capacity, 1H4 and 2C9 showed the best performance with the recognition of all the tested proteins, followed by 9H10 and 9E11. Clones 1A5, 1B12, and 10D7 showed relatively weak binding ability ().
Figure 3. Profiles of RmAbs binding with SARS-CoV-2 spike and RBD proteins from various subvariants. (a) RmAbs that bound to the SARS-CoV-2 spike protein from WT; Delta; and Omicron BA.1, BA.2, BA.3, BA.5, XBB.1, BQ.1, and BQ.1.1 were identified by ELISA. (b) RmAbs that bound to the SARS-CoV-2 RBD protein from WT; Delta; and Omicron BA.1, BA.2, BA.3, and BA.5 were identified by ELISA. (c) Heatmap of the binding capacity of seven RmAbs against the spike and RBD proteins of WT, Delta, and Omicron and its variants. The value represents the OD value of ELISA at 1 μg/mL concentration.
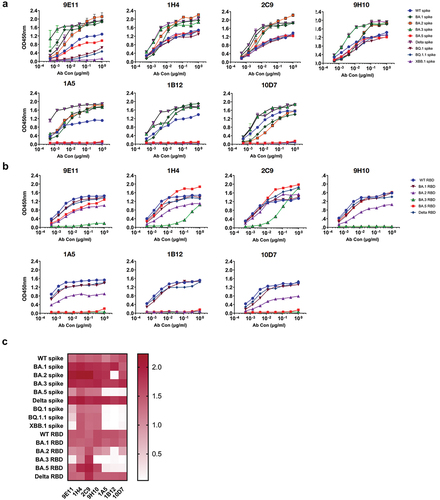
BLI analysis was used to measure the KD values of the top four most potent RmAbs against the spike proteins of the SARS-CoV-2 ancestral strain and variants. Clone 9E11 isolated from the Omicron RBD vaccine group showed a wide binding capability, with a high binding affinity to the spike proteins of BA.1, BA.2, BA.3, BA.5, BF.7, BQ.1.1, and WT (KD < 1 × 10−10 M) () and a strong affinity to the spike proteins of XBB.1 and BQ.1 (KD: 1.26 × 10−9 to 5.78 × 10−9M) (). Clone 10D7 showed a comparable binding affinity to the spike proteins of WT and Omicron BA.1, BA.2, and BA.3, with KD values ranging from 1.42 × 10−9 M to 1.57 × 10−10 M; however, it showed an impaired binding ability to the spike protein of BA.4/BA.5 with a KD value of 2.33 × 10−7 M and lost its binding ability to the spike proteins of XBB.1 and BQ.1 (Figure S2). Clone 1A5 showed a strong binding affinity to the spike proteins of BA.1, BA.2, BA.3, and BF.7, with KD values ranging from 9.66 × 10−10 M to 4.73 × 10−11 M, and a relatively weaker affinity to the spike proteins of BA.5, BQ.1, and WT, with KD values ranging from 2.88 × 10−8M to 1.18 × 10−9 M. However, 1A5 did not show binding to the spike and RBD proteins of both XBB.1 and BQ.1.1 (Figure S3). Clone 1B12 could easily recognize the spike proteins of BA.3, BA.1, WT, BQ.1.1, and BQ.1 (KD = 4.42 × 10−11 M, 4.64 × 10−11 M, 2.23 × 10−10 M, 7.77 × 10−10 M, and 5.55 × 10−8M, respectively), but failed to recognize the spike proteins of BA.2, BF.7, BA.4/BA.5, and XBB.1 (Figure S4).
Neutralization potency of RmAbs induced by the COVID-19 vaccine against SARS-CoV-2 variants
To evaluate the neutralization ability of RmAbs, pseudovirus neutralization assay was performed against SARS-CoV-2 WT and Omicron subvariants. To determine the neutralization capacity of seven RmAbs, the neutralization percentage of RmAbs was calculated at the fixed concentration of 50 ng/mL (). The supernatant of the top four RmAbs with a high neutralization capacity, including 9E11, 1A5, 1B12, and 10D7, which could neutralize > 25% of pseudovirus against WT and Omicron sublineages, were further tested. All four RmAbs showed highly effective neutralization potency against WT, BA.1, BA.1(R346K), BA.2, and BA.2.75 with comparable IC50 values ranging from 0.75 to 39.4 ng/mL. However, clone 10D7 showed worse potency in neutralizing BA.1 (IC50 = 265.3 ng/mL) and BA.2 (IC50 = 141.2 ng/mL), whereas 1B12 failed to neutralize BA.2 and BA.2.75. All RmAbs showed significantly impaired neutralization activity against BA.5. The IC50 value of 9E11 against BA.5 was reduced by more than 10-fold as compared to the other variants, while the remaining three RmAbs could not neutralize BA.5 at 1 μg/mL concentration; this finding indicated that the Omicron BA.5 sublineage showed remarkable immune escape. A notable finding was that 9E11 could neutralize all the tested SARS-CoV-2 strains, with superior capability to neutralize BA.2, BA.5, and BA.2.75 with IC50 values of 3.80, 61.7, and 1.47 ng/mL, respectively (). Next, we examined the neutralization potency of the above-mentioned four RmAbs against the alternative emerging subvariants, including BF.7, BQ.1, BQ.1.1, XBB, XBB.1, and BA.2.75.2. Consistent with previous findings, 9E11 could neutralize BF.7, BQ.1, and BQ.1.1 with IC50 values of 25, 438.5, and 503.6 ng/mL, respectively; however, their neutralization capability was lost against XBB, XBB.1, and BA.2.75.2 (IC50 >10 μg/mL). Furthermore, clones 10D7, 1A5, and 1B12 showed substantially lower neutralization capability against the above-mentioned circulating variants (Figure S5a,c). The correlation analysis showed that the binding affinity of RmAbs was associated with the IC50 value of their neutralization potency, thus suggesting that the high binding affinity of mAbs is critical for the acquisition of potent neutralizing activities (r = 0.444, p = .03) (Figure S5b)
Figure 5. Neutralization capability of the identified RmAbs determined by pseudovirus neutralization assay. (a) the neutralization capability of seven RmAbs at 50 ng/mL against WT and Omicron BA.1, BA.2, BA.5, BA.2.75, and BA.1.1. (b) Pseudovirus neutralization assay of four RmAbs (9E11, 10D7, 1A5, and 1B12) against SARS-CoV-2 WT and Omicron BA.1, BA.2, BA.5, BA.2.75, and BA.1.1. The curves represent the inhibition rate of the SARS-CoV-2 pseudovirus entry into HEK293T cells overexpressing ACE2.
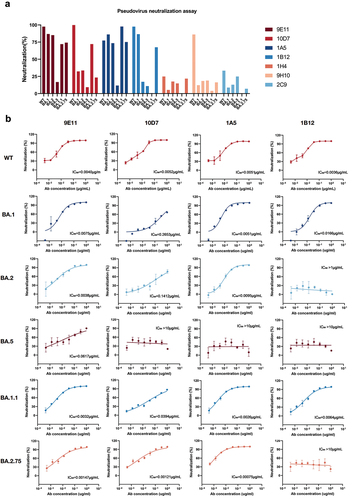
Germline gene analysis of RmAbs
To characterize the genetic features of our identified RmAbs induced by the vaccines, we analyzed the heavy and light chains using the IMGT/V-QUEST program (http://www.imgt.org/IMGT_vquest/vquest). Germline family usage, mutation frequency, and complementarity-determining region 3 (CDR3) length in the heavy and light chains were determined. Key residues in the immunoglobulin heavy chain genes responsible for antigen recognition were found to be germline-encoded.Citation32,Citation33 Our results showed that 9E11 used IGHV1S45 × 01F, 1A5 and 1B12 used IGHV1S69 × 01F, and 10D7 used 1S40 × 01F 1H4 as the germline heavy chain (). The germline family usage of the light chain variable regions (IGKV) of RmAbs was more diverse. The IGHV of 9E11, the most potent RmAb, harbored high somatic hypermutation (SHM) with a frequency of 21.18%, while the other three neutralizing RmAbs showed an SHM frequency of 6–9%. The SHM frequency of IGKV ranged from 6.81% to 11.47% (). The CDR3 length of the heavy chain is also a critical parameter for antigen recognition. In the present study, the CDR3 length of the top four RmAbs ranged from 11 amino acids (aa) to 19 aa.
Figure 6. Genetic analysis of the isolated RmAbs. (a) Mutations in the variable regions, joining regions, and CDR3 regions of the heavy and light chains of RmAbs compared to their germline gene sequences. (b) a phylogenetic tree was constructed using the maximum-likelihood method for the heavy chain (above) and light chain (below) of seven neutralizing RmAbs. Branch lengths are scaled to visualize sequence diversification.
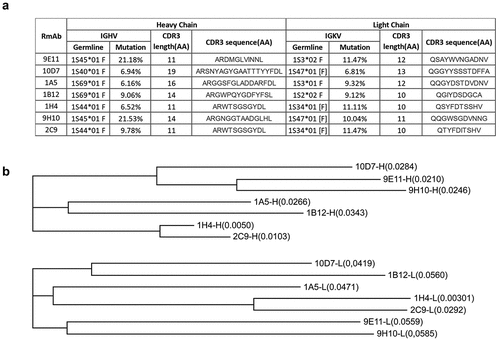
To determine the genetic relationship of the isolated RmAbs, a phylogenetic tree was constructed using the maximum-likelihood method. The top four neutralizing RmAbs, i.e., 10D7, 9E11, 1A5, and 1B12, appeared to form an independent subtree, thus indicating that RmAbs with potent neutralizing activities might evolve through a similar process of B cell lineage development and affinity maturation ().
Discussion
Although COVID-19 is no longer a public health emergency of international concern, it still poses a significant threat to global population health. The emergence of the highly contagious Omicron variant has increased the incidence of breakthrough infection even after the third dose of the COVID-19 vaccine.Citation34 Moreover, COVID-19-positive patients in the convalescent stage show long-term health consequences of the infection, including fatigue or muscle weakness, sleep difficulties, anxiety, and depression.Citation35 Therefore, it is critical to develop COVID-19 vaccines with an optimal composition that can induce broad-spectrum neutralizing responses against a diverse range of SARS-CoV-2 variants.
The immune system of rabbits is similar to the human immune system, and the genetic diversity between humans and rabbits is obviously lower than between humans and mice. The rabbit has also been wildly utilized as a reliable animal model during the study of infectious diseases.Citation36–38 Therefore, to some extent, the immune responses of rabbits to SARS-CoV-2 antigens resemble that of humans. Additionally, the clinical trial in human also confirmed that boosting with bivalent mRNA vaccines did not elicit superior immune responses as compared with boosting with the original monovalent vaccines.Citation16,Citation18
The optimal vaccine composition that can induce cross-protective humoral responses remains elusive. According to previous studies, Omicron sublineages show remarkable immune evasion in individuals with previous SARS-CoV-2 infection or a history of vaccination with the WT or Delta strain.Citation39 The antigenicity of Omicron BA.1 is also distinct from that of WT and Delta strains.Citation40 In the present study, we compared the immunogenicity of Delta RBD, Omicron RBD, and bivalent Delta and Omicron RBD through the DNA prime-protein boost immunization strategy. Our findings revealed that compared to the bivalent vaccine, the monovalent Omicron or Delta RBD elicited more potent serum binding antibodies and nAbs against Delta and Omicron sublineages. Therefore, Omicron BA.1 is likely to serve as a superior immunogen that could potentially provide an improved level of defense against Omicron sublineages circulating in the future. Interestingly, the bivalent vaccine did not show any detectable benefits in the immune response as compared to the monovalent vaccine. Consistent with our findings, a previous study showed that the sequential administration of an mRNA-based immunogen derived from distinct SARS-CoV-2 variants led to long-lasting, high antibody titers as compared to the co-immunization of distinct subtypes of mRNAs simultaneously.Citation41 Moreover, a relatively long interval between the two doses of mRNA vaccines could further enhance cellular and nAb responses.Citation42 Therefore, we speculate that the low antibody response in the bivalent vaccine group might be due to the suboptimal antigen delivery sequence and the interval of exposed antigens.
Our study also compared the immunogenicity between Omicron RBD and Delta RBD. The results showed that the Omicron vaccine group induced a significantly higher level of neutralizing activities against Omicron sublineages than the Delta vaccine group, although the former group had lower specific antibody binding titers than the latter group. We also further investigated the antibody responses by isolating two potent nAbs induced by the Omicron vaccine group, which could cross-neutralize against most Omicron sublineages. Thus, given the increasing incidence of the Omicron subvariants, RBD from Omicron could be a candidate immunogen that induced improved neutralizing activity against the SARS-CoV-2 Omicron sublineages. Our findings emphasize the importance of the composition of the specific variants used in the vaccine for immunization, which will have a high impact on the immune imprinting of humoral responses.
Nevertheless, the potential limitation for Omicron monovalent vaccine is that the vaccine-induced immune responses might be only protective for the Omicron sublineages, but not for the emerging subvariants with large amount of key residue mutation such as recently EG.5.Citation43,Citation44 Nevertheless, our data also showed that bivalent vaccines also induce limited cross-reactive immune responses. Therefore, novel vaccine implementation strategy shall be developed to induce broad and potent high-quality antibody responses.
Our study also discovered a panel of RmAbs induced by the vaccines, in which 9E11, 10D7, and 9H10 were derived from the monovalent Omicron group and 1A5, 1B12, 2C9, and 1H4 were identified from the monovalent Delta group. The RmAb 9E11 induced by the Omicron RBD vaccine recognized spike proteins with binding affinity in the picomolar range. 9E11 also neutralized all the nine SARS-CoV-2 variants tested, including WT and Omicron BA.1, BA.1.1, BA.2, BA.4/BA.5, BA.2.75, BF7, BQ.1, and BQ.1.1. 10D7. Another Omicron RBD-induced RmAb, also showed cross-reactivity against the spike proteins of Omicron BA.1, BA.2, BA.3, and BF.7, Delta, and WT, failed to recognize the spike proteins of BA.4/5, BQ.1.1, BQ.1, and XBB.1. Our findings indicate that the Omicron immunogen could induce broad humoral responses that showed cross-reactivity with the majority of Omicron sublineages. Moreover, 1A5 and 1B12, which were induced by the Delta RBD immunogen, had a narrow recognition ability for Omicron sublineages, thus suggesting that Delta RBD might not be an ideal immunogen to induce cross-reactive neutralizing activities.
We also investigated the potential relationship of the neutralization potency of RmAbs and their corresponding binding affinity, mutation frequency, and CDR3 length, which further enhanced our understanding of affinity maturation and convergent evolution of RmAbs. The IC50 value of the neutralization potency of RmAbs was positively associated with their binding affinity, thus suggesting that a high binding affinity could confer robust neutralization activity. However, the high mutation frequency of the germline gene or a longer CDR3 does not necessarily confer potent neutralizing activities.
Our present study also has several limitations. First, the number of animals per group was limited, and we only compared the immunogenicity of monovalent Delta, monovalent Omicron BA.1, and bivalent Delta and Omicron BA.1. Second, only the immunogenicity of Omicron BA.1 was tested in this study, but we did not further investigate the immunogenicity of other Omicron sublineages. Finally, the protective efficacy of RmAbs was not tested in the animal challenge study.
In conclusion, our study illustrates that the bivalent vaccine might not be the superior immunogens to induce potent protective immune responses, not only from polyclonal level, but also from monoclonal level. We identified several potent neutralizing antibodies induced by the monovalent Omicron vaccine could neutralize all the tested Omicron sublineages. Our findings provide further insights for the rational design of next-generation COVID-19 vaccines and offer a potential therapeutic strategy against emerging SARS-CoV-2 sublineages.
Ethics approval statement
The research protocol was approved by the Ethics Committee of the Nanjing Drum Tower Hospital on Animal Use (Protocol No. 2020AE01120).
Supplemental Table 3
Download MS Excel (36.5 KB)Supplemental Table 2
Download MS Excel (66.5 KB)Supplemental Table 1
Download MS Excel (63 KB)Supplemental Figure 5
Download TIFF Image (49.9 MB)Supplemental Figure 4
Download TIFF Image (37.5 MB)Supplemental Figure 3
Download TIFF Image (43.9 MB)Supplemental Figure 2
Download TIFF Image (46.8 MB)Supplemental Figure 1
Download TIFF Image (34.2 MB)Disclosure statement
No potential conflict of interest was reported by the author(s).
Supplementary material
Supplemental data for this article can be accessed on the publisher’s website at https://doi.org/10.1080/21645515.2023.2264589.
Additional information
Funding
References
- Mlcochova P, Kemp SA, Dhar MS, Papa G, Meng B, Ferreira IATM, Datir R, Collier DA, Albecka A, Singh S, et al. SARS-CoV-2 B.1.617.2 Delta variant replication and immune evasion. Nature. 2021 Nov;599(7883):114–14. doi:10.1038/s41586-021-03944-y. PubMed PMID: 34488225; PubMed Central PMCID: PMCPMC8566220.
- Planas D, Veyer D, Baidaliuk A, Staropoli I, Guivel-Benhassine F, Rajah MM, Planchais C, Porrot F, Robillard N, Puech J, et al. Reduced sensitivity of SARS-CoV-2 variant Delta to antibody neutralization. Nature. 2021 Aug;596(7871):276–80. doi:10.1038/s41586-021-03777-9. PubMed PMID: 34237773.
- Suryawanshi R, Ott M. SARS-CoV-2 hybrid immunity: silver bullet or silver lining? Nat Rev Immunol. 2022 Oct;22(10):591–2. doi:10.1038/s41577-022-00771-8. PubMed PMID: 35945353; PubMed Central PMCID: PMCPMC9362961.
- Bowen JE, Addetia A, Dang HV, Stewart C, Brown JT, Sharkey WK, Sprouse KR, Walls AC, Mazzitelli IG, Logue JK, et al. Omicron spike function and neutralizing activity elicited by a comprehensive panel of vaccines. Science. 2022 Aug 19;377(6608):890–4. doi:10.1126/science.abq0203. PubMed PMID: 35857529; PubMed Central PMCID: PMCPMC9348749.
- Cao Y, Yisimayi A, Jian F, Song W, Xiao T, Wang L, Du S, Wang J, Li Q, Chen X, et al. BA.2.12.1, BA.4 and BA.5 escape antibodies elicited by Omicron infection. Nature. 2022 Aug;608(7923):593–602. doi:10.1038/s41586-022-04980-y. PubMed PMID: 35714668; PubMed Central PMCID: PMCPMC9385493 series antibodies, which includes BD30-604 (DXP-604), BD55-5840 (SA58) and BD55-5514 (SA55). X.S.X. and Y. Cao are founders of Singlomics Biopharmaceuticals. The other authors declare no competing interests.
- Viana R, Moyo S, Amoako DG, Tegally H, Scheepers C, Althaus CL, Anyaneji UJ, Bester PA, Boni MF, Chand M, et al. Rapid epidemic expansion of the SARS-CoV-2 Omicron variant in southern Africa. Nature. 2022 Mar;603(7902):679–86. doi:10.1038/s41586-022-04411-y. PubMed PMID: 35042229; PubMed Central PMCID: PMCPMC8942855.
- Wang Q, Guo Y, Iketani S, Nair MS, Li Z, Mohri H, Wang M, Yu J, Bowen AD, Chang JY, et al. Antibody evasion by SARS-CoV-2 Omicron subvariants BA.2.12.1, BA.4 and BA.5. Nature. 2022 Aug;608(7923):603–8. doi:10.1038/s41586-022-05053-w. PubMed PMID: 35790190; PubMed Central PMCID: PMCPMC9385487 (WO2021236998) or provisional patent applications (63/271,627) filed by Columbia University for several SARS-CoV-2 neutralizing antibodies described in this paper. Both sets of applications are under review. D.D.H. is a cofounder of TaiMed Biologics and RenBio, consultant to WuXi Biologics and Brii Biosciences and board director for Vicarious Surgical.
- Cao Y, Song W, Wang L, Liu P, Yue C, Jian F, Yu Y, Yisimayi A, Wang P, Wang Y, et al. Characterization of the enhanced infectivity and antibody evasion of Omicron BA.2.75. Cell Host Microbe. 2022 Nov 9;30(11):1527–39 e5. doi:10.1016/j.chom.2022.09.018. PubMed PMID: 36270286; PubMed Central PMCID: PMCPMC9531665.
- Chi X, Yan R, Zhang J, Zhang G, Zhang Y, Hao M, Zhang Z, Fan P, Dong Y, Yang Y, et al. A neutralizing human antibody binds to the N-terminal domain of the spike protein of SARS-CoV-2. Science. 2020 Aug 7;369(6504):650–5. doi:10.1126/science.abc6952.
- Jian F, Yu Y, Song W, Yisimayi A, Yu L, Gao Y, Zhang N, Wang Y, Shao F, Hao X, et al. Further humoral immunity evasion of emerging SARS-CoV-2 BA.4 and BA.5 subvariants. Lancet Infect Dis. 2022 Nov 1;22(11):1535–7. doi:https://doi.org/10.1016/S1473-3099(22)00642-9.
- Kimura I, Yamasoba D, Tamura T, Nao N, Suzuki T, Oda Y, Mitoma S, Ito J, Nasser H, Zahradnik J, et al. Virological characteristics of the SARS-CoV-2 Omicron BA.2 subvariants, including BA.4 and BA.5. Cell. 2022 Oct 13;185(21):3992–4007.e16. doi:https://doi.org/10.1016/j.cell.2022.09.018.
- Qu P, Evans JP, Zheng Y-M, Carlin C, Saif LJ, Oltz EM, Xu K, Gumina RJ, Liu S-L. Evasion of neutralizing antibody responses by the SARS-CoV-2 BA.2.75 variant. Cell Host Microbe. 2022 Nov 9;30(11):1518–26.e4. doi:https://doi.org/10.1016/j.chom.2022.09.015.
- Sheward DJ, Kim C, Fischbach J, Muschiol S, Ehling RA, Björkström NK, Hedestam GBK, Reddy ST, Albert J, Peacock TP, et al. Evasion of neutralising antibodies by omicron sublineage BA.2.75. Lancet Infect Dis. 2022 Oct;22(10):1421–2. doi:10.1016/S1473-3099(22)00524-2. PubMed PMID: 36058228; PubMed Central PMCID: PMCPMC9436366 acquired by Alloy Therapeutics. DJS, GBKH, and BM have intellectual property rights associated with antibodies that neutralise omicron variants. All other authors declare no competing interests.
- Planas D, Saunders N, Maes P, Guivel-Benhassine F, Planchais C, Buchrieser J, Bolland W-H, Porrot F, Staropoli I, Lemoine F, et al. Considerable escape of SARS-CoV-2 Omicron to antibody neutralization. Nature. 2022 Feb 1;602(7898):671–5. doi:10.1038/s41586-021-04389-z.
- Panke Q, John PE, Julia F, Zheng YM, Carlin C, Anghelina M, Stevens P, Fernandez S, Jones D, Lozanski G, et al. Distinct neutralizing antibody escape of SARS-CoV-2 Omicron subvariants BQ.1, BQ.1.1, BA.4.6, BF.7 and BA.2.75.2. bioRxiv. 2022 Oct 19:512891. doi:10.1101/2022.10.19.512891.
- Offit PA. Bivalent covid-19 vaccines — a cautionary tale. N Engl J Med. 2023 Feb 9;388(6):481–3. doi:10.1056/NEJMp2215780.
- Administration, FaD. Moderna COVID-19 vaccine, bivalent now authorized for all doses. https://www.fda.gov/vaccines-blood-biologics/coronavirus-covid-19-cber-regulated-biologics/moderna-covid-19-vaccines.
- Wang Q, Bowen A, Valdez R, Gherasim C, Gordon A, Liu L, Ho DD. Antibody response to Omicron BA.4–BA.5 bivalent booster. N Engl J Med. 2023 Feb 9;388(6):567–9. doi:10.1056/NEJMc2213907.
- Collier A-R, Miller J, Hachmann NP, McMahan K, Liu J, Bondzie EA, Gallup L, Rowe M, Schonberg E, Thai S, et al. Immunogenicity of BA.5 bivalent mRNA vaccine boosters. N Engl J Med. 2023 Feb 9;388(6):565–7. doi:10.1056/NEJMc2213948.
- He Q, Liu S, Liang Z, Lu S, Cun W, Mao Q. Mouse study of combined DNA/protein COVID-19 vaccine to boost high levels of antibody and cell mediated immune responses. Emerging Microbes Infect. 2023 Dec 31;12(1):2152388. doi:10.1080/22221751.2022.2152388.
- Lu S. Timely development of vaccines against SARS-CoV-2. Emerging Microbes Infect. 2020 Jan 1;9(1):542–4. doi:10.1080/22221751.2020.1737580.
- Arvanitis P, Lerner AH, Vieira K, Almaghlouth N, Farmakiotis D. Outpatient anti-spike monoclonal antibody administration is associated with decreased morbidity and mortality among patients with cancer and COVID-19. Clin Exp Med. 2023 Feb 13. doi: 10.1007/s10238-023-01019-y.
- Morgan MS, Yan K, Le TT, Johnston RA, Amarilla AA, Muller DA, McMillan CLD, Modhiran N, Watterson D, Potter JR, et al. Monoclonal antibodies specific for SARS-CoV-2 spike protein suitable for multiple applications for Current variants of concern. Viruses. 2023;15(1):139. doi:10.3390/v15010139.
- Thümmler L, Lindemann M, Horn PA, Lenz V, Konik M, Gäckler A, Boss K, Theodoropoulos F, Besa V, Taube C, et al. Early treatment with monoclonal antibodies or convalescent plasma reduces mortality in non-vaccinated COVID-19 high-risk patients. Viruses. 2023;15(1):119. doi:10.3390/v15010119.
- Wang W, Fan B, Zhang X, Guo R, Zhao Y, Zhou J, Zhou J, Peng Q, Zhu M, Li J, et al. Development of a colloidal gold immunochromatographic assay strip using monoclonal antibody for rapid detection of porcine deltacoronavirus [Original Research]. Front Microbiol. 2023 Jan 5;13. doi:10.3389/fmicb.2022.1074513. English.
- Chu X, Ding X, Yang Y, Lu Y, Li T, Gao Y, Zheng L, Xiao H, Yang T, Cheng H, et al. Mechanism of an RBM-targeted rabbit monoclonal antibody 9H1 neutralizing SARS-CoV-2. Biochem Bioph Res Co. 2023 Jun 11;660:43–9. doi:10.1016/j.bbrc.2023.04.002.
- Guo H, Yang Y, Zhao T, Lu Y, Gao Y, Li T, Xiao H, Chu X, Zheng L, Li W, et al. Mechanism of a rabbit monoclonal antibody broadly neutralizing SARS-CoV-2 variants. Commun Biol. 2023Apr 3;6(1):364. doi:10.1038/s42003-023-04759-5. PubMed PMID: 37012333; PubMed Central PMCID: PMCPMC10069731. eng.
- Chen Y, Zhu L, Huang W, Tong X, Wu H, Tao Y, Tong B, Huang H, Chen J, Zhao X, et al. Potent RBD-specific neutralizing rabbit monoclonal antibodies recognize emerging SARS-CoV-2 variants elicited by DNA prime-protein boost vaccination. Emerg Microbes Infect. 2021 Dec;10(1):1390–403. doi:10.1080/22221751.2021.1942227. PubMed PMID: 34120577; PubMed Central PMCID: PMCPMC8274519. eng.
- Chen Y, Vaine M, Wallace A, Han D, Wan S, Seaman MS, Montefiori D, Wang S, Lu S. A novel rabbit monoclonal antibody platform to dissect the diverse repertoire of antibody epitopes for HIV-1 env immunogen design. J Virol. 2013 Sep 15;87(18):10232–43. doi:10.1128/JVI.00837-13.
- Nie J, Li Q, Wu J, Zhao C, Hao H, Liu H, Zhang L, Nie L, Qin H, Wang M, et al. Establishment and validation of a pseudovirus neutralization assay for SARS-CoV-2. Emerg Microbes Infect. 2020 Dec;9(1):680–6. doi:10.1080/22221751.2020.1743767. PubMed PMID: 32207377; PubMed Central PMCID: PMCPMC7144318. eng.
- Li H, Wang S, Hu G, Zhang L, Liu S, Lu S. DNA priming immunization is more effective than recombinant protein vaccine in eliciting antigen-specific B cell responses. Emerging Microbes Infect. 2021 Jan 1;10(1):833–41. doi:10.1080/22221751.2021.1918026.
- Mikocziova I, Greiff V, Sollid LM. Immunoglobulin germline gene variation and its impact on human disease. Genes Immun. 2021 Aug 1;22(4):205–17. doi:10.1038/s41435-021-00145-5.
- Yan Q, Hou R, Huang X, Zhang Y, He P, Zhang Y, Liu B, Wang Q, Rao H, Chen X, et al. Shared IGHV1-69-encoded neutralizing antibodies contribute to the emergence of L452R substitution in SARS-CoV-2 variants. Emerging Microbes Infect. 2022 Dec 31;11(1):2749–61. doi:10.1080/22221751.2022.2140611.
- Kuhlmann C, Mayer CK, Claassen M, Maponga T, Burgers WA, Keeton R, Riou C, Sutherland AD, Suliman T, Shaw ML, et al. Breakthrough infections with SARS-CoV-2 omicron despite mRNA vaccine booster dose. Lancet. 2022 Feb 12;399(10325):625–6. doi:10.1016/S0140-6736(22)00090-3.
- Huang C, Huang L, Wang Y, Li X, Ren L, Gu X, Kang L, Guo L, Liu M, Zhou X, et al. RETRACTED: 6-month consequences of COVID-19 in patients discharged from hospital: a cohort study. Lancet. 2021 Jan 16;397(10270):220–32. doi:10.1016/S0140-6736(20)32656-8.
- Weber J, Peng H, Rader C. From rabbit antibody repertoires to rabbit monoclonal antibodies. Experimental & Molecular Medicine. 2017 Mar 1;49(3):e305–e305. doi:10.1038/emm.2017.23.
- Esteves PJ, Abrantes J, Baldauf H-M, BenMohamed L, Chen Y, Christensen N, González-Gallego J, Giacani L, Hu J, Kaplan G, et al. The wide utility of rabbits as models of human diseases. Experimental & Molecular Medicine. 2018 May 1;50(5):1–0. doi:10.1038/s12276-018-0094-1.
- Soares J, Pinheiro A, Esteves PJ. 2022. The rabbit as an animal model to study innate immunity genes: is it better than mice? Front Immunol. 2022 Sept 9;13. doi:10.3389/fimmu.2022.981815. English.
- Hoffmann M, Krüger N, Schulz S, Cossmann A, Rocha C, Kempf A, Nehlmeier I, Graichen L, Moldenhauer A-S, Winkler MS, et al. The Omicron variant is highly resistant against antibody-mediated neutralization: implications for control of the COVID-19 pandemic. Cell. 2022 Feb 3;185(3):447–56.e11. doi:10.1016/j.cell.2021.12.032.
- Willett BJ, Grove J, MacLean OA, Wilkie C, De Lorenzo G, Furnon W, Cantoni D, Scott S, Logan N, Ashraf S, et al. SARS-CoV-2 Omicron is an immune escape variant with an altered cell entry pathway. Nature Microbiol. 2022 Aug 1;7(8):1161–79. doi:10.1038/s41564-022-01143-7.
- Peng L, Fang Z, Renauer PA, McNamara A, Park JJ, Lin Q, Zhou X, Dong MB, Zhu B, Zhao H, et al. Multiplexed LNP-mRNA vaccination against pathogenic coronavirus species. Cell Rep. 2022 Aug 2;40(5):111160. doi:10.1016/j.celrep.2022.111160.
- Payne RP, Longet S, Austin JA, Skelly DT, Dejnirattisai W, Adele S, Meardon N, Faustini S, Al-Taei S, Moore SC, et al. Immunogenicity of standard and extended dosing intervals of BNT162b2 mRNA vaccine. Cell. 2021 Nov 11;184(23):5699–714.e11. doi:10.1016/j.cell.2021.10.011.
- Dyer O. Covid-19: infections climb globally as EG.5 variant gains ground. BMJ. 2023;382:1900. doi:10.1136/bmj.p1900.
- Link-Gelles R, Ciesla AA, Roper LE, Scobie HM, Ali AR, Miller JD, Wiegand RE, Accorsi EK, Verani JR, Shang N, et al. Early estimates of bivalent mRNA booster dose vaccine effectiveness in preventing symptomatic SARS-CoV-2 infection attributable to Omicron BA.5- and XBB/XBB.1.5-related sublineages among immunocompetent adults - increasing community access to testing program, United States, December 2022-January 2023. Morb Mortal Wkly Rep. 2023Feb 3;72(5):119–24. doi:10.15585/mmwr.mm7205e1. PubMed PMID: 36730051; PubMed Central PMCID: PMCPMC9927070 Journal Editors form for disclosure of potential conflicts of interest. No potential conflicts of interest were disclosed. eng.