ABSTRACT
During the SARS-CoV-2 pandemic, the lack of standardized measurements of the immune response after vaccination or recovery from COVID-19 resulted in incomparable results and hindered correlation establishment. Prioritizing reliable and standardized methods to monitor pathogen-specific immunity is crucial, not only during the COVID-19 pandemic but also for future outbreaks. During our study of the humoral immune response, we used a SARS-CoV-2 wild-type neutralization assay, ensuring the measurement of the immune response directed to all SARS-CoV-2 antigens in their proper conformation. A head-to-head comparison of the neutralizing antibody (NAb) responses elicited by four vaccines used in Europe during 2021 (BNT162b2, mRNA-1273, ChAdOx nCoV-19, and Ad26.COV2.S) and their comparison to NAb responses in convalescents showed that while the amount was comparable, NAbs induced by natural infection were of higher quality. Namely, NAbs produced by disease were better activators of the complement system than NAbs induced by vaccination. Furthermore, the contribution of spike protein-specific IgGs to the SARS-CoV-2 neutralization was lower in convalescents compared to vaccinees, indicating that those who recovered from COVID-19 were armed with antibodies of additional specificities and/or classes that contributed to virus neutralization. These findings suggest that a higher stringency of public policy measures targeting individuals who have recovered from COVID-19, in comparison to those who have been vaccinated, may not have been fully justified.
Introduction
A rapid deciphering of the SARS-CoV-2 genome in January 2020Citation1 spurred the development of effective vaccines at an impressive pace, resulting in the approval of the first vaccine against COVID-19 just 11 months later.Citation2 COVID-19 vaccines have been so far approved for use in humans on the basis of the clinical studies comparing their efficacy to placebo. Head-to-head comparisons of the several approved vaccines are still rare, as well as comparisons of immune responses generated by vaccination to the ones created by the disease. Due to the lack of appropriate standardizationCitation3,Citation4 of assays that evaluate immune response, it is challenging to compare results from different studies. Two recent studies tried to overcome this problem by using data on antibody levels from different assays but normalized or calibrated to those published alongside for human convalescent sera.Citation5,Citation6 Such head-to-head analyses are inevitable for correct assessment of public policy measures during the second year of COVID-19 pandemic, which were applied differently to COVID-19 vaccinees and convalescents.
During 2021, for emergency use in the European Union, the EMA approved two adenovirus vector-based vaccines developed by Oxford/AstraZeneca (ChAdOx nCoV-19/Vaxzevria) and J&J/Janssen (Ad26.COV2.S) and two mRNA vaccines developed by Pfizer-BioNTech (BNT162b2/Comirnaty) and Moderna (mRNA-1273/Spikevax). All four deliver genetic information for the full-length spike (S) protein into the host cells that produce the functional protein, express it on cell surfaces, and induce both humoral and cellular branches of SARS-CoV-2-specific immune response. Thus, neutralizing antibody (NAb) response could serve as an indicator of the overall immune response quality induced by these similarly acting vaccines.
Goldblatt et al.Citation7 performed simultaneous analysis of S protein-binding and pseudovirus-neutralizing antibodies generated by these first four COVID-19 vaccines approved and used in Great Britain and the EU. They showed the importance of such studies for estimation of protective antibody threshold for COVID-19. These four vaccines were also compared by van Gils et al.Citation8 with the aim to understand differences in capability to neutralize SARS-CoV-2 variants.
In our study, we provide for the first time a head-to head comparison of wild-type SARS-CoV-2 NAb responses induced by four vaccines used during 2021 in Europe, as well as a comparison of NAb responses in COVID-19 vaccinees and convalescents. The standardized wild-type neutralization assay (using B.1.1.1. virus variant as a challenge virus)Citation9 calibrated to WHO standardCitation3,Citation10 enabled a reliable comparison of NAb responses induced by vaccines to those induced in convalescents during 2020, when circulating variant of SARS-CoV-2 was the same as the one used to design the vaccines. The usage of wild-type SARS-CoV-2 for neutralization provided not only data on similarities and differences in antibody quantity but also an insight into antibody quality, particularly the ability of virus-specific antibodies to trigger complement activation, as well as a better understanding of the contribution of S protein-specific antibodies in the neutralization of the wild-type virus.
To the best of our knowledge, this study represents the most comprehensive approach so far aimed to understand not only quantitative but also qualitative differences in the humoral immune response generated by both primary and booster vaccinations, as well as natural infection and additional vaccination of convalescents. Overall, the obtained data indicate that recovery from the disease induced quantitatively comparable but qualitatively superior neutralizing antibodies to the ones induced by vaccination at the population level. Such findings point to the need to reexamine the correctness of higher epidemiological measures’ stringency applied to people who have recovered from the disease during the COVID-19 pandemic. The data also demonstrate the importance of a standardized approach in assessing humoral immunity, by the usage of the most comprehensive assays as early as possible in the case of a new epidemic.
Materials and methods
Study cohorts
This study was conducted using several groups of volunteers who underwent frequent phlebotomies with the aim to determine seroconversion against SARS-CoV-2 induced by vaccines or natural infection.
A cohort of naïve vaccinees (cohort V) received the vaccine as a part of national vaccine rollout campaign. Cohort V consisted of 114 (35 M; 79 F; mean age 44.4 ± 2.2 y) naïve vaccinees, followed from March 2021 till March 2022, who received either BNT162b2 (n = 55 (9 M, 46 F); age 44.7 ± 3.1 y), mRNA-1273 (n = 19 (9 M, 10 F); age 47.4 ± 4.1 y), ChAdOx nCoV-19 (n = 19 (11 M, 8 F); age 50.8 ± 5.9 y), or Ad26.COV2.S (n = 21 (6 M, 15 F); 34.9 ± 3.0 y). Regarding the humoral response after booster vaccination with BNT162b2 (cohort VB; n = 52 (13 M, 39 F); age 49.8 ± 3.1), participants were followed from December 2021 till May 2022. All of them received booster dose of BNT162b2 vaccine while primary vaccination was conducted as follows: 40 (8 M, 32 F) received BNT162b2; 2 (1 M, 1 F) received mRNA-1273; and 4 (2 M, 2 F) received ChAdOx nCoV-19, while four volunteers (0 M, 4 F) completed Ad26.COV2.S primary vaccination. All individuals included in cohorts V and VB did not have a known history of SARS-CoV-2 infection or breakthrough infection. A number of samples were also tested before the first vaccine dose administration, and all were proved negative for virus-neutralizing activity. All cohort V participants were analyzed after receiving a single shot of any vaccine (2 and 4 weeks later; data not shown). The response after a single shot in naïve subject was significantly lower than the response in those previously sensitized (cca 2 logarithms lower). Some respondents, who would have developed a high level of NAbs after just one dose, in a follow-up interview pointed out the severe infection of unknown origin they overcame in the 2019/2020 season, before the established testing for COVID-19. Such volunteers were excluded from the study.
To enable comparison between post-vaccination and post-infection NAb responses, SARS-CoV-2 neutralization potencies in sera of COVID-19 convalescents, taken 1–3 months after infection (described in the study by Ravlić et al.Citation9) were included in the analysis. The cohort of COVID-19 convalescents (cohort C; n = 76 (46 M, 30 F); age 35.8 ± 2.5 y) was recruited in the period from July 2020 till March 2021 and included donors who recovered from mild to severe illness (excluding those who required hospitalization). The majority of donors provided blood sample only once. Six of them who provided blood several times were included in longitudinal monitoring of NAb persistence after infection. Cohort C was thoroughly analyzed and described before.Citation9 In this work, a total of 87 samples from 76 donors, collected in the period of 1–3 months after infection, were analyzed.
A cohort of vaccinees sensitized to COVID-19 (cohort C+V; n = 26 (5 M, 21 F); age 41.8 ± 4.4 y) consisted of the volunteers with a positive history of COVID-19 preceding the vaccine uptake and was followed in the period from May 2021 to February 2022. Nine volunteers received Ad26.COV2.S vaccine, while the rest of them received either one or two shots of ChAdOx nCoV-19 (n = 2), mRNA-1273 (n = 4), BNT162b2 (n = 10), or ChAdOx nCoV-19/BNT162b2 combination (n = 1) vaccine. In total, 124 samples from these 26 volunteers in cohort C+V were analyzed.
Additional 197 serum samples of 112 naïve primary vaccinated individuals from separate cohort of University Hospital for Infectious Diseases employees, collected continuously since the beginning of 2021, were included only in the analysis of complement activation. Serum samples were analyzed in both native and in heat-inactivated form (56°C for 30 min).
In cohorts V, VB, and C+V serum samples were taken approximately 2 and 4 weeks after each shot and in several occasions upon completion of vaccination, in approximately 45-d intervals. Information about vaccination was obtained from participants at visits by self-report that included the type of vaccination, the number of doses received, and the dates of applications. None of the participants reported the use of medications for immunosuppression.
Raw experimental data for human samples analyzed in this work are provided in Supplementary material.
Blood sample processing and storage
Sera for antibody assays (neutralization and ELISA) were prepared from collected venous blood in serum collection tubes containing a cloth activator (Becton Dickinson, 368815). Clots were removed by centrifugation at 800 × g for 10 min at room temperature and sera were split into 1000 µL aliquots and stored at −20°C until further use.
Neutralization assay
Wild-type SARS-CoV-2 neutralizing antibodies were measured in the ED50 assay, in which a wild-type SARS-CoV-2 served as a challenge virus. The challenge virus was derived from the PCR-positive oro- and nasopharyngeal swab designated 297/20 Zagreb taken from a patient in Zagreb, Croatia, and used to produce the laboratory working bank of the SARS-CoV-2, which served as the challenge virus for the assay. The virus belongs to B.1.1.1. lineage as determined by deep sequencing.Citation9 The assay was performed in a certified Biosafety level 3 laboratory. Details on the assay’s development and standardization have been described previously.Citation9 Briefly, the assay was performed in 96-well tissue culture microplates (Sarsted, 83.3920). Octaplicates of twofold serial dilution of the donor’s serum were preincubated with approximately 20 CCID50/well SARS-CoV-2 suspension at 37°C and 5% CO2 for 90 min. After adding 30 000 Vero E6/well, the plates were incubated at 37°C and 5% CO2 for 4 d followed by inspection of the grown cell layers in all wells using an inverted optical microscope. Wells with detected cytopathic effect (CPE) were counted. The effective dose 50 (ED50), the amount of undiluted serum that inhibits CPE in 50% of infected wells, was calculated according to Spearman–Kärber method. Neutralization titer (NT) was expressed as the number of ED50 doses in 1 mL of serum. The development and usage of challenge virus SARS-CoV-2 working stock (B.1.1.1. virus variant, genome sequence deposited in GISAID database as EPI_ISL_3013041), as well as the anti-SARS-CoV-2 in-house standard, were thoroughly described in our previously recorded findings.Citation9 Anti-SARS-CoV-2 in-house standard was calibrated against the 1st WHO International Standard for anti-SARS-CoV-2 (NIBSC, 20/136). All neutralization potencies of analyzed sera were recalculated from the ED50 mL−1 to the units of WHO International standard and are expressed as IU mL−1.
Complement-activating properties of SARS-CoV-2 neutralizing antibodies
For the purpose of measuring of complement-activating properties of SARS-CoV-2-specific antibodies, two aliquots of each serum sample were analyzed simultaneously – one in a native form and another after complement inactivation by heating at 56°C for 30 min.Citation9,Citation11 The ratio between the two values served as the indicator of the contribution of complement activity to SARS-CoV-2 neutralization potency (complement activity index, CAI).
Spike protein-specific IgG quantification
The Euroimmun anti-SARS-CoV-2 ELISA assay (Euroimmun, EI 2606–9601 G) was used for the detection of IgG against the S1 domain of the SARS-CoV-2 S protein. Briefly, serum samples were diluted at 1:101 in sample buffer and pipetted into microplate wells that were precoated with recombinant SARS-CoV-2 S protein. Positive control, negative control, calibrator, and our own in-house standard were carried out in each plate. In-house standard of a known value was included into the assay to minimize batch-to-batch variabilities in the assay performance.
Study approval
The study was performed in compliance with all relevant ethical regulations. The analyzed samples were collected according to the approvals obtained from several institutions: Ethical Committee of Croatian Institute of Transfusion Medicine (003–06/20–04/02, No. 251-541-06/6-20-2; May 7, 2020), Ethical Committee of the University Hospital Centre Zagreb (8.1–21/259–4; No. 02/21 AG; November 29, 2021) and Ethical Committee of the University Hospital for Infectious Diseases “Dr. Fran Mihaljević” (No. 01-228-1-2021; February 5, 2021). Each participant provided written informed consent.
Data analysis
When the average value from the set of n data was calculated, 95% confidence interval was provided as an indicator of measurement uncertainty. NT values expressed in IU mL−1 were linearized by calculating logarithmic values and then used for statistical analysis. Differences between several cohorts lacking a normal distribution of data were assessed by Kruskal–Wallis test, with post-hoc comparison between individual groups using Conover. When data in cohorts met normal distribution, their differences were analyzed by ANOVA. Complement activity in convalescents’ and vaccinees’ sera were compared by Mann–Whitney U test. Details on analyses performed and the level of significance detected are provided alongside each set of results. MedCalc v20.011 was used for statistical analysis.
Results
Quantity of SARS-CoV-2 neutralizing antibody developed by disease or vaccination
Contribution of primary vaccination to SARS-CoV-2 - directed neutralization potency of sera in previously COVID-19 naïve vaccinees
NAb responses to wild-type SARS-CoV-2 were compared in humans after vaccination with each of the first four vaccines approved for emergency use by EMA. ChAdOx nCoV-19, BNT162b2, mRNA-1273, and Ad26.COV2.S were the only vaccines used in 2021 within Croatia, as well as in the rest of the European Union. By plotting the NAb titer (NT) in samples versus time elapsed since the primary vaccination (in days), we observed that the highest NTs were developed within the first month after vaccination completion (). The same was observed also after natural infection, in convalescents ().Citation9 For comparison of peak responses, statistical analysis of differences in NAb responses was performed using only samples collected within the first month after vaccination completion or recovery from illness (framed points in and provided in ). The NAb response was quite uniform among samples within each vaccine group, while it was more dispersed in convalescent group (). Both mRNA vaccines induced similar (3.05 ± 0.17 and 3.20 ± 0.21 log IU mL−1 for BNT162b2 (n = 27) and mRNA-1273 (n = 16), respectively) and significantly (p < .05) higher NAb responses in comparison to ChAdOx nCoV-19 (2.32 ± 0.24 log IU mL−1; n = 11), and particularly Ad26.COV2.S vaccine (1.31 ± 0.57 log IU mL−1; n = 21). NAb responses in both mRNA vaccine groups were also significantly (p < .05) higher compared to convalescents (2.02 ± 0.30 log IU mL−1; n = 18) recovered from mild-to-severe illness (excluding those that required hospitalization). Responses of ChAdOx nCoV-19 and Ad26.COV2.S recipients were significantly (p < .05) lower compared to both mRNA vaccine recipients (). Values of ChAdOx nCoV-19 sera NTs were in the same range, while the Ad26.COV2.S NTs were significantly lower (p < .05) compared to convalescents’ NTs ().
Figure 1. SARS-CoV-2 neutralizing antibody responses (IU mL−1) in vaccinees after primary vaccination completion and in COVID-19 convalescents that recovered from mild to severe (outpatient) disease. Data are presented in relation to the time (in days) that passed from the event (either vaccination or disease). Peak responses were developed within the first month (framed) and were compared between the groups. Kruskal–Wallis analysis proved the difference between groups with high level of significance (p < .000001). Individual groups were post-hoc analyzed using Conover, and significant differences (p < .05) are denoted by *. Minimal and maximal SARS-CoV-2 NAb titers determined in COVID-19 convalescents are denoted by dashed lines.
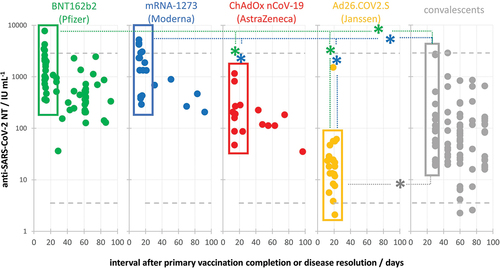
Table 1. Peak responses (within 12–30 d from the event) in all study cohorts with their demographic characteristics (size, age, abd sex distribution).
Contribution of booster vaccination to SARS-CoV-2 neutralization potency of sera in COVID-19 naïve vaccinees
The widely dispersed immune response at the population level after primary vaccination with four different vaccines (cohort V; samples collected within 1 month after vaccination completion, n = 75) became uniform and reached the plateau level in the entire population of vaccinees after booster vaccination with BNT162b2 (cohort VB; samples collected within 1 month after booster, n = 52), irrespective of the vaccine used for the primary vaccination (). The neutralization potencies of the entire population of vaccinees after booster vaccination (VB; 3.527 ± 0.083 log IU mL−1; n = 52) were significantly (p < .05) higher in comparison to the vaccinees’ population after primary vaccination (V; 2.488 ± 0.208 log IU mL−1; n = 75), and also higher compared to the population of COVID-19 convalescents (C; 2.017 ± 0.299 log IU mL−1; n = 18). The majority of volunteers in the VB cohort received BNT162b2 also in the primary vaccination (n = 40), so their post-booster vaccination NTs (3.487 ± 0.126 log IU mL−1; n = 40) were compared to the NTs in sera of cohort V BNT162b2 receivers after primary vaccination (3.050 ± 0.167 log IU mL−1; n = 27). BNT162b2 booster vaccination induced statistically significant (p < .0001) and on average 2.7 times higher NAb response compared to NT developed after primary vaccination with the same vaccine. Among the remaining BNT162b2 booster receivers, two were primary vaccinated with mRNA-1273, four with ChAdOx nCoV-19, and four with Ad26.COV2.S. Their titers were on average 3.0, 15.5, and 88.5 times higher than in cohort V mRNA-1273 (n = 16), ChAdOx nCoV-19 (n = 11), and Ad26.COV2.S (n = 21) primary vaccination receivers, respectively.
Figure 2. Comparison of SARS-CoV-2 NAb titers in vaccinees after the booster dose (VB) and primary vaccination (V), as well as in COVID-19 convalescents with (C+V) and without (C) additional vaccination. The C+V cohort is shown as two data sets corresponding to one- and two-dose vaccine recipients. Kruskal–Wallis analysis proved the difference between the groups with high level of significance (p < .000001). Individual groups were post-hoc analyzed using Conover, and significant differences (p < .05) are denoted by *. Minimal and maximal SARS-CoV-2 NAb titers determined in COVID-19 convalescents are denoted by dashed lines.
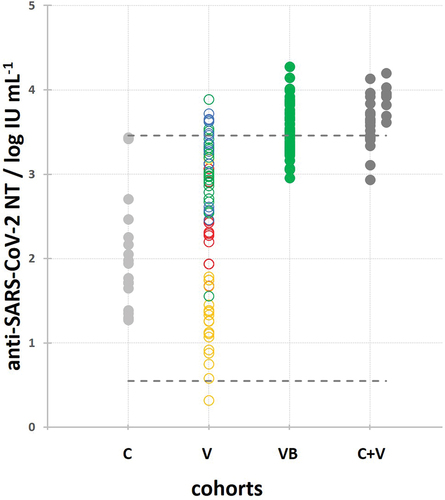
Overall, booster vaccination served as a kind of catch-up campaign to those who did not respond sufficiently to the primary vaccination, or to those who received the less effective vaccines in the primary vaccination. At the population level, booster vaccination induced significantly higher (p < .05) level of NAb response in comparison to the primary vaccination cycle completion ().
Contribution of vaccination to SARS-CoV-2 neutralization titer of sera in COVID-19 convalescents
Only a single shot of any vaccine in a previously COVID-19-sensitized person (C+V) induced a uniform and maximal NAb response (3.580 ± 0.167 log IU mL−1; n = 18), which was as high as the response achieved in BNT162b2 booster receivers (3.527 ± 0.083 log IU mL−1; n = 52) (). Equally as a booster in vaccinees, a single vaccine shot in COVID-19 convalescents induced the NT response significantly higher (p < .05) to the one induced either by primary vaccination or developed in COVID-19 convalescents (2.017 ± 0.399 log IU mL−1; n = 18).
High level of SARS-CoV-2 NT (around 3.5 log IU mL−1) was measured in all COVID-19-sensitized vaccinees after only a single vaccine dose, irrespective of whether they received it at two or up to 14 months after the infection and recovery ().
Figure 3. SARS-CoV-2 NAb titers in convalescents additionally vaccinated by a single dose of any vaccine in relation to the interval from recovery to vaccination. Minimal and maximal SARS-CoV-2 NAb titers determined in COVID-19 convalescents are denoted by dashed lines.
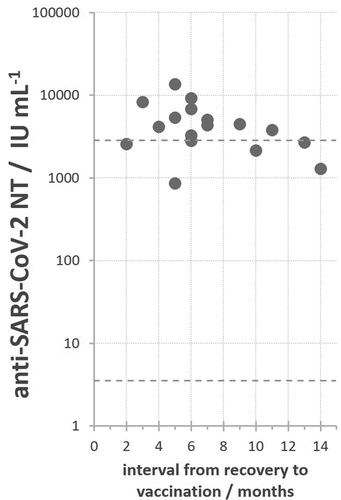
Two vaccine doses induced on average 2.1 times higher NT in COVID-19-sensitized people (3.903 ± 0.136 log IU mL−1; n = 9) in comparison to those who received one dose after recovery from COVID-19 (3.580 ± 0.114 log IU mL−1; n = 18) (). The small rise in the NT level, although statistically significant (p < .006), indicates that NT values in the range of 3.5 to 4.0 log IU mL−1 might have reached plateau, the highest achievable level of SARS-CoV-2 NAb levels in human population.
Quality of SARS-CoV-2 neutralizing antibodies developed by disease or vaccination
Contribution of complement to the neutralization of SARS-CoV-2 in COVID-19 vaccinees and convalescents
Complement activity was measured by simultaneous determination of SARS-CoV-2 NT of each serum sample in its native and heat-inactivated (HI) form. The ratio between the two values served as the indicator of the contribution of complement activity to SARS-CoV-2 neutralization potency (complement activity index, CAI). In total, 304 COVID-19 naïve vaccinees’ sera, collected after either single or both doses in primary vaccination, were analyzed. Heat inactivation in vaccinees induced on average 1.76 ± 0.30 (n = 304) in contrast to 2.37 ± 1.26 decrease (n = 69)Citation9 of NT in convalescents. The plot demonstrating the relationship between complement activity index (the ratio native NT:HI NT) for each serum and its NT in HI form (neutralization due to antibody activity only) showed that complement activity was not measurable in high-titer sera (CAI around 1) in both vaccinees and convalescents (). This phenomenon is due to the intrinsic properties of the SARS-CoV-2 neutralization assay itself.Citation9,Citation11 Namely, sera with high concentration of neutralizing antibodies have to be analyzed in neutralization assay in high dilutions. In such dilutions, complement components are also highly diluted, so their activation is not measurable anymore. For that reason, statistical analysis of differences in complement activity index between the two groups (COVID-19 convalescents and vaccinees) was performed using only sera with NT below 100 IU mL−1 in both groups. The analysis proved that SARS-CoV-2-specific immunoglobulins in convalescents (CAI 2.79 ± 0.39; n = 45) were significantly (p < .01) stronger activators of complement than those present in the serum of vaccinees (CAI 2.28 ± 0.24; n = 143) ().
Figure 4. Differences in complement-activating properties of SARS-CoV-2-specific antibodies developed after vaccination or in infection. (a) Complement activity index versus NAb titer plots in sera from vaccinees and convalescents, with NAb titers at which complement activity index was approaching to 1, is indicated by arrows. (b) Complement activity index in convalescents’ sera (n = 45) was significantly (*p < .01) stronger than in vaccinees’ sera (n = 143), analyzed by Mann–Whitney U test.
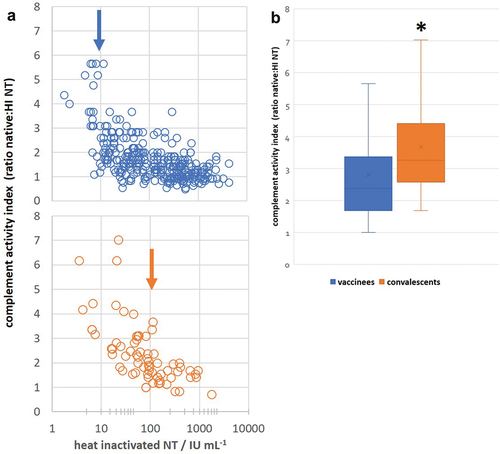
In addition, complement activity index in convalescents was approaching 1 in HI sera with NT of 100 IU mL−1, while in vaccinees already in HI sera with NT of 10 IU mL−1 (, indicated by arrows). Such results also supported the finding that SARS-CoV-2-specific immunoglobulins in COVID-19 convalescents were better activators of complement than S protein-specific immunoglobulins induced by vaccination, since complement activity was not measurable anymore in lower dilutions of vaccinees’ compared to convalescents’ sera.
Contribution of anti-spike protein IgGs to SARS-CoV-2 neutralization in COVID-19 vaccinees and convalescents
Commercial Euroimmun anti-SARS-CoV-2 ELISA was used for quantification of S protein-specific IgG quantity in the majority of collected sera. When plotting SARS-CoV-2 neutralization titer (in log IU mL−1) versus S protein-specific IgG quantity (in Euroimmun’s index) for each serum, two different and clearly separated populations were obtained – convalescent (gray) and vaccinees (blue) (). The relationship between these two values in convalescent sera was best described by logarithmic function (n = 23), while in vaccinees’ sera by polynomial function (n = 139) (). Such finding implied that the sera of convalescents having equal neutralization potency as vaccinees sera on average contains a lower amount of S protein-specific IgG.
Durability of SARS-CoV-2 neutralizing antibodies
Longitudinal monitoring of the quantity of antibodies in the serum of each volunteer made it possible to assess the durability of antibodies. Sera were collected regularly 2 and 4 weeks after vaccination completion, and then in regular intervals (every 45–60 d) for at least 4 months. NAb quantities for each serum (in log IU mL−1) were plotted against time after vaccination (in days), and the slope of the best fitting line through the spots served as the antibody titer decrease rate per day (in log IU mL−1/day). Due to low throughput and demandingness of the neutralization assay, longitudinal serum samples of previously COVID-19 naïve vaccinees (cohorts V and VB) were analyzed only by the commercial ELISA determining anti-S protein IgG quantity and then were back-calculated into neutralization titers using parameters of the polynomial curve describing relationship between NT (in log IU mL−1) and S protein-specific IgG (in Euroimmuns’s index) in vaccinees (). We were aware that the relationship between anti-S protein IgG quantity and neutralization titer does not follow the same curve in vaccinees and convalescents (), indicating the significant difference in neutralizing antibody specificity. For that reason, back-calculation of anti-S protein IgG quantity into NT would not be reliable in convalescents who were additionally vaccinated. So, the longitudinal monitoring of antibody duration in cohorts C and C+V was performed completely by the neutralization assay. In all groups, only the data from individuals that donated blood at least three times in the row were included in the analysis.
COVID-19 naïve volunteers who received Ad26.COV2.S (n = 20) developed low levels of NAbs, which remained detectable in the same quantity up to 4 months after vaccination (). The same picture was observed in ChAdOx nCoV-19 receivers after a single dose (data not shown). After completion of primary vaccination, NAb quantity was significantly higher in recipients of all three two-dose-scheduled vaccines than in Ad26.COV2.S receivers, and a decrease in their NAbs over time was observed (). Antibody decrease rates (in log IU mL−1/day) were −0.0087 ± 0.0011, −0.0063 ± 0.0015, and −0.0041 ± 0.0012 in BNT162b2 (n = 18), mRNA-1273 (n = 16), and ChAdOx nCoV-19 (n = 11) receivers, respectively. NAb decrease rates in two-dose vaccine recipients were used for further comparison to NAb decrease rates in booster receivers (cohort VB), in convalescents (cohort C), and in convalescents who were additionally vaccinated (cohort C+V).
Figure 6. Longitudinal monitoring of NAb titers in the sera of volunteers after completion of primary vaccination using each of the four investigated vaccines. Slopes of the lines obtained for samples of each volunteer in BNT162b2, mRNA-1273, and ChAdOx nCoV-19 group served as the NAb decrease rates (in log IU mL−1/day).
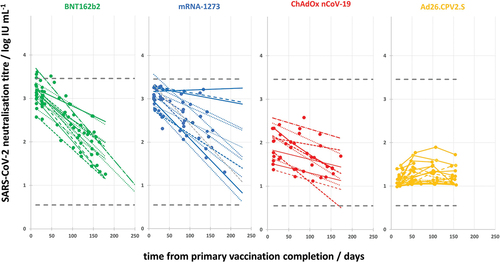
Analysis revealed that NTs in additionally vaccinated convalescents (cohort C+V) and in boosted vaccinees (cohort VB) had comparable decrease rates (in log IU mL−1/day): −0.0039 ± 0.0010 (n = 20) and −0.0035 ± 0.0013 (n = 26), respectively (). The equal decrease rate (−0.0039 ± 0.0037; n = 6) was previously measured also in convalescentsCitation9 (updated in ). NAbs developed in primary vaccination demonstrated significantly faster waning, with NAb titer decrease rate −0.0067 ± 0.0009 log IU mL−1/day being significantly higher (p < .05) in comparison to cohorts C+V and CB.
Figure 7. Decrease rate of anti-SARS-CoV-2 neutralizing antibody titers per day (log IU mL−1/day) in cohorts of convalescent with (C+V, n = 20) or without (C, n = 6) data fromCitation9 additional vaccination, as well as vaccinees after primary vaccination completion (V, n = 45) and additional booster vaccine dose (VB, n = 26). NAb titers measured in longitudinal monitoring for each individual during at least 4 months were plotted against the time after the vaccination, and the slope of the line served as the individual NAb titer decrease rate; * p < .05 in comparison to cohort V, according to pairwise comparison in ANOVA analysis.
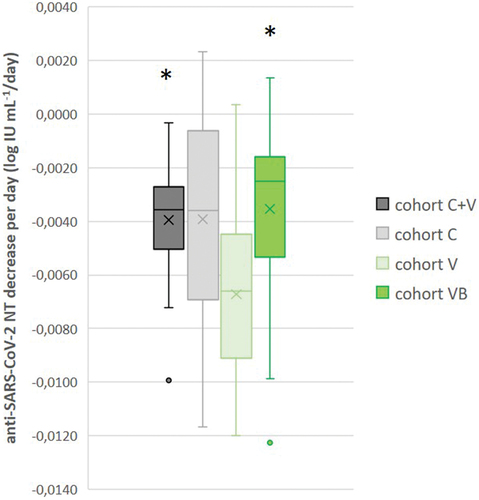
Discussion
We analyzed the functional antibodies of vaccinated and COVID-19 convalescents, processed with a standardized neutralization assay, and showed that those who recovered from COVID-19 were armed with humoral immunity that was quantitatively comparable and qualitatively superior than the immunity of the entire population of vaccinated people. We compared the ability of four SARS-CoV-2 vaccines (BNT162b2, mRNA-1273, ChAdOx nCoV-19 and Ad26.COV2.S) to induce NAbs against infectious SARS-CoV-2 (lineage B.1.1.1) with our results of NAb responses in convalescents, which we reported earlier.Citation9 Comparative data of the immune response generated by the first four vaccines approved against COVID-19 in Europe are limited. Furthermore, there is a lack of studies that compare vaccine humoral response directly with the response in convalescents. To our knowledge, only two studies have compared the humoral immune response generated by these four vaccines directly.Citation7,Citation8 It is difficult to compare the large amount of published data on individual vaccines, obtained using different neutralization test formats and using different and variable levels of standardization. In order to compare data from different studies Khoury et al.Citation6 pushed their efforts toward normalization of NTs from different assays to the mean convalescent titer obtained by the same assay in the same study. A comparison of such normalized values indicate that the highest NTs in the population were induced by mRNA vaccines, which were higher than the NT induced in COVID-19 convalescents. According to these data both adenovirus-based vaccines induced a similar NT response, which was lower in comparison to the one induced by both mRNA vaccines as well as by COVID-19 infection. In contrast, data from our study showed that the ChAdOx nCoV-19 vaccine induced a similar NT response to the COVID-19 infection (mild to severe, excluding the most severe patients requiring hospitalization) and a significantly higher response compared to the one induced by Ad26.COV2.S. The same approach used in another study resulted in quite comparable vaccine immunogenicity estimation to our data.Citation5 They estimated that ChAdOx nCoV-19 vaccine induced NT to the same extent as the COVID-19 infection, while NT induced by Ad26.COV2.S was lower, and the highest NT induced was by both mRNA vaccines. Karbiener et al. also recently reported comparable NTs measured after ChAdOx nCoV-19 vaccination and after recovery from COVID-19, obtained by the usage of wild-type SARS-CoV-2 neutralization assay for the analysis.Citation12 Moreover, since their assay was calibrated to the first WHO International standard like ours, the NT values they obtained were in perfect agreement to our results. Namely, in their study, the NT means for ChAdOx nCoV-19, BNT162b2, and convalescent group were 202 IU mL−1, 557 IU mL−1, and 140 IU mL−1, respectively, and they perfectly matched our corresponding results of 208 IU mL−1, 1123 IU mL−1 and 104 IU mL−1 Citation12.
From the extensive amount of literature, we can conclude that the various tests used to assess humoral immunity induced by vaccines and/or infection itself are precisely the cause of the incomparability of the tests themselves. More specifically, both pseudovirus neutralization assays or antibody binding assays lack all but selected specific virus proteins or epitopes from SARS-CoV-2, meaning they can only be neutralized by, e.g., S- or N- or RBD-specific antibodies, and the organization of those proteins may not be representative of authentic virus particles.Citation13 The most significant advantage of the authentic, wild-type neutralization assay is that it ensures that all antigens of SARS-CoV-2, that could be targeted by antibodies, are already on the surface of the challenge virus in the correct, physiological conformation.Citation14 The lower sensitivity of the pseudovirus neutralization assay might be the reason why no difference in antibodies was observed by Goldblatt et al.Citation7 between ChAdOx nCoV-19 and Ad26.COV2.S in contrast to our clearly observed significant difference in antibodies neutralizing wild-type SARS-CoV-2. Most of the results in Ad26.COV2.S group and many in the ChAdOx-1 nCoV-9 group were below the limit of detection in pseudoviral neutralization assay, while in our wild-type SARS-CoV-2 neutralization assay, only one sample in Ad26.COV2.S cohort provided result below the detection limit. In the same study of Goldblatt et al.,Citation7 binding antibody assay directed toward S and RBD proteins showed that mRNA-1273 induced a significantly higher amount of antibodies compared to the BNT162b2 vaccine, which is in contrast to our results. However, when they used mRNA-1273 data to estimate the protective threshold level from reverse cumulative distribution (RCDs) functions made from data on binding antibody quantities and known and published mRNA-1273 vaccine efficacies, the protective threshold calculated from the mRNA-1273 group stood out as outlier in these calculations, indicating that values obtained might be overestimated in their study. The results of another study were consistent with ours in the comparison of responses to four vaccines of interest, although the poor correlation of results between the binding assay and the pseudovirus assay used in the response assessment should be noted.Citation8 A confusing result was the equal amount of S protein-specific binding antibodies developed after the second and third BNT162b2 and mRNA-1273 vaccines, in contrast to pseudovirus NTs, which were significantly higher after the third compared to the second vaccine. Such finding implies that neutralization activity in vaccinees would not be correlated to S protein-specific IgG quantity, which would be hard to explain. In contrast, we found a perfect correlation between S protein-specific antibody quantity and SARS-CoV-2 NT in vaccinees described by polynomial function ().
Subsequent immunization of convalescents by vaccination modulates the immune response to SARS-CoV-2. Furthermore, booster vaccines evened out the different responses to primary vaccination cycles developed with four tested and used vaccines. Our data indicate that a single injection of any vaccine in previously infected COVID-19 individuals induced high, plateau-like level of SARS-CoV-2 neutralizing antibodies, which are significantly higher compared to the ones induced either by primary vaccination or developed in COVID-19 convalescents. Second vaccine dose in those with prior infection had no direct benefits for seropositive individuals, given the minimal further increase in neutralizing antibodies, consistent with similar early observations by others.Citation15–20 A significant boost of naturally acquired immunity occurs with the first dose, although it remains to be established whether this increase translates into improved protection from reinfection or simply reflects the secondary immune response to recall antigen, which would be protective per se.
Robust and efficient antibody responses in COVID-19 convalescents were generated after a single shot of vaccination, irrespective of whether received 2 to 14 months after recovery, supporting the observation by Jahrsdorfer et al. that immune memory did not significantly decrease within the observed time span.Citation21 Antibody trajectories after vaccination differed substantially by prior infection status. The longer durability of neutralizing antibodies was demonstrated in convalescents with or without an additional dose of the vaccine, as well as in naïve individuals who received the third booster vaccine dose in comparison to antibodies generated after the primary vaccination cycle, supporting published early observations.Citation16,Citation19,Citation20,Citation22
Besides quantitative, the usage of wild-type virus as a challenge in the neutralization assay enabled the detection of qualitative differences in the response developed after infection and vaccination. First, we observed significant differences in complement-mediated virus neutralization. The plasma complement system is composed of heat-labile proteins that activate each other in a cascade series of reactions. This cascade can be activated through three pathways – classical (activated in majority of cases by virus-specific antibodies), lectin, and alternative, all three described to be triggered by viruses.Citation23 Considering its role in immunity to viruses, complement contributes to virus elimination, owing to direct neutralization of cell-free viruses, among other mechanisms nicely reviewed by Agrawal et al.Citation23 Due to the heat-labile nature, complement components are easily inactivated by incubation at 56°C for 30 min, a procedure that does not affect thermally stable IgGs, as demonstrated also for SARS-CoV-2-specific IgG.Citation24 That allowed us to study SARS-CoV-2 neutralization in each serum sample comparatively – in the native form (neutralization due to both IgG and complement activity) and after complement inactivation (neutralization due to antibody activity only). We observed a significant drop in neutralization activity upon heat-inactivation in sera of both convalescentsCitation9 and vaccinees. Naïve, nonimmune human samples did not demonstrate any measurable SARS-CoV-2 neutralizing activity, indicating that complement-mediated neutralization triggered by other mechanisms, without the involvement of specific antibodies, could not be measured by our assay. Hence, we attributed difference in neutralization activity in native versus heat-inactivated sera of SARS-CoV-2 immune individuals to complement-mediated virus neutralization, triggered by SARS-CoV-2-specific antibodies to the virus surface proteins (hence via classical pathway (CP)). Complement component C3b and C4b deposition has already been demonstrated upon antibody binding to SARS-CoV-2 spike protein or RBD-coated ELISA plates, supporting CP activation by S-specific immunoglobulins.Citation25,Citation26 We could speculate that convalescents probably contain in their blood immunoglobulins targeting also other proteins expressed at the surface of SARS-CoV-2 (M and/or E), not only S protein as vaccinees. It is likely that the mixture of anti-S, anti-E, and anti-M antibodies results in a denser coverage of the virus surface with IgGs, favoring the clustering of IgGs required for C1q component binding and consequently the activation of the classical complement pathway.Citation27,Citation28
Although S protein has been considered the major target for virus neutralization, we observed that the lower quantity of anti-S protein IgG was required for the same neutralizing potency in convalescents, as opposed to vaccinees. A similar observation was made by Anichini et al.Citation17 Such finding further supported that antibodies of other specificities and/or classes might be involved in virus neutralization and that such antibodies are generated after recovery from the disease, while not after vaccination targeting single protein. Kurtović et al. demonstrated that SARS-CoV-2-specific IgM antibodies significantly contribute to virus neutralization and are present in different quantities in sera of recovered persons.Citation29 Wei et al. Citation22 also confirmed that the lower antibody levels produced in natural infection resulted in greater protection at the given antibody level compared to vaccination, reviewing assumption that the level of protection is associated with the antibodies and is dependent on the mechanism (infection or vaccination) generating the antibodies.
Our past experience throughout the history of the human race has taught us that in most infectious diseases, recovery from the disease, particularly its severe form, generates effective immunity. The evidence confirms that the same is true for COVID-19 disease.Citation30,Citation31 Vaccination is a public health strategy that aims to stimulate a more or less equally effective immunity, which should provide protection from at least severe disease, if not from infection at all. Vaccination against COVID-19 was undoubtedly the most effective strategy to combat COVID-19 epidemics, caused by the novel SARS-CoV-2, to which the complete population was naïve and vulnerable. However, our data on quantity and quality of SARS-COV-2 neutralizing antibodies indicated that higher stringency of public policy measures toward those convalescing from COVID-19 compared to those vaccinated were not fully justified. The most important lesson for the future may be that proper, reliable and standardized monitoring of pathogen-specific immunity must be one of the primary goals in emerging epidemics. Also, broad monitoring of induced immunity after vaccination and recovery with such standardized and relevant tests is of paramount importance for proper management of epidemic situations.
Limitation of the study
Complement-activating properties in our experimental setup are detectable only in sera with neutralizing titers below 100 IU mL−1. This phenomenon could be seen in , where complement activity index (the ratio between NTs in native and heat-inactivated form of each sample) is decreasing as the NT is increasing, finally approaching 1 (no difference in NTs measured in native and heat-inactivated form). The phenomenon is due to the intrinsic property of the SARS-CoV-2 neutralization assay (elaborated in detail in Results section), so only low-titer sera samples were included in this analysis, while majority of sera samples collected after second vaccine dose and booster immunization were excluded from the analysis of complement-activating properties of SARS-CoV-2 specific antibodies.
Supplemental Material
Download PDF (1.2 MB)Acknowledgments
The authors are grateful to Renata Jug and Stjepan Mitrović for the excellent technical assistance, as well as to Višnjica Šaronja, Dominka Kordić and Marijana Lukša for their expert help in volunteer blood sampling and storing. Particular gratitude goes to volunteers who provided blood for this research.
Disclosure statement
The authors declare that the research was conducted in the absence of any commercial or financial relationships that could be construed as a potential conflict of interest.
Data availability statement
The data generated in this study are provided in the Supplementary Material https://doi.org/10.1080/21645515.2023.2270310.
Supplementary data
Supplemental data for this article can be accessed on the publisher’s website at https://doi.org/10.1080/21645515.2023.2270310.
Additional information
Funding
References
- Wu F, Zhao S, Yu B, Chen YM, Wang W, Song ZG, Hu Y, Tao ZW, Tian JH, Pei YY, et al. A new coronavirus associated with human respiratory disease in China. Nature. 2020;579(7798):265–11. doi:10.1038/s41586-020-2008-3.
- Medicines and Healthcare products Regulatory Agency. Regulatory approval of Pfizer/BioNTech vaccine for COVID-19. 2020.
- Knezevic I, Mattiuzzo G, Page M, Minor P, Griffiths E, Nuebling M, Moorthy V. WHO International standard for evaluation of the antibody response to COVID-19 vaccines: call for urgent action by the scientific community. Lancet Microbe. 2022;3(3):e235–40. doi:10.1016/S2666-5247(21)00266-4.
- Bernasconi V. Use WHO antibody standards for emergency vaccine testing. Nature. 2023;613(7943):243. doi:10.1038/d41586-023-00007-2.
- Earle KA, Ambrosino DM, Fiore-Gartland A, Goldblatt D, Gilbert PB, Siber GR, Dull P, Plotkin SA. Evidence for antibody as a protective correlate for COVID-19 vaccines. Vaccine. 2021;39(32):4423–8. doi:10.1016/j.vaccine.2021.05.063.
- Khoury DS, Cromer D, Reynaldi A, Schlub TE, Wheatley AK, Juno JA, Subbarao K, Kent SJ, Triccas JA, Davenport MP. Neutralizing antibody levels are highly predictive of immune protection from symptomatic SARS-CoV-2 infection. Nat Med [Internet]. 2021;27(7):1205–11. doi:10.1038/s41591-021-01377-8.
- Goldblatt D, Fiore-Gartland A, Johnson M, Hunt A, Bengt C, Zavadska D, Snipe HD, Brown JS, Workman L, Zar HJ, et al. Towards a population-based threshold of protection for COVID-19 vaccines. Vaccine. 2022;40(2):306–15. doi:10.1016/j.vaccine.2021.12.006.
- Van Gils MJ, Lavell A, van der Straten K, Appelman B, Bontjer I, Poniman M, Burger JA, Oomen M, Bouhuijs JH, van Vught LA, et al. Antibody responses against SARS-CoV-2 variants induced by four different SARS-CoV-2 vaccines in health care workers in the Netherlands: a prospective cohort study. PLoS Med [Internet]. 2022;19(5):1–21. doi:10.1371/journal.pmed.1003991.
- Ravlić S, Hećimović A, Kurtović T, Ivančić Jelečki J, Forčić D, Slović A, Kurolt IC, Mačak Šafranko Ž, Mušlin T, Rnjak D, et al. Is better standardization of therapeutic antibody quality in emerging Diseases epidemics possible? Front Immunol. 2022;13:1–12. doi:10.3389/fimmu.2022.816159.
- Kristiansen PA, Page M, Bernasconi V, Mattiuzzo G, Dull P, Makar K, Plotkin S, Knezevic I. WHO International standard for anti-SARS-CoV-2 immunoglobulin. Lancet. 2021;397(10282):1347–8. doi:10.1016/S0140-6736(21)00527-4.
- Brgles M, Kurtović T, Lang Balija M, Hećimović A, Mušlin T, Halassy B. Impact of complement and difference of cell-based assay and ELISA in determination of neutralization capacity against mumps and measles virus. J Immunol Methods [Internet]. 2021;490:112957. doi:10.1016/j.jim.2021.112957.
- Karbiener M, Farcet MR, Zollner A, Masuda T, Mori M, Moschen AR, Kreil TR. Calibrated comparison of SARS-CoV-2 neutralizing antibody levels in response to protein-, mRNA-, and vector-based COVID-19 vaccines. NPJ Vaccines. 2022;7(1):2–4. doi:10.1038/s41541-022-00455-3.
- Wohlgemuth N, Whitt K, Cherry S, Kirkpatrick Roubidoux E, Lin C-Y, Allison KJ, Gowen A, Freiden P, Allen EK, Gaur AH, et al. An assessment of serological assays for SARS-CoV-2 as surrogates for authentic virus neutralization. Microbiol Spectr. 2021;9(2). doi:10.1128/Spectrum.01059-21.
- Lippi G, Adeli K, Plebani M. Commercial immunoassays for detection of anti-SARS-CoV-2 spike and RBD antibodies: urgent call for validation against new and highly mutated variants. Clin Chem Lab Med. 2022;60(3):338–42. doi:10.1515/cclm-2021-1287.
- Saadat S, Rikhtegaran Tehrani Z, Logue J, Newman M, Frieman MB, Harris AD, Sajadi MM. Binding and neutralization antibody titers after a single vaccine dose in health care workers previously infected with SARS-CoV-2. JAMA. 2021;325:1467–8. doi:10.1001/jama.2021.3341.
- Krammer F, Srivastava K, Alshammary H, Amoako AA, Awawda MH, Beach KF, Bermudez-Gonzalez MC, Bielak DA, Carreño JM, Chernet RL. Antibody responses in seropositive persons after a single dose of SARS-CoV-2 mRNA vaccine. N Engl J Med. 2021;384(14):1372–4. doi:10.1056/NEJMc2101667.
- Anichini G, Terrosi C, Gandolfo C, Savellini GG, Fabrizi S, Miceli GB, Cusi MG. SARS-CoV-2 antibody response in persons with past natural infection. N Engl J Med. 2023;385:90–2. doi:10.1056/NEJMc2103825.
- Manisty C, Otter AD, Treibel TA, McKnight Á, Altmann DM, Brooks T, Noursadeghi M, Boyton RJ, Semper A, Moon JC. Antibody response to first BNT162b2 dose in previously SARS-CoV-2-infected individuals. Lancet. 2021;397:1057–8. doi:10.1016/S0140-6736(21)00501-8.
- Vicenti I, Gatti F, Scaggiante R, Boccuto A, Zago D, Basso M, Dragoni F, Parisi SG, Zazzi M. The second dose of the BNT162b2 mRNA vaccine does not boost SARS-CoV-2 neutralizing antibody response in previously infected subjects. Infection [Internet]. 2022;50(2):541–3. doi:10.1007/s15010-021-01680-z.
- Claro F, Silva D, Rodriguez M, Rangel HR, De JH Since January 2020 Elsevier has created a COVID-19 resource centre with free information in English and mandarin on the novel coronavirus COVID- 19. The COVID-19 resource centre is hosted on Elsevier Connect, the company ’ s public news and information. 2020;
- Jahrsdörfer B, Fabricius D, Scholz J, Ludwig C, Grempels A, Lotfi R, Körper S, Adler G, Schrezenmeier H. BNT162b2 vaccination elicits strong serological immune responses against SARS-CoV-2 including variants of concern in elderly convalescents. Front Immunol. 2021;12:1–13. doi:10.3389/fimmu.2021.743422.
- Wei J, Pouwels KB, Stoesser N, Matthews PC, Diamond I, Studley R, Rourke E, Cook D, Bell JI, Newton JN, et al. Antibody responses and correlates of protection in the general population after two doses of the ChAdOx1 or BNT162b2 vaccines. Nat Med. 2022;28(5):1072–82. doi:10.1038/s41591-022-01721-6.
- Agrawal P, Nawadkar R, Ojha H, Kumar J, Sahu A. Complement evasion strategies of viruses: an overview. Front Microbiol. 2017;8:1–19. doi:10.3389/fmicb.2017.01117.
- Pastorino B, Touret F, Gilles M, De Lamballerie X. Heat inactivation of different types of SARS-CoV-2 samples: what protocols for biosafety, molecular detection and serological diagnostics? Viruses. 2020;12:6–13. doi:10.3390/v12070735.
- Jarlhelt I, Nielsen SK, Jahn CXH, Hansen CB, Pérez-Alós L, Rosbjerg A, Bayarri-Olmos R, Skjoedt MO, Garred P. SARS-CoV-2 antibodies mediate complement and cellular driven inflammation. Front Immunol. 2021;12:1–11. doi:10.3389/fimmu.2021.767981.
- Lamerton RE, Marcial-Juarez E, Faustini SE, Perez-Toledo M, Goodall M, Jossi SE, Newby ML, Chapple I, Dietrich T, Veenith T, et al. SARS-CoV-2 Spike- and nucleoprotein-specific antibodies induced after vaccination or infection promote classical complement activation. Front Immunol. 2022;13:1–13. doi:10.3389/fimmu.2022.838780.
- Merle NS, Church SE, Fremeaux-Bacchi V, Roumenina LT. Complement system part I - molecular mechanisms of activation and regulation. Front Immunol. 2015;6:1–30. doi:10.3389/fimmu.2015.00262.
- Jegerlehner A, Storni T, Lipowsky G, Schmid M, Pumpens P, Bachmann MF. Regulation of IgG antibody responses by epitope density and CD21-mediated costimulation. Eur J Immunol. 2002;32(11):3305–14. doi:10.1002/1521-4141(200211)32:11<3305:AID-IMMU3305>3.0.CO;2-J.
- Kurtović T, Ravlić S, Štimac A, Mateljak Lukačević S, Hećimović A, Kazazić S, Halassy B. Efficient and sustainable platform for preparation of a high-quality immunoglobulin G as an urgent treatment option during emerging virus outbreaks. Front Immunol. 2022;13:1–11. doi:10.3389/fimmu.2022.889736.
- Andreano E, Paciello I, Piccini G, Manganaro N, Pileri P, Hyseni I, Leonardi M, Pantano E, Abbiento V, Benincasa L, et al. Hybrid immunity improves B cells and antibodies against SARS-CoV-2 variants. Nature. 2021;600(7889):530–5. doi:10.1038/s41586-021-04117-7.
- Stein C, Nassereldine H, Sorensen RJD, Amlag JO, Bisignano C, Byrne S, Castro E, Coberly K, Collins JK, Dalos J, et al. Past SARS-CoV-2 infection protection against re-infection: a systematic review and meta-analysis. Lancet. 2023;401(10379):833–42. doi:10.1016/S0140-6736(22)02465-5.