ABSTRACT
Staphylococcal Enterotoxin B (SEB), produced by Staphylococcus aureus (S. aureus), is a powerful superantigen that induces severe immune disruption and toxic shock syndrome (TSS) upon binding to MHC-II and TCR. Despite its significant impact on the pathogenesis of S. aureus, there are currently no specific therapeutic interventions available to counteract the mechanism of action exerted by this toxin. In this study, we have identified a human monoclonal antibody, named Hm0487, that specifically targets SEB by single-cell sequencing using PBMCs isolated from volunteers enrolled in a phase I clinical trial of the five-antigen S. aureus vaccine. X-ray crystallography studies revealed that Hm0487 exhibits high affinity for a linear B cell epitope in SEB (SEB138–147), which is located distantly from the site involved in the formation of the MHC-SEB-TCR ternary complex. Furthermore, in vitro studies demonstrated that Hm0487 significantly impacts the interaction of SEB with both receptors and the binding to immune cells, probably due to an allosteric effect on SEB rather than competing with receptors for binding sites. Moreover, both in vitro and in vivo studies validated that Hm0487 displayed efficient neutralizing efficacy in models of lethal shock and sepsis induced by either SEB or bacterial challenge. Our findings unveil an alternative mechanism for neutralizing the pathogenesis of SEB by Hm0487, and this antibody provides a novel strategy for mitigating both SEB-induced toxicity and S. aureus infection.
Introduction
Staphylococcus aureus (S. aureus) is a leading and formidable pathogen both within hospitals and in the community, posing a great threat to global public health due to the widespread emergence of antibiotic-resistant strains. S. aureus possesses the capability to secrete a wide array of virulence factors, including toxins, proteases, adhesins, and immune-modulatory factors. Upon interacting with host components, these virulence factors play crucial roles in evading the immune system and inflicting severe damage to the human body,Citation1 and are critical in the pathogenesis of the bacterium. There are more than 20 different staphylococcal enterotoxins (SEs) encoded by different isolates of S. aureus. Among numerous clinical lineages, sequence type (ST) 59 has become the predominant community-associated methicillin-resistant S. aureus (CA-MRSA) in Aisa, and research in the southwest of China has found that seb was the most prevalent in the MRSA ST59 isolates, which implies a significant role and high prevalence of Staphylococcal enterotoxin B (SEB).Citation2,Citation3
SEB, a T cell superantigen secreted by S. aureus, is capable of activating immune cells, followed by forming a ternary complex with MHC-II on antigen-presenting cells (APCs) and TCR on T cells,Citation4–6 resulting in nonspecifically and sustained activation of massive T cell clones and triggering the production of a variety of pro-inflammatory cytokines and chemokines.Citation7,Citation8 This cytokine storm not only impairs the host’s capacity to mount a focused immune response against the bacterium but also has the potential to cause severe toxic shock syndrome, a life-threatening illness characterized by fever, rash, and systemic organ failure.Citation9 Besides, SEB also shows the ability to bind co-stimulatory receptors CD28 and CD86, thereby activating target cells without forming the ternary complex.Citation10 Furthermore, SEB is stable and has the capability of aerosol transmission, making it a potent biological warfare agent classified as Category B by the United States CDC.Citation11
Given the importance of SEB as a superantigen and bioterrorism threat agent, extensive research has been directed toward neutralizing its biological activity, and strategies being explored include the development of vaccines, monoclonal antibodies, small molecule inhibitors, and other blocking agents.Citation12,Citation13 Monoclonal antibodies, a conventional toxin neutralization strategy, have been proven to exhibit high specificity, affinity, and safety. A great number of antibody strains from various origins have been described elsewhere, with their capacity to neutralize toxins and antimicrobial activity validated by a variety of in vivo and ex vivo models.Citation14,Citation15 To date, the majority of SEB-specific monoclonal antibodies bind and neutralize the site where SEB forms ternary complexes with MHC-II and TCR. Additionally, a few antibodies recognize epitopes distant from this region and demonstrate substantial protection upon administration.Citation16
Previously, we have developed a genetically engineered vaccine against S. aureus comprised of five antigens, which contains a SEB mutant (L45R, Y89A, and Y94A) with no enterotoxin activity and is currently undergoing phase III clinical trials (CTR2016004). In this study, utilizing peripheral blood mononuclear cells (PBMCs) from clinical trial participants who received this vaccine, we successfully identified a monoclonal antibody against SEB by single B-cell sequencing. This antibody, namely Hm0487, exhibited high affinity with SEB, as confirmed by ELISA and biolayer interferometry (BLI) analysis. The structure of Hm0487-SEB complex revealed that Hm0487 binds to a linear B cell epitope (SEB138–147) that is located distant from the active site in which the MHC II-SEB-TCR ternary complexes were formed. This epitope-specific binding is functional and neutralizes wild-type SEB both in vitro and in vivo, and offers broader protective efficacy against both SEB-induced toxicity and S. aureus infection.
Materials and methods
Ethics statement
Animal experiments were performed with the approval of the Laboratory Animal Welfare and Ethics Committee of the Army Medical University (AMUWEC20230477). All animal handling procedures were carried out strictly in accordance with the regulations of the P.R. China on the use and care of laboratory animals.
Animals and bacterial strains
Six- to eight-week-old female BALB/c mice were purchased from Vital River (Beijing, China). Mice were bred and housed under specific-pathogen-free (SPF) conditions with free access to food and water. Two MRSA strains, namely ST59 and USA300, were used in this study. Among them, ST59 harbors genes encoding SEB, SEK, SEQ, and SEY, whereas USA300 expresses SEK and SEQ. Bacteria were grown in tryptic soy broth (TSB) with shaking at 220 rpm at 37°C.
Preparation of recombinant protein and mAb
Wild-type SEB and the SEB mutant (mSEB) were expressed using Escherichia coli expression system and then purified from the bacterial cell lysate using anion exchange chromatography column (Cytiva, Cat # 17516601),as described previously.Citation17 Hm0487 was expressed in HEK293F expression system. In detail, HEK293F cells were transfected with the plasmid DNAs encoding the heavy and light chains of Hm0487, the cells were then incubated in an orbital shaker incubator at 37°C, 125 rpm, and 5% CO2 for 5 days. Cellular supernatant was harvested by centrifuging, and Hm0487 was captured with protein A agarose (Beyotime, Cat # P2015). For the crystallization study, purified M0686 was digested using immobilized papain (Sigma, Cat # P4762) according to the manufacturer’s instructions. The Hm0487 was first buffered in 0.1 M PB and then digested in a buffer containing 0.1 M EDTA and 0.5 M cysteine-HCl (pH 7.0) at 37°C for 30 min. The Fab was purified by protein A affinity chromatography and gel filtration (Cytiva, Cat # 28990944).
Affinity assays and competitive binding assays
ELISA was used to determine the EC50 of Hm0487. In brief, the wells of microtiter plates were coated with 0.1 μg of SEB or mSEB overnight at 4°C. After washing the wells three times with PBS, 1% bovine serum albumin (BSA) was used to block the nonspecific binding. Then, serial dilutions of Hm0487 were added and incubated for 1 h at 37°C, followed by capture with anti-human HRP-IgG (Abcam, Cat # ab97225). Next, trimethyl benzene (TMB) solution was added and incubated for 10 min at 37°C, and the reaction was terminated by adding 2 M H2SO4. The absorbance of each well was read at 450 nm using a microplate reader.
BLI was performed to measure the dissociation constant (Kd) of Hm0487. Protein A biosensor was used to immobilize Hm0487, then incubated with a serial dilution of SEB or mSEB. The binding and dissociation of Hm0487 and antigens were detected using standard procedures. The Kd value was determined using association and dissociation rates.
Mouse model
To establish a mouse toxic shock model, mice were sensitized by intraperitoneally injection with 10 mg of D-galactosamine 30 min before SEB (10 μg) challenge.Citation18 Hm0487 or PBS were injected intravenously 24 h before the challenge. The morbidity and mortality were monitored for 24 h. For the cytokine assays, blood was collected and centrifuged 6 h after SEB challenge. The serum was then collected to detect IL-6, TNF-α and IFN-γ concentrations by ELISA kits (Dakewe, Cat # 1210002, 1210602, 1217202).
As for the sepsis model, mice were challenged intravenously by different strains of S. aureus 24 h after the intravenous administration of 150 μg or 300 μg of Hm0487 per mice, the morbidity and mortality were monitored for 7 days. Besides, the kidneys of the mice were collected 48 h after the challenge. The tissues were grounded in PBS using glass homogenizers and then streaked on TSA plates or fixed with paraformaldehyde and stained with HE for microscopic examination.
Western blotting (WB)
Western blotting was used to confirm the binding of Hm0487 or the expression of SEB by S. aureus. Samples were boiled with protein loading buffer for 5 min, and added to the gels. The proteins were then transferred from the gel onto PVDF membranes. The membranes were blocked in 5% skimmed milk at room temperature for 2 h, then washed and incubated with Hm0487 at 4°C overnight. They were then incubated with anti-human HRP-IgG for 1 h after washing. Finally, the membranes were washed and developed using a chemiluminescence method according to the manufacturer’s instructions.
Fluorescence-activated cell sorting (FACS)
Human PBMCs were isolated from healthy volunteers, and the T cells were isolated by CD3 magnetic cell sorting kit according to the manufactory guide (Biolegend, Cat # 480133). 2.5 × 106 CD3+ cells or CD3− cells were added into the 96-well plate and incubated with a mixture of SEB-FITC and antibodies or medium for a period, and then the cells were washed with PBS, stained and fixed. The binding of SEB to the immune cells was detected using a flow cytometry device.
Crystallization and structure determination
The concentration of the Fab fragment in complex with mSEB was adjusted to approximately 14 mg/mL. A total of 1056 conditions from Index, Crystal screen, Macrosol MD, Multixal, Proplex MD, Wizard Precipitant Synergy, PEG/ion, PEG/RX, Pi-PEG, and JCSG-Plus were used for screening of crystals using the sitting-drop vapor-diffusion technique. Diffraction data were collected at the Shanghai Synchrotron Radiation Facility on the beamline BL19U (wavelength, 0.97919 Å). For data collection, the crystals were cryoprotected by soaking them in a reservoir solution supplemented with 20% (v/v) glycerol before flash-cooling in liquid nitrogen. The dataset was processed using the HKL2000 software package.
Statistical analysis
Statistical analyses were performed using GraphPad Prism 9.0 (GraphPad Software). All data were represented as the mean ± standard deviation (SD). Data were analyzed using Log-rank (Mantel-Cox) test,One-way ANOVA, Tukey’s multiple comparison test, and two-tailed Student’s t test. p < .05 was considered statistically significant.
Results
Hm0487 binds SEB with high affinity
Hm0487 is determined as IgG1 by sequence analysis. This classification is significant as IgG1 antibodies are known for their ability to mediate effective immune responses, including opsonization and phagocytosis, making them a valuable class for therapeutic applications.Citation19 ELISA was then employed to quantitatively assess the binding of Hm0487 to both SEB and mSEB. The results, as illustrated in , revealed that Hm0487 bound to SEB and mSEB with half-maximal effective concentration (EC50) values of 9.131 ng/mL and 7.022 ng/mL, respectively. Further, the binding properties between Hm0487 and SEB were determined through BLI assays, which revealed that Hm0487 exhibited binding affinities of 46.6 nM and 0.288 nM for SEB and mSEB, respectively (). Additionally, western blotting analysis revealed that Hm0487 is capable of recognizing both SEB and mSEB (), which demonstrated that Hm0487 interacts with a linear B cell epitope on SEB. Taken together, these results indicate that Hm0487 binds to SEB and mSEB with high affinity.
Figure 1. Hm0487 binds SEB with high affinity.
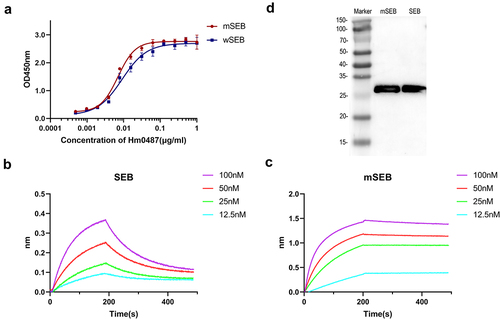
Hm0487 recognizes a novel liner B cell epitope of SEB
To reveal the structural basis for the interactions between Hm0487 and SEB, we determined the crystal structure of mSEB in complex with the Fab fragment of Hm0487 (). The crystal structure was ultimately refined to a resolution of 2.06 Å (), where one mSEB-Fab complex was located in one asymmetric unit. The structure of mSEB in the complex folds similarly to the wild-type SEB described previously, which contains two domains. Domain 1 is composed of three α helices (α1-α3) and five β strands (β1-β5), and this domain has been previously identified to exert superantigen activity upon interaction with TCR and MHC II.Citation20 In contrast, domain 2 consists of two α helices (α4 and α5) and two short β strands (β8 and β11), and a twisted β-sheet in domain 2 is formed by strands β6, β7, β9, β10, and β12 (). Notably, the Fab fragment of Hm0487 interacts with SEB by recognizing β7, which is distant from domain 1 and suggests that the antibody exhibits no direct impact on the superantigen activity of SEB.
Figure 2. Hm0487 recognizes a novel liner epitope in SEB.
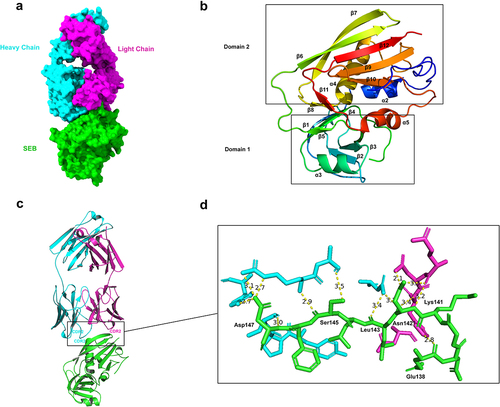
Table 1. Refinement statistics for mSEB-Hm0487Fab.
In Hm0487, CDR2 of light chain and CDR2, CDR3 of heavy chain are involved in the interactions with SEB β7 strand (). Structural analysis of the interface revealed that eight residues in SEB, including Glu138, Lys141, Asn142, Leu143, Ser145 and Asp147, are involved in direct interaction with Hm0487 (). A vast network of intermolecular hydrogen bonds and electrostatic interactions, such as salt bridges, are formed between these residues of SEB and Hm0487, which are listed in . These results illustrated that Hm0487 recognizes a liner B cell epitope, termed SEB138–147, on SEB.
Table 2. The interactions between SEB and the Fab of Hm0487.
To further investigate the impact of Hm0487 on the function of SEB, we aligned the structure of the MHC-SEB-TCR ternary complex derived from the Protein Database (PDB) with the Hm0487-SEB complex determined in this study. It is evident that Hm0487 and these immune receptors interact with distinct domains of SEB (), which are located distantly from each other, and there is no overlap between the key residues on the interfaces of these complexes (). However, research has demonstrated that SEB116–133, an immunodominant B cell epitope of SEB, can effectively elicit a protective humoral immune response in mice.Citation21 Moreover, another study revealed that SEB124–154 peptide blocked the activation of T-cells by SEB in in vitro experiments.Citation22 Additionally, CD28 was reported to recognize a linear epitope SEB150–161Citation23 (). All three epitopes were located adjacent to SEB138–147 identified in this study, suggesting that Hm0487 may potentially impact the binding of SEB to CD28 or other undiscovered receptors on T cells.
Figure 3. SEB interacts with TCR, MHC-II and Hm0487 through distinct domains.
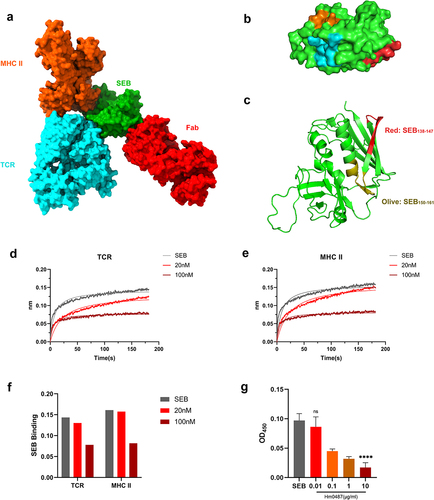
Hm0487 inhibits the interaction of SEB with target cells
MHC-II and TCR are two major receptors for SEB, and the interactions between these molecules are crucial as they form the basis of the ternary complex that is pivotal in the pathogenic mechanism of SEB.Citation24 Therefore, we investigated whether Hm0487, upon binding to SEB, could affect the binding of SEB to these receptors. As shown in , the BLI response signal clearly showed the interactions between SEB and both receptors. However, when SEB was pre-incubated with various concentrations of Hm0487, the response signal decreased obviously in a concentration-dependent manner (). This result suggested a potential allosteric effect of Hm0487 on SEB, which further interferes with the formation of the ternary complex between SEB, TCR, and MHC II. Furthermore, ELISA was employed to identify the capacity of Hm0487 to inhibit the binding of SEB to CD28. The results demonstrated that the binding of CD28 to the immobilized SEB was significantly inhibited when different concentrations of antibody solutions were added (). This indicates that antibodies may also inhibit toxin activity by affecting the binding of SEB to the costimulatory receptor CD28.
Then, using FITC-labeled SEB, we evaluated the capacity of Hm0487 in inhibiting the binding of SEB to T cells and other single nucleus cells isolated from PBMCs. The results from flow cytometry illustrated that SEB was able to bind approximately 10% of the T-cell subset and this binding capacity decreased following incubation with Hm0487, indicating that Hm0487 can impact the interaction between SEB and T cells (). In CD3-negative cells, Hm0487 showed a distinct effect with significantly increased SEB binding to the cells. This effect, however, was not observed after replacing the full-length antibody with its Fab fragment (), which indicated that the Fc fragment is efficient in interacting with Fc receptors on CD3-negative cells, and may potentially enhance phagocytosis by forming an antigen–antibody complex with SEB.
Figure 4. Hm0487 impact the binding of SEB with target cells.
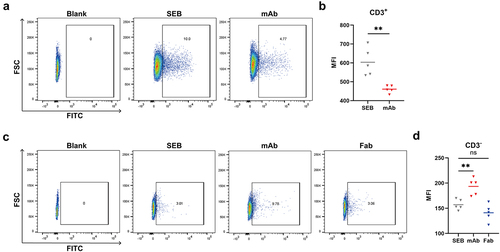
Hm0487 neutralizes SEB in vivo
In order to determine the protective efficacy of Hm0487 against SEB, we next employed the D-galactosamine-sensitized mouse, which is a widely accepted model,Citation16,Citation18,Citation25,Citation26 to test the protective efficacy of Hm0487 in vivo. As shown in , all mice in PBS group (n = 8) died within 24 h after being challenged with 10 μg of SEB, underscoring the lethal effect of SEB. In contrast, four and seven mice survived the lethal challenge of SEB in groups that received 20 µg and 100 µg of Hm0487, respectively, 24 h before the challenge. There was no significant improvement in the survival rate of mice following an increase in the antibody dosage to 400 μg per mice, indicating 100 μg per mice may be the minimum amount of Hm0487 to completely neutralize SEB. (Figure S1) Consistent with the survival rate, Hm0487 appeared to suppress the SEB-induced cytokine storm, as evidenced by the inflammatory cytokines detected in the serum of mice (). These results highlight the efficacy of Hm0487 in neutralizing SEB and suggest a dose-dependent response in conferring protective efficacy.
Figure 5. Hm0487 protect mice from lethal SEB challenge.
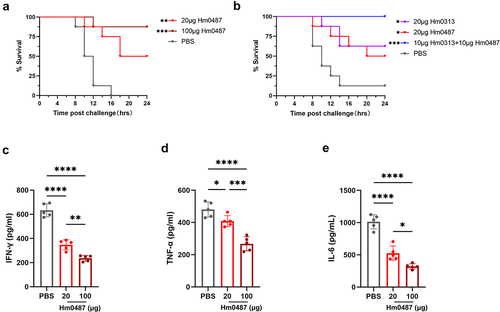
In addition, Hm0487 exerted a comparable protective effect with the previously validated SEB neutralizing antibody named Hm0313,Citation17 which recognized a linear epitope involved in the formation of ternary complex (). Furthermore, enhanced neutralizing activity was observed when the two antibodies were co-administered. This synergistic effect suggests that Hm0487 and Hm0313 bind to different epitopes on SEB, and they could provide a wider range of protection against the toxin.
Hm0487 protects S. aureus induced sepsis in mice
The in vivo efficacy of Hm0487 was further evaluated using a mouse sepsis model. First, mice were challenged with different doses of S. aureus ST59 and USA300. Although USA300 does not express SEB, it does express two enterotoxins, SEK and SEQ, which contain sequences that are conserved with antibody recognition epitopes (). The cross-reactivity of the antibody with the enterotoxins produced by USA300 was confirmed by Western blot analysis. (Figure S2) According to the results, 1 × 108 CFUs of ST59 and 3 × 108 CFUs of USA300 were identified as lethal in mice (data not shown). Then, we tested the protective effect of Hm0487 against these two S. aureus strains, and mice treated with PBS were served as control. As expected, mice in PBS group succumbed within 4 days challenged with ST59 and USA300; by contrast, significantly higher survival rates were observed in the group treated with 150 ug and 300 ug of Hm0487, and the dead mice in these groups exhibited significantly longer survival time compared to PBS group (), suggesting that Hm0487 is protective in vivo.
Figure 6. Hm0487 protect against S. aureus-induced sepsis.
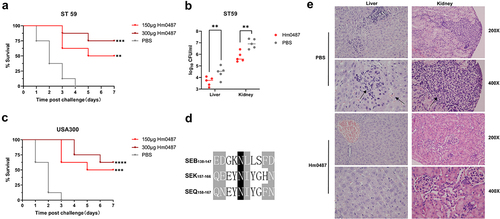
Next, mice were challenged intravenously with 4 × 107 CFUs of ST59 24 h after the intravenous administration of 300 µg of Hm0487, the bacterial burden and histopathological changes in the liver and kidneys, two crucial organs that suffer from sepsis, were evaluated. Consistent with the above results, mice treated with Hm0487 resulted in a significant reduction in, bacterial burden in liver and kidney compared to PBS group (). Furthermore, S. aureus has the potential to induce congestion and inflammatory cell infiltration in the liver. In the kidney, S. aureus can cause severe abscesses. Notably, in the Hm0487-treated group, these pathological changes and the extent of inflammatory cell infiltration were markedly alleviated compared to the PBS control group (). These data established that Hm0487 not only protects against S. aureus-induced mortality but also mitigates the pathological damage caused by bacterial infection.
Discussion
SEB has long been considered a highly virulent and hazardous bacterial exotoxin, and extensive research has focused on elucidating its pathogenicity and structural basis.Citation27–29 Structural analysis of the interaction between SEB, MHC II, and TCR has provided a clear understanding that SEB exerts its super-antigenic activity through the formation of a ternary complex, which triggers a robust and unlimited T-cell response.Citation30 Various monoclonal antibodies originating from different sources have been discovered against the activity center of SEB, and most of them have demonstrated potent in vivo neutralizing capabilities.Citation8 As the research progressed, another conserved domain of SEB was found to bind surface receptors of immune cells.Citation31 One group successfully confirmed that SEB binds to the co-stimulatory receptors B7–2 and CD28 that are responsible for immune cell activation, and they efficiently blocked the superantigenic activity of SEB using mimetic peptides, both in vitro and in vivo.Citation32 Furthermore, researchers have discovered that SEB not only enhances TNF-α production in response to the TLR2 ligand Pam3Cys in central nervous system (CNS) infection but also causes damage to nasal polyp epithelial cell integrity by triggering TLR2 in chronic rhinosinusitis with nasal polyps (CRSwNP).Citation33,Citation34 Additionally, studies have demonstrated that SEB is capable of stimulating the production of chemokines from human vaginal epithelial cells (HVECs) through human CD40.Citation35 These findings indicate that SEB may target multiple receptors to exert its activity.
Several strains of human monoclonal antibodies against SEB were obtained from the peripheral blood of clinical subjects. Among these strains, Hm0487 was extensively studied and found to specifically recognize a linear epitope of SEB and its mutant with high affinity and binding activity. The Fab fragment of Hm0487 was enzymatically purified, co-crystallized with SEB, and the linear epitope recognized by Hm0487 was identified using X-ray diffraction. Structural analysis showed that this epitope was located within the β7 strand and did not overlap with the super-antigenic active center of SEB. This result suggests that Hm0487 may function solely as a binding antibody without neutralizing toxin activity. Nevertheless, we conducted in vitro and in vivo evaluations to assess its protective efficacy.
In vitro studies demonstrated that Hm0487 affected the binding capacity of SEB to the structural domains of TCR Vβ and MHC IIα, without completely obstructing the formation of the ternary complex. This result is consistent with the findings of a previous study that antibodies can indirectly reduce the binding of SEB to receptors through allosteric changes.Citation16 It is plausible that the antibody induced subtle alterations in the structure of SEB, thereby disrupting its equilibrium when binding to the receptor. Upon incubating of Hm0487 with the toxin at the cellular level, we revealed that this antibody inhibited the interaction between SEB and T cells. Since SEB was able to directly bind to TCR and CD28 on T cells and activate them even in the absence of APCs,Citation36,Citation37 and the domain where SEB utilized to bind CD28 is adjacent to the epitope recognized by Hm0487. Hence, it is speculated that the antibody acts as a neutralizing antibody by affecting the binding of SEB to TCR and CD28, consequently attenuating signals associated with T cell activation. Furthermore, it was experimentally observed that Hm0487, after forming an antigen–antibody complex with SEB, was able to enhance the uptake of toxin by antigen-presenting cells, and this function may potentially be mediated through the Fc fragment of the antibody.
In animal experiments, it was found that Hm0487 significantly improved survival and prolonged survival time in a mouse model of toxic shock. This effect was comparable to that of a previously identified strain of neutralizing antibody.Citation17 Moreover, the antibody demonstrated significant improvements in survival, reduced pathological damage, and decreased bacterial colonization in mice with S. aureus sepsis. Combined with the findings from in vitro experiments, it is hypothesized that Hm0487 exerts its protective efficacy through two mechanisms. Firstly, it inhibits the binding of SEB to TCR and CD28, thereby attenuating the over-activation of T-cells. Secondly, it forms an antigen–antibody complex with SEB, which accelerates phagocytosis and clearance of toxins by the APCs through Fc segments mediated by the APCs.
Increasing evidence suggests that superantigens, including SEB, may have more potential receptors in the host, and their pathogenic mechanisms are more intricate than previously discovered.Citation38–40 One limitation of this study is that we have not identified any new potential receptors or pathogenic mechanisms. While Hm0487 identified in this study can provide effective protection in animal models, it is important to note that blocking a single site of SEB may not completely neutralize the pathogenic activity of the toxin. This limitation arises from the fact that laboratory simulations often fail to accurately replicate real-life toxin invasions or bacterial infections. Additionally, some of the selected assays may not provide a comprehensive understanding of the pathological process due to limitations in existing knowledge and methods.
In conclusion, we have confirmed that Hm0487 is a highly effective human monoclonal antibody that targets a novel linear epitope on SEB, and it efficiently mitigates both SEB-induced toxicity and S. aureus infection. Further, this study demonstrates the potential existence of an additional active structural domain in SEB, apart from the active center, to form a ternary complex that is susceptible to antibody blockade. Moreover, staphylococcal superantigens like enterotoxins may possess unidentified or unverified receptors and target cells of action.
Author contributions
JZ, HF, and HZ designed research. HF, LZ, WW, FY, YY, and HJ performed the experiments. HF, XZ, ZZ analyzed the data. QG, WZ contributed reagents/materials/analysis tools. HF, and JZ wrote the paper.
Supplemental Material
Download Zip (1.9 MB)Acknowledgments
This work was supported by the National Key Research and Development Program of China (2021YFC2301405), National Natural Science Foundation of China (32070941, 32270989).
Disclosure statement
The authors declare that the research was conducted in the absence of any commercial or financial relationships that could be construed as a potential conflict of interest.
Data availability statement
Data associated with this study are present in the manuscript or the supplementary information.
Supplementary material
Supplemental data for this article can be accessed on the publisher’s website at https://doi.org/10.1080/21645515.2024.2360338
Additional information
Funding
References
- Foster TJ. Immune evasion by Staphylococci. Nat Rev Microbiol. 2005;3(12):948–10. doi:10.1038/nrmicro1289.
- Bae JS, Da F, Liu R, He L, Lv H, Fisher EL, Rajagopalan G, Li M, Cheung GYC, Otto M. et al. Contribution of staphylococcal enterotoxin B to Staphylococcus aureus systemic infection. J Infect Dis. 2020;223(10):1766–75. doi:10.1093/infdis/jiaa584.
- Sun C, Wang Q, Li W-T, Wen D-N, Chen C-H, Yang X, Shi W, Meng Q-H, Yao K-H, Qian S-Y. et al. Molecular characteristics and antimicrobial susceptibility of Staphylococcus aureus among children with respiratory tract infections in southwest China. World J Pediatr. 2020;16(3):284–92. doi:10.1007/s12519-019-00317-4.
- Garcia C, Briggs C, Zhang L, Guan L, Gabriel JL, Rogers TJ. Molecular characterization of the putative T-cell receptor cavity of the superantigen staphylococcal enterotoxin B. Immunology. 1998;94(2):160–6. doi:10.1046/j.1365-2567.1998.00493.x.
- Jardetzky TS, Brown JH, Gorga JC, Stern LJ, Urban RG, Chi Y-I, Stauffacher C, Strominger JL, Wiley DC. Three-dimensional structure of a human class II histocompatibility molecule complexed with superantigen. J Immunol. 2018;201(7):711–8. doi:10.1038/368711a0.
- Wang LM, Zhao YW, Li Z, Guo Y, Jones LL, Kranz DM, Mourad W, Li H. Crystal structure of a complete ternary complex of TCR, superantigen and peptide-MHC. Nature Structural & Molecular Biology. 2007;14(2):169–71. doi:10.1038/nsmb1193.
- Thammavongsa V, Kim HK, Missiakas D, Schneewind O. Staphylococcal manipulation of host immune responses. Nat Rev Microbiol. 2015;13(9):529–43. doi:10.1038/nrmicro3521.
- Fraser JD, Proft T. The bacterial superantigen and superantigen-like proteins. Immunol Rev. 2008;225:226–43. doi:10.1111/j.1600-065X.2008.00681.x.
- Darenberg J, Söderquist B, Normark BH, Norrby‐Teglund A. Differences in potency of intravenous polyspecific immunoglobulin G against streptococcal and staphylococcal superantigens: Implications for therapy of toxic shock syndrome. Clin Infect Dis. 2004;38(6):836–42. doi:10.1086/381979.
- Kunkl M, Amormino C, Spallotta F, Caristi S, Fiorillo MT, Paiardini A, Kaempfer R, Tuosto L. Bivalent binding of staphylococcal superantigens to the TCR and CD28 triggers inflammatory signals independently of antigen presenting cells. Front Immunol. 2023;14:15. doi:10.3389/fimmu.2023.1170821.
- Benkerroum N. Staphylococcal enterotoxins and enterotoxin-like toxins with special reference to dairy products: An overview. Crit Rev Food Sci Nutr. 2018;58(12):1943–70. doi:10.1080/10408398.2017.1289149.
- Mantis NJ. Vaccines against the category B toxins: Staphylococcal enterotoxin B, epsilon toxin and ricin. Adv Drug Deliv Rev. 2005;57(9):1424–39. doi:10.1016/j.addr.2005.01.017.
- Kaempfer R, Arad G, Levy R, Hillman D, Nasie I, Rotfogel Z. CD28: direct and critical receptor for superantigen toxins. Toxins. 2013;5(9):1531–42. doi:10.3390/toxins5091531.
- Varshney AK, Wang XB, MacIntyre J, Zollner RS, Kelleher K, Kovalenko OV, Pechuan X, Byrne FR, Fries BC. Humanized staphylococcal enterotoxin B (SEB)–specific monoclonal antibodies protect from SEB intoxication and Staphylococcus aureus infections alone or as adjunctive therapy with vancomycin. J Infect Dis. 2014;210(6):973–81. doi:10.1093/infdis/jiu198.
- Chen G, Karauzum H, Long H, Carranza D, Holtsberg FW, Howell KA, Abaandou L, Zhang B, Jarvik N, Ye W. et al. Potent neutralization of staphylococcal enterotoxin B in vivo by antibodies that block binding to the T-Cell receptor. J Mol Biol. 2019;431(21):4354–67. doi:10.1016/j.jmb.2019.03.017.
- Dutta K, Varshney AK, Franklin MC, Goger M, Wang X, Fries BC. Mechanisms mediating enhanced neutralization efficacy of staphylococcal enterotoxin B by combinations of monoclonal antibodies. J Biol Chem. 2015;290(11):6715–30. doi:10.1074/jbc.M114.630715.
- Liu YY, Song Z, Ge S, Zhang J, Xu L, Yang F, Lu D, Luo P, Gu J, Zou Q. et al. Determining the immunological characteristics of a novel human monoclonal antibody developed against staphylococcal enterotoxin B. Human Vaccines Immunother. 2020;16(7):1708–18. doi:10.1080/21645515.2020.1744362.
- Miethke T, Wahl C, Heeg K, Echtenacher B, Krammer PH, Wagner H. T cell-mediated lethal shock triggered in mice by the superantigen staphylococcal enterotoxin B: critical role of tumor necrosis factor. J Exp Med. 1992;175(1):91–8. doi:10.1084/jem.175.1.91.
- Dan N, Setua S, Kashyap VK, Khan S, Jaggi M, Yallapu M, Chauhan S. Antibody-drug conjugates for cancer therapy: chemistry to clinical implications. Pharmaceuticals. 2018;11(2):22. doi:10.3390/ph11020032.
- Swaminathan S, Furey W, Pletcher J, Sax M. Crystal structure of staphylococcal enterotoxin B, a superantigen. Nature. 1992;359(6398):801–6. doi:10.1038/359801a0.
- Zhao Z, Sun HQ, Wei SS, Li B, Feng Q, Zhu J, Zeng H, Zou Q-M, Wu C. Multiple B-cell epitope vaccine induces a staphylococcus enterotoxin B-specific IgG1 protective response against MRSA infection. Sci Rep. 2015;5(1):12371. doi:10.1038/srep12371.
- Soos JM, Johnson HM. Multiple binding sites on the superantigen, staphylococcal enterotoxin B, imparts versatility in binding to MHC class II molecules. Biochem Biophys Res Commun. 1994;201(2):596–602. doi:10.1006/bbrc.1994.1743.
- Kaempfer R. Bacterial superantigen toxins, CD28, and drug development. Toxins. 2018;10(11):6. doi:10.3390/toxins10110459.
- Deacy AM, Gan SK, Derrick JP. Superantigen recognition and interactions: functions, mechanisms and applications. Front Immunol. 2021;12:731845. doi:10.3389/fimmu.2021.731845.
- Hu N, Qiao C, Wang J, Wang Z, Li X, Zhou L, Wu J, Zhang D, Feng J, Shen B. et al. Identification of a novel protective human monoclonal antibody, LXY8, that targets the key neutralizing epitopes of staphylococcal enterotoxin B. Biochem Biophys Res Commun. 2021;549:120–7. doi:10.1016/j.bbrc.2021.02.057.
- Xia T, Liang S, Wang H, Hu S, Sun Y, Yu X, Han J, Li J, Guo S, Dai J. et al. Structural basis for the neutralization and specificity of staphylococcal enterotoxin B against its MHC class II binding site. Mabs-austin. 2014;6(1):119–29. doi:10.4161/mabs.27106.
- Levy R, Rotfogel Z, Hillman D, Popugailo A, Arad G, Supper E, Osman F, Kaempfer R. Superantigens hyperinduce inflammatory cytokines by enhancing the B7-2/CD28 costimulatory receptor interaction. Proc Natl Acad Sci U S A. 2016;113(42):E6437–e46. doi:10.1073/pnas.1603321113.
- Leder L, Llera A, Lavoie PM, Lebedeva MI, Li H, Sékaly R-P, Bohach GA, Gahr PJ, Schlievert PM, Karjalainen K. et al. A mutational analysis of the binding of staphylococcal enterotoxins B and C3 to the T cell receptor β chain and major histocompatibility complex class II. J Exp Med. 1998;187(6):823–33. doi:10.1084/jem.187.6.823.
- Hu DL, Nakane A. Mechanisms of staphylococcal enterotoxin-induced emesis. Eur J Pharmacol. 2014;722:95–107. doi:10.1016/j.ejphar.2013.08.050.
- Deacy AM, Gan SKE, Derrick JP. Superantigen recognition and interactions: functions, mechanisms and applications. Front Immunol. 2021;12:16. doi:10.3389/fimmu.2021.731845.
- Di Stefano A, Paulesu L, Niccolai N, Scarselli M, Soldani P, Neri P. Identification of critical residues of staphylococcal enterotoxin B for lymphomonocyte proliferation and cytokine production. J Pept Res. 1998;52(2):130–6. doi:10.1111/j.1399-3011.1998.tb01367.x.
- Kaempfer R, Popugailo A, Levy R, Arad G, Hillman D, Rotfogel Z. Bacterial superantigen toxins induce a lethal cytokine storm by enhancing B7-2/CD28 costimulatory receptor engagement, a critical immune checkpoint. Recept & Clin Invest. 2017;4(1):e1500. doi:10.14800/rci.1500.
- Vidlak D, Mariani MM, Aldrich A, Liu S, Kielian T. Roles of toll-like receptor 2 (TLR2) and superantigens on adaptive immune responses during CNS staphylococcal infection. Brain Behav Immun. 2011;25(5):905–14. doi:10.1016/j.bbi.2010.09.016.
- Martens K, Seys SF, Alpizar YA, Schrijvers R, Bullens DMA, Breynaert C, Lebeer S, Steelant B. Staphylococcus aureus enterotoxin B disrupts nasal epithelial barrier integrity. Clin Exp Allergy. 2021;51(1):87–98. doi:10.1111/cea.13760.
- Schlievert PM, Cahill MP, Hostager BS, Brosnahan AJ, Klingelhutz AJ, Gourronc FA, Bishop GA, Leung DYM. Staphylococcal superantigens stimulate epithelial cells through CD40 to produce chemokines. mBio. 2019;10(2):11. doi:10.1128/mBio.00214-19.
- Lamphear JG, Stevens KR, Rich RR. Intercellular adhesion molecule-1 and leukocyte function-associated antigen-3 provide costimulation for superantigen-induced T lymphocyte proliferation in the absence of a specific presenting molecule. J Immunol. 1998;160(2):615–23. doi:10.4049/jimmunol.160.2.615.
- Kunkl M, Amormino C, Caristi S, Tedeschi V, Fiorillo MT, Levy R, Popugailo A, Kaempfer R, Tuosto L. Binding of staphylococcal enterotoxin B (SEB) to B7 receptors triggers TCR- and CD28-mediated inflammatory signals in the absence of MHC class II molecules. Front Immunol. 2021;12:16. doi:10.3389/fimmu.2021.723689.
- Truant SN, Redolfi DM, Sarratea MB, Malchiodi EL, Fernández MM. Superantigens, a paradox of the immune response. Toxins. 2022;14(11):30. doi:10.3390/toxins14110800.
- Shaler CR, Choi J, Rudak PT, Memarnejadian A, Szabo PA, Tun-Abraham ME, Rossjohn J, Corbett AJ, McCluskey J, McCormick JK. et al. MAIT cells launch a rapid, robust and distinct hyperinflammatory response to bacterial superantigens and quickly acquire an anergic phenotype that impedes their cognate antimicrobial function: Defining a novel mechanism of superantigen-induced immunopathology and immunosuppression. PLOS Biol. 2017;15(6):35. doi:10.1371/journal.pbio.2001930.
- Lee J, Park N, Park JY, Kaplan BLF, Pruett SB, Park JW, Park YH, Seo KS. Induction of immunosuppressive CD8+CD25+FOXP3+Regulatory T cells by suboptimal stimulation with staphylococcal enterotoxin C1. J Immunol. 2018;200(2):669–80. doi:10.4049/jimmunol.1602109.