ABSTRACT
For decades, immunotherapies have offered hope for patients with advanced cancer. However, they show distinct benefits and limited clinical effects. Tumor vaccines have the potential to prime tumor-antigen-specific T cells and induce broad subsets of immune responses, ultimately eradicating tumor cells. Here, we classify tumor vaccines by their anti-tumor mechanisms, which include boosting the immune system, overcoming tumor immunosuppression, and modulating tumor angiogenesis. We focus on multidimensional tumor vaccine strategies using combinations of two or three of the above mechanisms, as these are superior to single-dimensional treatments. This review offers a perspective on tumor vaccine strategies and the future role of vaccine therapies in cancer treatment.
Introduction
Although surgical resection, chemotherapy, and radiotherapy still play important roles in tumor treatment, the powerful therapeutic ability of tumor vaccines has attracted the attention of scholars. Tumor vaccines use tumor antigens to activate the immune system, provoking both specific cellular immunity and a humoral immune response to prevent tumor growth and ultimately eradicate tumor cells.Citation1 Many studies have shown that tumor vaccines can not only prevent or slow the occurrence and development of tumors and prolong survival, but can also prevent the growth, angiogenesis, and even metastasis of established tumors. The core mechanism of tumor vaccine therapy is the induction of an active immune response to antigens or cells by delivering the target antigen or cells to the body, thereby inducing cytotoxic T cells and serum antibodies to specifically kill target cells and antigens, and establishing an immune microenvironment for tumor inhibition. Th1 cytokines (IFN- γ, TNF- α, IL-2) tend to show high levels during this process.Citation2,Citation3 The intensity of the cellular immune response or humoral immune response produced by the immune system is limited by many factors, including the immunogenicity of the tumor vaccine, the treatment of tumor vaccine by antigen-presenting cells, the immune tolerance of the body to the tumor vaccine, the integrity of the immune system, and the malignancy of the tumor. In order to overcome inherent resistance, researchers have developed tumor vaccines with different target cells and various antigen forms and have combined the use of adjuvants and DC ligands to modify or co-express DC-activating molecules to enhance the immune response to tumor vaccines. The most direct way to induce an immune response to a tumor is to deliver proteins characteristic of tumor cells or lysates of tumor cells to the immune system. However, tumor-specific antigens are often difficult to identify because the vast majority of molecular antigens of tumor cells are common to human cells, meaning that the immune system cannot recognize them as foreign antigens. Moreover, tumor cells have high mutagenicity, which creates limitations and challenges for tumor vaccines that directly target tumor cells. Therefore, we focus on the potential and development of tumor vaccines that activate the tumor immune microenvironment and inhibit tumor angiogenesis. Tumor vaccines induce the activation of effector T cells that kill tumor cells, weaken the functional activity of Treg in tumors, and enhance tumor immunity, making it difficult for tumor cells to continue to grow. Additionally, tumor vaccines inhibit tumor angiogenesis, assist tumor treatment, and allow clinical possibilities to prevent tumor growth and prolong survival. Tumor angiogenesis provides the key source of nutrition for tumor cells to survive, thus blocking it is critical. As tumor development and metastasis is a complex process involving multiple environments and cells, single-treatment tumor vaccines still face the problems of drug resistance, tumor cell variation and compensatory mechanisms. Therefore, to take full advantage of therapeutic tumor vaccines, greater efforts are needed to explore and develop multidimensional tumor vaccines. Different forms of tumor vaccines such as adenovirus vaccines, DNA vaccines, mRNA vaccines, protein vaccines, polypeptide vaccines, and whole-cell vaccines show different degrees of anti-tumor immune response. The selection of antigen type is important to allow multidimensional targeting by tumor vaccines.
Tumor vaccines based on boosting the immune system
Boosting the immune system is an important goal in cancer immunotherapy. To achieve this goal, several immunotherapies have been developed. In this section, we focus on the research status of tumor vaccines that induce and enhance the anti-tumor effects of CD8+ and CD4+ T cells. illustrates the anti-tumor mechanism of tumor vaccine strategies based on boosting the immune system.
Figure 1. Tumor vaccine strategies based on boosting the immune system. Different types of tumor vaccines are inoculated and presented by DC to activate the CD8+ T cells, CD4+ T cells and B cells to kill tumor cells directly or indirectly. Abbreviations: DC: dendritic cells; ICAM-1: intercellular cell adhesion molecule-1; CTL: cytotoxic T cells; MHC: Major histocompatibility complex; IFN-γ: interferon γ; GzmB: granzyme B; PFN: perforin; TAMs: tumor-associated macrophages.
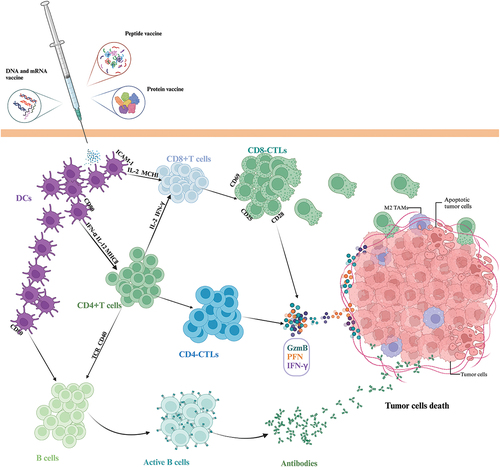
Cross-presentation
The cross-presentation pathway is an antigen presentation pathway by antigen-presenting cells (APC) to exogenous antigens, that is, exogenous antigens form complexes with MHC class I molecules after being treated with APC and are then presented to CD8+ T cells.Citation4 Through the cross-presentation pathway, exogenous antigens in tumor vaccines can be presented to CD8+ T cells, thus inducing them to kill the target cells. APCs, particularly dendritic cells (DCs), are essential for presenting tumor antigens on HLA-I to CD8+ T cells.Citation5,Citation6
Many studies have been devoted to promoting the cross-presentation of DCs to induce the cytotoxic functions of tumor-specific CD8+ T cells. Enhancing the cross-presentation of antigens promotes the initiation of tumor immune responses. DCs are immature before being exposed to antigens, and only mature DCs can effectively initiate antigen-specific T cell responses. There are two ways to promote DC maturation: one is antigenic stimulation and the other is adjuvant. Dc-based cancer vaccines are typically loaded with antigens to immature DC, combined with the use of adjuvants. Dc-based cancer vaccines have been well discussed in other articles.Citation7 One notable result was SiPuleucel-T, the first vaccine approved by the FDA in 2010 for the treatment of early-stage asymptomatic metastatic trend-resistant prostate cancer. In a randomized placebo-controlled trial involving 512 men with metastatic castration-resistant prostate cancer, a dendritic cell-based vaccine (sipuleucel-T) significantly improved overall survival in men with metastatic castration-resistant prostate cancer.Citation8 The vaccine extracts APCs from the patient’s peripheral blood and pulses them with a fusion protein consisting of GM-CSF and human prostatic acid phosphatase (PAP), stimulating them and inducing them to mature before being injected back into the patient to trigger a systemic antigen-specific T cell response.Citation9–11 However, host factors, such as race population, and the lack of prostate specific antigen (PSA) or radiographic response in clinical trials negatively impact the clinical benefit of sipuleucel-T.Citation12 Cytoplasmic transduction peptides (CTP) were successfully used to mediate the localization of BCR-ABL protein-derived antigen peptides in the cytoplasm of dendritic cells, which stimulated a cross-antigen presentation reaction in dendritic cells, thereby presenting exogenous antigens to CD8+ T lymphocytes and inducing a specific cellular immune response in chronic myeloid leukemia, ultimately killing BP210 target cells.Citation13 Based on the existing anti-tumor vaccine of DC cells, this experiment introduced CTP into the immunotherapy of CML to carry out DC antigen loading, thus stimulating the anti-CML effect. This provides a theoretical basis for dendritic cell immunotherapy. DC subsets are usually divided into five categories according to their developmental origin: conventional DC (including type 1 conventional DC (cDC1) and type 2 conventional DC (cDC2)), monocyte derived DC (MoDC), plasma cell-like DC (pDC) and a new type of DC (DC3).Citation14 Choosing the right DC subgroup is crucial. In a mouse melanoma model, CD103+ DCs were the only APCs used to prime tumor-specific CD8+ T cells, and injection of a cytokine-adjuvant vaccine led to the major expansion of CD103+ DC precursor cells and immature CD103+ DC cells at the tumor site, thus promoting anti-tumor immunity.Citation15 CD103+ DCs has been proved to be the main antigen presenting cell involved in the initiation of anti-tumor T cells.Citation16 Good adjuvants stimulate DC antigen cross presentation and DC maturation at the same time.There are two main DC cross pathways related to vaccine adjuvant action: the vacuolar pathway and the cytosolic pathway.Citation17 It is feasible to enhance the effectiveness of CD8+ T cell response by using different vaccine adjuvants that participate in these two pathways. Studies on the administration of adjuvants and chemokines and on targeting strategies to activate, recruit, and deliver antigens to cross-presenting dendritic cells are summarized in detail in.Citation18 In addition, various types of nanoparticle vaccines to improve cross-presentation have been developed and studied. Several research teams have designed nanoparticle vaccines that contain interferon gene stimulator (STINT) agonists to stimulate interferon-I production, leading to more antigen cross-stimulation and stronger downstream CD8+ T cell responses.Citation19 Boosting the cross-presentation of antigens in tumor vaccines to CD8+ T cells is a promising strategy for cancer immunotherapy, however, the selection of the best antigen, suitable DC subsets and adjuvants has always been the direction of researchers’ efforts.
Cytotoxic CD8+ T cells
The discovery of tumor-associated antigens (TAA) has led to the development of therapeutic tumor vaccines based on synthetic peptides, DNA, DCs or recombinant proteins in an attempt to enhance the anti-tumor immune response, especially that of tumor antigen-specific cytotoxic T cells (CTL). Cytotoxic CD8+ T cells (CD8-CTLs) play an important role in tumor immunity.
Most peptide vaccines are based on epitope peptides that stimulate CD8+ T cells to target tumor-associated antigens (TAA) or tumor-specific antigens (TSA).Citation20 Short peptides (8–11 amino acids) can directly bind to human leukocyte antigen class I molecules to enhance MHC-I-restricted antigen-specific CD8+ T cellular immunity. When combined with effective adjuvants, long-peptide vaccines can usually produce more extensive immunity than short-peptide vaccines by inducing responses from both CD8-CTLs and CD4+ helper T cells. A study on the immunity induced by MHC I epitopes of tumor antigen SIM2 showed that a single peptide containing multiple SIM2 epitopes can be used to induce CD8+ CTL responses, providing a peptide-based vaccine formula for immunotherapy for various cancers.Citation21 The first generation of tumor vaccines is based on non-mutant TAAs, which are also known as shared antigens, as they are expressed in many tumors. They include MART-1, gp100, tyrosinase, Trp-2, NY-ESO-1, MAGE-A3, and Her2/neu or telomerase proteins. They have been shown to be immunogenic and able to induce clinical responses in advanced cancer patients. Alpha-fetoprotein (AFP), a tumor-specific antigen, is an important index for the clinical diagnosis of hepatocellular carcinoma. AFP-specific CD8+ T cells activated by DCs showed significant anti-tumor immune activity in vitro.Citation22 In an invasive B16F10 melanoma model, CD8+ T cells were strongly activated after a single immunization with a liposome-assisted mRNA vaccine. In a melanoma model of transgenic mice, the vaccine induced the proliferation of not only CD8+ T cells but also killer cells, which prolonged the overall survival time.Citation23 An individualized mRNA neoantigen vaccine, in combination with atezolizumab and mFOLFIRINOX induces substantial T cell activity in patients with surgically resected PDAC that correlates with delayed recurrence.Citation24 Preclinical studies demonstrated that a DNA vaccine (pTVG-AR, MVI-118) encoding the androgen receptor ligand-binding domain (AR LBD) augmented antigen-specific CD8+ T cells, delayed prostate cancer progression and emergence of castration-resistant disease, and prolonged survival of tumor-bearing mice. PTVG-AR was demonstrated to be safe and immunologically active in patients with mCSPC in a phase I clinical trial. And a prospective evaluation will be carried out in future trials.Citation25 A key challenge for nucleic acid vaccines is how to protect them from degradation before reaching the target. To this end, scientists are using a variety of nanomaterials for delivery to protect them from degradation. Lipid Nanoparticles and Liposomes has become the preferred drug delivery system (DDS, drug delivery system) for gene therapy and other complex injection drug products, representing the future of nanomedicine.Citation26 Studies have shown that, as a new type of viral vector, CMV (Cytomegalovirus) can induce different CD8+ T cell responses, and the tumor-specific CMV vector vaccine can induce a large number of CMV-specific CD8+ T cells.Citation27,Citation28 Adenovirus-based vectors play an important role in promoting T cell broadening, expansion, and differentiation to maintain anti-tumor response. Development of virus-based vector vaccines, researchers have developed a fast process for producing viral vectors encoding 60 unique patient-specific neoantigens and recently initiated a clinical trial in patients with metastatic melanoma and non – small cell lung cancer.Citation29 A promising ISV (in situ vaccination) strategy is to use oncolytic virus, which can not only induce immunogenic cell death to load tumor Ag, but also stimulate pro-inflammatory signals to activate DC.Citation30 This strategy can be quickly translated into clinical practice. At present, it has entered the phase I clinical trial (NCT03889275) (NCT04613492). Advances in rapid genome sequencing have led to the development of personalized cancer vaccines (PCV) for tumor-specific mutations known as new antigens. Early studies in mice and phase I clinical trials have proven the feasibility of PCV to produce T-cell responses to new antigens. New antigen peptides were ligated to TLR7/8 agonists (SNP-7/8a) by a self-assembled nanoparticle vaccine. Intravenous administration of SNP-7/8a produced TCF1+PD-1+CD8+ T cells with anti-tumor ability after anti-PD-L1 treatment and mediated anti-tumor effects dependent on immune checkpoint inhibition.Citation31 A nanoparticle (NP) vaccine significantly enhanced the activation of dendritic cells and antigen cross-presentation, resulting in the strong activation of new-antigen-specific CD8+ T cells. After a single subcutaneous injection, the frequency of CD8+ T cells in systemic circulation was as high as 23 ± 7%. An NP vaccine combined with STING agonist treatment eliminated tumors in MC-38 colon cancer and B16F10 melanoma mice and established long-term immune memory. These studies present a new treatment strategy based on combined nano-immunotherapy for personalized tumor immunotherapy.Citation32 A retrospective study of seven patients with advanced pancreatic cancer showed that patients with longer overall survival had higher titers of interferon-γ and CD8+ effect memory T cells in their peripheral blood after vaccination with a new antigen peptide vaccine, indicating that this new antigen peptide vaccine had the potential to activate of specific T cell subsets to kill cancer cells.Citation33 The gene mutation of tumor is very important in the process of T cell activation, because the gene mutation can produce new antigens, which are more easily recognized and attacked by the immune system. Because of this, the higher the mutation frequency of tumor cells, the more the number of new antigens produced, and tumors with this characteristic tend to respond better to immunotherapy. Many studies have shown that the new antigen vaccine has the potential to induce anti-tumor immunity.Citation34
Effective CD4+ T cell responses
Tumor reactive CD4+ helper T cells
CD4+ T cells are well known for their auxiliary effects. For example, CD4+ T cells are involved in the regulation of anti-tumor immunity by helping to initiate and enhance the cytotoxic activity of CD8+ T cells. Although CD8+ T cells were initially considered to be the primary acting cells, it is now clear that CD4+ T cells also play a key role in the anti-tumor response. Tumor-reactive CD4+ helper T cells (Th1) produce interferon-γ, tumor necrosis factor-α, and IL-2, which play key roles in cell-mediated anti-tumor immunity. Newly identified telomerase reverse transcriptase (TERT)-derived peptides are known as universal cancer peptides (UCP). UCP vaccines can induce high affinity and tumor-specific CD4+ Th1 polarization.Citation35 By combining synthetic peptides with TLR agonists and OX40/CD40 co-stimulation, a major CD4+ T cell response can be produced. This vaccine strategy is effective in overcoming immune tolerance to self-tumor-associated antigens and had a significant anti-tumor effect in malignant melanoma mouse models.Citation36 The animal and clinical trials of tumor vaccines based on boosting immune response are listed in respectively.
Table 1. Experimental studies of tumor vaccines based on boosting the immune response.
Table 2. Clinical trials of tumor vaccines based on boosting the immune response.
Cytotoxic CD4+ T cells
In addition to conventional CD4+ T cells including Th1, Th2, Th17, peripherally- induced regulatory T cells, T follicular helper T cells (Tfh),Citation44,Citation45 there is a group of CD4 cells that express cytotoxic molecules such as perforin and granzyme, which are defined as cytotoxic CD4+ T cells (CD4-CTLs).Citation46
The earliest discovery of CD4-CTLs in cancer patients was reported in 2001Citation47; the number of CD4-CTLs expressing perforin was significantly increased in chronic B-cell lymphocytic leukemia patients compared with healthy controls.Citation47 Moreover, this group of cells can kill autogenous B-cell tumor cells dependent on the release of perforin without the involvement of FAS-FASL pathways or tumor necrosis factor.Citation48 In a melanoma mouse model, a small amount of tumor-reactive CD4+ T cells induced significant tumor regression, and the anti-tumor effect was more significant after blocking the co-suppressor molecule CTLA-4. This anti-tumor effect is achieved through MHC II molecule-restricted degranulation, which does not depend on apoptosis induced by the CD95-CD95L pathway or on the participation of CD8+ T cells, B cells, NK cells, or NKT cells. This suggests that CD4+ T cells have a strong killing effect.Citation49 Retrospective immunoanalysis of circulating T cells from melanoma patients treated with ipilimumab showed that an increase in the percentage of CD4-CTLs cells at 8–14 weeks is associated with positive clinical outcomes. CD4+ T cells established from peripheral blood mononuclear cells (PBMCs) of melanoma patients express granzyme B, perforin and LAMP (CD107a), and can lyse autologous melanoma cells in an MHC II-dependent manner.Citation50
The number of CD4-CTLs was increased in patients with early liver cancer but decreased significantly with the progression of the disease.Citation51 Through analysis of a comprehensive RNA-seq gene expression data set of neuroblastoma, the effector cell of high-risk neuroblastoma was determined to be CD4+CD3+NKG2C/E+CRTAM+, also known as CD4-CTLs. In 2021, it was found that the expression of Fas is not related to the survival of patients with high-risk neuroblastoma. On the contrary, the expression of GZMA/GZMB in CD4-CTLs is related to longer survival time in patients with high-risk neuroblastoma. The expression of OX40-encoding costimulatory molecules in CD4-CTLs is also associated with better outcomes. A similar trend was observed in 4-1BB, although its expression level was lower than that of OX40. These observations suggest that the anti-neuroblastoma effect of CD4-CTLs depends on the perforin/granzyme pathway.Citation52 In addition, CD4-CTLs were found in patients with other solid tumors, such as breast cancerCitation53 and osteosarcoma.Citation54
After adoptive transfer of CD4+Trp1+ cells to mice received 5 Gy of irradiation, the cells were rapidly expanded and differentiated into cytotoxic CD4+ T cells, which efficiently eliminated established melanoma tumors in a mouse model of advanced disease.Citation49 This study provided preliminary evidence of the clinical potential of CD4-CTL-based adoptive cellular therapy (ACT). A clinical trial reported that adoptive transferred amplified autologous CD4+ T cell clones with melanoma-associated antigen NY-ESO-1 specificity mediated a durable clinical response and caused an endogenous response to melanoma antigens other than NY-ESO-1.Citation55 Prostate cancer patients have received the AE37 vaccine (a HER2 hybrid peptide vaccine) to induce AE37-specific CD4-CTLs.Citation56 The anti-tumor effect of CD4-CTLs has also been shown in studies on dendritic cell vaccines combined with IL-12 for the treatment of liver cancer in mice. Overall, these studies show that CD4-CTLs play a role in anti-tumor therapy. Although the specific mechanism of their anti-tumor effect needs to be further explored, these findings emphasize the possibility of using CD4-CTLs in tumor immunotherapy.
Tumor vaccines based on overcoming tumor immunosuppression
Studies have identified multiple tumor vaccine strategies, but clinical adoption rates remain low due to the occurrence of immune-related adverse events. These events are related to immunosuppressive cells such as regulatory T cells (Tregs), myeloid-derived suppressor cells (MDSCs), tumor-associated neutrophils (TANs), tumor-associated macrophages (TAMs), and tumor-associated fibroblasts (CAFs) in the tumor microenvironment (TME).Citation57 This has led to an increasing interest in tumor vaccines that can overcome tumor immunosuppression. The anti-tumor mechanism of tumor vaccine strategies based on overcoming tumor immunosuppression is described in .
Figure 2. Tumor vaccine strategies based on overcoming tumor immunosuppression. Tumor vaccines reshape the tumor microenvironment by remodeling, repolarizing, gene silencing or target immunosuppressive cells, turning immunosuppression into immunostimulation. [Figure reproduced from bleve A, durante B, sica A, consonni FM. Lipid metabolism and cancer immunotherapy: immunosuppressive myeloid cells at the Crossroad. Int J Mol Sci. 2020 Aug 14; 21(16): 5845. doi: 10. 3390/ijms21165845. PMID: 32823961; PMCID: PMC7461616] Abbreviations: MDSC: myeloid-derived suppressor cells; TAN: tumor-associated neutrophils.
![Figure 2. Tumor vaccine strategies based on overcoming tumor immunosuppression. Tumor vaccines reshape the tumor microenvironment by remodeling, repolarizing, gene silencing or target immunosuppressive cells, turning immunosuppression into immunostimulation. [Figure reproduced from bleve A, durante B, sica A, consonni FM. Lipid metabolism and cancer immunotherapy: immunosuppressive myeloid cells at the Crossroad. Int J Mol Sci. 2020 Aug 14; 21(16): 5845. doi: 10. 3390/ijms21165845. PMID: 32823961; PMCID: PMC7461616] Abbreviations: MDSC: myeloid-derived suppressor cells; TAN: tumor-associated neutrophils.](/cms/asset/7527c597-d09d-42fc-9570-30700b7765fc/khvi_a_2271334_f0002_oc.jpg)
Inhibiting tregs
Tregs are a subset of T cells that have the function of suppressing excessive immune activation. They are present in all body tissues. Tregs in different organs exert different functions through different mediators; in addition to maintaining immune tolerance, Tregs also act as organ-specific suppressors of tumor immunity, thereby mediating tumor immune escape.Citation58,Citation59 An increased number of Tregs is the primary factor minimizing the protective effect of tumor vaccines and reducing the number of immunosuppressive cells in the TME. Therefore, eliminating them or weakening their function can enhance the immune response to tumor vaccines.Citation60
IMU-131/HER-Vaxx is a therapeutic B-cell epitope vaccine consisting of three fused B-cell epitopes from the HER2/neu extracellular domain coupled to CRM197 and adjuvanted with Montanide. In a gastroesophageal adenocarcinoma (GEA) phase Ib experiment, 14 patients with either gastric or gastroesophageal junction adenoma were enrolled. The effectiveness of the cancer vaccine, evaluated by progression-free survival, and tumor response, belonged to the exploratory endpoints of this phase Ib trial. IMU-131/HER-Vaxx induced a HER2-specific Ab and cellular immune response after inoculation, with a significantly reduced number of regulatory T cells and the reductions of Tregs were often in accordance with tumor regression. This may be due to the expansion of the T effect population, resulting in a relative decrease in Tregs in peripheral blood. Vaccine-induced Ab has functions similar to trastuzumab.Citation61 Trastuzumab is a widely used mAb targeting HER 2/neu EGF. The IMU-131/HER-Vaxx vaccine not only overcomes the shortcomings of passive antibody administration; it also overcomes the tumor-promoting effect of tumor immunosuppressive cells, making treatment more comprehensive.
A study of the CHP-MAGE-A4 cancer vaccine explored whether the treatment effect and immune response caused by cancer vaccines can be predicted by the percentage of CD4+CD25+Foxp3+ Tregs in peripheral blood mononuclear cells (PBMCs) after vaccination. The results show that the proportion of Tregs after vaccination seems to have predictive value for the prognosis of patients.Citation62 It has been found that the FoxP3 transcription factor, a specific intracellular marker of Tregs, helps distinguish the phenotype of suppressive CD4+CD25+ T cells from that of effector T cells.Citation63 Foxp3 is expressed not only in CD4+CD25+ and CD4+CD25− T cells but also in immunosuppressive CD8+T cells. Therefore, immunotherapy targeting Foxp3 was developed to achieve tumor immunity.Citation64 In B16 melanoma, Foxp3 silenced GM-CSF gene-modified tumor vaccine,Citation65 prophylactic Foxp3 DNA/recombinant protein vaccine (Foxp3 vaccine)Citation66 could target and inhibit Tregs in the TME to enhance the anti-tumor effect. Some studies have also found that the immunogenicity of tumor antigens can be enhanced when they are fused with segment C (Fc) of the immunoglobulin heavy chain to produce antigen-Fc fusion proteins such as Foxp3-Fc (IgG). Foxp3 silenced vaccine combined with other vaccines can stimulate higher immune responses than its single application.Citation67–69
Inhibiting other immunosuppression
In contrast to the Tregs-inhibiting tumor vaccines described above, some vaccines inhibit other immunosuppressors to generate anti-tumor immunity. A novel synthetic tyrosinase (Tyr) DNA vaccine improved the survival rate of melanoma mice by reducing the number of MDSCs in the TME and improving the inhibition environment.Citation70 Some studies have found that a dendritic cell-based hepatocellular carcinoma (HCC) neoadjuvant nano-vaccine can reshape TANs to enhance the anti-tumor immune response, thus enhancing the vaccine’s therapeutic effect.Citation71 A DC-shA20-FAP-TRP2 vaccine in a B16 melanoma model targeted cancer-associated fibroblasts (CAFs) in addition to cancer cells and may enhance vaccine-induced immunity and effects.Citation72 In other cases, such as in studies on DC and CAF fusion vaccines, FAD-modified transgenic tumor cell vaccines, etc., the accumulation of immunosuppressive cells in the TME was significantly reduced.Citation73,Citation74 We list tumor vaccines that reduce tumor immunosuppression in .
Table 3. Tumor vaccines based on overcoming tumor immunosuppression.
Tumor vaccines based on anti-angiogenesis
Professor J Folkman proposed that the growth and metastasis of solid tumors depend on neovascularization,Citation78,Citation79 which opens the door to study the mechanism of tumor growth and leads to extensive exploration of immune strategies against tumor angiogenesis. Immunotherapy against tumor angiogenesis can be divided into passive immunotherapy and active immunotherapy. The former focuses on angiogenesis-related molecules and their interaction with receptors. Using antibodies to neutralize pro-angiogenic factors and blocking pro-angiogenic factor receptors to reduce the proliferation and migration of tumor endothelial cells, which can quickly block tumor neovascularization. However, long-term anti-angiogenesis often requires repeated high-dose continuous administration. The latter induces specific immune responses directly targeting tumor endothelial cells and or tumor cells by inoculating the body with vaccines based on allogeneic endothelial cells, pro-angiogenic molecules or tumor-associated antigens involved in angiogenesis. The anti-tumor effects of these three anti-angiogenic vaccines last for a long time and have little side effects, showing excellent therapeutic advantages.Citation80 Among them, the third one has attracted the attention of scholars because of its combination of anti-tumor angiogenesis and anti-tumor mechanism that directly attacks tumor cells. The goal of tumor vaccines is usually to induce the immune system to produce adaptive immune responses targeting tumor cells expressing tumor-specific antigens or tumor-associated antigens in order to dissolve or kill these cells and eventually lead to tumor growth inhibition and tumor regression.Citation81–83 Different from most vaccines that rely on a single anti-tumor mechanism, tumor vaccines that inhibit tumor progression by multiple mechanisms are multi-dimensional targets for tumors and tumor microenvironment, and have strong and lasting anti-tumor ability. This part mainly describes the research progress and achievements of tumor vaccine based on tumor associated antigen involved in tumor angiogenesis in preclinical animal experiments and clinical trials, which is mediated by the combined anti-tumor mechanism of inhibition of intratumoral angiogenesis and direct destruction of tumor cells. To explore its prospect and importance as a tumor treatment strategy. It also emphasizes the therapeutic potential and advantages of this tumor vaccine with multiple mechanisms (anti-tumor angiogenesis, weakening tumor immunosuppression, enhancing anti-tumor immune function) to prevent tumor progression. shows the anti-tumor mechanism of tumor vaccine strategies based on anti-tumor angiogenesis.
Figure 3. Tumor vaccine strategies based on anti-angiogenesis. Tumor vaccines induce cellular and humoral immune responses targeting endothelial cells, and inhibit tumor angiogenesis through CTL-mediated killing of TAMs that secrete pro-angiogenic factors. Abbreviations: MMP-9: matrix metalloproteinase-9.
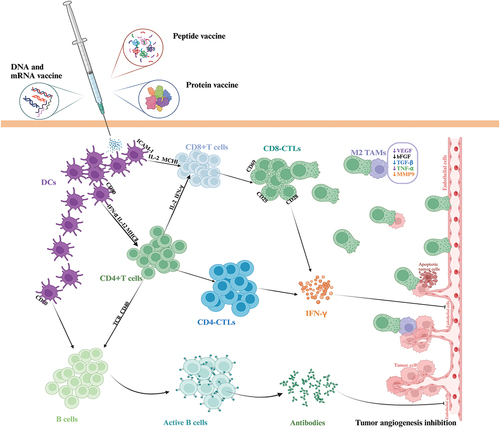
Animal experimental studies on tumor vaccines based on anti-angiogenesis
After it was found that tumor angiogenesis is the driving factor of tumor growth and metastasis, more attention has been paid to the tumor-associated antigen involved in tumor angiogenesis. This antigen protein is overexpressed on the surface of tumor cells and produced by endothelial cells.Citation84 The specific toxic T lymphocyte response and or humoral immune response produced by the tumor vaccine based on this antigen stimulate the immune system not only against tumor cells, but also target endothelial cells, both of which synergistically antagonize tumor growth and invasion. The National Cancer Institute pilot project tests well-reviewed criteria to develop a priority list of cancer vaccine target antigens,Citation85 suggesting the possibility of vascular endothelial growth factor receptor 2 (VEGFR2) and survivin, which are highly expressed in tumor cells, as promising tumor vaccine target antigens. This view is supported by experimental studies on the delivery of these target antigens into the body through various antigen delivery platforms.
In the delivery platform of molecular vaccines, DNA vaccines have the advantage of not being limited by major histocompatibility complex (MHC) types.Citation82 We found that the injection of DNA vaccine encoding survivin into melanoma-bearing mice not only induced specific toxic T lymphocyte responses targeting melanoma cells expressing the antigen, but also inhibited angiogenesis in tumor tissue, thus promoting its protective effect on highly invasive melanoma.Citation86,Citation87 The strong anti-tumor ability shown by this DNA vaccine which encodes a protein encourages scholars to explore the anti-tumor ability of DNA vaccines that encode multiple proteins. In fact, DNA vaccines encoding VEGFR2 and many other different tumor antigens have produced significant tumor treatment, protection and survival benefits in mouse tumor models such as metastatic melanoma,Citation88,Citation89 lung cancerCitation90and cervical cancer.Citation91 Among them, the DNA vaccine encoding VEGFR2 and mouse MHCI heavy chain (H-2Db)Citation88 or IL-12Citation89 enhanced the immune system’s recognition of VEGFR2 antigen and CTL response, and reduced the intratumoral blood vessel density. Whether it was DNA vaccine encoding VEGFR2 and HPV16E6E7 antigenCitation91/MUC1 antigenCitation90 or nanoliposomal VEGFR2 peptide vaccine,Citation92 it could reduce tumor vascular density and kill tumor cells by promoting VEGFR2-specific and E6E7/MUC1 antigen-specific CTL response. Importantly, these vaccines also increased the release of IFN- γ and IL-4 to enhance anti-tumor immunity mediated by Th1 and Th2 immune responses. In the delivery platform of cellular vaccine and carrier vaccine, the main carriers for delivering immune antigens are antigen-presenting cells-dendritic cells (DC), tumor cells and viruses, bacteria and fungi. It was found that DC vaccine loaded with Flk1,Citation93,Citation94 the 4T1 breast cancer cell vaccine infected by adenovirus encoding VEGFR2,Citation95 the oral attenuated Salmonella typhimurium vaccine carrying Flk1Citation96 and the adenovirus vaccine encoding TERT andVEGFR-2Citation97,Citation98 induced the immune system to produce a strong immune response targeting tumor antigen through the efficient antigen delivery ability of DC and the strong immunogenicity of tumor cells and viruses, respectively. Tumor angiogenesis was inhibited by enhancing humoral and cellular immune responses mediated by Flk1 antibody and Flk1-specific CTL respectively, which ultimately inhibited tumor growth, metastasis and prolonged the survival time of tumor-bearing mice.
In addition, the view that tumor vaccine treatment needs to rely on a variety of anti-tumor mechanisms to achieve excellent tumor inhibition was also confirmed in vaccines based on other angiogenic molecules. After immunization with MUC1 peptide vaccine with mouse VEGF (mVEGF165b) as adjuvant,Citation99 VEGF164 modified lung cancer cell vaccine,Citation100 VE-cadherin loaded DC vaccineCitation101 and VEGFR3 attenuated Salmonella typhimurium oral vaccine,Citation102 in addition to activating antigen specific CTL and antibody immune response mediated anti-tumor angiogenesis and tumor cell destruction. It also reduced the proportion of myelogenous suppressor cells and Treg cells in tumor tissue or increased the tumor infiltration of CD68+ monocytes / macrophages to improve the tumor immune microenvironment. It was also found that Th1 and Th2 anti-tumor immune responses were induced. These vaccines have significant tumor treatment and protection effects as well as survival benefits, suggesting that we should further explore the anti-tumor mechanism of tumor vaccines in order to develop tumor vaccines with multi-dimensional mechanisms to mediate anti-tumor effects. lists a summary of preclinical animal experimental studies of tumor vaccines based on anti-angiogenesis.
Table 4. List of animal experimental studies on tumor vaccines based on anti-angiogenesis.
Clinical trials of tumor vaccines based on anti-angiogenesis
In view of the strong anti-tumor growth and metastasis ability of VEGFR2 and survivin tumor vaccines in various preclinical tumor-bearing mice models, clinical studies based on this vaccine were also continuing to evaluate the feasibility and effectiveness of this vaccine in the treatment of tumor patients. In the past decade, there has been a tendency to carry out clinical research on VEGFRs multi-epitope peptide vaccines. The peptide epitopes of these vaccines come from VEGFRs with or without multiple tumor antigens. Although Elpamotide (VEGFR2 peptide vaccine) showed good safety and tolerance in phase I trials for patients with pancreatic cancer,Citation103,Citation104 its combination with gemcitabine did not achieve overall survival benefits in phase II/III trials.Citation105 However, it was gratifying that studies have found that VEGFR2 and tumor antigen multi-epitope peptide cocktail vaccine have achieved initial success in a variety of patients with recurrent, metastatic or refractory solid tumors.Citation106–115 The polypeptide vaccine regimens used in patients were well tolerated and there were virtually no serious systemic adverse events associated with treatment. Further studies have shown that the dipeptide epitope vaccine of VEGFR2 and VEGFR1 enhances the therapeutic efficacy of temozolomide (TMZ) in patients with high-grade gliomas,Citation116 suggesting that multi-epitope peptide vaccine might be a promising tumor therapy. Surprisingly, the II phase trial of multi-epitope peptide cocktail vaccine prepared by VEGFR1/2 and a variety of tumor antigens (such as KIF20A, CDCA1, URLC10, TTK, RNF43, TOMM34, FOXM1, DEPDC1, MELK, HJURP, KOC1, HIG2) in patients with pancreatic cancer,Citation117,Citation118 advanced colon cancer,Citation119,Citation120 refractory cervical cancer and ovarian cancerCitation121 showed that more patients remained stable for a long time. And in these studies, we observed that patients with simultaneous immune response to multiple antigens had longer disease-free survival and total survival time, especially when combined with gemcitabine drugs. These data suggested that multi-epitope peptide vaccine was a promising choice for the treatment of advanced solid tumors and could be considered in combination with other antineoplastic drugs or immune checkpoint inhibitors (ICIs) to find the best combination for clinical cancer patients.Citation122 However, there were also survivin peptide vaccines that produced excellent clinical results in clinical trials conducted by patients with various solid tumors. The survivin peptide vaccine strategy alone was safe in patients with colon cancer, but the immune response was not sufficient to produce visible clinical effects.Citation123 In order to improve the immunogenicity of the vaccine, the strategy of vinvin peptide vaccine combined with different adjuvants was proposed. The addition of adjuvants did enhance the immune response of vinvin peptide vaccine in patients with advanced breast cancer,Citation124 colon cancerCitation125 and pancreatic cancer,Citation126,Citation127 mainly in the overall survival of patients was significantly improved.
In addition to the VEGFR2 molecular vaccine, the attenuated salmonella vaccine encoding VEGFR2 plasmid had also conducted phase I clinical trials in pancreatic cancer patients, but the reactivation or induction of VEGFR reactive Treg might lead to the failure of angiogenesis inhibition in some patients.Citation128,Citation129 The above tumor vaccines based on VEGFR2 molecules could effectively control the progress of patients with various refractory solid tumors, supporting the conclusion of previous animal experiments that they had a strong ability to treat and protect tumors. lists a summary of clinical trials and clinical applications of tumor vaccines based on anti-angiogenesis.
Table 5. List of clinical trials of tumor vaccines based on anti-angiogenesis.
In summary, tumor vaccines based on anti-angiogenesis mediate anti-tumor effects by preventing tumor angiogenesis, weakening tumor immunosuppression (mainly targeting tumor immunosuppressive cells such as Treg and monocytes/macrophages), and enhancing anti-tumor immune response (inducing CTLs response of anti-tumor cells and activating Th1 and Th2 immune responses). These vaccines have shown satisfactory therapeutic effects in both preclinical tumor-bearing animal experiments and clinical trials. The preclinical tumor-bearing animal experiments clarified the specific mechanism of these vaccines in inhibiting tumor growth and metastasis, which provided theoretical support for their entry into clinical trials. The results of clinical trials confirmed the clinical benefits of these vaccines. In the future, the research of these vaccines should be focused on the clinical efficacy of their combination with different adjuvants or with various radiotherapy and chemotherapy strategies in advanced solid tumors.
Future perspectives
Although tumor vaccines with single targeting mechanisms are almost mature, their clinical application has not achieved the expected therapeutic benefits. We know that tumor cells grow in a complex tumor microenvironment and can escape anti-tumor immunity in a variety of ways, which may be an important reason for the limited effects of one-dimensional tumor vaccine therapy. However, we found that some vaccines exhibit multidimensional targeting of tumors by not only enhancing the immune response but also weakening tumor immunosuppression and inhibiting tumor growth. In the B16 melanoma mouse model, the accumulation of tumor-infiltrating lymphocytes (TILs) increased after the injection of an HCA587/MAGE-C2 protein vaccine.Citation75 The vaccine resulted in the enrichment of IFN-γ+CD4+ T cells and the reduction of Treg expression in the tumor microenvironment.Citation130 In a phase I trial to overcome immunosuppression in head and neck square cell carcinoma (HNSCC), inoculation with a dendritic cell p53 peptide vaccine increased patients’ p53-specific T-cell frequencies, and IFN-γ secretion was detected.Citation77 Treg frequencies were consistently decreased relative to pre-vaccination values. A two-year disease-free survival (DFS) rate of 88% was feasible, providing a basis for a confirmatory phase II vaccine test in HNSCC in the future. In addition, peptide vaccines targeting VEGF165b induced humoral immune responses to VEGF, thereby inhibiting tumor angiogenesis. More importantly, the vaccine also reduced Tregs in breast cancer tissues and directly reduced tumor immunosuppression.Citation131 When a VEGFR peptide vaccine was used in neurofibromatosis type 2 (NF2)-derived schwannoma patients,Citation115 tumor volume was reduced by more than 20% in two patients. Additionally, the number of Foxp3-positive cells was reduced and Foxp3 gene expression was lower in tumors.
Recent research advances have revealed significant differences in the efficacy of tumor vaccines at different tissue sites, which have been observed not only in primary tumors but also in metastatic sites. To extend the benefits of tumor vaccines, more comprehensive vaccine strategies are needed. Decades of progress have provided proof of principle that multidimensional tumor vaccines can indeed elicit systemic tumor regression.
These studies suggest that tumor vaccines can utilize a variety of mechanisms to inhibit tumor progression and that this has good therapeutic benefits for highly variable and invasive tumors. Therefore, we believe that follow-up research should focus more on the development of multidimensional tumor vaccines and explore more anti-tumor mechanisms to find potential tumor-dominant antigens. In , based on the three anti-tumor mechanisms of tumor vaccines – boosting the immune system, overcoming immunosuppression, and modulating angiogenesis – we compare the differences between single-dimensional therapy and multidimensional tumor vaccine strategies.
Figure 4. Multidimensional tumor vaccine strategies versus single-dimensional therapy. Tumor growth inhibition is more potent when tumor vaccines mediate antitumor effects through two mechanisms, while maybe the inhibition is even the most potent when three mechanisms used. The three mechanisms of the multidimensional tumor vaccine strategies are mainly outlined here: (a) Tumor vaccines activate the immune system to kill tumor cells; (b)Tumor vaccines inhibit the tumor growth through overcoming the immunosuppression status; (c) Tumor vaccines activate the immune system to target the endothelial cells or TAMs, thus inhibit tumor angiogenesis.
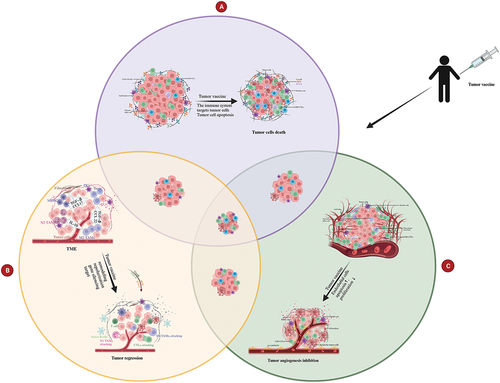
Discussion
In addition to directly stimulating immune responses targeting tumor cells, tumor vaccines have also been developed to activate the immune system. Under pathological tumor conditions, tumor cells secrete a large number of immunosuppressive cytokines to transmit inhibitory signals to reduce the infiltration of CTLs into tumor tissue and prevent their proliferation and activation, as well as enhance the regulatory function of immunosuppressive cells such as Treg and macrophages, which help tumor cells to escape immune cell attack. Although many studies have recognized that the anti-tumor effect of tumor vaccines mainly depends on the immune response of CD8-CTLs, studies have also found that CD4+ T cells play an indispensable role in tumor therapy. High levels of CD4+ T cells are associated with high levels of serum antibodies because CD4+ T cells help activate the humoral immune response produced by B-cell antibodies. After tumor vaccination, CD4+ T cells can secrete a large amount of IFN- γ, which directly interferes with the growth of tumor cells and inhibits tumor angiogenesis.Citation132,Citation133 In addition, some studies have found that vaccines can increase the expression of granzyme B in CD4+ T cells to produce a specific tumor immune response, which is also an important anti-tumor mechanism of CD4+ T cells.Citation130 In addition, given the important role of Tregs in tumor cell immune escape mechanisms, tumor treatment strategies should explore vaccines or drugs that inhibit Tregs in tumors. When tumor vaccines directly inhibit or deplete Tregs in tumor tissue, tumor growth is significantly inhibited. In addition, the anti-tumor effect of vaccines is enhanced when combined with the depletion of Tregs, indicating that Treg-targeted tumor vaccine strategies are worth considering. It is likely that Treg inhibition can effectively assist in combined therapy. We believe that this presents a new multi-directional way to stimulate the immune system: activating CD4+ T cells, improving the immune microenvironment of tumor tissue, and strengthening the effect of the humoral immune response to tumor growth. The advantages of immunization may be maximized through combined immunization strategies.
Judah Folkman and colleagues proposed for the first time that the growth of solid tumors in the avascular period was inhibited at the diameter of 2–3 mm. Therefore, tumor cells secreted tumor angiogenesis factor (TAF) to stimulate the rapid formation of new blood vessels and induce tumor to grow at an exponential rate.Citation78,Citation79 These studies emphasized that tumor angiogenesis was a possible control point in tumor growth, and introduced the concept of anti-angiogenesis. Anti-angiogenesis refers to preventing new vessels sprouts from penetrating into an early tumor implant, which does not apply to vasoconstriction or infarction of vessels already connected to the tumor. Although anti-angiogenic-factor antibodies and immune-targeted angiogenesis inhibitors have shown a certain degree of anti-tumor effect, high drug dosages, long drug-use times, and adverse reactions are often not acceptable to patients. Thus, the high safety, high efficacy, and low toxicity of anti-angiogenic tumor vaccines can provide clinical confidence in their use. Both whole-cell anti-angiogenic tumor vaccines and vascular endothelial growth factor-targeted tumor vaccines can significantly reduce the angiogenesis of tumor tissue, block the blood perfusion of tumor tissue, and inhibit the growth and spread of tumor cells.
Tumor growth and progression is a complex process involving the whole and local immune state. Thus, a single-dimensional tumor vaccine strategy may have limitations to fight tumor cells. Multidimensional tumor vaccine therapies can play each role of different mechanisms, such as boosting the immune system, overcoming tumor immunosuppression, and modulation of tumor angiogenesis. Many studies have pointed to multidimensional tumor vaccine strategies to maximize the anti-tumor effect by boosting the immune system and inhibiting tumor growth. For example, HCA587 protein combined with CpG and ISCOM adjuvants can enhance immune system against tumor attack by inducing CD4+ T cells to express granzyme BCitation130 and IFN- γ to kill tumor cells, and reducing the levels of Tregs in tumor tissue, then inhibit the growth of melanoma cells and improve the survival of mice.Citation75 We have observed that single-dimension tumor vaccines show certain anti-tumor effects in both animal and clinical studies, but in many cases, they cannot prevent tumor progression and metastasis. Relatively speaking, when tumor vaccines have multidimensional anti-tumor mechanisms, they can inhibit tumor growth and slow malignant development thoroughly.
Conclusions
In this review, we have emphasized the role of multidimensional tumor vaccines in tumor immunotherapy. We have illustrated the importance of three anti-tumor mechanisms: boosting the immune system, overcoming tumor immunosuppression, and modulating tumor angiogenesis. Tumor vaccines can increase the infiltration of effector T lymphocytes into tumor tissue, reduce the inhibiting factors in the tumor microenvironment, and blocks the blood supply of tumor cells to inhibit tumor growth. When a tumor vaccine or a combination of tumor vaccines utilizes two or more of these mechanisms, it may more effectively eliminate tumor cells.
Author contributions
Conceptualization, J.C.; writing – original draft preparation, Y.T., H.C. and X.G.; writing – review and editing, J.C., Q.F., Y.T., H.C. and X.G. All authors have read and agreed to the published version of the manuscript.
Disclosure statement
No potential conflict of interest was reported by the author(s).
Additional information
Funding
References
- Miao L, Zhang Y, Huang L. mRNA vaccine for cancer immunotherapy. Mol Cancer. 2021;20(1):41. doi:10.1186/s12943-021-01335-5.
- Zamani P, Navashenaq JG, Teymouri M, Karimi M, Mashreghi M, Jaafari MR. Combination therapy with liposomal doxorubicin and liposomal vaccine containing E75, an HER-2/neu-derived peptide, reduces myeloid-derived suppressor cells and improved tumor therapy. Life Sci. 2020;252:117646. doi:10.1016/j.lfs.2020.117646.
- Wang X, Li X, Ito A, Sogo Y, Ohno T Synergistic anti-tumor efficacy of a hollow mesoporous silica-based cancer vaccine and an immune checkpoint inhibitor at the local site.
- Joffre OP, Segura E, Savina A, Amigorena S. Cross-presentation by dendritic cells. Nat Rev Immunol. 2012;12(8):557–24. doi:10.1038/nri3254.
- Blander JM. Regulation of the cell biology of antigen cross-presentation. Annu Rev Immunol. 2018;36(1):717–53. doi:10.1146/annurev-immunol-041015-055523.
- Alloatti A, Kotsias F, Magalhaes JG, Amigorena S. Dendritic cell maturation and cross-presentation: timing matters! Immunol Rev. 2016;272(1):97–108. doi:10.1111/imr.12432.
- Santos PM, Butterfield LH. Dendritic cell–based cancer vaccines. J Immunol. 2018;200(2):443–9. doi:10.4049/jimmunol.1701024.
- Kantoff PW, Higano CS, Shore ND, Berger ER, Small EJ, Penson DF, Redfern CH, Ferrari AC, Dreicer R, Sims RB, et al. Sipuleucel-T immunotherapy for castration-resistant prostate cancer. N Engl J Med. 2010;363(5):411–22. doi:10.1056/NEJMoa1001294.
- Goldman B, DeFrancesco L. The cancer vaccine roller coaster. Nat Biotechnol. 2009;27(2):129–39. doi:10.1038/nbt0209-129.
- Fong L, Carroll P, Weinberg V, Chan S, Lewis J, Corman J, Amling CL, Stephenson RA, Simko J, Sheikh NA, et al. Activated lymphocyte recruitment into the tumor microenvironment following preoperative sipuleucel-T for localized prostate cancer. J Natl Cancer Inst. 2014;106(11). doi:10.1093/jnci/dju268.
- Sutherland SIM, Ju X, Horvath LG, Clark GJ. Moving on from sipuleucel-T: new dendritic cell vaccine strategies for prostate cancer. Front Immunol. 2021;12:641307. doi:10.3389/fimmu.2021.641307.
- Holl EK, McNamara MA, Healy P, Anand M, Concepcion RS, Breland CD, Dumbudze I, Tutrone R, Shore N, Armstrong AJ, et al. Prolonged PSA stabilization and overall survival following sipuleucel-T monotherapy in metastatic castration-resistant prostate cancer patients. Prostate Cancer Prostatic Dis. 2019;22(4):588–92. doi:10.1038/s41391-019-0144-3.
- Yang H, Zhou H, Huang Z, Tao K, Huang N, Peng Z, Feng W, et al. Induction of CML-specific immune response through cross-presentation triggered by CTP-mediated BCR-ABL-derived peptides. Cancer Lett. 2020;482:44–55. doi:10.1016/j.canlet.2020.04.010.
- Marciscano AE, Anandasabapathy N. The role of dendritic cells in cancer and anti-tumor immunity. Semin Immunol. 2021;52:101481. doi:10.1016/j.smim.2021.101481.
- Salmon H, Idoyaga J, Rahman A, Leboeuf M, Remark R, Jordan S, Casanova-Acebes M, Khudoynazarova M, Agudo J, Tung N, et al. Expansion and activation of CD103(+) dendritic cell progenitors at the tumor site enhances tumor responses to therapeutic PD-L1 and BRAF inhibition. Immunity. 2016;44(4):924–38. doi:10.1016/j.immuni.2016.03.012.
- Roberts EW, Broz ML, Binnewies M, Headley MB, Nelson AE, Wolf DM, Kaisho T, Bogunovic D, Bhardwaj N, Krummel MF, et al. Critical role for CD103(+)/CD141(+) dendritic cells bearing CCR7 for tumor antigen trafficking and priming of T cell immunity in melanoma. Cancer Cell. 2016;30(2):324–36. doi:10.1016/j.ccell.2016.06.003.
- Lee W, Suresh M. Vaccine adjuvants to engage the cross-presentation pathway. Front Immunol. 2022;13:940047. doi:10.3389/fimmu.2022.940047.
- Audsley KM, McDonnell AM, Waithman J. Cross-presenting XCR1(+) dendritic cells as targets for cancer immunotherapy. Cells. 2020;9(3):565. doi:10.3390/cells9030565.
- Shae D, Baljon JJ, Wehbe M, Christov PP, Becker KW, Kumar A, Suryadevara N, Carson CS, Palmer CR, Knight FC, et al. Co-delivery of peptide neoantigens and stimulator of interferon genes agonists enhances response to cancer vaccines. ACS Nano. 2020;14(8):9904–16. doi:10.1021/acsnano.0c02765.
- Liu W, Tang H, Li L, Wang X, Yu Z, Li J. Peptide-based therapeutic cancer vaccine: Current trends in clinical application. Cell Prolif. 2021;54(5):e13025. doi:10.1111/cpr.13025.
- Kissick HT, Sanda MG, Dunn LK, Arredouani MS, van Hall T. Immunization with a peptide containing MHC class I and II epitopes derived from the tumor antigen SIM2 induces an effective CD4 and CD8 T-cell response. PloS One. 2014;9(4):e93231. doi:10.1371/journal.pone.0093231.
- Liu Y, Song Z, Zhou B, Fu X, Wu M, Butterfield LH. An in vitro study on the inhibition of hepatocellular carcinoma growth by AFP-specific CD8 + T/CD4 + T activated by lentivirally engineered and AFP pulsed DC. J Tongji Univ. 2011;32:18–23.
- Oberli MA, Reichmuth AM, Dorkin JR, Mitchell MJ, Fenton OS, Jaklenec A, Anderson DG, Langer R, Blankschtein D. Lipid nanoparticle assisted mRNA delivery for potent cancer immunotherapy. Nano Lett. 2017;17(3):1326–35. doi:10.1021/acs.nanolett.6b03329.
- Rojas LA, Sethna Z, Soares KC, Olcese C, Pang N, Patterson E, Lihm J, Ceglia N, Guasp P, Chu A, et al. Personalized RNA neoantigen vaccines stimulate T cells in pancreatic cancer. Nature. 2023;618(7963):144–50. doi:10.1038/s41586-023-06063-y.
- Kyriakopoulos CE, Eickhoff JC, Ferrari AC, Schweizer MT, Wargowski E, Olson BM, McNeel DG. Multicenter phase I trial of a DNA vaccine encoding the androgen receptor ligand-binding domain (pTVG-AR, MVI-118) in patients with metastatic prostate cancer. Clin Cancer Res. 2020;26(19):5162–71. doi:10.1158/1078-0432.CCR-20-0945.
- Graván P, Aguilera-Garrido A, Marchal JA, Navarro-Marchal SA, Galisteo-González F. Lipid-core nanoparticles: classification, preparation methods, routes of administration and recent advances in cancer treatment. Adv Colloid Interface Sci. 2023;314:102871. doi:10.1016/j.cis.2023.102871.
- Qiu Z, Huang H, Grenier JM, Perez OA, Smilowitz HM, Adler B, Khanna KM. Cytomegalovirus-based vaccine expressing a modified tumor antigen induces potent tumor-specific CD8(+) T-cell response and protects mice from melanoma. Cancer Immunol Res. 2015;3(5):536–46. doi:10.1158/2326-6066.CIR-14-0044.
- Tršan T, Vuković K, Filipović P, Brizić AL, Lemmermann NAW, Schober K, Busch DH, Britt WJ, Messerle M, Krmpotić A, et al. Cytomegalovirus vector expressing RAE-1γ induces enhanced anti-tumor capacity of murine CD8 + T cells. Eur J Immunol. 2017;47(8):1354–67. doi:10.1002/eji.201746964.
- D’Alise AM, Brasu N, De Intinis C, Leoni G, Russo V, Langone F, Baev D, Micarelli E, Petiti L, Picelli S, et al. Adenoviral-based vaccine promotes neoantigen-specific CD8 + T cell stemness and tumor rejection. Sci Transl Med. 2022;14(657):eabo7604. doi:10.1126/scitranslmed.abo7604.
- Svensson-Arvelund J, Cuadrado-Castano S, Pantsulaia G, Kim K, Aleynick M, Hammerich L, Upadhyay R, Yellin M, Marsh H, Oreper D, et al. Expanding cross-presenting dendritic cells enhances oncolytic virotherapy and is critical for long-term anti-tumor immunity. Nat Commun. 2022;13(1):7149. doi:10.1038/s41467-022-34791-8.
- Baharom F, Ramirez-Valdez RA, Tobin KKS, Yamane H, Dutertre CA, Khalilnezhad A, Reynoso GV, Coble VL, Lynn GM, Mulè MP, et al. Intravenous nanoparticle vaccination generates stem-like TCF1(+) neoantigen-specific CD8(+) T cells. Nat Immunol. 2021;22(1):41–52. doi:10.1038/s41590-020-00810-3.
- Park KS, Nam J, Son S, Moon JJ. Personalized combination nano-immunotherapy for robust induction and tumor infiltration of CD8(+) T cells. Biomaterials. 2021;274:120844. doi:10.1016/j.biomaterials.2021.120844.
- Chen Z, Zhang S, Han N, Jiang J, Xu Y, Ma D, Lu L, Guo X, Qiu M, Huang Q, et al. A neoantigen-based peptide vaccine for patients with advanced pancreatic cancer refractory to standard treatment. Front Immunol. 2021;12:691605. doi:10.3389/fimmu.2021.691605.
- Awad MM, Govindan R, Balogh KN, Spigel DR, Garon EB, Bushway ME, Poran A, Sheen JH, Kohler V, Esaulova E, et al. Personalized neoantigen vaccine NEO-PV-01 with chemotherapy and anti-PD-1 as first-line treatment for non-squamous non-small cell lung cancer. Cancer Cell. 2022;40(9):1010–26.e11. doi:10.1016/j.ccell.2022.08.003.
- Adotevi O, Dosset M, Galaine J, Beziaud L, Godet Y, Borg C. Targeting antitumor CD4 helper T cells with universal tumor-reactive helper peptides derived from telomerase for cancer vaccine. Hum Vaccin Immunother. 2013;9(5):1073–7. doi:10.4161/hv.23587.
- Kumai T, Lee S, Cho HI, Sultan H, Kobayashi H, Harabuchi Y, Celis E. Optimization of peptide vaccines to induce robust antitumor CD4 T-cell responses. Cancer Immunol Res. 2017;5(1):72–83. doi:10.1158/2326-6066.CIR-16-0194.
- Chen J, Ye Z, Huang C, Qiu M, Song D, Li Y, Xu Q. Lipid nanoparticle-mediated lymph node–targeting delivery of mRNA cancer vaccine elicits robust CD8+ T cell response. Proc Natl Acad Sci USA. 2022;119(34):e2207841119. doi:10.1073/pnas.2207841119.
- Amaya L, Grigoryan L, Li Z, Lee A, Wender PA, Pulendran B, Chang HY. Circular RNA vaccine induces potent T cell responses. Proc Natl Acad Sci USA. 2023;120(20):e2302191120. doi:10.1073/pnas.2302191120.
- Daiko H, Marafioti T, Fujiwara T, Shirakawa Y, Nakatsura T, Kato K, Puccio I, Hikichi T, Yoshimura S, Nakagawa T, et al. Exploratory open-label clinical study to determine the S-588410 cancer peptide vaccine-induced tumor-infiltrating lymphocytes and changes in the tumor microenvironment in esophageal cancer patients. Cancer Immunol Immunother. 2020;69(11):2247–57. doi:10.1007/s00262-020-02619-3.
- Gridelli C, Ciuleanu T, Domine M, Szczesna A, Bover I, Cobo M, Kentepozidis N, Zarogoulidis K, Kalofonos C, Kazarnowisz A, et al. Clinical activity of a htert (vx-001) cancer vaccine as post-chemotherapy maintenance immunotherapy in patients with stage IV non-small cell lung cancer: final results of a randomised phase 2 clinical trial. Br J Cancer. 2020;122(10):1461–6. doi:10.1038/s41416-020-0785-y.
- Hirokazu O, Taylor JW, Takahide N, David G, Watchmaker PB, Kaori O, Saijo A, Tedesco MR, Shai A, Wong CM, et al. Randomized trial of neoadjuvant vaccination with tumor-cell lysate induces T-cell response in low-grade gliomas. J Clin Invest. 2022;132(3). doi:10.1172/jci151239.
- Frank MJ, Khodadoust MS, Czerwinski DK, Haabeth OA, Chu MP, Miklos DB, Advani RH, Alizadeh AA, Gupta NK, Maeda LS, et al. Autologous tumor cell vaccine induces antitumor T cell immune responses in patients with mantle cell lymphoma: a phase I/II trial. J Exp Med. 2020;217(9). doi:10.1084/jem.20191712.
- Crosby EJ, Gwin W, Blackwell K, Marcom PK, Chang S, Maecker HT, Broadwater G, Hyslop T, Kim S, Rogatko A, et al. Vaccine-induced memory CD8(+) T cells provide clinical benefit in HER2 expressing breast cancer: a mouse to human translational study. Clin Cancer Res. 2019;25(9):2725–36. doi:10.1158/1078-0432.CCR-18-3102.
- Mousset CM, Hobo W, Woestenenk R, Preijers F, Dolstra H, van der Waart AB. Comprehensive phenotyping of T cells using flow cytometry. Cytometry A. 2019;95(6):647–54. doi:10.1002/cyto.a.23724.
- Saravia J, Chapman NM, Chi H. Helper T cell differentiation. Cell Mol Immunol. 2019;16(7):634–43. doi:10.1038/s41423-019-0220-6.
- Cenerenti M, Saillard M, Romero P, Jandus C. The era of cytotoxic CD4 T cells. Front Immunol. 2022;13:867189. doi:10.3389/fimmu.2022.867189.
- Porakishvili N, Roschupkina T, Kalber T, Jewell AP, Patterson K, Yong K, Lydyard PM. Expansion of CD4+ T cells with a cytotoxic phenotype in patients with B-chronic lymphocytic leukaemia (B-CLL). Clin Exp Immunol. 2001;126(1):29–36. doi:10.1046/j.1365-2249.2001.01639.x.
- Fu J, Wang F. Research progress of CD4+ killing T cells in viral infection and tumor diseases. Chin J Cell Mol Immunol. 2012;28:102–4.
- Quezada SA, Simpson TR, Peggs KS, Merghoub T, Vider J, Fan X, Blasberg R, Yagita H, Muranski P, Antony PA, et al. Tumor-reactive CD4(+) T cells develop cytotoxic activity and eradicate large established melanoma after transfer into lymphopenic hosts. J Exp Med. 2010;207(3):637–50. doi:10.1084/jem.20091918.
- Kitano S, Tsuji T, Liu C, Hirschhorn-Cymerman D, Kyi C, Mu Z, Allison JP, Gnjatic S, Yuan JD, Wolchok JD, et al. Enhancement of tumor-reactive cytotoxic CD4+ T cell responses after ipilimumab treatment in four advanced melanoma patients. Cancer Immunol Res. 2013;1(4):235–44. doi:10.1158/2326-6066.CIR-13-0068.
- Zhou L, Wang H, Fu J, Chen Y, Li Y, Chen L, Sa LV, Wang FS. Study on the correlation between CD4+ cytotoxic T and course of primary hepatocellular carcinoma. Med J Chin People’s Liberation Army. 2013;38:137–40.
- Tang XX, Shimada H, Ikegaki N. Clinical relevance of CD4 cytotoxic T cells in high-risk neuroblastoma. Front Immunol. 2021;12:650427. doi:10.3389/fimmu.2021.650427.
- Azizi E, Carr AJ, Plitas G, Cornish AE, Konopacki C, Prabhakaran S, Nainys J, Wu K, Kiseliovas V, Setty M, et al. Single-cell map of diverse immune phenotypes in the breast tumor microenvironment. Cell. 2018;174(5):1293–308 e36. doi:10.1016/j.cell.2018.05.060.
- Zhou Y, Yang D, Yang Q, Lv X, Huang W, Zhou Z, Wang Y, Zhang Z, Yuan T, Ding X, et al. Single-cell RNA landscape of intratumoral heterogeneity and immunosuppressive microenvironment in advanced osteosarcoma. Nat Commun. 2020;11(1):6322. doi:10.1038/s41467-020-20059-6.
- Hunder NN, Wallen H, Cao J, Hendricks DW, Reilly JZ, Rodmyre R, Jungbluth A, Gnjatic S, Thompson JA, Yee C, et al. Treatment of metastatic melanoma with autologous CD4+ T cells against NY-ESO-1. N Engl J Med. 2008;358(25):2698–703. doi:10.1056/NEJMoa0800251.
- Anastasopoulou EA, Voutsas IF, Papamichail M, Baxevanis CN, Perez SA. MHC class II tetramer analyses in AE37-vaccinated prostate cancer patients reveal vaccine-specific polyfunctional and long-lasting CD4 + T-cells. Oncoimmunology. 2016;5(7):e1178439. doi:10.1080/2162402X.2016.1178439.
- Vergati M, Schlom J, Tsang KY. The consequence of immune suppressive cells in the use of therapeutic cancer vaccines and their importance in immune monitoring. J Biomed Biotechnol. 2011;2011:182413. doi:10.1155/2011/182413.
- Sakaguchi S. Regulatory T cells: key controllers of immunologic self-tolerance. Cell. 2000;101(5):455–8. doi:10.1016/S0092-8674(00)80856-9.
- Sakaguchi S, Miyara M, Costantino CM, Hafler DA. FOXP3+ regulatory T cells in the human immune system. Nat Rev Immunol. 2010;10(7):490–500. doi:10.1038/nri2785.
- Ahmad M, Rees RC, Ali SA. Escape from immunotherapy: possible mechanisms that influence tumor regression/progression. Cancer Immunol Immunother. 2004;53(10):844–54. doi:10.1007/s00262-004-0540-x.
- Wiedermann U, Garner-Spitzer E, Chao Y, Maglakelidze M, Bulat I, Dechaphunkul A, Arpornwirat W, Charoentum C, Yen C-J, Yau TC, et al. Clinical and immunologic responses to a B-cell epitope vaccine in patients with HER2/neu-overexpressing advanced gastric cancer—results from phase Ib trial IMU.ACS.001. Clin Cancer Res. 2021;27(13):3649–60. doi:10.1158/1078-0432.CCR-20-3742.
- Wada M, Tsuchikawa T, Kyogoku N, Abiko T, Miyauchi K, Takeuchi S, Kuwatani T, Shichinohe T, Miyahara Y, Kageyama S, et al. Clinical implications of CD4(+)CD25(+)Foxp3(+)Regulatory T cell frequencies after CHP-MAGE-A4 cancer vaccination. Anticancer Res. 2018;38:1435–44.
- Beyer M, Schultze JL. Regulatory T cells in cancer. Blood. 2006;108(3):804–11. doi:10.1182/blood-2006-02-002774.
- Mousavi-Niri N, Naseroleslami M, Hadjati J. Anti-regulatory T cell vaccines in immunotherapy: focusing on FoxP3 as target. Hum Vaccin Immunother. 2019;15(3):620–4. doi:10.1080/21645515.2018.1545625.
- Miguel A, Sendra L, Noé V, Ciudad CJ, Dasí F, Hervas D, Herrero MJ, Aliño S. Silencing of Foxp3 enhances the antitumor efficacy of GM-CSF genetically modified tumor cell vaccine against B16 melanoma. Onco Targets Ther. 2017;10:503–14. doi:10.2147/OTT.S104393.
- Namdar A, Mirzaei R, Memarnejadian A, Boghosian R, Samadi M, Mirzaei HR, Farajifard H, Zavar M, Azadmanesh K, Elahi S, et al. Prophylactic DNA vaccine targeting Foxp3(+) regulatory T cells depletes myeloid-derived suppressor cells and improves anti-melanoma immune responses in a murine model. Cancer Immunol Immunother. 2018;67(3):367–79. doi:10.1007/s00262-017-2088-6.
- Mousavi Niri N, Memarnejadian A, Hadjati J, Aghasadeghi MR, Shokri M, Pilehvar-Soltanahmadi Y, Akbarzadeh A, Zarghami N. Construction and production of Foxp3-Fc (IgG) DNA vaccine/fusion protein. Avicenna J Med Biotechnol. 2016;8:57–64.
- Mousavi Niri N, Memarnejadian A, Pilehvar-Soltanahmadi Y, Agha Sadeghi M, Mahdavi M, Kheshtchin N, Arab S, Namdar A, Jadidi F, Zarghami N, et al. Improved anti-Treg vaccination targeting Foxp3 efficiently decreases regulatory T cells in mice. J Immunother (1991). 2016;39(7):269–75. doi:10.1097/CJI.0000000000000133.
- Mattarollo SR, Steegh K, Li M, Duret H, Foong Ngiow S, Smyth MJ. Transient Foxp3+ regulatory T-cell depletion enhances therapeutic anticancer vaccination targeting the immune-stimulatory properties of NKT cells. Immunol Cell Biol. 2013;91(1):105–14. doi:10.1038/icb.2012.58.
- Yan J, Tingey C, Lyde R, Gorham TC, Choo DK, Muthumani A, Myles D, Weiner LP, Kraynyak KA, Reuschel EL, et al. Novel and enhanced anti-melanoma DNA vaccine targeting the tyrosinase protein inhibits myeloid-derived suppressor cells and tumor growth in a syngeneic prophylactic and therapeutic murine model. Cancer Gene Ther. 2014;21(12):507–17. doi:10.1038/cgt.2014.56.
- Wang Y, Zhao Q, Zhao B, Zheng Y, Zhuang Q, Liao N, Wang P, Cai Z, Zhang D, Zeng Y, et al. Remodeling tumor-associated neutrophils to enhance dendritic cell-based HCC neoantigen nano-vaccine efficiency. Adv Sci. 2022;9(11):e2105631. doi:10.1002/advs.202105631.
- Gottschalk S, Yu F, Ji M, Kakarla S, Song XT, Haass NK. A vaccine that co-targets tumor cells and cancer associated fibroblasts results in enhanced antitumor activity by inducing antigen spreading. PloS One. 2013;8(12):e82658. doi:10.1371/journal.pone.0082658.
- Chen M, Xiang R, Wen Y, Xu G, Wang C, Luo S, Yin T, Wei X, Shao B, Liu N, et al. A whole-cell tumor vaccine modified to express fibroblast activation protein induces antitumor immunity against both tumor cells and cancer-associated fibroblasts. Sci Rep. 2015;5(1):14421. doi:10.1038/srep14421.
- Qian L, Tang Z, Yin S, Mo F, Yang X, Hou X, Liu A, Lu X. Fusion of dendritic cells and cancer-associated fibroblasts for activation of anti-tumor cytotoxic T lymphocytes. J Biomed Nanotechnol. 2018;14(10):1826–35. doi:10.1166/jbn.2018.2616.
- Chen J, Zhang L, Wen W, Hao J, Zeng P, Qian X, Zhang Y, Yin Y. Induction of HCA587-specific antitumor immunity with HCA587 protein formulated with CpG and ISCOM in mice. PloS One. 2012;7(10):e47219. doi:10.1371/journal.pone.0047219.
- Yang Y, Che Y, Zhao Y, Wang X. Prevention and treatment of cervical cancer by a single administration of human papillomavirus peptide vaccine with CpG oligodeoxynucleotides as an adjuvant in vivo. Int Immunopharmacol. 2019;69:279–88. doi:10.1016/j.intimp.2019.01.024.
- Schuler JP, Malgorzata H, Carmen V, Albert D, Sumita T, Yu L, Argiris A, Gooding W, Butterfield LH, Whiteside TL, et al. Phase I dendritic cell p53 peptide vaccine for head and neck cancer. Clin Cancer Res. 2014;20(9):2433–44. doi:10.1158/1078-0432.CCR-13-2617.
- Holleb AI, Folkman J. Tumor angiogenesis. CA Cancer J Clin. 1972;22:226–9.
- Folkman J. Tumor angiogenesis: a possible control point in tumor growth. Ann Intern Med. 1975;82(1):96–100. doi:10.7326/0003-4819-82-1-96.
- 侯建梅, 田聆, 魏于全. 抗肿瘤血管生成免疫治疗的研究进展. 中国科学(C辑:生命科学). 2004;34(3):203–9.
- Neek M, Kim TI, Wang SW. Protein-based nanoparticles in cancer vaccine development. Nanomedicine. 2019;15(1):164–74. doi:10.1016/j.nano.2018.09.004.
- Shahnazari M, Samadi P, Pourjafar M, Jalali A. Therapeutic vaccines for colorectal cancer: the progress and future prospect. Int Immunopharmacol. 2020;88:106944. doi:10.1016/j.intimp.2020.106944.
- Kim CG, Sang YB, Lee JH, Chon HJ. Combining cancer vaccines with immunotherapy: establishing a new immunological approach. Int J Mol Sci. 2021;22(15):22. doi:10.3390/ijms22158035.
- Wagner S, Mullins CS, Linnebacher M. Colorectal cancer vaccines: tumor-associated antigensvsneoantigens. World J Gastroentero. 2018;24(48):5418–32. doi:10.3748/wjg.v24.i48.5418.
- Cheever MA, Allison JP, Ferris AS, Finn OJ, Hastings BM, Hecht TT, Mellman I, Prindiville SA, Viner JL, Weiner LM, et al. The prioritization of cancer antigens: a National cancer institute pilot project for the acceleration of translational research. Clin Cancer Res. 2009;15(17):5323–37. doi:10.1158/1078-0432.CCR-09-0737.
- Lladser A, Párraga M, Quevedo L, Carmen Molina M, Silva S, Ferreira A, Billetta R, Quest AF. Naked DNA immunization as an approach to target the generic tumor antigen survivin induces humoral and cellular immune responses in mice. Immunobiology. 2006;211(1–2):11–27. doi:10.1016/j.imbio.2005.08.002.
- Lladser A, Ljungberg K, Tufvesson H, Tazzari M, Roos A-K, Quest AFG, Kiessling R. Intradermal DNA electroporation induces survivin-specific CTLs, suppresses angiogenesis and confers protection against mouse melanoma. Cancer Immunol Immunother. 2009;59(1):81–92. doi:10.1007/s00262-009-0725-4.
- Chen R, Wang S, Yao Y, Zhou YUN, Zhang C, Fang JIE, Zhang D, Zhang L, Pan J. Anti-metastatic effects of DNA vaccine encoding single-chain trimer composed of MHC I and vascular endothelial growth factor receptor 2 peptide. Oncol Rep. 2015;33(5):2269–76. doi:10.3892/or.2015.3820.
- Yin X, Wang W, Zhu X, Wang Y, Wu S, Wang Z, Wang L, Du Z, Gao J, Yu J, et al. Synergistic antitumor efficacy of combined DNA vaccines targeting tumor cells and angiogenesis. Biochem Bioph Res Co. 2015;465(2):239–44. doi:10.1016/j.bbrc.2015.08.003.
- Ruan J, Duan Y, Li F, Wang Z. Enhanced synergistic anti-Lewis lung carcinoma effect of a DNA vaccine harboring a MUC1-VEGFR2 fusion gene used with GM-CSF as an adjuvant. Clin Exp Pharmacol Physiol. 2017;44(1):71–8. doi:10.1111/1440-1681.12654.
- Gao J, Fan L, Ma W, Xiao H. Synergistic antitumor effect of a human papillomavirus DNA vaccine harboring E6E7 fusion gene and vascular endothelial growth factor receptor 2 gene. Microbiol Immunol. 2016;60(9):626–33. doi:10.1111/1348-0421.12408.
- Zahedipour F, Zamani P, Mashreghi M, Astaneh M, Sankian M, Amiri A, Jamialahmadi K, Jaafari MR. Nanoliposomal VEGF-R2 peptide vaccine acts as an effective therapeutic vaccine in a murine B16F10 model of melanoma. Cancer Nanotechnol. 2023;14(1):14. doi:10.1186/s12645-023-00213-7.
- Li Y, Wang MN, Li H, King KD, Bassi R, Sun H, Santiago A, Hooper AT, Bohlen P, Hicklin DJ, et al. Active immunization against the vascular endothelial growth factor receptor flk1 inhibits tumor angiogenesis and metastasis. J Exp Med. 2002;195(12):1575–84. doi:10.1084/jem.20020072.
- Pan J, Heiser A, Marget M, Steinmann J, Kabelitz D. Enhanced antimetastatic effect of fetal liver kinase 1 extracellular domain and interferon-gamma fusion gene-modified dendritic cell vaccination. Gene Ther. 2005;12(9):742–50. doi:10.1038/sj.gt.3302470.
- Yan HX, Cheng P, Wei HY, Shen GB, Fu LX, Ni J, Wu Y, Wei Y-Q. Active immunotherapy for mouse breast cancer with irradiated whole-cell vaccine expressing VEGFR2. Oncol Rep. 2013;29(4):1510–16. doi:10.3892/or.2013.2282.
- Zuo SG, Chen Y, Wu ZP, Liu X, Liu C, Zhou YC, Wu CL, Jin CG, Gu YL, Li J, et al. Orally administered DNA vaccine delivery by attenuated Salmonella typhimurium targeting fetal liver kinase 1 inhibits murine Lewis lung carcinoma growth and metastasis. Biol Pharm Bull. 2010;33(2):174–82. doi:10.1248/bpb.33.174.
- Zhang J, Wang Y, Wu Y, Ding ZY, Luo XM, Zhong WN, Liu J, Xia X-Y, Deng G-H, Deng Y-T, et al. Mannan-modified adenovirus encoding VEGFR-2 as a vaccine to induce anti-tumor immunity. J Cancer Res Clin Oncol. 2014;140(5):701–12. doi:10.1007/s00432-014-1606-6.
- Wang Y, Zhang J, Wu Y, Ding ZY, Luo XM, Liu J, Zhong W-N, Deng G-H, Xia X-Y, Deng Y-T, et al. Mannan-modified adenovirus targeting TERT and VEGFR-2: a universal tumour vaccine. Sci Rep. 2015;5(1):11275. doi:10.1038/srep11275.
- Zhang H, Jia E, Xia W, Lv T, Lu C, Xu Z, Zhu W. Utilizing VEGF165b mutant as an effective immunization adjunct to augment antitumor immune response. Vaccine. 2019;37(15):2090–8. doi:10.1016/j.vaccine.2019.02.055.
- Kan B, Yang L, Wen YJ, Yang JR, Niu T, Li J, Deng H-X, Wei W, Chen L-G, Zhang Q, et al. Irradiated VEGF164-modified tumor cell vaccine protected mice from the parental tumor challenge. Anticancer Drugs. 2017;28(2):197–205. doi:10.1097/CAD.0000000000000447.
- Zhou J, Xi Y, Mu X, Zhao R, Chen H, Zhang L, Wu Y, Li Q. Antitumor immunity induced by VE-cadherin modified DC vaccine. Oncotarget. 2017;8(40):67369–79. doi:10.18632/oncotarget.18654.
- Chen Y, Liu X, Jin CG, Zhou YC, Navab R, Jakobsen KR, Chen XQ, Li J, Li TT, Luo L, et al. An orally administered DNA vaccine targeting vascular endothelial growth factor receptor-3 inhibits lung carcinoma growth. Tumor Biol. 2015;37(2):2395–404. doi:10.1007/s13277-015-4061-3.
- Miyazawa M, Ohsawa R, Tsunoda T, Hirono S, Kawai M, Tani M, Nakamura Y, Yamaue H. Phase I clinical trial using peptide vaccine for human vascular endothelial growth factor receptor 2 in combination with gemcitabine for patients with advanced pancreatic cancer. Cancer Sci. 2010;101(2):433–9. doi:10.1111/j.1349-7006.2009.01416.x.
- Okamoto I, Arao T, Miyazaki M, Satoh T, Okamoto K, Tsunoda T, Nishio K, Nakagawa K. Clinical phase I study of elpamotide, a peptide vaccine for vascular endothelial growth factor receptor 2, in patients with advanced solid tumors. Cancer Sci. 2012;103(12):2135–8. doi:10.1111/cas.12014.
- Yamaue H, Tsunoda T, Tani M, Miyazawa M, Yamao K, Mizuno N, Okusaka T, Ueno H, Boku N, Fukutomi A, et al. Randomized phase II/III clinical trial of elpamotide for patients with advanced pancreatic cancer: PEGASUS-PC study. Cancer Sci. 2015;106(7):883–90. doi:10.1111/cas.12674.
- Masuzawa T, Fujiwara Y, Okada K, Nakamura A, Takiguchi S, Nakajima K, Miyata H, Yamasaki M, Kurokawa Y, Osawa R, et al. Phase I/II study of S-1 plus cisplatin combined with peptide vaccines for human vascular endothelial growth factor receptor 1 and 2 in patients with advanced gastric cancer. Int J Oncol. 2012;41(4):1297–304. doi:10.3892/ijo.2012.1573.
- Matsushita N, Aruga A, Inoue Y, Kotera Y, Takeda K, Yamamoto M. Phase I clinical trial of a peptide vaccine combined with tegafur-uracil plus leucovorin for treatment of advanced or recurrent colorectal cancer. Oncol Rep. 2013;29(3):951–9. doi:10.3892/or.2013.2231.
- Okuyama R, Aruga A, Hatori T, Takeda K, Yamamoto M. Immunological responses to a multi-peptide vaccine targeting cancer-testis antigens and VEGFRs in advanced pancreatic cancer patients. Oncoimmunology. 2013;2(11):e27010. doi:10.4161/onci.27010.
- Suzuki H, Fukuhara M, Yamaura T, Mutoh S, Okabe N, Yaginuma H, Hasegawa T, Yonechi A, Osugi J, Hoshino M, et al. Multiple therapeutic peptide vaccines consisting of combined novel cancer testis antigens and anti-angiogenic peptides for patients with non-small cell lung cancer. J Transl Med. 2013;11(1):97. doi:10.1186/1479-5876-11-97.
- Hazama S, Nakamura Y, Takenouchi H, Suzuki N, Tsunedomi R, Inoue Y, Tokuhisa Y, Iizuka N, Yoshino S, Takeda K, et al. A phase I study of combination vaccine treatment of five therapeutic epitope-peptides for metastatic colorectal cancer; safety, immunological response, and clinical outcome. J Transl Med. 2014;12(1):63. doi:10.1186/1479-5876-12-63.
- Okuno K, Sugiura F, Inoue K, Sukegawa Y. Clinical trial of a 7-peptide cocktail vaccine with oral chemotherapy for patients with metastatic colorectal cancer. Anticancer Res. 2014;34:3045–52.
- Hasegawa K, Ikeda Y, Kunugi Y, Kurosaki A, Imai Y, Kohyama S, Nagao S, Kozawa E, Yoshida K, Tsunoda T, et al. Phase I study of multiple epitope peptide vaccination in patients with recurrent or persistent cervical cancer. J Immunother (1991). 2018;41(4):201–7. doi:10.1097/CJI.0000000000000214.
- Shibao S, Ueda R, Saito K, Kikuchi R, Nagashima H, Kojima A, Kagami H, Pareira ES, Sasaki H, Noji S, et al. A pilot study of peptide vaccines for VEGF receptor 1 and 2 in patients with recurrent/progressive high grade glioma. Oncotarget. 2018;9(30):21569–79. doi:10.18632/oncotarget.25131.
- Kikuchi R, Ueda R, Saito K, Shibao S, Nagashima H, Tamura R, Morimoto Y, Sasaki H, Noji S, Kawakami Y, et al. A Pilot study of vaccine therapy with multiple glioma oncoantigen/glioma angiogenesis-associated antigen peptides for patients with recurrent/progressive high-grade glioma. J Clin Med. 2019;8(2):263. doi:10.3390/jcm8020263.
- Tamura R, Fujioka M, Morimoto Y, Ohara K, Kosugi K, Oishi Y, Sato M, Ueda R, Fujiwara H, Hikichi T, et al. A VEGF receptor vaccine demonstrates preliminary efficacy in neurofibromatosis type 2. Nat Commun. 2019;10(1):5758. doi:10.1038/s41467-019-13640-1.
- Tamura R, Morimoto Y, Kosugi K, Sato M, Oishi Y, Ueda R, Kikuchi R, Nagashima H, Hikichi T, Noji S, et al. Clinical and histopathological analyses of VEGF receptors peptide vaccine in patients with primary glioblastoma - a case series. BMC Cancer. 2020;20(1):196. doi:10.1186/s12885-020-6589-x.
- Miyazawa M, Katsuda M, Maguchi H, Katanuma A, Ishii H, Ozaka M, Yamao K, Imaoka H, Kawai M, Hirono S, et al. Phase II clinical trial using novel peptide cocktail vaccine as a postoperative adjuvant treatment for surgically resected pancreatic cancer patients. Int J Cancer. 2017;140(4):973–82. doi:10.1002/ijc.30510.
- Suzuki N, Hazama S, Iguchi H, Uesugi K, Tanaka H, Hirakawa K, Aruga A, Hatori T, Ishizaki H, Umeda Y, et al. Phase II clinical trial of peptide cocktail therapy for patients with advanced pancreatic cancer: VENUS-PC study. Cancer Sci. 2017;108(1):73–80. doi:10.1111/cas.13113.
- Hazama S, Nakamura Y, Tanaka H, Hirakawa K, Tahara K, Shimizu R, Ozasa H, Etoh R, Sugiura F, Okuno K, et al. A phase ΙI study of five peptides combination with oxaliplatin-based chemotherapy as a first-line therapy for advanced colorectal cancer (FXV study). J Transl Med. 2014;12(1):108. doi:10.1186/1479-5876-12-108.
- Kanekiyo S, Hazama S, Takenouchi H, Nakajima M, Shindo Y, Matsui H, Tokumitsu Y, Tomochika S, Tsunedomi R, Tokuhisa Y, et al. IgG response to MHC class I epitope peptides is a quantitative predictive biomarker in the early course of treatment of colorectal cancer using therapeutic peptides. Oncol Rep. 2018;39:2385–92. doi:10.3892/or.2018.6288.
- Takeuchi S, Kagabu M, Shoji T, Nitta Y, Sugiyama T, Sato J, Nakamura Y. Anti-cancer immunotherapy using cancer-derived multiple epitope-peptides cocktail vaccination clinical studies in patients with refractory/persistent disease of uterine cervical cancer and ovarian cancer [phase 2]. Oncoimmunology. 2020;9(1):1838189. doi:10.1080/2162402X.2020.1838189.
- Tamura R, Morimoto Y, Sato M, Hikichi T, Yoshida K, Toda M. A pilot study of the adverse events caused by the combined use of bevacizumab and vascular endothelial growth factor receptor-targeted vaccination for patients with a malignant glioma. Vaccines (Basel). 2020;8(3):498. doi:10.3390/vaccines8030498.
- Tsuruma T, Hata F, Torigoe T, Furuhata T, Idenoue S, Kurotaki T, Yamamoto M, Yagihashi A, Ohmura T, Yamaguchi K, et al. Phase I clinical study of anti-apoptosis protein, survivin-derived peptide vaccine therapy for patients with advanced or recurrent colorectal cancer. J Transl Med. 2004;2(1):19. doi:10.1186/1479-5876-2-19.
- Tsuruma T, Iwayama Y, Ohmura T, Katsuramaki T, Hata F, Furuhata T, Yamaguchi K, Kimura Y, Torigoe T, Toyota N, et al. Clinical and immunological evaluation of anti-apoptosis protein, survivin-derived peptide vaccine in phase I clinical study for patients with advanced or recurrent breast cancer. J Transl Med. 2008;6(1):24. doi:10.1186/1479-5876-6-24.
- Kameshima H, Tsuruma T, Torigoe T, Takahashi A, Hirohashi Y, Tamura Y, Tsukahara T, Ichimiya S, Kanaseki T, Iwayama Y, et al. Immunogenic enhancement and clinical effect by type-I interferon of anti-apoptotic protein, survivin-derived peptide vaccine, in advanced colorectal cancer patients. Cancer Sci. 2011;102(6):1181–7. doi:10.1111/j.1349-7006.2011.01918.x.
- Kameshima H, Tsuruma T, Kutomi G, Shima H, Iwayama Y, Kimura Y, Imamura M, Torigoe T, Takahashi A, Hirohashi Y, et al. Immunotherapeutic benefit of α-interferon (IFNα) in survivin2B-derived peptide vaccination for advanced pancreatic cancer patients. Cancer Sci. 2013;104(1):124–9. doi:10.1111/cas.12046.
- Shima H, Tsurita G, Wada S, Hirohashi Y, Yasui H, Hayashi H, Miyakoshi T, Watanabe K, Murai A, Asanuma H, et al. Randomized phase II trial of survivin 2B peptide vaccination for patients with HLA-A24-positive pancreatic adenocarcinoma. Cancer Sci. 2019;110(8):2378–85. doi:10.1111/cas.14106.
- Niethammer AG, Lubenau H, Mikus G, Knebel P, Hohmann N, Leowardi C, Beckhove P, Akhisaroglu M, Ge Y, Springer M, et al. Double-blind, placebo-controlled first in human study to investigate an oral vaccine aimed to elicit an immune reaction against the VEGF-Receptor 2 in patients with stage IV and locally advanced pancreatic cancer. BMC Cancer. 2012;12(1):361. doi:10.1186/1471-2407-12-361.
- Schmitz-Winnenthal FH, Hohmann N, Niethammer AG, Friedrich T, Lubenau H, Springer M, Breiner KM, Mikus G, Weitz J, Ulrich A, et al. Anti-angiogenic activity of VXM01, an oral T-cell vaccine against VEGF receptor 2, in patients with advanced pancreatic cancer: a randomized, placebo-controlled, phase 1 trial. Oncoimmunology. 2015;4(4):e1001217. doi:10.1080/2162402X.2014.1001217.
- Yang W, Zhang W, Wang X, Tan L, Li H, Wu J, Wu Q, Sun W, Chen J, Yin Y, et al. HCA587 protein vaccine induces specific antitumor immunity mediated by CD4(+) T-cells expressing granzyme B in a mouse model of melanoma. Anticancer Agents Med Chem. 2021;21(6):738–46. doi:10.2174/1871520620666200728131951.
- Lokhov PG, Mkrtichyan M, Mamikonyan G, EE B. SANTAVAC(TM): summary of research and development. Vaccines (Basel). 2019;7(4):186. doi:10.3390/vaccines7040186.
- Painter MM, Mathew D, Goel RR, Apostolidis SA, Pattekar A, Kuthuru O, Baxter AE, Herati RS, Oldridge DA, Gouma S, et al. Rapid induction of antigen-specific CD4(+) T cells is associated with coordinated humoral and cellular immunity to SARS-CoV-2 mRNA vaccination. Immunity. 2021;54(9):2133–2142 e3. doi:10.1016/j.immuni.2021.08.001.
- Awad MM, Govindan R, Balogh KN, Spigel DR, Garon EB, Bushway ME, Poran A, Sheen JH, Kohler V, Esaulova E, et al. Personalized neoantigen vaccine NEO-PV-01 with chemotherapy and anti-PD-1 as first-line treatment for non-squamous non-small cell lung cancer. Cancer Cell. 2022;40(9):1010–1026.e11. doi:10.1016/j.ccell.2022.08.003.