ABSTRACT
Purified subunit viral antigens are weakly immunogenic and stimulate only the antibody but not the T cell-mediated immune response. An alternative approach to inducing protective immunity with small viral peptides may be the targeting of viral epitopes to immunocompetent cells by DNA and protein-engineered vaccines. This review will focus on DNA and protein-generated chimeric molecules carrying engineered fragments specific for activating cell surface co-receptors for inducing protective antiviral immunity. Adjuvanted protein-based vaccine or DNA constructs encoding simultaneously T- and B-cell peptide epitopes from influenza viral hemagglutinin, and scFvs specific for costimulatory immune cell receptors may induce a significant increase of anti-influenza antibody levels and strong CTL activity against virus-infected cells in a manner that mimics the natural infection. Here we summarize the development of several DNA and protein chimeric constructs carrying influenza virus HA317–41 fragment. The generated engineered molecules were used for immunization in intact murine and experimentally humanized NSG mouse models.
Influenza and influenza viruses
Influenza is one of the most contagious acute infectious diseases, affecting the whole body. One of the worst pandemics occurred in 1918, when approximately 50 million people died worldwide from the flu.Citation1 Currently, influenza viruses are among the most common and significant causes of respiratory infections in humans causing high morbidity and mortality. In infants, and in adults, people with chronic diseases, influenza is associated with particularly high mortality rate.Citation2
Influenza viruses are characterized by segmented linear single-stranded RNA and are classified into three types: A, B, C, and D based on the main antigenic differences. Viruses of the three types have a very similar structure. Those of type A circulate in many species of birds and infect several species of mammals, including humans; type B viruses only infect humans; type C viruses infect humans and pigs;Citation3 type D viruses are distributed mainly in cattle.Citation4 Influenza viruses type A and B are responsible for periodic seasonal outbreaks and global pandemics, influenza C viruses can affect children in some cases, while influenza D viruses do not have an actual participation in the human disease.Citation4–7
In turn, type A viruses are divided into subtypes based on their surface glycoproteins – hemagglutinins (HA) and neuraminidases (NA). HA and NA are not only the major surface glycoproteins on influenza virus particles but also the most variable and immunogenic antigens of influenza viruses. HA is responsible for the binding of the virus to sialic acid, a component of target cell receptors.Citation8,Citation9 Due to the major role of HA in the life cycle of the virus, as well as its exposure to the viral envelope, this protein is the main target of the neutralizing humoral immune response. HA is also an attractive molecule for the development of prophylactic and therapeutic approaches. The HA molecule contains different immunogenic epitope regions that are highly variable due to antigenic shift and drift, which is a major challenge in vaccine design. Among them, several conservative HA epitopes can induce antiviral immunity, and are therefore attractive targets for the construction of effective vaccines.Citation10–13
Influenza vaccines
Vaccination with live influenza vaccines or influenza subunits (purified glycoprotein) inducing a high titer of anti-HA antibodies that directly block virus adhesion to the cell surface is considered the most effective way to prevent influenza virus infection.Citation14 The antigenic shift and drift of the main viral antigens are the reason for the loss of generated antiviral immunity, also complicating the development of vaccines.Citation15,Citation16
A widely used form of human influenza vaccine is the traditional trivalent inactivated influenza vaccine, which involves circulating viral strains in humans. Groups of conservative epitopes that are able to induce an effective immune response are of potential interest for the development of influenza vaccines.Citation17,Citation18 One of these epitopes, a cortical peptide from the internal subunit of the HA protein (HA317–41), contains a conformation-dependent B-cell epitope and an I-Ed-restricted T-cell epitope.Citation19,Citation20 We have already used this peptide to create various protein or DNA chimeric molecules.Citation21–23
DNA vaccines
In recent decades, it has been shown that the injection of pure DNA encoding an antigenic molecule that is subsequently expressed by the transcription/translation systems of host cells () can induce antibody and cytotoxic responses directed to the specific antigen.Citation24–26 Anti-influenza DNA vaccines are an innovative approach consisting in the application of expression vectors encoding influenza antigen sequences that are represented by the major tissue compatibility complex (MHC) classes I and II, thus mimicking natural viral infection.Citation27–29
Figure 1. Schematic representation of the cloning of a viral fragment in a DNA expression system and in vitro expression in cell culture, followed by immunization with the DNA vaccine and boosting with the expressed fusion protein.
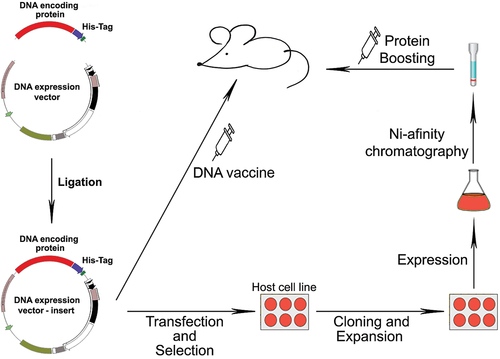
Since DNA vaccine plasmids are unable to reproduce or propagate into the surrounding cell populations, there is no risk of secondary infection.Citation30
Intramuscular and subcutaneous immunizations were shown to be the most effective approaches to DNA delivery. Only a small fraction of the injected plasmid survives after intramuscular immunization and is able to transfect the target cells. Despite the limited amount of protein expressed and the lack of transfection of professional antigen presenting cells (APCs), intramuscular immunization with DNA vectors resulted in surprisingly stable levels of virus-specific cytotoxic CD8 +T cells as well as specific antibodies.Citation31
By delivery of viral proteins directly to host cells, DNA vaccines avoid many of the problems associated with egg-based vaccine production. To create a DNA vaccine, the antigen-encoding gene is cloned into an expression plasmid for replication, and the latter is delivered to the host by traditional vaccination methods. Host cells receiving the plasmid express the vaccine antigen, which can be presented to immune cells in the context of major tissue compatibility complex (MHC) molecules. Activation of CD4+ helper cells by DNA-coded peptides presented in the context of MHC class II molecules is crucial for the production of antigen-specific antibodies, while the generation of CD8+ T cells important for viral elimination is activated mainly by endogenously expressed antigens presented by MHC class I molecules.Citation32
Protection induced by evolutionary stable influenza antigens has been associated with viral clearance mediated by viral-specific multi-reactive cytotoxic CD8+ T cells, reducing the severity of clinical disease.Citation33 Attempts to develop HA-based universal influenza vaccines have focused on the preserved stem region of the HA protein.Citation34 Mice vaccinated with plasmids encoding the PR8 antigen with headless HA generate serum antibodies for a wider range of influenza viruses than animals vaccinated with wild-type HA DNA.Citation35 HA-expressing DNA vaccines are capable of eliciting a strong humoral immune response, providing effective protection against lethal doses of homologous influenza viruses by generating a cytotoxic T-cell response.Citation36,Citation37 Immunization with a DNA vaccine with built-in adjuvant, alone or followed by protein replication stimulation, may enhance the generation of a combined protective immune response.
Cell targets for vaccine delivery
In the body, innate and acquired immunity function synchronously, with several basic connections between them. Multiple cellular receptors expressed by different types of immune cells contribute to this synchrony. One of the most important elements of this relationship is the complement system.Citation38 All evidence points to the activating fragments of the complement component 3 (C3) and their receptors, designated as complement receptor 1 (CR1) and 2 (CR2), responsible for directing and regulating the early immune response. CR1 and CR2 (, left) are expressed on B-lymphocytes and follicular dendritic cells in both humans and mice.Citation39 In mice, the simultaneous binding of CR1/2 and the B cell antigen receptor (BCR) reduces the activation threshold of antigen-specific B cells up to 1000-fold.Citation40
Antibodies bind to antigens through their antigen-binding regions (Fab fragment) and to Fc receptors on the surface of leukocytes through their Fc fragments. The binding of Fc receptors to their ligands activates different cells of the immune system, leading to phagocytosis, cell degranulation, production of various cytokines and chemokines, antibody-dependent cellular cytotoxicity (ADCC), and activation of various genes.Citation41 Of particular importance are FcγR – the receptors for IgG – the largest immunoglobulin fraction in human serum. Among the heterogeneous and widespread FcγR family, the expression of activating FcγRI (, middle) with the highest affinity for IgG molecules is limited to myeloid cells.
Recognition of FcγRI by DCs and macrophages is involved in phagocytosis, processing, and antigen presentation to neighboring T cells, which enhances the immune response. A specific sequence of the cytoplasmic region, the immunoreceptor tyrosine-based activation motif (ITAM), plays a very important role in the intracellular signaling through FcγRI. Receptor aggregation results in the phosphorylation of tyrosine from the activating ITAM motif, followed by kinase cascade activation and signal transduction to regulatory elements promoting FcγR-mediated phagocytosis.Citation42 Targeting of HA epitopes with molecules recognizing FcγRI using engineered immunoglobulin antigen-binding Fab fragments could be an effective strategy for generation of specific antibodies and cytotoxic immune response.
Other possible targets for epitopes delivering on the surface of APCs are the C-type lectin receptors (such as mannose receptor) (, right), and toll-like receptor (TLR) 4, responsible for potent cellular and humoral immune responses. These receptors could be stimulated by protein epitope carriers such as hemocyanins (Hcs) isolated from different arthropod and mollusk species and are expected to be followed by the viral epitopes internalization to either T or B lymphocytes. Thus, stimulated macrophages respond with cytokine patterns displaying Th1 profile.Citation43,Citation44
A possible approach to improve the specificity of the immune response is the use of epitope-specific vaccines. As the latter are weakly immunogenic without adjuvant, alternative pathways for presentation of the epitope to APC are being investigated. Such an alternative may be the delivery of epitopes to APC receptors involved in antigen capture during the natural pathway of infection, with subsequent activation of the corresponding T-cell subsets. Such targeting would result in selective antigen uptake and processing, leading to increased antigen presentation.Citation45
Conjugated hemocyanin-epitope vaccines
Viral recombinant or synthetic epitopes alone are weak antigens and epitope-based vaccines exhibit reduced immunogenicity. In order to enhance the entire immune response and to build protective immunity, numerous adjuvants have been developed and studied. Among them are Hcs – huge biodegradable protein molecules whose foreign biochemical structure containing specific carbohydrates expand their immunogenicity and properties as bio-adjuvants and carrier proteins. Due to their high immunogenicity, safety, and lack of side effects, the peptide-conjugated Hcs could deliver the viral epitopes to the APCs, generating cell activation and epitope processing. Immunization of influenza type A viral HA peptide-epitope adjuvanted with Hc isolated from terrestrial snail Helix pomatia (HPH) generated protective immune response in Balb/C mice.Citation46 Another epitope vaccine consisting of Tilapia lake virus (TiLV) segment 1 epitope TYTTRMHITLPI with keyhole limpet hemocyanin (KLH-S1399–410) induced a protective immune response against TiLV challenge.Citation47
Single-chain variable antibody fragments
Single-chain variable antibody fragments (scFv) are genetically engineered molecules that contain linked variable regions of antibody heavy and light chains. These recombinant molecules do not possess the constant regions of the original immunoglobulin, but retain the potential of the intact antibody to bind antigen. After genetic fusion of a pre-selected peptide epitope to engineered scFv antibodies, the entire construct may be introduced into an expression vector and used as a DNA vaccine. Such single-chain chimeric molecules can provide viral epitopes to target cells.Citation48,Citation49
The Fv fragment is the smallest immunoglobulin molecular unit with antigen-binding activities. An antibody in the scFv format consists of variable regions of heavy (VH) and light (VL) chains that are linked by a flexible peptide linker. The newly generated constructs are small in size and easily expressed in a functional form in E. coli or cell lines, which allows to improve the affinity and specificity of scFv by protein engineering.Citation50
The length of the flexible DNA linker between the two V domains is critical for obtaining the correct folding of the polypeptide chain. It was previously calculated that the peptide linker should span 3.5 nm (35 A) between the C end of the variable domain and the N end of the other one, without affecting the ability of the domains to fold and form intact antigen-binding sites.Citation51
In addition to the length, the amino acid composition also plays an important role in the design of the peptide linker. There must be a hydrophilic sequence to avoid insertion of the peptide into or between the variable domains during protein folding. Currently, the most widely used designs include sequences containing portions of Gly (glycine) and Ser (serine) residues that impart flexibility and/or charged residues such as Glu (glutamine) and Lys (lysine), which increase solubility.Citation52
Numerous scFvs have been constructed against haptens,Citation53 proteins,Citation54 carbohydrates,Citation55 receptors, tumor antigens,Citation56 and viruses.Citation57 All of these scFvs are good for use in many areas, such as medical therapies and diagnostic applications. In our studies, we used a B/T cell conserved influenza viral epitope to target activating cellular receptors. Thus, the scFv fragment of an antibody to murine CR1/2 associated with the influenza virus epitope would bind activating murine CR1/2 and immunoglobulin receptors on the surface of virus-specific B cells. An alternative system is the scFv fragment generated from an antibody to human FcγRI, delivering influenza epitopes directly to the APC.
Mouse models for testing of engineered vaccines
The generation of mouse models to study the pathogenesis of viral infections and the development of immune response is an important tool for human therapy investigations. In addition to testing vaccines and antiviral drugs, mouse models are an important part of preclinical studies. In intact mouse models, the host response to infection can be analyzed in detail, and the effectiveness of drugs and vaccines, as well as their safety, can be evaluated. The major obstacle of using intact mouse models lies in the interpretation of results in the human context. Due to differences in the cellular receptors used by viruses to penetrate targets and basic differences in human and murine innate immunity, human viruses rarely infect mice. Therefore, most mouse models exhibit susceptibility to infection by human viruses and show no symptoms of disease. Indeed, the study of immune responses in vivo with any human virus is difficult, even when appropriate animal models are used. This species barrier limiting the use of mouse models to study viral infections in humans can be overcome by generating transgenic (Tg) mouse models, with embedded human genes encoding receptors for virus entry into cells.Citation58
Unlike intact animals, knockout (KO) mouse models with blocked functions and elements involved in innate immunity may be used to study the molecular mechanisms and subcellular elements participating in the immune response to viral infections.Citation59 In our experiments, we used an intact Balb/C line as well as CR1/2 KO C57B6 mice.
Humanized mouse models are an alternative approach whereby human cells and tissues are transferred to immunodeficient mice. These models almost reproduce human disease and provide many advantages for testing human viral infections. They also represent a very important tool for testing the effectiveness of vaccines and antivirals.Citation60
A mutation in the gene encoding a key protein kinase (Prkdc) gives rise to mice with severe combined immunodeficiency (SCID), allowing the transplantation of human tissues, human peripheral blood mononuclear cells (PBMC), and hematopoietic stem cells (HSC). In addition, mice with mutations in the recombination-activating gene RAG-1 or RAG-2 (Rag1null or Rag2null) lack T and B cells. Reverse crossing of PrkdcSCID mice (CB17-PrkdcSCID) to NOD mice (non-obese diabetes) resulted in NOD.CB17-PrkdcSCID/J mice, which enhances human cell transplantation. The generation of immunodeficient mice with mutations in the common gamma chain of the interleukin 2 receptor (IL2rγnull) further improves human cell transplantation due to the lack of natural killer (NK) cells. By crossing IL2rγnull mice with PrkdSCID, or SCID, or Rag1null, or Rag2null mice, hybrids with no adaptive immunity and markedly deficient in innate immunity mechanisms are obtained. In addition, mouse NK cells are missing, which allows high levels of human cells transplantation.Citation60–62 Invariably, humanized mice are susceptible to a number of human viruses, and can be used to study the corresponding diseases caused. The biological responses of this type of humanized mice, which have been transplanted with human tissues and cells, reproduce the biological responses observed in humans better than all known murine models.Citation63–65
The efficacy of vaccines developed using various strategies, including antiviral DNA vaccines, can be tested in humanized mouse models. Sasaki et al.Citation66 were able to create a humanized mouse model to perform the safety assessment of a flu vaccine based on the expression of biomarker genes in the lungs of mice. Transferred human PBMCs were used for testing on NOD/Shi-SCID IL2r null mice in the short and long terms.
The most commonly used murine adapted influenza strains are H1N1 influenza A/Puerto Rico/8/34 (PR8) or H1N1 influenza A/WSN/1933 (WSN).Citation67 The lack of suitable small animal models for research in influenza pathogenesis and for development of vaccines and antiviral drugs is one of the most serious obstacles to progress in this field.
Genetic and protein work strategy – a mouse model system
Genetic constructs carrying the scFv and viral peptide were developed and introduced into the eukaryotic expression vector pNut having a viral promoter for foreign protein expression. The VL and VH genetic elements of the scFv encoding variants of the heavy and light chain variable regions of a monoclonal antibody (7G6) specific for CR1/2 were cloned into the eukaryotic expression vector pNut as described.Citation68 Since the generated construct is monovalent, its binding is not expected to result in multi-receptor cooperation. Making it bivalent can greatly increase the efficiency of epitope targeting. The products of human oncogenes Fos and Jun have a high binding affinity for each other. Insertion of a DNA fragment encoding a Fos protein into the scFv and a DNA fragment encoding a Jun protein into another scFv allowed the construction of a bivalent antibody.
RNA encoding HA was isolated from influenza strain A/PR/8/34, the HA317–41 fragment from HA (IP peptide) was amplified by RT-PCR and re-cloned into the pNut vector. The resulting vector constructs (pNut-IP-7G6scFv-Fos (Jun)) were amplified by the transformation of a prokaryotic competent culture.
A protein replica of a DNA chimeric molecule was constructed for prime-boost. The rat hybridoma 7G6Citation69 producing a monoclonal antibody specific for murine CR1/CR2 was cultured for large-scale production in a serum-free medium. The covalent binding of isolated and purified 7G6 monoclonal antibody to hemagglutinin IP peptide Ac-MVTGLRNIPSIQSRGLFGAIAGFIE-Ahx-K-NH2 from influenza virus strain A/PR/8/34 (7G6-IP protein chimera) was performed using the classical EDC spacer cross linking technique (Ahx-K-NH2) at the C-terminus of the peptides as described.Citation70
Another engineered protein construction was built using a Helix pomatia hemocyanin combined with the same viral hemagglutinin IP peptide.Citation71 This engineered molecule was also constructed using EDC spacer cross linking technique and could provide a strong adjuvant effect to the small IP peptide delivering IP to the targeted APC-receptors ().
What to expect – selective binding of engineered molecules to IP-specific murine B cells or activated APCs
Following in vivo expression, genetically engineered molecules are expected to bind selectively to IP-specific B cells and conduct a strong activation signal through their surface CR1/2, generating an efficient anti-influenza immune response in the host (). Recognition of the IP peptide from the 7G6-IP protein chimera by the IP-specific monoclonal antibody in the Western blot analysis confirms that the peptides conjugated with rat IgG retain their B-cell epitope and the latter is available for interaction with IP-specific B-lymphocytes.Citation68
Figure 4. Specific binding of the expressed DNA (vaccination) or protein-engineered (boosting) chimeric molecules to CR1/2 and BCR on mouse IP-specific B cells.
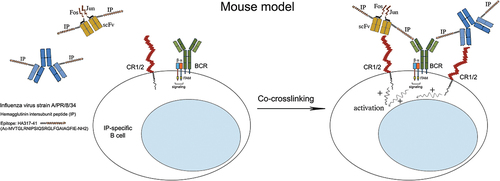
Using flow cytometry, we demonstrated the ability of the 7G6-IP chimera to bind to CR1/2 on a murine B lymphoma cell line IIA1.6 expressing CR1/2. We also found that culturing splenocytes from IP-CFA-immunized intact mice in the presence of the 7G6-IP chimera resulted in an increased number of plasma cells producing anti-IP IgG, as well as activation of peptide-specific T cells detected by IL-2 production in antigen presentation assay in vitro. Signal transduction was confirmed by the observed tyrosine phosphorylation of the receptor subunits in the CR1/2-expressing B-cell line IIA1.6 after incubation with a 7G6-IP chimeric molecule.Citation68
Alternatively, HPH-IP chimeric molecules bound the APCs and after their direct stimulation could co-stimulate the IP-specific B and T cells resulting in enhanced proliferation, activation, and secretion of antibodies and cytokines.Citation71
Induction of strong anti-influenza cytotoxic response after vaccination with engineered molecules of intact Balb/c mice
Groups of intact Balb/C mice or C57/B6 CR1/2 KO mice were immunized intramuscularly (i.m.) with the generated DNA constructs pNut-IP-7G6scFv-Fos (Jun) with or without boosting with the protein chimera (). The Balb/C group of mice treated with the protein chimera 7G6-IP produced high levels of anti-IP IgG antibodies after the second immunization, while in the group vaccinated with pNut-IP-7G6scFv-Fos/Jun increased IgG antibody levels were detected 5 weeks after the second DNA immunization and were not detected at the endpoint of observation. As expected, no IgG anti-IP antibodies were detected in the sera of C57/B6 CR1/2 KO mice injected with either DNA chimeric molecules or a protein engineered construct.Citation68
In the sera of Balb/C mice treated with pNut-IP-7G6scFv-Fos/Jun, increased levels of IFN-γ as compared to PBS-injected mice, were detected only after double DNA immunization, while animals treated with the protein chimera 7G6-IP generated high levels of IFN-γ starting with the first immunization.
Thе most important component of the antiviral immune response is the generation of virus-specific cytotoxic T cells (CTL). CTL activity of isolated spleen cells in all animals against influenza-infected 3T3 cells was studied 2 weeks after the last immunization of each group. Immunization with the 7G6-IP protein chimera caused low cytotoxic activity, while the cytotoxicity in the immunized groups with the DNA vaccine pNut-IP-7G6scFv-Fos/Jun and pNut-IP-7G6scFv-Fos/Jun +7G6-IP was significantly higher in comparison with other treated groups (, left panel).
Figure 6. Immunization with the genetic construct pNut-IP-7G6scFv-Fos/Jun boosted with protein chimera 7G6-IP Fos (left), or with HPH-adjuvanted IP (right) induces strong CTL activity in intact Balb/C mice. After the last immunization, cells from the spleen and lymph nodes were isolated and incubated together with influenza virus-infected 3T3 cells in effector: target cell ratio of 40:1. The concentration of LDH in the supernatant was measured by a commercial CytoTox assay. Results are expressed as the mean value ±SD of triplicates. p values were calculated using the two-way ANOVA test (*p < 0.05, **p < 0.01, *** p < .001), in comparison to pNut-treated or PBS-treated controls.
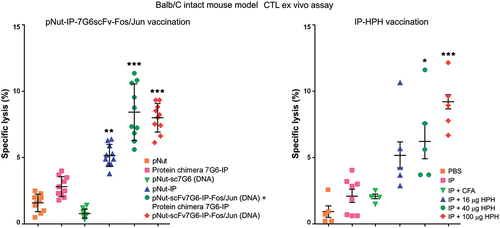
In another study, we have analyzed the ability of conjugated-IP peptides to generate protective immune response in intact Balb/C mice after immunization with HPH-IP. The administration of Hc-adjuvanted IP peptide led to the generation of specific antibody-producing B lymphocytes and virus-specific cytotoxic T lymphocytes (, right panel).Citation71
Genetic and protein work strategy – a human model system
Plasmids carrying humanized variants of the m22 heavy and light chain variable regions from human CD64-specific monoclonal antibodies were used as a PCR template. The light and heavy chains of the variable fragments were joined by overlapping PCR to generate scFv with a VL-linker-VH arrangement.Citation72
RNA encoding the HA317–41 fragment of HA (IP peptide) was isolated as described above. The IP coding region was bound to the N-terminus of scFv22 in one, two, or three copies. A dimerization domain of the Fos/Jun transcription factor was linked to the C-terminal end of scFv in the various scFv chains. The final scFv constructs were transferred to the eukaryotic expression vector pTriEx-3 Neo, having a viral promoter for foreign protein expression. pTriEx-sc22-IP-Jun contained one copy of IP, while the construct pTriEx-sc22-IP3-Fos contained three IP copies. After expression, each scFv containing Fos was able to conjugate to another scFv containing Jun at the protein level, forming these bivalent chimeric molecules.
Chinese hamster ovary cells (CHO) were transfected and used to prepare the recombinant proteins encoded by the DNA constructs pTriEx-L-sc22-IP-Jun and pTriEx-L-sc22-IP3-Fos. Stably transfected and selected clones were cultured in CHO medium, and the expressed recombinant proteins were purified from the supernatants by Ni-affinity chromatography.
Expressed sc22-IP-Jun/sc22-IP3-Fos proteins successfully bind to human FcγRI
As an alternative to the murine DNA vaccine model, the humanized version is expected to bind selectively to APCs after expression of genetically engineered molecules in vivo and conduct a strong activation signal through their surface FcγRI, generating an efficient anti-influenza immune response in the host ().
Figure 7. pTriex-sc22-IP-Jun/sc22-IP3-Fos is expected to bind selectively APCs after the expression of genetically engineered molecules in vivo followed by boosting with the expressed fusion protein, and to generate a strong activation signal through the FcγRI.
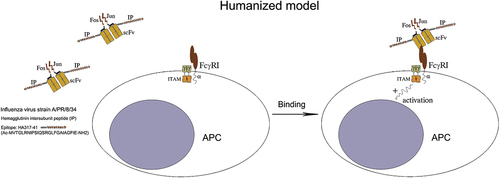
The expressed recombinant sc22-IP-Jun/sc22-IP3-Fos proteins were successfully bound to FcγRI and a binding specificity was demonstrated by flow cytometry on FcγRI-expressing human lymphoma cell line U937. Cultivation of tonsil mononuclear cells (TMC) in the presence of the protein chimeric molecules sc22-IP-Jun/sc22-IP3-Fos resulted in a significant increase in the number of anti-IP IgG secreting plasma cells in vitro. The specific binding of the fusion protein to FcγRI on APC leads to signal transduction via FcγRI.Citation72
Enhancement of anti-flu immune response after human DNA vaccination in humanized NSG mouse model
In the next step, the effect of the generated chimeric molecules sc22-IP-Jun/sc22-IP3-Fos was studied in vivo in humanized female NOD-scid IL2rγnull (NSG) mice. TMCs isolated from IP-positive healthy donors were used for humanization of the animals ().
Immunization of TMC-transferred NSG mice with sc22-IP-Jun/sc22-IP3-Fos constructs followed by stimulation with the expressed fusion protein sc22-IP-Jun/sc22-IP3-Fos resulted in high levels of anti-IP IgG antibodies in mouse sera after the last immunization as compared to empty pTriEx vector immunization. On the other hand, similar levels of anti-IP antibodies were detected after immunization and stimulation with fusion protein sc22-IP-Jun/sc22-IP3-Fos only or with DNA pTriEx-sc22-IP-Jun/sc22-IP3-Fos.Citation72
CTL activity of isolated spleen and lymph node cells from all animals against influenza-infected 3T3 cells was studied 2 weeks after the last immunization. The cytotoxic activity of NSG mice transferred with TMC and immunized with pTriEx-sc22-IP-Jun/sc22-IP3-Fos + sc22-IP-Jun/sc22-IP3-Fos fusion protein was significantly higher as compared to the other immunized groups ().
Figure 9. pTriex-sc22-IP-Jun/sc22-IP3-Fos vaccinated humanized NSG mice, boosted with the same expressed fusion protein generated an efficient anti-influenza CTL immune response in the host. Human cells were isolated from the spleens and lymph nodes of all treated animals and control groups respectively, at day 14 after the last immunization. Target 3T3 influenza virus-infected cells were cultured with isolated human cells (as effector cells) at a ratio 1:40 and LDH concentration in the supernatants was measured by a commercial CytoTox assay. Results are expressed as the mean value ±SD of triplicates. p values were calculated using the two-way ANOVA test (*p < 0.05, **p < 0.01, *** p < .001), in comparison to pTriex-treated controls.
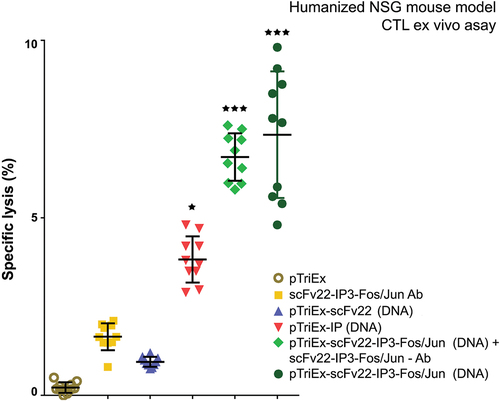
Will there be an effective anti-flu vaccine?
Viruses generally exhibit high host species specificity depending on the presence or absence of cellular receptors through which the virus enters the cell. Influenza virus is a pathogen that causes acute respiratory diseases in animals and humans and is responsible for periodic epidemics with global pandemics potential.Citation5,Citation7 Vaccination seems to be the most plausible way for preventing infection and spread of influenza viruses.
DNA vaccines have been studied in various viral and bacterial infections, as well as in some tumors, demonstrating properties suitable for fighting influenza.Citation28–30 While inactivated influenza vaccines induce mainly antiviral antibodies,Citation73 DNA vaccines engage both humoral and cellular immunity to provide effective protection.Citation35 Their production does not require classical viral multiplication, and thus allows a rapid response to the onset of influenza epidemics.Citation74 However, the very good results achieved in mouse models are not reproduced in larger animals and humans.Citation25,Citation75 The results in mice were obtained in genetically homogeneous animals and cannot be compared with the high heterogeneity of the human population. Larger animal models, and primates in particular, are much closer to humans and provide useful information about the need to develop adjuvants and means for the delivery of vaccine particles.Citation58
Host-defense mechanisms against influenza virus have been intensively investigated through the years. Different engineered chimeric molecules, exploring various B and T cell epitopes built in protein or DNA constructs, have been used for generation of protective anti-flu cellular and humoral immune response.Citation76–84 The attempt to obtain a universal immune response covering all influenza A viral strains requires the detection and design of time-preserved B and T cell epitopes from the most immunogenic components of the virus. The ability of the immunodominant viral proteins HA and NA to mutate rapidly, known as antigenic drift and antigenic shift, can render any existing immunity useless. NA proteins comprise up to 80% of all proteins on the viral surface and, logically, a lot of vaccines rely on this protein. The antigenic drift in HA epitopes complicates this task, and it is now clear that major viral HA epitopes mutate faster than the other regions of the protein.Citation85 On the other hand, various conserved HA epitopes are possible targets for vaccines, including the 25-amino acid peptide HA317–41, containing an I-Ed-restricted T-cell epitope and a conformation-dependent B-cell epitope.Citation21–23 In our research, the HA317–41 peptide was used as a part of the recombinant DNA molecules employed in both animal models.
Cytotoxic CD8+ T cells are a crucial factor in anti-influenza immunity, eliminating virus-infected cells in humans and in animal models of infection.Citation5,Citation86 The development of these cells is directly dependent on the effective presentation of MHC-I type viral epitopes. Information about these epitopes is necessary for the construction of T-cell inducing vaccines containing conserved epitopes.
Several HA-based vaccine candidates have been constructed and tested in animal models and clinical trials. The different approaches elicit both broadly protective CD4+ and CD8+ T cell responses, local antigen-specific T cell responses, antibody-dependent cellular cytotoxicity (ADCC), neutralizing cross-reactive antibodies, IFN-γ and IL-4 production, as well as full protection of mice challenged with a broad panel of influenza A virus strains and subtypes.Citation87–90
A possible way to increase the immunogenicity of small viral peptides carrying recombinant epitopes is to deliver them to the cells directly involved in the immune response. The role of co-stimulatory surface receptors in enhancing the immune response has been well studied.Citation91 Murine CR1/2 are expressed on B-lymphocytes and regulate B cell activation. Crosslinking of BCR and complement receptors lowers the threshold for B cell activation, leading to enhanced antigen presentation by B cells.Citation92 We relied on this mechanism when immunizing intact Balb/C mice with chimeric DNA and protein molecules to ensure the crosslinking of the two receptors and the generation of an antiviral immune response.
An alternative approach is to target the model viral epitope by DNA-encoding and protein antibodies to professional APC, such as dendritic cells and macrophages. Both cell types possess activating receptors such as FcγRI and play a major role in the natural antiviral immune response. We employed this approach for targeting of the immune response in the native Balb/C mice targeting the IP peptide to mannose receptors and TLR4. Vaccination of mice demonstrates that the HPH is acceptable as a potential bio-adjuvant for subunit vaccines and it could be used as a natural adjuvant or protein carrier.Citation46 Alternatively, we exploited humanized NSG mice immunized with the recombinant DNA molecule and its expressed protein replica.Citation72 In both mouse models, anti-viral antibodies and a strong anti-influenza CD8+ T cell cytotoxic response against influenza A virus were generated. Against the background of contradictory data about DNA vaccines using viral carriers of the antigens of interest, our results are a good argument to focus on DNA vaccines containing safely modified vectors, providing transcription and export from host cells after immunization.
DNA vector vaccines have a potent promoter for eukaryotic expression and unmethylated CpG motifs acting as adjuvants. Thus, once DNA enters the host cells, the encoded antigen is expressed and presented to the immune system in a manner that mimics the natural infection.Citation93 Such preventive influenza vaccines provide a broadly cross-reactive and long-lasting CD8+ T and B cell response that can be developed by the specific targeting of conserved multi-epitope systems to the cells involved in the immune response.Citation94,Citation95
In conclusion, this study revealed a concept for screening antigen epitopes of emerging viral diseases, providing promising approaches for development and evaluation of protective epitope vaccines against viral diseases.
Acknowledgments
We thank Polia Marinova (American College of Sofia, Bulgaria) for the helpful discussions and ideas.
Disclosure statement
No potential conflict of interest was reported by the author(s).
Additional information
Funding
References
- Quiñones-Parra S, Clemens E, Wang Z, Croom H, Kedzierski L, McVernon J, Vijaykrishna D, Kedzierska K. A role of influenza virus exposure history in determining pandemic susceptibility and CD8+ T cell responses. J Virol. 2016;90(15):6936–12. doi:10.1128/JVI.00349-16.
- Zimmer S, Burke D. Historical perspective — Emergence of influenza A (H1N1) viruses. N Engl J Med. 2009;361(3):279–285. doi:10.1056/NEJMra0904322.
- Hause BM, Collin EA, Liu R, Huang B, Sheng Z, Lu W, Wang D, Nelson EA, Li F. Characterization of a novel influenza virus in cattle and swine: proposal for a new genus in the Orthomyxoviridae family. MBio. 2014;5(2):e00031–e14. doi:10.1128/mBio.00031-14.
- Ruiz M, Puig A, Bassols M, Fraile L, Armengol R. Influenza D virus: a review and update of its role in bovine respiratory syndrome. Viruses. 2022;14(12):2717. doi:10.3390/v14122717.
- Krammer F, Smith G, Fouchier R, Peiris M, Kedzierska K, Doherty PC, Palese P, Shaw ML, Treanor J, Webster RG, et al. Influenza. Nat Rev Dis Primers. 2018;4(1): Article number: 3. doi:10.1038/s41572-018-0002-y.
- Mishra AC, Chadha MS, Choudhary ML, Potdar VA. Pandemic influenza (H1N1) 2009 is associated with severe disease in India. PLoS ONE. 2010;5:e10540. doi:10.1371/journal.pone.0010540.
- Guthmiller J, Wilson P. Harnessing immune history to combat influenza viruses. Curr Opin Immunol. 2018;53:187–95. doi:10.1016/j.coi.2018.05.010.
- Wilson JR, Guo Z, Reber A, Kamal RP, Music N, Gansebom S, Bai Y, Levine M, Carney P, Tzeng W-P, et al. An influenza a virus (H7N9) anti-neuraminidase monoclonal antibody with prophylactic and therapeutic activity in vivo. Antivir Res. 2016;135:48–55. doi:10.1016/j.antiviral.2016.10.001.
- Sakai T, Nishimura SI, Naito T, Saito M. Influenza A virus hemagglutinin and neuraminidase act as novel motile machinery. Sci Rep. 2017;7(1):45043. doi:10.1038/srep45043.
- Pizzorno A, Padey B, Terrier O, Rosa-Calatrava M. Drug repurposing approaches for the treatment of influenza viral infection: reviving old drugs to fight against a long-lived enemy. Front Immunol. 2019. doi:10.3389/fimmu.2019.00531.
- Tutykhina I, Esmagambetov I, Bagaev A, Pichugin A, Lysenko A, Shcherbinin D, Sedova E, Logunov D, Shmarov M, Ataullakhanov R, et al. Vaccination potential of B and T epitope-enriched NP and M2 against influenza a viruses from different clades and hosts. PLoS ONE. 2018;13(1):e0191574. doi:10.1371/journal.pone.0191574.
- Weidenbacher P, Kim P. Protect, modify, deprotect (PMD): a strategy for creating vaccines to elicit antibodies targeting a specific epitope. Proc Natl Acad Sci. 2019;116(20):9947–9952. doi:10.1073/pnas.1822062116.
- Guo C, Zhang H, Xie X, Liu Y, Sun L, Li H, Yu P, Hu H, Sun J, Li Y, et al. H1N1 influenza virus epitopes classified by monoclonal antibodies. Exp Ther Med. 2018;16:2001–7. doi:10.3892/etm.2018.6429.
- Gamblin SJ, Skehel JJ. Influenza hemagglutinin and neuraminidase membrane glycoproteins. J Biol Chem. 2010;285(37):28403–9. doi:10.1074/jbc.R110.129809.
- Hamilton BS, Whittaker GR, Daniel S. Influenza virus-mediated membrane fusion: determinants of hemagglutinin fusogenic activity and experimental approaches for assessing virus fusion. Viruses. 2012;4(7):1144–68. doi:10.3390/v4071144.
- Chambers BS, Parkhouse K, Ross TM, Alby K, Hensley SE. Identification of hemagglutinin residues responsible for H3N2 antigenic drift during the 2014–2015 influenza season. Cell Rep. 2015;12(1):1–6. doi:10.1016/j.celrep.2015.06.005.
- Mallajosyula VV, Citron M, Ferrara F, NJ T, Liang X, JA F, Varadarajan R. Hemagglutinin sequence conservation guided stem immunogen design from influenza a H3 subtype. Front Immunol. 2015;6:329. doi:10.3389/fimmu.2015.00329.
- Krammer F, Palese P. Advances in the development of influenza virus vaccines. Nat Rev Drug Discov. 2015;14(3):167–82. doi:10.1038/nrd4529.
- Nagy Z, Rajnavolgyi E, Hollosi M, Tóth GK, Váradi G, Penke B, Tóth I, Horváth A, Gergely J, Kurucz I, et al. The intersubunit region of the influenza virus haemagglutinin is recognized by antibodies during infection. Scand J Immunol. 1994;40:281–91. doi:10.1111/j.1365-3083.1994.tb03464.x.
- Rajnavolgyi E, Horvath A, Gogolak P, Tóth GK, Fazekas G, Fridkin M, Pecht I. Characterizing immunodominant and protective influenza hemagglutinin epitopes by functional activity and relative binding to major histocompatibility complex class II sites. Eur J Immunol. 1997;27:3105–14. doi:10.1002/eji.1830271205.
- Ivanovska N, Tchorbanov A, Prechl J, Maximova V, Voynova E, Vassilev TL. Immunization with a DNA chimeric molecule encoding a hemagglutinin peptide and a scFv CD21-specific antibody fragment induces long-lasting IgM and CTL responses to influenza virus. Vaccine. 2006;24:1830–1837. doi:10.1016/j.vaccine.2005.10.029.
- Prechl J, Tchorbanov A, Horvath A, Baiu DC, Hazenbos W, Rajnavölgyi É, Kurucz I, Capel PJA, Erdei A. Targeting of influenza epitopes to murine CR1/CR2 using single-chain antibodies. Immunopharmacol. 1999;42:159–65. doi:10.1016/S0162-3109(99)00025-9.
- Gesheva V, Idakieva K, Kerekov N, Nikolova K, Mihaylova N, Doumanova L, Tchorbanov A. Marine gastropod hemocyanins as adjuvants of non-conjugated bacterial and viral proteins. Fish And Shellfish Immunol. 2011;30(1):135–142. doi:10.1016/j.fsi.2010.09.018.
- Donnelly JJ, Wahren B, Liu MA. DNA vaccines: progress and challenges. J Immunol. 2005;175(2):633–9. doi:10.4049/jimmunol.175.2.633.
- Porter KR, Raviprakash K. DNA vaccine delivery and improved immunogenicity. Curr Issues Mol Biol. 2017;22:129–38. doi:10.21775/cimb.022.129.
- Li L, Petrovsky N. Molecular mechanisms for enhanced DNA vaccine immunogenicity. Expert Rev Vaccines. 2016;15(3):313–29. doi:10.1586/14760584.2016.1124762.
- Song L, Xiong D, Kang X, Yang Y, Wang J, Guo Y, Xu H, Chen S, Peng D, Pan Z, et al. An avian influenza a (H7N9) virus vaccine candidate based on the fusion protein of hemagglutinin globular head and salmonella typhimurium flagellin. BMC Biotechnol. 2015;15(1):79. doi:10.1186/s12896-015-0195-z.
- Wong TM, Ross TM. Use of computational and recombinant technologies for developing novel influenza vaccines. Expert Rev Vaccines. 2016;15(1):41–51. doi:10.1586/14760584.2016.1113877.
- Berlanda Scorza F, Tsvetnitsky V, Donnelly JJ. Universal influenza vaccines: shifting to better vaccines. Vaccine. 2016;34(26):2926–33. doi:10.1016/j.vaccine.2016.03.085.
- Carter DM, Darby CA, Lefoley BC, Crevar CJ, Alefantis T, Oomen R, Anderson SF, Strugnell T, Cortés-Garcia G, Vogel TU, et al. Design and characterization of a computationally optimized broadly reactive hemagglutinin vaccine for H1N1 influenza viruses. J Virol. 2016;90(9):4720–34. doi:10.1128/JVI.03152-15.
- Rice J, Ottensmeier C, Stevenson F. DNA vaccines: precision tools for activating effective immunity against cancer. Nat Rev Cancer. 2008;8(2):108–20. doi:10.1038/nrc2326.
- Grodeland G, Fredriksen AB, GÅ L, Vikse E, Fugger L, Bogen B. Antigen targeting to human HLA class II molecules increases efficacy of DNA vaccination. J Immunol. 2016;197:3575–85. doi:10.4049/jimmunol.1600893.
- Barría MI, Garrido JL, Stein C, Scher E, Ge Y, Engel SM, Kraus TA, Banach D, Moran TM. Localized mucosal response to intranasal live attenuated influenza vaccine in adults. J Infect Dis. 2013;207:115–24. doi:10.1093/infdis/jis641.
- Whittle JR, Wheatley AK, Wu L, Lingwood D, Kanekiyo M, Ma SS, Narpala SR, Yassine HM, Frank GM, Yewdell JW, et al. Flow cytometry reveals that H5N1 vaccination elicits cross-reactive stem-directed antibodies from multiple ig heavy-chain lineages. J Virol. 2014;88(8):4047–57. doi:10.1128/JVI.03422-13.
- Yan J, Morrow MP, Chu JS, Racine T, Reed CC, Khan AS, Broderick KE, Kim JJ, Kobinger GP, Sardesai NY, et al. Broad cross- protective anti-hemagglutination responses elicited by influenza microconsensus DNA vaccine. Vaccine. 2018;36(22):3079–89. doi:10.1016/j.vaccine.2017.09.086.
- DeZure AD, Coates EE, Hu Z, Yamshchikov GV, Zephir KL, Enama ME, Plummer SH, Gordon IJ, Kaltovich F, Andrews S, et al. An avian influenza H7 DNA priming vaccine is safe and immunogenic in a randomized phase I clinical trial. NPJ Vaccines. 2017;2:15. doi:10.1038/s41541-017-0016-6.
- Stachyra A, Pietrzak M, Macioła A, Protasiuk A, Olszewska M, Śmietanka K, Minta Z, Góra-Sochacka A, Kopera E, Sirko A, et al. A prime/boost vaccination with HA DNA and pichia-produced HA protein elicits a strong humoral response in chickens against H5N1. Virus Res. 2017;232:41–7. doi:10.1016/j.virusres.2017.01.025.
- Shibabaw T, Molla MD, Teferi B, Ayelign B. Role of IFN and complements system: innate immunity in SARS-CoV-2. J Inflamm Res. 2020;13:507–18. doi:10.2147/JIR.S267280.
- Kranich J, Krautler N-J. How follicular dendritic cells shape the B-Cell antigenome. Front Immunol. 2016;7:225. doi:10.3389/fimmu.2016.00225.
- Bennett K, Rooijakkers S, Gorham R. Let’s tie the knot: marriage of complement and adaptive immunity in pathogen evasion, for better or worse. Front Microbiol. 2017;8:89. doi:10.3389/fmicb.2017.00089.
- García-García E, Rosales C. Fc receptor signaling in leukocytes: role in host defense and immune regulation. Curr Immunol Rev. 2009;5(3):227–42. doi:10.2174/157339509788921229.
- Guilliams M, Bruhns P, Saeys Y, Hammad H, Lambrecht BN. The function of Fcγ receptors in dendritic cells and macrophages. Nat Rev Immunol. 2014;14:94–108. doi:10.1038/nri3582.
- Zhong T-Y, Arancibia S, Born R, Tampe R, Villar J, Campo M, Manubens A, Becker MI. Hemocyanins stimulate innate immunity by inducing different temporal patterns of proinflammatory cytokine expression in macrophages. J Immunol. 2016;196(11):4650–4662. doi:10.4049/jimmunol.1501156.
- Jiménez J, Salazar M, Arancibia S, Villar J, Salazar F, Brown G, Lavelle EC, Martínez-Pomares L, Ortiz-Quintero J, Lavandero S, et al. TLR4, but neither dectin-1 nor dectin-2, participates in the Mollusk hemocyanin-induced proinflammatory effects in antigen-presenting cells from mammals. Front Immunol. 2019;10:1136. doi:10.3389/fimmu.2019.01136.
- Grødeland G, Fossum E, Bogen B. Polarizing T and B cell responses by APC-Targeted subunit vaccines. Front Immunol. 2015;6:367. doi:10.3389/fimmu.2015.00367.
- Gesheva V, Chausheva S, Stefanova N, Mihaylova N, Doumanova L, Idakieva K, Tchorbanov A. Helix pomatia hemocyanin - a novel bio-adjuvant for viral and bacterial antigens. Int Immunopharmacol. 2015;26(1):162–168. doi:10.1016/j.intimp.2015.03.011.
- Gong YM, Wei XF, Zheng YY, Li Y, Yu Q, Li PF, Zhu B, Schultz-Cherry S. Combining phage display technology with in silico-designed epitope vaccine to elicit robust antibody responses against emerging pathogen tilapia lake virus. J Virol. 2023;97(4):e0005023. doi:10.1128/jvi.00050-23.
- Ahmad Z, Yeap S, Ali A, Ho W-Y, Noorjahan B, Hamid M. scFv antibody: principles and clinical application. Clin Dev Immunol. 2012;2012:1–15. doi:10.1155/2012/980250.
- Satheeshkumar PK. Expression of single chain variable fragment (scFv) molecules in plants: a comprehensive update. Mol Biotechnol. 2020;62(3):151–67. doi:10.1007/s12033-020-00241-3.
- Sarker A, Rathore AS, Gupta RD. Evaluation of scFv protein recovery from E. coli by in vitro refolding and mild solubilization process. Microb Cell Fact. 2019;18:5. doi:10.1186/s12934-019-1053-9.
- Ahmad ZA, Yeap SK, Ali AM, Ho WY, Alitheen NB, Hamid M. scFv antibody: principles and clinical application. Clin Dev Immunol. 2012;2012:980250. doi:10.1155/2012/980250.
- Yusakul G, Sakamoto S, Pongkitwitoon B, Tanaka H, Morimoto S. Effect of linker length between variable domains of single chain variable fragment antibody against daidzin on its reactivity. Biosci Biotechnol Biochem. 2016;80(7):1306–12. doi:10.1080/09168451.2016.1156482.
- Kobayashi N, Ohtoyo M, Wada E, Kato Y, Mano N, Goto J. Generation of a single-chain Fv fragment for the monitoring of deoxycholic acid residues anchored on endogenous proteins. Steroids. 2005;70(4):285–94. doi:10.1016/j.steroids.2004.11.012.
- Dai K, Zhu H, Ruan C. Generation and characterization of recombinant single chain Fv antibody that recognizes platelet glycoprotein Ibα. Thromb Res. 2003;109(2–3):137–44. doi:10.1016/S0049-3848(03)00152-X.
- Sakai K, Shimizu Y, Chiba T, Matsumoto-Takasaki A, Kusada Y, Zhang W, Nakata M, Kojima N, Toma K, Takayanagi A, et al. Isolation and characterization of phage-displayed single chain antibodies recognizing nonreducing terminal mannose residues. 1. A new strategy for generation of anti-carbohydrate antibodies. Biochemistry. 2007;46(1):253–62. doi:10.1021/bi061875e.
- Liang GQ, Liu J, Zhou XX, Lin ZX, Chen T, Chen G, Wei H. Anti-CXCR4 single-Chain variable fragment antibodies have anti-tumor activity. Front Oncol. 2020;10:571194. doi:10.3389/fonc.2020.571194. PMID: 33392074; PMCID: PMC7775505.
- Rajput R, Sharma G, Rawat V, Gautam A, Kumar B, Pattnaik B, Pradhan HK, Khanna M. Diagnostic potential of recombinant {scFv} antibodies generated against hemagglutinin protein of influenza A virus. Front Immunol. 2015;6. doi:10.3389/fimmu.2015.00440.
- Herati RS, Wherry EJ. What is the predictive value of animal models for vaccine efficacy in humans? Consideration of strategies to improve the value of animal models. Cold Spring Harb Perspect Biol. 2018;10(4):a031583. doi:10.1101/cshperspect.a031583.
- Krishnakumar V, Durairajan SSK, Alagarasu K, Li M, Dash AP. Recent updates on mouse models for human immunodeficiency, influenza, and dengue viral infections. Viruses. 2019;11(3):252. doi:10.3390/v11030252.
- Lai F, Chen Q. Humanized mouse models for the study of infection and pathogenesis of human viruses. Viruses. 2018;10(11):643. doi:10.3390/v10110643. PMID: 30453598; PMCID: PMC6266013.
- Theocharides AP, Rongvaux A, Fritsch K, Flavell RA, Manz MG. Humanized hemato-lymphoid system mice. Haematologica. 2016;101(1):5–19. doi:10.3324/haematol.2014.115212.
- Ishikawa F, Yasukawa M, Lyons B, Yoshida S, Miyamoto T, Yoshimoto G, Watanabe T, Akashi K, Shultz LD, Harada M. Development of functional human blood and immune systems in NOD/SCID/IL2 receptor γ chainnull mice. Blood. 2005;106(5):1565–73. doi:10.1182/blood-2005-02-0516.
- Brehm MA, Bortell R, Verma M, Shultz LD, Greiner DL. Chapter 11 - Humanized mice in translational immunology. In: Tan SL, editors. Translational immunology. Academic Press; 2016. p. 285–326. doi:10.1016/B978-0-12-801577-3.00012-5.
- Shultz LD, Brehm MA, Garcia-Martinez JV, Greiner DL. Humanized mice for immune system investigation: progress, promise and challenges. Nat Rev Immunol. 2012;12(11):786–98. doi:10.1038/nri3311.
- Walsh N, Kenney L, Jangalwe S, Aryee KE, Greiner DL, Brehm MA, Shultz LD. Humanized mouse models of clinical disease. Annu Rev Pathol. 2017;12(1):187–215. doi:10.1146/annurev-pathol-052016-100332.
- Sasaki E, Momose H, Hiradate Y, Furuhata K, Mizukami T, Hamaguchi I. Development of a preclinical humanized mouse model to evaluate acute toxicity of an influenza vaccine. Oncotarget. 2018;9(40):25751. doi:10.18632/oncotarget.25399.
- Dadonaite B, Gilbertson B, Knight ML, Trifkovic S, Rockman S, Laederach A, Brown LE, Fodor E, Bauer DLV. The structure of the influenza a virus genome. Nature Microbiology. 2019;4(11):1781–1789. doi:10.1038/s41564-019-0513-7.
- Kerekov N, Ivanova I, Mihaylova N, Nikolova M, Prechl J, Tchorbanov A. Built-in adjuvanticity of genetically and protein engineered chimeric molecules for targeting of influenza a peptide epitopes. Immunol Research. 2014;60(1):23–34. doi:10.1007/s12026-014-8489-0.
- Kinoshita T, Takeda J, Hong K, Kozono H, Sakai H, Inoue K. Monoclonal antibodies to mouse complement receptor type 1 (CR1): their use in a distribution study showing that mouse erythrocytes and platelets are CR1-negative. J Immunol. 1988;140:3066. doi:10.4049/jimmunol.140.9.3066.
- Tchorbanov A, Voynova E, Mihaylova N, Todorov T, Nikolova M, Yomtova VM, Chiang B-L, Vassilev T. Selective silencing of DNA-specific B lymphocytes delays lupus activity in MRL/lpr mice. Eur J Immunol. 2007;37:3587–3596. doi:10.1002/eji.200737143.
- Gesheva V, Chausheva S, Stefanova N, Mihaylova N, Doumanova L, Idakieva K, Tchorbanov A. Helix pomatia hemocyanin - a novel bio-adjuvant for viral and bacterial antigens. Int Immunopharmacol. 2015;1:162–8. doi:10.1016/j.intimp.2015.03.011. Epub 2015 Mar 20. PMID: 25799956.
- Ivanova I, Mihaylova N, Manoylov I, Makatsori D, Lolov S, Nikolova M, Mamalaki A, Prechl J, Tchorbanov A. Targeting of Influenza viral epitopes to antigen presenting cells by Genetically Engineered Chimeric Molecules in Humanized NSG transfer model. Hum Gene Ther. 2018;29:(9):1056–1070. doi:10.1089/hum.2018.100.
- Rimmelzwaan GF, McElhaney JE. Correlates of protection: novel generations of influenza vaccines. Vaccine. 2008;26:D41–4. doi:10.1016/j.vaccine200807043.
- Xenopoulos A, Pattnaik P. Production and purification of plasmid DNA vaccines: is there scope for further innovation? Expert Rev Vaccines. 2014;13(12):1537–51. doi:10.1586/14760584.2014.968556.
- Gerdts V, Wilson HL, Meurens F, Van Drunen Littel-Van Den Hurk S, Wilson D, Walker S, Wheler C, Townsend H, Potter AA. Large animal models for vaccine development and testing. ILAR J. 2015;56:53. doi:10.1093/ilar/ilv009.
- Klenerman P, Zinkernagel R. Original antigenic sin impairs cytotoxic T lymphocyte responses to viruses bearing variant epitopes. Nature. 1998;394(6692):482–5. doi:10.1038/28860.
- Bot A, Isobe H, Bona C. Immunodeficient mouse models in the characterization of the protective immunity to influenza virus. Folia Microbiol. 1998;43(5):477–8. doi:10.1007/BF02820795.
- Brumeanu TD, Casares S, Harris PE, Dehazya P, Wolf I, von Boehmer H, Bona CA. Immunopotency of a viral peptide assembled on the carbohydrate moieties of self immunoglobulins. Nat Biotechnol. 1996;14(6):722–5. doi:10.1038/nbt0696-722. PMID: 9630978.
- Zaghouani H, Steinman R, Nonacs R, Shah H, Gerhard W, Bona C. Presentation of a viral T cell epitope expressed in the CDR3 region of a self immunoglobulin molecule. Sci. 1993;259(5092):224–7. doi:10.1126/science.7678469. PMID: 7678469.
- Casares S, Brumeanu TD, Bot A, Bona CA. Protective immunity elicited by vaccination with DNA encoding for a B cell and a T cell epitope of the A/PR/8/34 influenza virus. Viral Immunol. 1997;10(3):129–36. PMID: 9344335. doi: 10.1089/vim.1997.10.129.
- Bot A, Antohi S, Bot S, Garcia-Sastre A, Bona C. Induction of humoral and cellular immunity against influenza virus by immunization of newborn mice with a plasmid bearing a hemagglutinin gene. Int Immunol. 1997;9(11):1641–50. doi: 10.1093/intimm/9.11.1641. PMID: 9418125.
- Brumeanu TD, Bot A, Bona CA, Dehazya P, Wolf I, Zaghouani H. Engineering of doubly antigenized immunoglobulins expressing T and B viral epitopes. Immunotechnology. 1996;2(2):85–95. doi:10.1016/1380-2933(96)85196-7. PMID: 9373317.
- Li SQ, Schulman JL, Moran T, Bona C, Palese P. Influenza a virus transfectants with chimeric hemagglutinins containing epitopes from different subtypes. J Virol. 1992;66(1):399–404. doi:10.1128/JVI.66.1.399-404.1992. PMID: 1370088; PMCID: PMC238299.
- Bot A, Reichlin A, Isobe H, Bot S, Schulman J, Yokoyama WM, Bona CA. Cellular mechanisms involved in protection and recovery from influenza virus infection in immunodeficient mice. J Virol. 1996;70(8):5668–72. doi:10.1128/JVI.70.8.5668-5672.1996. PMID: 8764086; PMCID: PMC190532.
- Kirchenbaum GA, Ross TM. Eliciting broadly protective antibody responses against influenza. Curr Opin Immunol. 2014;28:71–6. doi:10.1016/j.coi.2014.02.005.
- Nussing S, Sant, S., Koutsakos, M., Subbarao, K., Nguyen, T.H.O., Kedzierska, K. Innate and adaptive T cells in influenza disease. Front Med. 2018;12(1):34–47. doi:10.1007/s11684-017-0606-8.
- Liao HY, Wang SC, Ko YA, Lin KI, Ma C, Cheng TR, Wong CH. Chimeric hemagglutinin vaccine elicits broadly protective CD4 and CD8 T cell responses against multiple influenza strains and subtypes. Proc Natl Acad Sci U S A. 2020 Jul 28;117(30):17757–17763. doi:10.1073/pnas.2004783117. Epub 2020. PMID: 32669430; PMCID: PMC7395492.
- Puente-Massaguer E, Vasilev K, Beyer A, Loganathan M, Francis B, Scherm MJ, Arunkumar GA, González-Domínguez I, Zhu X, Wilson IA, et al. Chimeric hemagglutinin split vaccines elicit broadly cross-reactive antibodies and protection against group 2 influenza viruses in mice. Sci Adv. 2023;9(37):eadi4753. doi:10.1126/sciadv.adi4753. Epub 2023 Sep 13. PMID: 37703367; PMCID: PMC10499326.
- Nachbagauer R, Feser J, Naficy A, Bernstein DI, Guptill J, Walter EB, Berlanda-Scorza F, Stadlbauer D, Wilson PC, Aydillo T, et al. A chimeric hemagglutinin-based universal influenza virus vaccine approach induces broad and long-lasting immunity in a randomized, placebo-controlled phase I trial. Nat Med. 2021;27(1):106–14. doi:10.1038/s41591-020-1118-7.
- Puente-Massaguer E, Beyer A, Loganathan M, Sapse I, Carreño JM, Bajic G, Sun W, Palese P, Krammer F. Bioprocess development for universal influenza vaccines based on inactivated split chimeric and mosaic hemagglutinin viruses. Front Bioeng Biotechnol. 2023;11:1097349. doi:10.3389/fbioe.2023.1097349. PMID: 37342504; PMCID: PMC10277804.
- Carroll MC. The complement system in B cell regulation. Mol Immunol. 2004;41(2–3):141–6. doi:10.1016/j.molimm.2004.03.017.
- Molina H, Holers VM, Li B, Fang Y-F, Mariathasan S, Goellner J, Strauss-Schoenberger J, Karr RW, Chaplin DD. Markedly impaired humoral immune response in mice deficient in complement receptors 1 and 2. Proc Natl Acad Sci U S A. 1996;93:3357. doi:10.1073/pnas.93.8.3357.
- Saha R, Killian S, Robert D. DNA vaccines: a mini review. Recent patents on DNA & gene sequences. 2011;5(2):92–96. doi:10.2174/187221511796392114.
- Carter DM, Darby CA, Johnson SK, Carlock MA, Kirchenbaum GA, DiNapoli J, Vogel TU, Delagrave S, DiNapoli J, Kleanthous H, et al. Elicitation of protective antibodies against a broad panel of H1N1 viruses in ferrets pre-immune to historical H1N1 influenza viruses. J Virol. 2017;91. doi:10.1128/JVI.01283-17.
- Crevar CJ, Carter DM, Lee KY, Ross TM. Cocktail of H5N1 COBRA HA vaccines elicit protective antibodies against H5N1 viruses from multiple clades. Hum Vaccin Immunother. 2015;11(3):572–83. doi:10.1080/21645515.2015.1012013.