ABSTRACT
Tetanus toxin (TeNT) is one of the most toxic proteins. Neutralizing antibodies against TeNT are effective in prevention and treatment. In this study, 14 anti-tetanus nanobodies were obtained from a phage display nanobody library by immunizing a camel with the C-terminal receptor-binding domain of TeNT (TeNT-Hc) as the antigen. After fusion with the human Fc fragment, 11 chimeric heavy-chain antibodies demonstrated nanomolar binding toward TeNT-Hc. The results of toxin neutralization experiments showed that T83–7, T83–8, and T83–13 completely protected mice against 20 × the median lethal dose (LD50) at a low concentration. The neutralizing potency of T83–7, T83–8, and T83–13 against TeNT is 0.4 IU/mg, 0.4 IU/mg and 0.2 IU/mg, respectively. In the prophylactic setting, we found that 5 mg/kg of T83–13 provided the mice with full protection from tetanus, even when they were injected 14 days before exposure to 20 × LD50 TeNT. T83–7 and T83–8 were less effective, being fully protective only when challenged 7 or 10 days before exposure, respectively. In the therapeutic setting, 12 h after exposure to TeNT, 1 ~ 5 mg/kg of T83–7, and T83–8 could provide complete protection for mice against 5 × LD50 TeNT, while 1 mg/kg T83–13 could provide complete protection 24 h after exposure to 5 × LD50 TeNT. Our results suggested that these antibodies represent prophylactic and therapeutic activities against TeNT in a mouse model. The T83–7, T83–8, and T83–13 could form the basis for the subsequent development of drugs to treat TeNT toxicity.
Introduction
Tetanus is an acute, fatal disease caused by the tetanus toxin (TeNT) secreted by Clostridium tetani, which is characterized by generalized rigidity and convulsive spasms of skeletal muscles. Although vaccines made from tetanus toxoids are effective in preventing tetanus, tetanus remains prevalent in low- and middle-income countries.Citation1 In addition, immunization with tetanus vaccine does not provide lifelong protection and requires a booster every 10 years.Citation2 Additionally, the immunization rate has decreased in recent years, especially during the COVID-19 pandemic.Citation3 Even today, tetanus causes thousands of paralytic deaths each year.Citation4 Globally 38,000 people died from tetanus in 2017 and around half (49%) were younger than five years old.Citation5 Treatment of tetanus is mainly divided between active vaccination with formaldehyde inactivated tetanus toxoid vaccines and passive administration of human tetanus immunoglobulin (TIG) or antitoxins containing polyclonal antibodies. However, TIG and tetanus antitoxins have significant limitations: TIG is expensive and cannot destroy completely Clostridium tetani, and tetanus antitoxins tend to cause allergic reactions. Therefore, specific antiTeNT antibodies produced in vitro without immunization would be a better alternative to TIG or antitoxins.Citation6,Citation7
Previous studies have described the isolation of TeNT-neutralizing antibodies derived from rodents or humans.Citation8–14 However, some neutralizing antibodies reported to date are unable to completely neutralize TeNT when used alone.Citation8,Citation15 Meanwhile, a few nanobodies against tetanus toxin have been reported.Citation11 Nanobodies, corresponding to the variable heavy-chain domain of heavy-chain-only IgGs from camelids, can bind to antigens with affinities similar to those of conventional antibodies.Citation16 The molecular mass of a nanobody is only 12–15 kDa, which is the smallest known antibody and easy for engineering modification.Citation17,Citation18 One drawback of nanobodies is that they are quickly cleared by the kidneys due to their small size. In order to overcome the drawback, many studies have fused multiple nanobodies for expression or fused nanobodies with other proteins to increase the molecular weight to a desired level.Citation11,Citation19,Citation20 In the study conducted by de Smit et al., it was found that a single nanobody had weak neutralizing activity against TeNT, but the neutralizing activity was significantly improved when three nanobodies formed a trimer.Citation11 Based on these results, we speculate that fusing nanobodies with the Fc fragment of human antibodies to form chimeric heavy-chain antibodies might prevent rapid clearance by the kidneys and prolong the half-life.Citation20 This strategy might become a very promising approach for the development of tetanus neutralizing antibodies.
Phage display technology, which fuses small molecules such as antibody fragments ScFv or Fab with phage shell proteins and expresses them on the surface of phage particles, is a highly feasible method to obtain antibodies.Citation21 Proper antigen selection is important to screen for specific neutralizing TeNT antibodies from phage-displayed antibody libraries. Tetanus toxin proteins contain an N-terminal light chain (L, ~50 kDa) and a C-terminal heavy chain (H, ~100 kDa), connected by a disulfide bond. The light chain is the active part of the toxin and the heavy chain mediates the entry of the toxin protein into the nerve cells.Citation22 The TeNT heavy chain contains two functional domains: the N-terminal translocation domain (HN, ~50 kDa) and the C-terminal receptor-binding domain (Hc, ~50 kDa). TeNT-Hc can bind to cell receptors in a nontoxic form and induce protective antibodies in animals, making it a potential candidate subunit vaccine to replace tetanus toxoid vaccines.Citation23–25 TeNT-Hc is a protective antigen, and immunization with TeNT-Hc alone can achieve immunity similar to that of the full-length tetanus toxoid.
In this study, a phage display nanobody library was constructed by immunizing a camel with TeNT-Hc as the antigen, and 14 chimeric heavy-chain antibodies that specifically bound to TeNT-Hc were developed after screening. Three antibodies (T83–7, T83–8, and T83–13) that effectively neutralized TeNT have potential for development as prophylactic and therapeutic antibodies.
Materials and methods
Reagents
FreeStyle™ 293 Expression Medium (catalog no. 12338018) was purchased from Thermo Fisher Scientific (Waltham, MA, USA). Goat anti-human IgG H&L (horseradish peroxidase (HRP)) (catalog no. ab6858) was purchased from Abcam (Cambridge, UK). Co-phage M13K07 (catalog no. #N0315S), restriction endonucleases Nco I (catalog no. #R3193), Not I (catalog no. #R3189), and EcoR I (catalog no. #R3101V), were purchased from New England Biolabs (Ipswich, MA, USA). HRP-labeled M13 mouse monoclonal antibody (catalog no. 1973-MM05TH) was purchased from Sino Biological (Beijing, China). Fuchs’ complete adjuvant (catalog no. F5881), Fuchs’ incomplete adjuvant (catalog no. F5506), and ophenylenediamine (OPD) chromogenic substrate (catalog no. P8287-50TAB) were purchased from Sigma-Aldrich (St. Louis, MO, USA). FectoPRO DNA Transfection Reagent (catalog no. 116–001) was purchased from Polyplus-transfection® (Illkirch-Graffenstaden, France). Escherichia coli TG1 competent cells (catalog no. C1170) were purchased from Beijing Solarbio Science & Technology Co. (Beijing, China).
Cell lines and animals
FreeStyleTM HEK293-F cells (Thermo Fisher Scientific) were cultured in FreeStyle 293 Expression Medium to express the antibodies. Female-specific pathogen-free (SPF) KM mice, weighing 18–20 g each, were obtained from Beijing Spefo Biotechnology Co. (Beijing, China). After injection, the mice were observed for 5 days to check for any signs of paralysis or death. All efforts were made to minimize their pain. The mice were monitored daily for their condition and the occurrence of endpoints. The final total number of deaths was determined 5 days after injection. An adult male healthy Bactrian camel was purchased from Deschamps Camel Breeding Co. (Inner Mongolia, China).
Immunization of the camel
The camel was immunized with TeNT-Hc prepared according to a previously described method.Citation26 Briefly, purified TeNT-Hc was mixed with Freud’s complete adjuvant in equal volumes. After full emulsification, the mixture was injected it into a healthy adult Bactrian camel at multiple points, with an interval of 14 days. After the first immunization, Freud’s incomplete adjuvant was used in the rest of the immunizations. After four immunizations, camel serum was collected. An enzyme-linked immunosorbent assay (ELISA) method was used to detect the specific antibody titer against TeNT-Hc in camel serum. The serum before immunization was used as the negative control. After determining that a high antibody titer had been obtained, a fifth immunization was carried out.
Construction of the phage display nanobody library
Seven days after the fifth immunization, whole blood was collected, and lymphocytes were separated using lymphocyte isolation solution. Total RNA was extracted from the lymphocytes and reverse transcribed. After amplification of the nanobody-coding region by two rounds of PCR, the nanobody coding fragment was digested using Nco I and Not I enzymes, and the vector (scFv-NEN) was digested with Nco I, Not I, and EcoR I, and then the fragment and the vector were ligated using T4 DNA ligase. The ligation products were electrotransformed into receptive Escherichia coli TG1 cells, which were plated on 2YT-GA agar plates, and incubated overnight at 37°C in an inverted position. In addition, the electrotransformed TG1 cells were diluted using a 10-fold gradient, plated, and the number of clones was calculated at an appropriate dilution level. Clones were picked and sequenced. Sequencing was performed to determine that the gene fragment of the nanobody was inserted in the phage vector, the integrity of the gene, and the accuracy of the reading frame. The correct insertion rate and the diversity of the library were evaluated based on the sequencing results.
Panning of the TeNT-Hc-specific phage display nanobody library
The TeNT-Hc protein was coated on the immunotube for panning of specific phage clones. The amount of TeNT-Hc used in the three rounds of panning was 20, 10, and 5 μg, respectively. After coating, the immunotube was sealed with blocking solution (phosphate buffered saline (PBS) containing 3% skimmed milk powder) and incubated at room temperature for 2 h. Subsequently, the phage display nanobody library was added to the immunotube and incubated at room temperature for 2 h. After 10 washes with PBS-Tween 20 (PBST) and 5 washes with PBS, bound phage was eluted by adding 1 mL of elution buffer (0.1 M Glycine-HCl, pH 2.2), incubating at 400 rpm for 20 min at room temperature, and then neutralized with neutralization solution (1 M Tris-HCl, pH 8.0). Then, the E. coli TG1 cells grown to the logarithmic phase were infected with the eluted phage and incubated at 37°C for 30 min. After shaking for 30 min at 37°C with a low speed of 150 rpm, the mixture was centrifuged at room temperature (2000 g, 10 min) and the supernatant was discarded. After resuspension in fresh medium, clone counting and preparation of the phage display nanobody library was performed. After three rounds of panning, the single phage colonies were prepared and used for subsequent identification of specific clones. The amount of phage used in each panning represented the input of the round. The number of clones after each panning represented the output of the round. The ratio of the input/output ratio of each round to the input/output ratio of the previous round was used as the enrichment factor for each round.
Identification of TeNT-Hc-specific single positive clones
The TeNT-Hc protein was coated on 96-well ELISA microtiter plates and the irrelevant antigens were coated as negative controls. After blocking with blocking solution (PBS containing 3% skimmed milk), the single phage colonies were added to plates and incubate at 37°C for 1.5 h. The wells were washed six times with PBST, and then 2 µg/mL HRP-labeled M13 mouse monoclonal antibody was added at 100 µL/well of the ELISA plate and incubated at 37°C for 45 min. After washing six times with PBST, the OPD chromogenic substrate was added to develop the color. The reaction was stopped by adding 2 M H2SO4 (50 µL per well) and the absorbance was measured at 492 nm and 630 nm using a microplate reader (Molecular Devices, Sunnyvale, CA, USA). The clones for which the absorption value of the experimental group was more than three times that of the control group were considered as positive clones. The positive clones were sequenced and analyzed to obtain several nanobody sequences specifically targeting TeNT-Hc.
Construction, expression, purification, and identification of the chimeric heavy chain antibodies
The positive clones were used as templates, and primers were designed to amplify the gene fragments of the nanobodies, which were then cloned into expression vector pTSE-hFc, which contains a sequence encoding a human antibody Fc fragment, through the restriction enzyme cleavage sites SalI and NheI. The constructed expression plasmids were sequenced to ensure the correct sequence of the nanobody-hFc fusion protein, which we named chimeric heavy-chain antibodies. The plasmids were transfected into FreeStyleTM HEK293-F cells for expression, separately. The cell culture supernatant was harvested, purified using an AKTA Pure purification system (GE Healthcare, Piscataway, NJ, USA). A HiTrap MabSelect Xtra purification column (Cytiva, Uppsala, Sweden) was used to purify the chimeric heavy-chain antibodies from the cell culture supernatant, which was eluted using citrate solution (100 mM, pH 3.0). Then, the buffer was changed to citrate solution (10 mM, pH 6.0) using a desalting column (Cytiva) to obtain the chimeric heavy-chain antibodies. The molecular weight and purity of the chimeric heavy-chain antibodies were confirmed using sodium dodecyl sulfate-polyacrylamide gel electrophoresis (SDS-PAGE).
Binding assay of the chimeric heavy chain antibodies
The TeNT-Hc and other different antigens were coated onto 96 well plates at 200 ng per well and incubated at 4°C overnight. After blocking with 3% skimmed milk, the candidate antibodies were diluted to 0.0625 μg/mL, added to the 96-well plates, and incubated at 37°C for 1.5 h. After washing with PBST, 100 μL/well of HRP-labeled goat antihuman IgG was added and incubated for 45 min at 37°C. The plate was washed six times with PBST and the OPD chromogenic substrate was added to develop the color. The reaction was stopped with 2 M H2SO4 (50 µL per well), and the absorbance was measured at 492 and 630 nm using a microplate reader (Molecular Devices).
Furthermore, the candidate antibodies were diluted to 0.25 μg/mL and then 2-fold diluted 16 times. The relative binding of the subject antibodies to TeNT-Hc was determined as the effective concentration of the antibodies (EC50) and curve fitting was performed using GraphPad Prism software 8 (version 8.0.2.263, GraphPad Inc., San Diego, CA, USA).
Antibody affinity was determined using the ForteBIO® Octet Qke System (Pall ForteBio Corporation), based on biolayer interferometry (BLI). HBS-EP buffer (Cytiva) was used to dilute the antibodies to 200 nM, followed by fixation on Anti-hIgG Fc Capture biosensors. The biosensors were immersed in HBS-EP for baseline determination, followed by incubation in diluted TeNT-Hc proteins for association, and lastly by a dissociation step. Data Analysis 7.0 software (Pall ForteBio Corporation) was used to analyze the data and plot the kinetic curve.
Neutralization activity of the chimeric heavy chain antibodies against TeNT
The neutralizing effect of chimeric heavy-chain antibodies against the toxin was investigated in mice. The fourteen chimeric heavy-chain antibodies were mixed with 20 × LD50 TeNT at a fixed dose of 10 μg/per mouse and injected intraperitoneally into the mice to initially screen for antibodies with a neutralizing effect on TeNT. Six different doses (10 μg, 5 μg, 2.5 μg, 1.25 μg, 0.625 μg, and 0.3125 μg) of the identified TeNT-neutralizing chimeric heavy-chain antibodies were further tested to determine the minimum dose that completely protected mice from 20 × LD50 TeNT. The antibodies were mixed with 20 × LD50 TeNT toxin, incubated at 37°C for 30 min, and injected intraperitoneally into KM mice (four mice per group). The mice were observed for 5 days to determine if they exhibited symptoms of poisoning, such as limited mobility, muscle stiffness, convulsions, and mortality. The neutralizing ability of anti-TeNT products is estimated in international units (IU), and 1 IU of antitoxin protects mice from 10,000 × LD50 TeNT.Citation26 In our study, the antitoxin potency was calculated as IU/mg, 1 IU/mg means 1 mg antibody neutralize 10,000 × LD50 TeNT.
Prophylactic and therapeutic effects of the chimeric heavy chain antibodies in a mouse model
In the present study, the protective efficacy of the antibodies was investigated using a mouse model.
To investigate the prophylactic effect of the antibodies against TeNT, KM mice (18–22 g) were injected with the antibody (1 or 5 mg/kg) in the experimental group, PBS was injected in the negative control group, A1 (a neutralizing chimeric heavy-chain antibody against BoNT/A), as an irrelevant antibody, was injected in the control group, and TIG was injected in the positive control group. After 12 h, 24 h, 48 h, 3 d, 5 d, 7 d, 10 d and 14 d, the experimental groups of mice (four mice per group) were injected intraperitoneally with different doses of TeNT (20 or 100 × LD50) and continued to be observed for 5 days.
To assess the therapeutic efficacy of the antibodies after exposure to TeNT, KM mice (18–22 g) were injected intraperitoneally with different doses of TeNT (5 or 20 × LD50), and 1, 3, 6, 12, or 24 h later, the experimental group (four mice per group) was injected with antibodies (1 or 5 mg/kg) via the tail vein; the negative control group was injected with PBS, and A1 served as an unrelated control for the chimeric heavy-chain antibodies. The positive control group was injected with TIG.
Statistical analysis
The data from the prophylactic and therapeutic efficacy experiments were analyzed using GraphPad Prism software. The Log-rank test was used to evaluate the significance of the difference in protection compared with the control group. Statistical significance was accepted when the p value of the difference was < .05.
Results
Construction of the phage display nanobody library against TeNT-Hc
A Bactrian camel was immunized with the TeNT-Hc protein as the antigen, and to demonstrate the immunization effect, camel serum was collected before and after immunization and subjected to ELISA analysis. The results showed that antibody titers increased significantly after immunization (). Camel peripheral blood was collected within 1 week after booster immunization. Total peripheral blood mononuclear cells (PBMCs) were isolated to extract total RNA nanobody fragments. Amplified nanobody encoding fragments were ligated into an expression vector and electrotransformed to construct E. coli libraries containing the nanobody fragments. The library capacity of the bacterial library reached 2.3 × 109 colony-forming units (CFU). The titer of the phage library was 4.8 × 1012 cfu/mL.
Panning of TeNT-Hc specific nanobodies
Three rounds of panning were performed using TeNT-Hc as the antigen. In the second round of panning, to obtain clones with better specificity and binding, we reduced the coating concentration of TeNT-Hc from 20 µg to 10 µg, and to 5 µg in the three rounds of panning. As shown in , the ratios of output to input were 3.2 × 10−5, 5.4 × 10−5, and 2.8 × 10−6 in the three rounds of panning, and the enrichment factors were 1.69, and 5.17 in the second and third rounds, respectively. Preliminary phage ELISA identified over 100 positive binders to TeNT-Hc from 240 phage clones, among which 113 were successfully sequenced. The sequences repeated more than twice in the third round of panning were selected for further study. Finally, 14 highly enriched nanobody sequences were selected for subsequent analysis, referred to as VHH-T83-1, 2, 3, 4, 5, 6, 7, 8, 9, 10, 11, 12, 13, 14.
Table 1. TeNT-Hc phage nanobody library panning enrichment analysis.
Construction, expression, and purification of the chimeric heavy chain antibodies
After three rounds of panning, the nanobody coding sequences of the selected positive clones were inserted into the expression vector pTSE-hFc containing the constant region gene of the human Fc fragment to express the chimeric heavy-chain antibodies. The antibodies were purified using the AKTA purification system, and then the molecular weight of the product antibodies were determined using SDS-PAGE. The results showed that the molecular weight of these 14 chimeric heavy-chain antibodies was 80 kDa under non-reducing condition () and 40 kDa under reducing condition (), respectively, which corresponded to the theoretical molecular weight of the chimeric heavy-chain antibody.
Figure 2. Evaluation of the basic properties of the chimeric heavy chain antibodies. (a) Fourteen chimeric heavy chain antibodies analyzed by SDS-PAGE under non-reducing conditions (b) Fourteen chimeric heavy chain antibodies analyzed by SDS-PAGE under reducing conditions. (c) ELISA binding assay of the chimeric heavy chain antibodies to TeNT-Hc proteins. (d) The binding characteristics of the chimeric heavy chain antibodies. The 96-well plates were coated with different antigens (200 ng per well) and incubated with the candidate antibodies. 3% skimmed milk was used as the control. AHc, BHc, EHc, and FHc were the Hc domain of botulinum neurotoxins. TL-HN was another fragment of the full-length tetanus toxin, and 7Fibre was the fiber protein of human adenovirus 7.
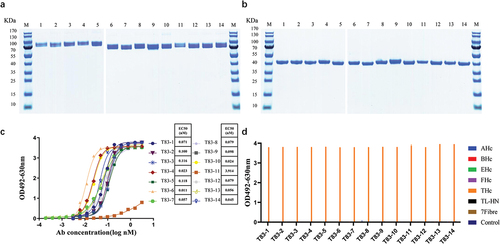
Binding characteristics of the chimeric heavy chain antibodies
The binding of the 14 candidate antibodies to TeNT-Hc were determined using an indirect ELISA. The candidate chimeric heavy-chain antibodies bound to TeNT-Hc in a concentration-dependent manner, and T83–1, 2, 4, 6, 7, 8, 9, 10, 12, 13, and T83–14 showed strong binding, with EC50 values ranging from 0.01 to 0.1 nM (). Additionally, the affinity of the 14 candidate antibodies to TeNT-Hc was measured using BLI (Figure S1). The equilibrium dissociation constant (KD) values for the binding of all the chimeric heavy-chain antibodies to TeNT-Hc were ranged from 5.85 to 87.25 nM (). We also assessed the binding of the 14-candidate chimeric heavy-chain antibodies to other antigens using indirect ELISA. All 14 antibodies were only bound to TeNT-Hc but did not react with the other proteins tested ().
Table 2. The affinity between antibodies and TeNT-Hc determined by bio-layer interferometry.
The toxin neutralization capacity of the chimeric heavy chain antibodies
Mice were used to detect the toxin neutralizing effect of these chimeric heavy-chain antibodies, and TIG was used as a reference control for the ability of the 14 antibodies to neutralize 20 × LD50 TeNT in mice at a dose of 10 μg/injection (). Mice injected with 20 × LD50 TeNT alone had the signs of poisoning, such as limited mobility, muscle stiffness and convulsions, and died within 72 h post-injection. We identified that all 14 antibodies extended the survival time, ranging from a few hours to 5 days (). TeNT could be completely neutralized by T83–7, T83–8, T83–11, and T83–13, as well as by TIG, which served as a positive control, i.e., all mice survived within the 5-day observation period (). Next, we evaluated the protective neutralizing efficacy of these four antibodies against TeNT challenge at doses of 10 µg, 5 µg, 2.5 µg, 1.25 µg, 0.625 µg, and 0.3125 µg. We found that minimal doses of 5 µg of T83–7, T83–13, and 10 µg of T83–8 completely neutralized 20 × LD50 TeNT (), with a neutralizing potency of 0.4 IU/mg for T837 and T83–13, and 0.2 IU/mg for T83–8.
Figure 3. The toxin neutralization capacity of the chimeric heavy chain antibodies. (a) A fixed dose of 10 μg of antibodies and 20 × LD50 TeNT was mixed, followed by intraperitoneal injection into mice, with 4 mice in each group. The time of death and the number of surviving mice were observed once a day, and the percentage of surviving mice was plotted for each antibody. A1 was a neutralizing antibody against BoNT/A and served as an unrelated control. (b) Four neutralizing antibodies at doses of 10 µg, 5 µg, 2.5 µg, 1.25 µg, 0.625 µg, and 0.3125 µg were mixed with 20 × LD50 TeNT and then injected intraperitoneally into mice, with four mice in each group. The time of death and the number of surviving mice were observed once a day, and the percentage of surviving mice was plotted for each antibody. All mice in the negative control group died within 48 h after injection.
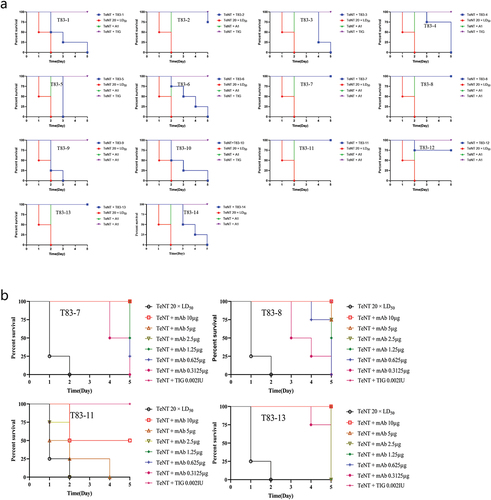
Prophylactic effects of the chimeric heavy chain antibodies against TeNT in a mouse model
Subsequently, we investigated the protective effect of these chimeric heavy-chain antibodies against TeNT and whether this protective effect exhibited a dose-dependent effect in the mouse model. The paralysis symptoms of the mice exposed to TeNT were relieved or delayed in the mice pre-treated with anti-TeNT antibodies, and a significant protective effect was observed (). Pre-treatment with the high dose of antibody (5 mg/kg), T83–7, T83–8, and T83–13 provided the mice with full protection from 20 × LD50 TeNT within 7 days, 10 days, and 14 days, respectively. The low-dose antibody (1 mg/kg), T83–7, T83–8, or T83–13 were less effective, since they were fully protective only when a challenge was given on 3 days, 5 days, or 10 days before exposure, respectively. Mice pre-treated with the irrelevant chimeric heavy-chain antibody A1 were not protected against TeNT. These results suggested that prophylaxis with tetanus antibodies was effective to protect mice against TeNT () and that the protection was dose and pre-treatment time dependent.
Table 3. Prophylactic efficacy of antibodies against TeNT in a mouse model.
Therapeutic effects of the chimeric heavy chain antibodies against TeNT in a mouse model
In this study, we investigated the therapeutic and dose-dependent effects of the chimeric heavy-chain antibodies against TeNT in a mouse model. The mice in the blank control group became paralyzed and died, whereas the mice treated with the antibodies showed delayed onset of paralysis and were protected from the deadly effects of the toxins (). For the high-dose TeNT exposure (20 × LD50), 5 mg/kg of T83–13 treatment was effective up to 12 h after exposure, but not after 24 h. For the low-dose TeNT exposure (5 × LD50), 1 mg/kg of T83–13 treatment was still effective at 24 h after exposure. Early treatment, especially before the mice developed significant paralysis, was most effective in preventing the development of paralysis and protecting toxin-exposed mice. The experimental results showed that the antibodies were effective in treating mice exposed to TeNT (). In summary, we demonstrated that of the chimeric heavy-chain antibodies are effective to treat TeNT exposure in a mouse model and that the therapeutic effect is related to the duration and dose of treatment.
Table 4. Therapeutic efficacy of antibodies against TeNT in a mouse model.
Discussion
Although tetanus can be prevented by vaccination and post-exposure prophylaxis, it remains a major cause of death in some parts of the world where vaccination is limited and is a serious public health problem. Nanobody is a unique form of antibody maintaining a high affinity for the ligand binding site.Citation27 To date, few nanobodies or nanobody multimers against tetanus toxin have been reported.Citation11 Godakova et al. reported VHHs fused to human Fc fragments provide long-term protection against Botulinum Neurotoxin A in mice.Citation28 Herein, we demonstrated that anti-tetanus nanobodies were fused with the Fc fragment of human antibody to form chimeric heavy-chain antibodies, which are effective in both the treatment and prevention of TeNT exposure in a mouse model, and that their protective effect is related to the duration and dose of treatment.
TeNT comprises a light chain (L) and a heavy chain (H), held together by interchain disulfide bonds. The two functional fragments of the heavy chain are Hc, which is required for binding of the toxin to neuronal cells and subsequent endocytosis into vesicles, and HN, which is required for translocation of fragment L across the vesicular membrane into the neuronal cytoplasm.Citation29–36 Studies have identified Hc as a potential target for neutralizing the toxin and for the development of neutralizing antibodies. In this study, TeNT Hc was chosen as the antigen for immunization of one camel. After screening, 14 nanobodies were obtained, which indicated that the Hc of TeNT is an effective immunogen that can produce antibodies that neutralize the toxin. The results also indicated that the mechanism of our antibodies’ neutralizing effects should be by binding to the Hc of TeNT, thereby clearing the toxin from circulation and blocking the binding of TeNT to the receptors on neuronal cell surfaces.
Numerous reports have described either murine or human TeNT neutralizing antibodies.Citation8,Citation37 Some of the neutralizing antibodies, when used alone, did not completely neutralize TeNT, but only prolonged the survival of the mice,Citation8,Citation15,Citation38 unless used in combination or at high doses.Citation15,Citation39,Citation40 The tetanus-neutralizing antibodies 4E12, 3A7, and 4C1, which were developed by Li et al., could completely neutralize 10 × LD50 using 18 μg of antibody (6 μg each) when used in combination.Citation13 The human monoclonal antibodies 8A7, 17F7, 8D8, and 16E8 could completely neutralize 1 × LD50 using 37.5 μg of single antibody.Citation41 By contrast, our antibody used alone at 5 μg was able to completely protect the mice from 20 × LD50 tetanus toxin. 1 mg/kg of our antibody T83–13 could provide fully protective when a challenge of 20 × LD50 tetanus toxin, while TT104, a human monoclonal antibody reported by Pirazzini et al., was more effective than our antibody. TT104 (400 ng/kg) provided the mice with full protection from a challenge of 5 × LD50 of tetanus toxin, even when they were injected 15 days before TeNT inoculation.Citation42 Although the effectiveness of the antibodies in our study was not as good as that of human monoclonal antibodies from Pirazzini et al., it was a novel type of antibody with certain advantages and characteristics, such as smaller molecular weight and ease of engineering modification. Among the tetanus neutralizing antibodies, there are few nanobodies developed so far,Citation11,Citation43 yet nanobodies have great potential for development in the fields of retreatment and diagnosis. Rossotti et al. demonstrated that the neutralizing potency of single-domain antibodies was drastically improved based on their fusion to a second nanobody specific for the complement receptor CD11b/CD18 (Mac-1) and the bispecific construct still has a minimum size (~30 kDa) that favors its rapid biodistribution and is compatible with a high production yield in bacteria.Citation43 Multimers contained either 1 or 2 different TeNT binding VHHs fused to 1 VHH binding to either albumin (A12) or immunoglobulin (G13) to extend serum half-life in animals, increase in affinity compared to multimers containing only one TeNT binding VHH and show a very high TeNT neutralizing capacity.Citation11 The present study developed anti-tetanus neutralizing chimeric heavy-chain antibodies derived of nanobodies using phage display technology, which provides a novel strategy to obtain effective tetanus neutralizing antibodies and lays the foundation for the prevention and treatment of TeNT exposure.
Human mAbs BUT-TT-243-10, BUT-TT-143-10, and BUT-TT-120-10 were identified by sorting memory B cells producing specific anti-tetanus human antibodies by Aliprandini et al.Citation44 The three mAbs bound to different domains of TeNT. When the three mAbs were tested individually, 2.5 μg of mAb could not neutralize the toxin injected in the mice. However, 0.625 μg of the mixture of the three human mAbs could completely neutralize the toxin injected in the mice. This indicated that the combination of antibodies targeting different domains of TeNT could improve the neutralizing activity. Similar results were also found in other studies.Citation41,Citation42 We have also screened candidate antibodies targeting the L-HN fragment of TeNT (data not shown), and we will evaluate the neutralizing activity of the combined antibodies targeting different domains of the tetanus toxin in a future study.
In summary, we identified three protective TeNT-neutralizing chimeric heavy-chain antibodies with high development potential, which are expected to serve as an alternative to TIG or to the equine anti-TeNT antibody currently used in the clinic to prevent and treat tetanus, mainly due to that the antibodies are easier to produce on a large scale and humanized antibodies can effectively reduce the heterogeneity of antibodies and improve safety. In this study, we successfully obtained TeNT-Hc binding nanobodies using phage display libraries technology and developed neutralizing chimeric heavy-chain antibodies against TeNT. These findings provide valuable insights for future TeNT detection and antibody drug development. This technical solution is effective. In the next step, we will prepare neutralizing antibodies targeting the L-HN, L, or HN of TeNT to enrich the source of antibodies and validate the technology. Meanwhile, our results provide the basis for linking antibodies with different epitopes to develop more effective therapeutic antibody drugs.
ARRIVE guidelines
This study is consistent with the ARRIVE guidelines.
Author contribution
Conceived and designed the experiments: WW, YZY, JSL and ZXY. Performed the experiments: KXC, JSL, JZG, LC, XW, YJJ, YTL, CYX, QLK and GQ. Analyzed the data: KXC, YZY, JSL, CG and RW. Contributed reagents/materials/analysis tools: YZX, WW, RW and PD. Wrote the paper: KXC, YZY and JSL. All authors read and approved the final version of the manuscript.
Ethics approval
The Animal Care and Use Committee of the Beijing Institute of Biotechnology approved all the animal experiments (ID: IACUC-DWZX-2019-017). The procedures of all animal experiments were carried out following the institute’s ethical guidelines.
Figure S1.tif
Download TIFF Image (28.7 MB)Supplementary Material.docx
Download MS Word (804.5 KB)Disclosure statement
No potential conflict of interest was reported by the author(s).
Data availability statement
The datasets generated during and/or analyzed during the current study are available from the corresponding author on reasonable request.
Supplementary material
Supplemental data for this article can be accessed on the publisher’s website at https://doi.org/10.1080/21645515.2024.2366641
Additional information
Funding
References
- Thwaites CL, Beeching NJ, Newton CR. Maternal and neonatal tetanus. Lancet. 2015 Jan 24. 385(9965):362–10. doi:10.1016/S0140-6736(14)60236-1.
- Mizuno Y, Yamamoto A, Komiya T, Takeshita N, Takahashi M. Seroprevalence of tetanus toxoid antibody and booster vaccination efficacy in Japanese travelers. J Infect Chemother. 2014 Jan. 20(1):35–7. doi:10.1016/j.jiac.2013.11.003.
- Lamberto Y, Vargas C, Sanchez Cunto M, Saul P, Chediack V, Cunto E. Tetanus: an immunopreventable disease. Medicina. 2023;83(5):841–5. Tetanos: una enfermedad inmunoprevenible.
- World Health Organization. Total tetanus: reported cases by WHO region. [accessed 2024 Jan 10].https://apps.who.int/gho/data/view.main.1520_46?lang=en.
- Behrens H, Ochmann S, Dadonaite B, Roser M. Tetanus. 2019. [accessed 2024 Jan 5]. https://ourworldindata.org/tetanus
- Goulon M, Girard O, Grosbuis S, Desormeau JP, Capponi MF. Changes in anti-tetanus antibodies under sero-toxoid therapy. Study in 50 patients with tetanus. Nouv Presse Med. 1976 27;5(13):847–50. Evolution sours sero-anatoxinotherapie des anticorps anti-tetaniques. Etude chez 50 tetaniques.
- Gibson RB, Banzhaf EJ. The quantitative changes in the proteins in the blood plasma of horses in the course of immunization. J Exp Med. 1910 May 1;12(3):411–34. doi:10.1084/jem.12.3.411.
- Wang H, Yu R, Fang T, Yu T, Chi X, Zhang X, Liu S, Fu L, Yu C, Chen W. Tetanus neurotoxin neutralizing antibodies screened from a human immune scfv antibody phage display library. Toxins. 2016 Sep 11;8(9):266. doi:10.3390/toxins8090266.
- Fitzsimmons SP, Clark KC, Wilkerson R, Shapiro MA. Inhibition of tetanus toxin fragment C binding to ganglioside G(T1b) by monoclonal antibodies recognizing different epitopes. Vaccine. 2000 Aug 15;19(1):114–21. doi:10.1016/s0264-410x(00)00115-8.
- Scott N, Qazi O, Wright MJ, Fairweather NF, Deonarain MP. Characterisation of a panel of anti-tetanus toxin single-chain Fvs reveals cooperative binding. Mol Immunol. 2010 Jun;47(10):1931–41. doi:10.1016/j.molimm.2010.02.020.
- de Smit H, Ackerschott B, Tierney R, Stickings P, Harmsen MM. A novel single-domain antibody multimer that potently neutralizes tetanus neurotoxin. Vaccine: X. 2021 Aug;8:100099. doi:10.1016/j.jvacx.2021.100099.
- Ghotloo S, Golsaz-Shirazi F, Amiri MM, Jeddi-Tehrani M, Shokri F. Neutralization of tetanus toxin by a novel chimeric monoclonal antibody. Toxicon: Official J Int Soc Toxinol. 2021 Oct 15;201:27–36. doi:10.1016/j.toxicon.2021.08.011.
- Li Y, Chen Y, Cui J, Liu D, Zhang W, Xue C, Xiong X, Liu G, Chen H. Preparation and characterization of a neutralizing murine monoclonal antibody against tetanus toxin. J Immunol Methods. 2023 Feb;513:113427. doi:10.1016/j.jim.2023.113427.
- Nejad HR, Mehrabadi JF, Saeedi P, Zanganeh S. Phage display technology for fabricating a recombinant monoclonal ScFv antibody against tetanus toxin. Toxicol Res (Camb). 2023 Aug;12(4):591–8. doi:10.1093/toxres/tfad050.
- Yousefi M, Younesi V, Bayat AA, Jadidi-Niaragh F, Abbasi E, Razavi A, Khosravi-Eghbal R, Asgarian-Omran H, Shokri F. Comparative human and mouse antibody responses against tetanus toxin at clonal level. J Immunotoxicol. 2016;13(2):243–8. doi:10.3109/1547691X.2015.1046572.
- Konning D, Zielonka S, Grzeschik J, Empting M, Valldorf B, Krah S, Schröter C, Sellmann C, Hock B, Kolmar H, et al. Camelid and shark single domain antibodies: structural features and therapeutic potential. Curr Opin Struct Biol. 2017 Aug;45:10–16. doi:10.1016/j.sbi.2016.10.019.
- Muyldermans S. Nanobodies: natural single-domain antibodies. Annu Rev Biochem. 2013;82(1):775–97. doi:10.1146/annurev-biochem-063011-092449.
- Steeland S, Vandenbroucke RE, Libert C. Nanobodies as therapeutics: big opportunities for small antibodies. Drug Discov Today. 2016 Jul;21(7):1076–113. doi:10.1016/j.drudis.2016.04.003.
- Fu Y, da Fonseca Rezende EMJ, Fleming BD, Renn A, Chen CZ, Hu X, Xu M, Gorshkov K, Hanson Q, Zheng W, et al. A humanized nanobody phage display library yields potent binders of SARS CoV-2 spike. PLOS ONE. 2022;17(8):e0272364. doi:10.1371/journal.pone.0272364.
- Ye G, Gallant JP, Massey C, Shi K, Tai W, Zheng J, Odle AE, Vickers MA, Shang J, Wan Y, et al. The development of a novel nanobody therapeutic for SARS-CoV-2. bioRxiv : Preprint Server Biol. 2020 Nov 17. doi:10.1101/2020.11.17.386532.
- Yu R, Wang S, Yu YZ, Du W-S, Yang F, Yu W-Y, Sun Z-W. Neutralizing antibodies of botulinum neurotoxin serotype a screened from a fully synthetic human antibody phage display library. J Biomol Screen. 2009 Sep;14(8):991–8. doi:10.1177/1087057109343206.
- Turton K, Chaddock JA, Acharya KR. Botulinum and tetanus neurotoxins: structure, function and therapeutic utility. Trends Biochem Sci. 2002 Nov;27(11):552–8. doi:10.1016/s0968-0004(02)02177-1.
- Anderson R, Gao XM, Papakonstantinopoulou A, Roberts M, Dougan G. Immune response in mice following immunization with DNA encoding fragment C of tetanus toxin. Infect Immun. 1996 Aug;64(8):3168–73. doi:10.1128/iai.64.8.3168-3173.1996.
- Fairweather NF, Chatfield SN, Makoff AJ, Strugnell RA, Bester J, Maskell DJ, Dougan G. Oral vaccination of mice against tetanus by use of a live attenuated Salmonella carrier. Infect Immun. 1990 May;58(5):1323–6. doi:10.1128/iai.58.5.1323-1326.1990.
- Tregoning JS, Nixon P, Kuroda H, Svab Z, Clare S, Bowe F, Fairweather N, Ytterberg J, Wijk, KJ, Dougan G, et al. Expression of tetanus toxin fragment C in tobacco chloroplasts. Nucleic Acids Res. 2003;31(4):1174–9. doi:10.1093/nar/gkg221. 15.
- Liu FJ, Shi DY, Li ZY, Lu J-S, Wang R, Pang X-B, Yang Z-X, Yu Y-Z. Evaluation of a recombinant tetanus toxin subunit vaccine. Toxicon: Official J Int Soc Toxinol. 2020 Nov;187:75–81. doi:10.1016/j.toxicon.2020.08.001.
- Wesolowski J, Alzogaray V, Reyelt J, Unger M, Juarez K, Urrutia M, Cauerhff A, Danquah W, Rissiek B, Scheuplein F, et al. Single domain antibodies: promising experimental and therapeutic tools in infection and immunity. Med Microbiol Immunol. 2009 Aug;198(3):157–74. doi:10.1007/s00430-009-0116-7.
- Godakova SA, Noskov AN, Vinogradova ID, Ugriumova, GA, Solovyev, AI, Esmagambetov, IB, Tukhvatulin, AI, Logunov, DY, Naroditsky, BS, Shcheblyakov, DV, et al. Camelid VHHs fused to human fc fragments provide long term protection against botulinum neurotoxin a in mice. Toxins. 2019 Aug 7;11(8):464. doi:10.3390/toxins11080464.
- Rossetto O, Montecucco C. Tables of toxicity of botulinum and tetanus neurotoxins. Toxins. 2019 Nov 22;11(12):686. doi:10.3390/toxins11120686.
- Ghotloo S, Golsaz-Shirazi F, Amiri MM, Jeddi-Tehrani M, Shokri F. Epitope mapping of tetanus toxin by monoclonal antibodies: implication for immunotherapy and vaccine design. Neurotox Res. 2020 Feb;37(2):239–49. doi:10.1007/s12640-019-00096-w.
- Chen C, Fu Z, Kim JJ, Barbieri JT, Baldwin MR. Gangliosides as high affinity receptors for tetanus neurotoxin. J Biol Chem. 2009 Sep 25;284(39):26569–77. doi:10.1074/jbc.M109.027391.
- Schiavo G, Papini E, Genna G, Montecucco C. An intact interchain disulfide bond is required for the neurotoxicity of tetanus toxin. Infect Immun. 1990 Dec;58(12):4136–41. doi:10.1128/iai.58.12.4136-4141.1990.
- Restani L, Novelli E, Bottari D, Leone P, Barone I, Galli‐Resta L, Strettoi E, Caleo M. Botulinum neurotoxin a impairs neurotransmission following retrograde transynaptic transport. Traffic. 2012 Aug; 13(8):1083–9. doi:10.1111/j.1600-0854.2012.01369.x.
- Pirazzini M, Azarnia Tehran D, Zanetti G, Megighian A, Scorzeto M, Fillo S, Shone C, Binz T, Rossetto O, Lista F, et al. Thioredoxin and its reductase are present on synaptic vesicles, and their inhibition prevents the paralysis induced by botulinum neurotoxins. Cell Rep. 2014 Sep 25;8(6):1870–8. doi:10.1016/j.celrep.2014.08.017.
- Pirazzini M, Azarnia Tehran D, Zanetti G, Lista F, Binz T, Shone CC, Rossetto O, Montecucco C. The thioredoxin reductase–Thioredoxin redox system cleaves the interchain disulphide bond of botulinum neurotoxins on the cytosolic surface of synaptic vesicles. Toxicon: Official J Int Soc Toxinol. 2015 Dec 1;107(Pt A):32–6. doi:10.1016/j.toxicon.2015.06.019.
- Pirazzini M, Azarnia Tehran D, Leka O, Zanetti G, Rossetto O, Montecucco C. On the translocation of botulinum and tetanus neurotoxins across the membrane of acidic intracellular compartments. Biochim Biophys Acta (BBA) - Biomembranes. 2016 Mar;1858(3):467–74. doi:10.1016/j.bbamem.2015.08.014.
- Wang Y, Wu C, Yu J, Lin S, Liu T, Zan L, Li N, Hong P, Wang X, Jia Z. et al. Structural basis of tetanus toxin neutralization by native human monoclonal antibodies. Cell Rep. 2021 May 4;35(5):109070. doi:10.1016/j.celrep.2021.109070.
- Sadreddini S, Seifi-Najmi M, Ghasemi B, Kafil HS, Alinejad V, Sadreddini S, Younesi V, Jadidi-Niaragh F, Yousefi M. Design and construction of immune phage antibody library against Tetanus neurotoxin: production of single chain antibody fragments. Hum Antibodies. 2015 Dec 23;23(3–4):73–9. doi:10.3233/HAB-150287.
- Arunachalam B, Ghosh S, Talwar GP, Raghupathy R. A single human monoclonal antibody that confers total protection from tetanus. Hybridoma. 1992 Apr;11(2):165–79. doi:10.1089/hyb.1992.11.165.
- Chin J, Sohn Y, Lee SH, Park YI, Choi MJ. Production of neutralizing human monoclonal antibody directed to tetanus toxin in CHO cell. Biol: J Int Assoc Biol Standardization. 2003 Mar;31(1):45–53. doi:10.1016/s1045-1056(02)00092-1.
- Minamitani T, Kiyose K, Otsubo R, Ito T, Akiba H, Furuta RA, Inoue T, Tsumoto K, Satake M, Yasui T, et al. Novel neutralizing human monoclonal antibodies against tetanus neurotoxin. Sci Rep. 2021 June 9;11(1):12134. doi:10.1038/s41598-021-91597-2.
- Pirazzini M, Grinzato A, Corti D, Barbieri S, Leka O, Vallese F, Tonellato M, Silacci-Fregni C, Piccoli L, Kandiah E, et al. Exceptionally potent human monoclonal antibodies are effective for prophylaxis and treatment of tetanus in mice. J Clin Invest. 2021 Nov 15;131(22). doi:10.1172/JCI151676.
- Rossotti MA, Gonzalez-Techera A, Guarnaschelli J, Yim L, Camacho X, Fernández M, Cabral P, Leizagoyen C, Chabalgoity JA, González-Sapienza G. et al. Increasing the potency of neutralizing single-domain antibodies by functionalization with a CD11b/CD18 binding domain. Mabs-austin. 2015;7(5):820–8. doi:10.1080/19420862.2015.1068491.
- Aliprandini E, Takata DY, Lepique A, Kalil J, Boscardin SB, Moro AM. An oligoclonal combination of human monoclonal antibodies able to neutralize tetanus toxin in vivo. Toxicon: X. 2019 Apr;2:100006. doi:10.1016/j.toxcx.2019.100006.