ABSTRACT
The cumulative weight of the evidence demonstrates the safety and equivalence of genetically engineered (GE) crops compared to the conventional varieties from which they have been derived. Confirmatory toxicology and animal nutrition studies have nevertheless become an expected/mandated component of GE crop safety assessments, despite the lack of additional value these studies provide for product safety assessment. Characterization and safety data (e.g. trait protein safety; molecular, compositional, and agronomic/phenotypic assessments), and animal feeding studies form a weight of the evidence supporting the safety of insect-protected maize MON 810. Independent animal testing has recently confirmed the lack of MON 810 toxicity in subchronic and chronic toxicity studies. These results could have been predicted from the available safety data. Animal testing of GE crops should be supported by testable scientific hypotheses and testing should be consistent with ethical obligations to reduce, refine, and replace (3Rs) animal testing when possible.
Introduction
Genetically engineered (GE) crops have played an important role in global agriculture since their commercial introduction in the mid-1990s. GE crops are developed through the introduction of a gene or genes that confer to the plant a trait, i.e., a desirable characteristic such as agronomic, phenotypic, or nutritional enhancement. To date, GE crops have been cumulatively planted on over 2.5 billion hectares and have led to substantial agricultural productivity gains.Citation1 In 2018, GE crops were planted in 26 different countries of which 21 were developing countries and 5 were industrial countries. The United States, which represents 39% of the total global acreage, has the largest GE crop footprint, but there is also considerable planting of GE crops outside the US with Brazil, Argentina, Canada and India representing an additional 52% of the global acreage. The economic benefits of GE crops are represented by an additional gain of $186.1 billion US dollars from 1996 to 2016.Citation1 Environmental benefits include: CO2 reduction impact (27.1 billion kg in 2016 alone) due to reduced fuel use from less insecticide/herbicide spraying and reduced plowing through low/no-till agricultural production methods,Citation2 and a reduction of pesticide use (insecticides and in some cases, herbicides) by 671 million kg of active ingredients during the period from 1996 to 2016.Citation1 In addition, GE crops are associated with reduced pesticide poisonings in developing countries when insect-resistant varieties of GE cotton are used instead of broad-spectrum chemical insecticides.Citation3–Citation5
The most broadly available GE crops are those tolerant to herbicides and those protected against insect damage. For example, YieldGard (MON 810) maize is resistant to the European Corn Borer insect (Ostrinia nubilalis) and other lepidopteran insect pests, a trait conferred through the introduction of a gene encoding the insecticidal protein Cry1Ab, derived from the soil-dwelling bacterium, Bacillus thuringiensis (Bt), subspecies kurstaki (Btk). Prior to the introduction of Cry1Ab into GE crops, strains of Btk [expressing Cry1Ab] had been used in agriculture since the early 1960s as topical sprays for insect control,Citation6,Citation7 supporting the history of safe use of this protein and safety of any residues associated with the use of products containing this protein. Before the first GE crops were ever placed into commerce, consideration was given to the potential for unique risks that might arise in these crops or their derived food and feed products, and a framework for the safety assessment of these (at the time) novel crops was developed. For GE crops, the potential for novel impacts of both the gene insertion process and the introduced gene product(s) was explicitly considered prior to the commercialization of the technology.Citation8 It is important to note that this evaluation placed GE plants into the overall context of plant breeding. All breeding techniques, including conventional (i.e. non-GE) breeding, are targeted toward the introduction of desirable traits, and all have a potential to introduce unexpected effects. In practical terms, the impact of any unexpected effects in both GE and conventional plant breeding (e.g. traditional crossing and/or leveraging of mutagenesis) is minimized through plant selection processes.Citation9 These considerations are reflected in the assessment paradigm that has been globally adopted for the safety assessment of GE crops, as described below.
The Conceptual Framework for GE Crop Safety Assessment
Other than the introduction of a trait and the intended changes it confers, the process of developing a GE plant through the insertion of a transgene does not inherently lead to a plant that is substantially different from its non-engineered counterparts.Citation10–Citation12 However, due to the untargeted insertion of the gene and potential impacts of the expressed protein on the plant, there is a potential to introduce unintended characteristics that are not native to the plant species. Consequently, characterization and safety evaluations of GE crops have been included as an integral component of their path to the marketplace since the introduction of the technology. It is important to note that traditional plant breeding can result in the introduction of additional genes or alleles and novel gene combinations, and that notable changes in nature can occur within plants due to crossing with wild relatives, gene transfer across phylogenetic boundaries, the activity of transposable elements, and through naturally occurring genetic rearrangements.Citation9,Citation12–Citation15 Therefore, considering natural variation and the potential impacts of breeding noted above, the assessment of GE crops should be considered within the context of the potential for unexpected novel phenotypes to occur in nature or through plant breeding. However, the advent of GE crops triggered an assessment framework that generates vastly more characterization data for these products than that performed on their conventionally bred counterparts, including products derived from chemical or radiation-induced mutagenesis. Based on the weight of the evidence to date, the data collected in this assessment framework demonstrate the absence of unexpected effects of genetic engineering and illustrate the substantial equivalence of GE crops to their conventional counterparts.
The framework for the safety evaluation of GE crops was developed to enable hazard identification and risk assessment for these crops. This framework recognized that traditional foods used in traditional ways have a history of safe use, despite the fact that some foods contain naturally occurring toxic components,Citation16 see Table 1. Thus, for assessment of the GE crop, a comparative safety assessment paradigm was adopted by the Codex Alimentarius Commission (CAC) of the Joint FAO/WHO Food Standards Programme. Within this framework, a comparison is made between the GE crop and a conventional non-GE comparator, to determine similarities and differences in key components relevant to the safety and nutrition of the crop.Citation17–Citation19 provides a graphical depiction of this comparative assessment process. For any identified differences, further evaluation focuses on the nature of those differences and the evaluation of any potential hazards and/or risks they could potentially pose for consumers. Recall that non-GE plants naturally contain hazardous substances,Citation16 see , but are not considered to present unacceptable risks to consumers. In addition to the comparative assessment, characterization focused on the introduced gene and gene product is conducted to directly address the safety of newly introduced gene products, and to confirm insertion of the expected gene, to provide information about its copy number and stability, and to evaluate the insertion location.
Table 1. Toxic substances present in safely consumed foods.Citation16.
Figure 1. A rigorous, integrated process to assess the safety of GE crops/food.
Figure content is adapted from the guidance provided by the Codex Alimentarius Commission of the UN Food and Agriculture Organization.Citation17–Citation19
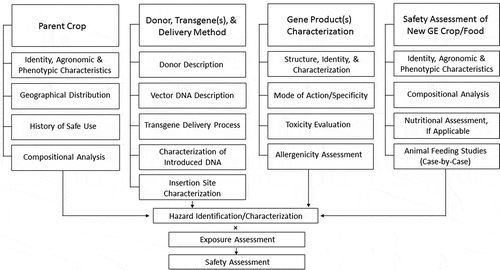
Although the CAC guidance and other guidance documents described the comparative assessment approach for the safety evaluation of GE crops, the regulatory process for hazard identification and risk assessment of GE crops has evolved based in part on considerations relevant to other substances in food and agricultural production, e.g. food additives or agricultural chemicals. Unlike GE crops, food additives and agricultural chemicals tend to be synthetic small molecules, and there is often little known about potential hazards during early product development. As a result, an extensive testing regimen is therefore conducted that includes numerous toxicological studies to enable the establishment of a hazard profile. However, this approach is not well suited to whole foods that cannot be added to the diet at high multiples of potential human exposures as such levels would present a nutritional imbalance and because food intake is limited. Furthermore, these whole foods are readily broken down in the digestive tract and absorbed in the form of their naturally-occurring constituent components (e.g. nutrients, amino acids, nucleic acids). Due to robustness of the comparative assessment, as well as the lack of suitability of animal studies for testing whole foods, an extensive battery of toxicology studies was determined to be unnecessary for demonstrating the safety of GE crops and therefore not included as a component of the safety assessment paradigm articulated in CAC guidance documents. Rather, as indicated, a demonstration of the safety of the introduced gene product, of the overall lack of disruptive impact of transgene insertion, and of the similarity of the nutritional characteristics to those of non-GE comparators provides an indication that the GE crop is as safe as conventional varieties of the crop. In spite of this regulatory guidance and other similar guidance,Citation20,Citation21 publications in the peer-reviewed literature,Citation22 and the imperfect scientific fit for purpose; feeding studies have frequently been conducted on GE crops, nominally to provide confirmatory support for the safety conclusion arising from the weight of evidence assessment of the characterization and comparative safety data for the GE crop.
Here we review what is necessary to evaluate the safety of a GE crop, and put this in the context of safety assessment of the insect-resistant maize product MON 810, one of the first GE crops placed in commercial use. This review demonstrates that the hazard identification principles now codified within the CAC guidance documentCitation18 are indeed sufficient for determining whether a GE crop is as safe as its conventional comparator. MON 810 was selected for this evaluation because considerable testing has been performed on this product by the technology developer, by academic laboratories and government agencies, and because MON 810 has also been tested in independent long term feeding studies conducted in the EU.Citation23,Citation24 As many feeding studies have been conducted for MON 810, this review considers the context for these studies, the results of the original and repeated whole food feeding studies conducted for this product and examines the necessity of feeding study data for determining whether MON 810 is as safe as its conventional comparator.
Weight of Evidence Assessment, Mon 810 Maize
As previously described in this paper, MON 810 maize has been genetically engineered to contain and express a gene encoding the Cry1Ab insecticidal crystal (Cry) protein (δ-endotoxin) to protect the plant from damage due to targeted insect pests.
MON 810 was characterized within the framework of the GE crop safety assessment paradigm, as outlined in CAC guidance documents and in other guidance documentsCitation8,Citation17,Citation18,Citation19,Citation25,Citation26 with the caveat that these assessments utilized available published and draft guidance in place at the time they were conducted. The aspects specified in these guidance documents that are directly related to MON 810 and support the conclusions that MON 810 is as safe as conventional maize varieties are discussed briefly below and have been provided in more detail elsewhere.Citation27,Citation28
MON 810 Characterization:
Description of the Donor Organism
The source organism of the cry1Ab gene in MON 810 is Bt (subspecies kurstaki), a soil bacterium that has been used safely in biopesticide applications for half a century to provide pest control in agriculture, including organic agricultural production.Citation29–Citation31
Description of the Genetic Modification
MON 810 was produced by microprojectile bombardment of maize embryonic tissue with a plasmid containing the cry1Ab gene.Citation27 Following this transgene insertion, embryonic maize tissue expressing the construct is grown through tissue culture to produce multiple “transgenic events” (e.g. thousands). These plants are then thoroughly characterized and evaluated for molecular, agronomic, and phenotypic characteristics that enable identification of which transgenic events to move forward in the product development process. This process ultimately led to the selection of one event, MON 810, for regulatory testing.
Characterization of the Genetic Modification
Southern blot analysis of MON 810 demonstrated that a single copy of the cry1Ab gene was integrated into the genome. It was further demonstrated that the introduced gene was inherited in an expected Mendelian pattern over successive generations, indicating stable integration.Citation27 MON 810 expresses a truncated (trypsin-resistant core or tryptic core) version of the Cry1Ab protein toxin rather than the full-length version expressed in Bt; however, the active site is retained.Citation28 The tryptic core of Cry1Ab is the insecticidally active form of the proteinCitation32 as further evidenced by the activity of the MON 810 expressed Cry1Ab protein against targeted insect pests. Therefore, further distinction is not made in this manuscript between the MON 810 produced Cry1Ab tryptic core protein and that of the full-length Cry1Ab toxin produced by Bt as both proteins are insecticidally active against the targeted insect pests. Both proteins are therefore interchangeably referred to simply as “Cry1Ab”. Furthermore, data were generated to compare the truncated tryptic core of Cry1Ab produced in the plant to that produced in E. coli to demonstrate the appropriateness of the bacterially produced protein used in the protein safety studies as a suitable analogue to that produced in the plant (e.g. comparable molecular weight, amino acid sequence, immunoreactivity, insecticidal activity, etc.).Citation27,Citation28 Therefore, the use of this surrogate protein is not further addressed in this manuscript.
The expression level of Cry1Ab protein in MON 810 was quantified to enable the evaluation of potential exposures to this protein for consumers of the harvested products. The expression level of Cry1Ab protein in MON 810 was approximately 3–5 µg/g fresh weight in forage and approximately 0.3–0.5 µg/g fresh weight in harvested grain.Citation27 For context, maize grain is approximately 7.8% protein (or 78 mg of protein/g of grain), and glutelin makes up approximately 40% (or 31.2 mg of glutelin/g of grain) of the protein in maize grain.Citation33
Safety Assessment:
Expressed Substances
Bt protein safety and mode of action have been reviewed elsewhere in the scientific literature.Citation30,Citation31
The mode of action of Cry1Ab and related Bt endotoxins is well understood,Citation34–Citation37 Briefly, the ingested toxin is cleaved in the alkaline insect gut and binds to the cadherin-like receptor protein Bt-R1 (Accession CAC41165.1)Citation38,Citation39 leading to oligomerization of the toxin and subsequent binding to aminopeptidase N in the insect.Citation34,Citation39 Specific binding to these two receptors leads to pore formation and disruption of ion flow in the insect midgut resulting in cell swelling and lysis. This results in insecticidal activity of Cry1Ab only in lepidopteran insects that express the target receptors. Unlike insects, humans and other mammals have an acidic gut, and invitro simulated gastric fluid assays indicate that these conditions trigger degradation and loss of insecticidal activity of the Cry1Ab protein.Citation27 Based on a lack of binding to the rat GI tract following oral administration of Cry1Ab and the lack of specific Cry1Ab binding to intestinal tissues from rats, mice, rhesus monkeys, and humans, mammals appear to lack high-affinity receptors for Bt proteins.Citation40 This is consistent with bioinformatic screens that show Bt-R1 truncated alignments with identity levels in the mid to low 20% range () when compared to mammalian, human, and known human intestinally expressed cadherin-like proteins (CAD17, Accession Q12864.3).Citation42 Collectively, the acidic environment of the mammalian GI tract and the absence of Cry1Ab receptors make these toxins ineffective in humans and other mammals. Based on the safe use of Cry1Ab and other three-domain Cry proteins since the 1920s as topical biopesticides (consisting of Bt spores and crystalline insecticidal proteins) and the above weight of the scientific evidence, the favorable results of the safety studies for Cry1Ab and MON 810 outlined in this paper is not surprising.
Table 2. Bioinformatics search for Bt-R1 (Bt toxin receptor) homologs in humans and mammals.
Bioinformatic assessment of the Cry1Ab amino acid sequence confirmed homology with other Cry1 proteins and demonstrated a lack of similarity of the Cry1Ab protein to known mammalian toxins or to known allergens.Citation6,Citation27,Citation43
Cry1Ab protein (in its insecticidal tryptic core form) was evaluated in an in vitro digestion assay and found to be rapidly degraded under simulated gastric digestion conditions and was also found to be heat-labile.Citation6,Citation27,Citation43 These findings indicate that systemic exposure to intact, active Cry1Ab protein following the ingestion of foods derived from MON 810 would be negligible. Furthermore, such exposure may not even occur given that dietary proteins are mainly absorbed from the intestines as free amino acids and peptides ≤3 amino acids in length.Citation44–Citation46 In addition to toxicological potential, the aforementioned bioinformatics, heat stability, and digestibility data can be leveraged as a weight of the evidence to address potential allergenicity of Cry1Ab. In addition, Cry1Ab is from a non-allergenic source organism and constitutes a very small portion of the total protein present in the grain of MON 810. This evidence collectively indicates that Cry1Ab is not likely to have any allergenic potential. Lack of immunogenic, allergenic, or adjuvant capacity of Cry1Ab (trypsin-activated toxin) was indicated in data generated from a mouse food allergy model.Citation47 The absence of allergenicity of MON 810 and Cry1Ab is further confirmed by human clinical data in sensitive populations (children with allergies and individuals with asthma) in the form of skin prick testing and testing for IgE antibodies against Cry1Ab.Citation48 Such animal testing and human testing provide confirmatory data for MON 810 and Cry1Ab and yet, these data are not necessary for the evaluation of allergenic potential of proteins in GE crops, as these data can be predicted from the weight of evidence generated during product safety evaluation as described above.
Based upon the history of safe use and empirical safety data concerning Bt-derived proteins within biopesticide products, safety testing has been conducted on a number of individual Cry proteins, including Cry1Ab.Citation6 Since insecticidal Cry proteins act through acute mode of action in controlling insect pests, the potential human health hazards of these Cry proteins can be assessed through acute oral toxicological assessments.Citation28 Cry proteins generally have been shown to be nontoxic to mammals in oral toxicity tests at high dosesCitation31,Citation49 such as those exceeding 1000 mg/kg body weight. Cry1Ab (in its insecticidal tryptic core form) was not toxic at oral doses of up to 4000 mg/kg body weight in mice.Citation28,Citation49 Such doses typically represent very large margins of exposure relative to their anticipated human exposures following consumption of GE crops (e.g. on the order of thousands to hundreds of thousands or higher). The weight of the scientific evidence demonstrates that both biopesticides from Bt and their component proteins, including Cry1Ab, are not toxic to mammals.Citation6,Citation31,Citation43,Citation50 Thus, the established history of safe use and the lack of mammalian toxicity demonstrate the safety of Cry1Ab in MON 810 for consumption in human food and livestock feed.
Compositional Analysis of Key Components
A comparative assessment of the nutritional composition of the grain and forage of MON 810 relative to an appropriate conventional comparator was conducted. The purpose of the assessment was to determine if the grain and forage of MON 810 were compositionally equivalent to the comparator variety, and therefore provide evidence regarding whether there are any differences that have the potential to impact the safety of MON 810 relative to conventional maize. The compositional assessment of grain was conducted in a manner consistent with international guidance from the OECD and included measuring the levels of proximates (protein, fat, ash, crude fiber, carbohydrates (by difference), and moisture), amino acids, fatty acids, and the minerals calcium and phosphorus. For forage, proximates were similarly assessed. The conclusion of the compositional assessment was that MON 810 is compositionally equivalent to conventional maize.Citation27 These results for MON 810 are typical of those that have been observed for many other GE crops, as reviewed by Herman and Price.Citation11 Those authors concluded that notable unintended effects on nutritional composition due to genetic modification processes have not been observed.
Agronomic and Phenotypic Assessments
In addition to the food/feed safety assessment, agronomic parameters of MON 810 were assessed relative to its conventional counterpart in agronomic field trials, and no unexpected impacts on the plant from the genetic modification were observed.Citation51 Agronomic and phenotypic evaluations demonstrated that MON 810 grew normally and exhibited the expected physiological properties for maize.Citation6,Citation51 These outcomes were as expected given the nature of the introduced trait and given the product screening (i.e., plant selection for product advancement selects against off-types) that occurs during crop development prior to selecting a line for commercialization.Citation9 In addition, evaluation of the insect activity spectrum of Cry1Ab was conducted, and the environmental safety of this protein including potential risks to non-target organisms has been thoroughly assessed based on both laboratory and field experiments.Citation27,Citation51
Feeding Trials for Nutritional Equivalence
As noted above, the assessment of MON 810 was characterized within the framework of the internationally recognized GE crop safety assessment paradigm in place at the time these assessments were conducted.Citation25,Citation26,Citation8 These evaluations support the conclusion that MON 810 is as safe as conventional maize varieties.Citation27,Citation28
MON 810 was one of the first insect-protected GE crops and, therefore, it was considered at the time that it would be helpful to have additional data to complement the existing agronomic, compositional, and other data that demonstrated the safety and nutrition of grain from MON 810. Nutritional equivalence was empirically demonstrated through the compositional equivalence of MON 810 to its non-GE comparator(s). Nevertheless, nutritional equivalence studies have also been performed to demonstrate the wholesomeness of the GE crop relative to its non-GE comparator. These studies have included nutritional equivalence studies in livestock as well as repeated-dose toxicity studies in rodents. Some of these studies were conducted and published by the product developer, to support the equivalence of this grain to enable its use in animal feed, to build further on the weight of the scientific evidence for product safety, and to facilitate global regulatory evaluations and approvals for MON 810. Due to heightened interest in this product as one of the first widely cultivated GE crops, numerous additional studies have also been conducted by independent third parties. As this technology was new at the time of the introduction of MON 810, the early confirmatory studies with this product provided further confirmation of product safety to consumers, policymakers, and regulatory decision-makers. Considering the weight of the evidence amassed in evaluating GE crop safety to date, these types of feeding studies should be conducted only when hypothesis-based and should not be conducted on a routine basis as a default assessment. Nutritional feeding studies with MON 810 have been conducted using a wide variety of species, including broiler chickens,Citation52–Citation54 dairy cattle,Citation55,Citation56 pigs,Citation57,Citation58 and Japanese quail.Citation59,Citation60 These studies are summarized below and consistently demonstrate that MON 810 is nutritionally equivalent to its conventional counterpart (Supplemental Table 1).
Monsanto, the developer of MON 810, conducted a 42-d nutritional equivalence study in broiler chickensCitation54 in which 55–60% MON 810 was fed. Broiler chickens are a rapidly growing species that is sensitive to nutritional differences that could impact growth and is, therefore, useful for the prediction of impacts on both animal health and performance. This broiler study demonstrated that there were no significant differences between MON 810 and the conventional comparator in body weights, feed conversion, chill weights, fat pad weights, or in weights of thighs, wings, breast, or drums. An academic study found that MON 810 at a dietary incorporation rate of 30–35% did not impact mortality, feed consumption, weight gain, terminal body weights, or hematological and biochemical parameters in broiler chickens after 42 d.Citation52 Additional independent research also found that MON 810 did not impact body weight, weight gain, feed intake/conversion, or mortality in a 42-d broiler chicken studyCitation53 and that MON 810 did not impact carcass or meat yields, abdominal fat pad, selected organ weights, nor breast muscle chemical composition. These studies each demonstrate comparable performance in broiler chickens fed MON 810 relative to its conventional maize comparator, as would be expected due to the equivalence conclusion derived from the comparative weight of evidence assessment between MON 810 and its non-GE counterpart.
In a collaborative effort between academic scientists and Monsanto, MON 810 or its conventional counterpart were fed to lactating dairy cows as grain (20–34% feed) and silage (42–60% feed) over two successive years.Citation55 In this study, there were no differences in nutrient composition between grain or silage sources and cows were fed ad libitum and milked twice per day. There were no impacts of MON 810 on feed intake nor milk production and composition. Production efficiency and nutritional value of MON 810 and its conventional counterpart were considered equivalent. In a 25-month feeding study (spanning two consecutive lactations), dairy cows fed MON 810 or its isogenic control in nutritionally equivalent diet rations, feed intake was comparable across treatments.Citation56 Milk yield, milk consumption, and body condition were unaffected by treatment, confirming compositional and nutritional equivalence of MON 810 and its conventional counterpart. Therefore, MON 810 does not impact the performance of lactating dairy cows which utilize a high dietary incorporation level of maize (~28–42% kernels and ~14–21% cobs).
An academic study demonstrated in a 110-d feeding study that MON 810 maize (~40% to 80% dietary incorporation during various study phases) did not impact the growth or health of pigs relative to that of isogenic maize fed animals.Citation57 Endpoints included body weight and feed intake, body composition, organ weights, serum biochemistry, urinalysis, and histological analysis. When MON 810 was fed to pigs (at ~74–87% dietary inclusion rate) during gestation, lactation, and throughout a 115-d post-weaning period, there were no impacts on growth (offspring and carcass weights), organ weights, histopathological endpoints, serum biochemistry, or other changes indicative of adverse impacts to animal health.Citation58 These data demonstrate that MON 810 does not adversely impact the health or growth of adult, fetal or juvenile pigs.
A 10-generation feeding study with MON 810 in Japanese quail at a dietary incorporation rate of 25% w/w was conducted.Citation59,Citation60 There was no impact of MON 810 on egg-laying/egg mass, hatching, survival, or bodyweight of either adult or young quail.Citation59 There were no treatment-related differences in organ and tissue weights and no adverse impacts of MON 810 on quail after 10 generations of feeding.Citation59 Necropsy and histopathological examination on 17-week old animals from each generation did not indicate pathological impacts of MON 810 on quail.Citation60 These feeding study data confirm the nutritional equivalence of MON 810 to its conventional counterpart and indicate no adverse impacts of MON 810 on the health or growth of Japanese quail over 10 successive generations.Citation59,Citation60
Collectively, these feeding trials demonstrate the nutritional equivalence of MON 810 to its conventional counterpart. This indicates that there are no negative impacts of the genetic modification that impact the feed value of MON 810.
Additional Animal Studies with MON 810
As discussed above, a favorable nutritional and safety profile can be predicted based on the weight of the evidence for equivalence of MON 810 to its non-GE counterpart, with confirmatory animal feeding studies providing little to the overall safety assessment in the absence of a risk hypothesis. The molecular characterization and equivalence data are more than sufficient to demonstrate the safety of MON 810 and it is now understood that the animal feeding studies done at the time to support the initial approval of this product provided assurance to regulators and the public but were not scientifically necessary to demonstrate the safety of this nutritionally equivalent product.
A 90-d rat feeding study evaluating toxicology response variables was conducted by the product developer to complement the weight of the evidence regarding its safety and nutritional equivalence and as an additional means to evaluate for biological impacts of any potential unintended effects of the genetic modification. In this assessment, MON 810 maize grain was fed to rats for 90 d.Citation61 MON 810 or its near-isogenic control grain were fed to 20 rats/sex/group at 11% and 33% dietary incorporation levels in a nutritionally balanced diet that conformed to the specifications for PMI Certified LabDiet® 5002, a commonly used rodent chow in the US. Evaluated parameters included a range of toxicology response variables including: clinical observations, body weight and calculated gain, food consumption, clinical pathology assessment (blood chemistry, hematology, clotting, urinalysis), organ weights, gross and microscopic pathology. Weight gain and food consumption were comparable in test and control groups and there were no treatment-related differences in any of the evaluated parameters, confirming the weight of the evidence from a multitude of other studies discussed herein, that MON 810 is nutritionally equivalent to its conventional counterpart at substantial levels of dietary incorporation (33% of the daily food intake) and does not adversely impact animal growth or health. These study results further support the safe use of MON 810 for food and feed as demonstrated by the weight of the evidence above.Citation61
According to the principles of the comparative safety assessment paradigm in its current form, feeding studies are not necessary to demonstrate the safety of the GE crop unless the crop is designed for health benefits, the composition of the crop is not comparable to conventional foods, or there is an expected impact to nutrient bioavailability.Citation18 In the case of MON 810, the assessments outlined above support a conclusion of equivalence and do not indicate any concerns relative to non-GE varieties of the crop. Therefore, although conducted at the time of MON 810 development based on this being a new type of product in the marketplace, the weight of evidence approach for GE crop evaluation did not indicate that feeding studies were necessary to have reached a conclusion of safety. In agreement with this concept, the European Food Safety Authority (EFSA) has indicated that, “In the situation where molecular, compositional, phenotypic, agronomic and other analyses have demonstrated equivalence between the GM [GE] plant-derived food and feed and their near-isogenic counterpart, except for the inserted trait(s), and do not indicate the occurrence of unintended effects, experiences with GM [GE] plants modified for agronomic input traits have demonstrated that the performance of 90-day feeding trials with rodents or feeding trials with target animal species have provided little if anything to the overall safety assessment (except for added confirmation of safety)”.Citation20 Furthermore, a detailed review on the use of whole-food animal studies for GE crop safety assessment concluded that based on the comparative robustness and reliability of the compositional and agronomic evaluations and the minimal potential for toxicologically significant compositional alterations due to transgene insertion, that these studies are “unnecessary and scientifically unjustifiable”.Citation22 Based on the safety of GM crops in farm animals and the weight of evidence amassed from favorable toxicological results in animal feeding studies, it has been concluded that for GM crops, “90-day feeding studies are not needed on a routine basis, and that further long-term animal feeding studies are not needed either, unless there are specific reasons to do so”.Citation62 Despite these science-based assessments calling into question the need to conduct animal feeding studies based on the weight of the scientific evidence, including the above assessment by the European Food Safety Authority, a political decision was made to make the 90-d rat feeding study a legal requirement in the European Union per the EU Commission Implementing Regulation No. 503.2013.Footnote1
There is a broad scientific consensus on the food and feed safety of current GE crops,Citation30,Citation31,Citation62,Citation63,Citation64,Citation65 developed in part by the body of safety data for MON 810. MON 810 has been approved for food use, feed use, and/or cultivation by 27 countries/geographical regions (http://www.isaaa.org/gmapprovaldatabase; queried 5/20/2019), signaling a broad international consensus on the safety of this product. Despite this scientific consensus and the available safety assessment data for MON 810 (including the above-published weight of the evidence and the confirmatory toxicology data) and many years of safe use of this product in agricultural production, a decision was made by the European Commission, the EU’s legislative body (i.e., a political rather than scientific body), to fund and conduct toxicological assessments that independently assess the safety of this product. The European research efforts to replicate MON 810 feeding studies were inconsistent with guidance from EFSA, who indicated that for GE crops with agronomic input traits that are equivalent to their conventional counterpart and for which unintended effects have not been noted, that, “ … the performance of 90-day feeding trials with rodents or feeding trials with target animal species have provided little if anything to the overall safety assessment (except for added confirmation of safety)”.Citation20
Through the European Commission funded GMO Risk Assessment and Communication of Evidence (GRACE; www.grace-fp7.eu) Project, two 90-d rat studies and a one-year rat feeding study were conducted with MON 810.Citation23,Citation24 The two 90-d rat feeding studies were conducted using germplasm (i.e., background genetics) from two different seed companies, Monsanto and Pioneer. The 90-d studies were conducted based on considerations from OECD test guideline 408 on repeated dose 90-day oral toxicity studies in rodents for the testing of chemicalsCitation66 and EFSA guidance on the conduct of 90-d oral toxicity studies with whole food/feed.Citation67 Each study compared Wistar Han RCC rats fed diets containing MON 810 (11% and 33% dietary incorporation levels; 33% total maize incorporation) to those fed its corresponding near-isogenic non-GE maize or conventional reference maize varieties (N = 16 rats/sex/group). Evaluated parameters included clinical observations, body weight, food consumption, clinical pathology assessment (blood chemistry and hematology), ophthalmological exams, organ weights, gross and microscopic pathology. There were no treatment-related differences in any of these parameters, confirming that MON 810 is nutritionally equivalent to its conventional counterpart at substantial levels of dietary incorporation (up to 33% of the daily food intake) and that MON 810 does not impact animal growth or health.Citation24 Collectively, the 90-d studies conducted by the GRACE project and by Hammond et al.Citation61 provide corroborative data on top of the existing weight of the evidence supporting the safe use of MON 810 for food and feed. However, the additional studies conducted by the GRACE project were not necessary for concluding on the safety of MON 810 in light of the existing weight of the scientific evidence.
Although Zeljenková and colleaguesCitation24 noted that the two GRACE 90-d feeding studies, “did not reveal any scientific trigger for an extension of the feeding period”, the GRACE project also included a one-year chronic oral toxicity assessment of this product.Citation23 This one-year chronic feeding study was conducted taking into consideration the OECD Test Guideline 452 on chronic toxicity studies for the testing of chemicalsCitation68 and also applicable guidance from EFSA on the conduct of 90-d oral toxicity studies with whole food/feed.Citation67 This study included MON 810 at 11% or 33% incorporation, its near-isogenic non-GE control, and a conventional reference variety (33% total maize incorporation), fed to 20 paired animals/sex/group.Citation23 Evaluated parameters included a range of toxicology response variables such as clinical observations, body weight, food consumption, clinical pathology assessment (blood chemistry and hematology, urinalysis), ophthalmological exams, organ weights, gross and microscopic pathology. There were no treatment-related differences in any of the in-life toxicology response variables, confirming the weight of the evidence for the mammalian safety of MON 810. The authors conclude that, “The results obtained show that the MON 810 maize at a level of up to 33% in the diet did not induce adverse effects in male and female Wistar Han RCC rats after a chronic exposure”.Citation23 These independent research data provide confirmation of the safe use of MON 810 for food and feed. Based on its collective toxicology database on MON 810, the GRACE project concluded that, “GRACE data support the scientific reasoning that only in case a trigger is available from the initial molecular, compositional, phenotypic and/or agronomic analyses, feeding trials with whole food/feed may provide an added scientific value for the risk assessment of GM [GE] crops”.Citation23 Furthermore, the GRACE project reported that, “The 1-year study conducted in GRACE did not provide relevant additional information compared with the 90-day studies (http://www.grace-fp7.eu/).”
An additional feeding study in the EU, the GMO90+ Project, also concluded in a 6-month rat feeding trial (30 Wistar Han RCC rats/sex/group) with MON 810 at up to 33% in the diet.Citation69 This study included an assessment of traditional toxicology response variables and also included non-targeted “omics” assessments (transcriptomics and metabolomics). There were no treatment-related effects of MON 810 noted in this study and therefore, these results by Coumoul and colleagues provide further support for the conclusion that MON 810 produces no adverse impacts in rats. Although the additional “omics” assessments provide more measurements, these conclusions could have been reached without the additional non-targeted data as any toxicologically relevant findings in the animals would have manifested themselves in physiological effects detectable within a standard toxicological assessment. This study further confirms that when there is compositional equivalence between a GE crop and its conventional comparator, there should not be an expectation that additional measurements will result in a difference that is relevant to the wholesomeness or safety of the GE crop. The existing data sets generated for GE crops are therefore sufficiently robust to reach a definitive conclusion on the potential impacts of these crops on the health of the consuming organism.
Discussion
As one of the first insect-protected GE crops to reach the marketplace, MON 810 has been widely cultivated and represents one of the few products that was approved for cultivation within the European Union. As an early GE product, an unusually large weight of the evidence exists for the safety of MON 810 and the Bt crystal protein Cry1Ab that confers insect control to this maize product.
One of the key components supporting the determination of potential adverse effects of the genetic modification on the crop is a detailed understanding of the identity and location within the plant of the inserted genetic material. In MON 810, this analysis confirms that the inserted genetic material has not interrupted a critical gene or locus in the plant and would therefore not have an adverse impact on the physical properties or on the edible portions of the plant (unpublished data, http://www.isaaa.org/gmapprovaldatabase/default.asp). The lack of agronomic or phenotypic impact of the modification on MON 810 can be demonstrated by its yield and normal field performance (e.g. normal plant height, pollen production, ear length, etc.; http://www.isaaa.org/gmapprovaldatabase/default.asp). These endpoints indicate that the plants have not been fundamentally altered by insertion of the transgene in such a way that would impact plant physiology and therefore, it is highly unlikely that fundamental changes in plant metabolism have occurred (e.g. no physiological manifestation if there were metabolic changes) and this is also further demonstrated through the compositional assessment.
The most critical component of the weight of the evidence for MON 810 as it pertains to food or feed safety was a demonstration of compositional equivalence between MON 810 and its appropriate non-GE conventional comparator, as summarized above. The compositional evaluation showed that there were no unanticipated compositional impacts of the genetic modification that would have food or feed safety impacts, and therefore demonstrated that MON 810 was compositionally equivalent to and as safe as conventional maize. It is worth noting that the analyses of many other GE crops have likewise demonstrated compositional equivalence between the GE crop and a close non-GE comparator, providing strong empirical evidence that the process of creating a GE crop is not intrinsically disruptive of crop metabolism.Citation11
The safety of the Cry1Ab protein expressed in MON 810 was based on the history of safe use of the source organism for this protein (Bt), the lack of similarity of Cry1Ab to known toxic proteins, its rapid degradation by gastric proteases (some allergens are refractory to digestion), and its heat lability (indicative of degradation due to cooking and/or processing).Citation6 These data reflect the “Tier I” safety assessment and are sufficient for demonstrating the safety of Cry1Ab for food and feed use.Citation70 Nevertheless, due to the nascent status of GE crops at the time MON 810 was under development, a toxicological evaluation of Cry1Ab in animals was conducted as a confirmatory approach to further illustrate the safety of this protein. Cry1Ab did not exhibit toxicity in mice after exposure to a very high dose of this protein via the oral exposure route. Specifically, Cry1Ab protein was dosed at up to 4000 mg/kg in mice and did not produce oral toxicity.Citation43,Citation49 Using a Cry1Ab expression level in a grain of 0.5 µg/g as described aboveCitation27, a 70 kg person would have to eat 560,000 kg of maize grain in a single day to reach a Cry1Ab exposure equivalent to this tested dose. Alternatively, using the estimated expression level in grain from MON 810 of 0.5 µg/g and US maize consumption at the 95th percentile of 2.0 g/kg/day2 (1.0 µg/g of Cry1Ab exposure/day), mice administered 4000 mg/kg/day of Cry1Ab received 1.8 × 105 times as much of this protein as humans would consume in the most highly exposed US subpopulation if all grain consumed in the US contained MON 810. This margin of exposure (MOE) far exceeds that targeted to ensure human health protection, as an MOE of 1,000 represents a conservative value to protect human health (covers 10x for animal to human extrapolation, 10x for inter-individual variation within the human population, and an additional 10x to account for any additional uncertainties).
The collective weight of the evidence demonstrating the safety of the Cry1Ab protein and equivalent agronomic, phenotypic, and compositional profiles between MON 810 and its near-isogenic non-GE maize counterpart provides assurance of the food/feed safety of this product. However, studies with GE crops fed to food-producing animals were also conducted on this early GE crop product to determine if these crops were nutritionally equivalent for livestock, the most extensive consumers of GE cropsCitation63 such as MON 810. These studies conducted by the technology provider and by independent academic and government laboratories demonstrate that MON 810 is nutritionally equivalent to its conventional counterpart in broiler chickens, dairy cattle, pigs, and quail. Collectively, the weight of the evidence from technology providers and independent researchers provides a robust demonstration of the equivalence and food and feed safety for insect-protected maize MON 810.
Despite the weight of evidence for the safety of MON 810, further assurance to the consumer, policymaker, and regulatory evaluators were provided by the technology provider through the conduct of a 90-d rat feeding study with MON 810 that included assessment of toxicology response variables. This comparative feeding study addressed the potential for food and feed safety impacts of any unintended changes made to MON 810 during the genetic modification. This study did not identify any impacts of MON 810 to the growth or health of Sprague–Dawley rats following 90 d of feeding with a whole grain at up to 33% in the diet. Therefore, after approximately 10% of the rat lifespan, a food source representing about a third of daily caloric intake had no impact on the consuming organism.
Based on the weight of the evidence described above, there were no adverse impacts of the genetic modification on MON 810. Therefore, the conduct of a 90-d rat feeding study was unnecessary for demonstrating the safety of MON 810. Nevertheless, such data were generated in light of the timing of this product development, as an early example of a GE crop, when there was a less robust overall dataset supporting the safety of GE crops. Unfortunately, this historical precedent has led to some regulatory expectations that such an analysis be conducted for each new GE variety, despite such assessments to date having simply confirmed the safety already determined by evaluating the weight of the evidence from other endpoints as discussed herein. While unnecessary for demonstration of safety, these confirmatory studies have confirmed the predictive value for safety assessment afforded by the agronomic, phenotypic, and compositional equivalence data and Tier I safety data of the trait. Thus, as previously noted, 90-d rat feeding studies should only be conducted in the case that there is a testable scientific hypothesis-driven by changes in agronomic, phenotypic, or compositional analyses that could signal unintended effects with potential for safety implications in the consuming organism.
Despite the breadth of evidence available to support the safety of GE crops, in particular, MON 810, and the lack of necessity for feeding studies to demonstrate their safety, millions of euros were spent to confirm the lack of impact of MON 810 to animal growth or health. These studies included feeding studies of 90 d, 6 months, and 1-y duration.Citation23,Citation24,Citation69 These studies utilized approximately 700 rats to provide confirmatory data on a product that has been approved for two decades and has been extensively grown around the globe. These confirmatory data were generated on top of existing data from feeding studies on thousands of experimental animals (e.g. rats, pigs, cows, chickens, quail; Supplemental Table 1) and after years of safe consumption by livestock animals in commercial feed. Despite the lack of value added by whole food feeding studies on GE crops and the additional use of significant quantities of animals for confirmatory feeding studies, feeding projects and regulatory requirements for the 90-d rat study continue in the EU and globally.
The weight of the scientific evidence on GE crops derived from product characterization data and protein safety evaluation data provides the necessary information for the assurance of food and feed safety of new GE crops. Rather than defaulting to animal feeding studies and whole-food toxicological assessments, such studies should only be conducted, if needed, based on a hypothesis that leverages the data generated during product development and characterization. Based on two decades of safe use of GE crops, and their robust characterization, adoption of a streamlined regulatory paradigm that appropriately weighs these safety data are in order. This refined approach could raise consumer confidence by removing the implication of putative unanticipated impacts on the crop and putative toxicological hazards from the GE crop when both an understanding of the GE crop development process and empirical evidence demonstrate that such effects are not observed in GE crop production. This approach would eliminate unnecessary animal testing for products that are already demonstrated to be safe based on the weight of the scientific evidence. This animal testing of GE crops by default, therefore, represents the usage of animals in research that is not scientifically justified nor consistent with ethical obligations to reduce, refine, and replace (3Rs) animal testing where possible. Furthermore, this new approach of using the risk-based determination of study requirements would also result in a lower barrier to entry for product registration by universities, not for profit institutions, and start-up companies that could potentially result in more traits to benefit a broader range of agricultural production systems.
Declaration of Interest Statement
All funding for this manuscript was provided by Monsanto Company and Bayer Crop Science. Jay Petrick, Michael Koch, and Erin Bell were all employed by Monsanto Company or Bayer Crop Science during the period in which this paper was authored. Note that Monsanto became a member of the Bayer Group as of August 21, 2018. However, as some of the work on this paper was conducted before the specified date, the name ‘Monsanto’ is maintained within the document and Erin Bell, who left the company prior to August 21, 2018, is only listed as a Monsanto employee. MON 810 is a genetically modified maize product from Bayer Crop Science, a producer of genetically modified seeds, of which the safety evaluation of these products and technology is the subject of this manuscript.
Supplemental Material
Download PDF (19 KB)Acknowledgments
The authors would like to acknowledge Nicolo R. Visconti for support in assembling the table on toxins in food and Colton R. Kessenich for conducting the bioinformatics assessment for Bt-R1 homologs in humans and other mammals.
Supplementary material
Supplemental data for this article can be accessed on the publisher’s website.
Notes
1. EC Commission. Implementing Regulation (EU) No 503/2013 of 3 April 2013 on applications for authorization of GM [GE] food and feed in accordance with Regulation (EC) No 1829/2003 of the European Parliament and of the Council and amending Commission Regulations (EC) No 641/2004 and (EC) No 1981/2006. Off. J. Eur. Communities 2013, L157, 1−52.
2. Corn consumption was based on Dietary Exposure Evaluation Model Food Commodity Intake Database (DEEMFCID/Calendex version 4.02, 05-10-c, https://www.epa.gov/pesticide-science-and-assessing-pesticide-risks/models-pesticide-risk-assessment#deem) and food consumption data from the National Health and Nutrition Examination Survey/“What We Eat in America” dietary survey for the years 2005–2010. Maize consumption on a g/kg/day user basis is derived using an average body weight of 57 kg for the overall US population. Commodities included: corn flour, corn flour-baby food, cornmeal, corn meal-baby food, corn bran, corn starch, corn starch-baby food, popcorn, sweet corn, and sweet corn-baby food.
References
- ISAAA. Global status of commercialized biotech/GM Crops in 2018: biotech crops continue to help meet the challenges of increased population and climate change. Ithaca (New York): International Service for the Acquisition of Agri-biotech Applications; 2018.
- Brookes G, Barfoot P. Environmental impacts of genetically modified (GM) crop use 1996–2015: impacts on pesticide use and carbon emissions. GM Crops Food. 2017;8:117–47. doi:10.1080/21645698.2017.1309490.
- Kouser S, Qaim M. Impact of Bt cotton on pesticide poisoning in smallholder agriculture: A panel data analysis. Ecol Econ. 2011;70:2105–13. doi:10.1016/j.ecolecon.2011.06.008.
- Kouser S, Qaim M. Valuing financial, health, and environmental benefits of Bt cotton in Pakistan. Ag Econ. 2013;44:323–35. doi:10.1111/agec.12014.
- Huang J, Hu R, Pray C, Qiao F, Rozelle S. Biotechnology as an alternative to chemical pesticides: a case study of Bt cotton in China. Ag Econ. 2005;29:55–67. doi:10.1111/j.1574-0862.2003.tb00147.x.
- ILSI. A review of the food and feed safety of the Cry1Ab protein. Washington (D.C): ILSI Research Foundation; 2016.
- EPA, U.S. Reregistration eligibility decision (RED): bacillus thuringiensis. Washington (D.C): U.S. Environmental Protection Agency; 1998.
- FDA U.S. Statement of policy: foods derived from new plant varieties. Fed Regist. 1992;57:22984–3005.
- Glenn KC, Alsop B, Bell E, Goley M, Jenkinson J, Liu B, Martin C, Parrott W, Souder C, Sparks O, et al. Bringing new plant varieties to market: plant breeding and selection practices advance beneficial characteristics while minimizing unintended changes. Crop Sci. 2017;57:2906–21. doi:10.2135/cropsci2017.03.0199.
- Conko G, Kershen DL, Miller H, Parrott W. A risk-based approach to the regulation of genetically engineered organisms. Nature Biotechnol. 2016;34:493–503. doi:10.1038/nbt.3568.
- Herman RA, Price WD. Unintended compositional changes in genetically modified (GM) crops: 20 years of research. J Agric Food Chem. 2013;61:11695–701. doi:10.1021/jf400135r.
- Schnell J, Steele M, Bean J, Neuspiel M, Girard C, Dormann N, Pearson C, Savoie A, Bourbonnière L, Macdonald P. A comparative analysis of insertional effects in genetically engineered plants: considerations for pre-market assessments. Transgenic Res. 2015;24:1–17. doi:10.1007/s11248-014-9843-7.
- Weber N, Halpin C, Hannah LC, Jez JM, Kough J, Parrott W. Editor’s choice: crop genome plasticity and its relevance to food and feed safety of genetically engineered breeding stacks. Plant Physiol. 2012;160:1842–53. doi:10.1104/pp.112.204271.
- Hirsch CN, Hirsch CD, Brohammer AB, Bowman MJ, Soifer I, Barad O, Shem-Tov D, Baruch K, Lu F, Hernandez AG, et al. Draft assembly of elite inbred line PH207 provides insights into genomic and transcriptome diversity in maize. Plant Cell. 2016;28:2700–14. doi:10.1105/tpc.16.00353.
- Lisch D. How important are transposons for plant evolution? Nature Rev Genet. 2013;14:49–61. doi:10.1038/nrg3374.
- Dolan LC, Matulka RA, Burdock GA. Naturally occurring food toxins. Toxins. 2010;2:2289–332. doi:10.3390/toxins2092289.
- Codex Alimentarius. Guideline for the conduct of food safety assessment of foods derived from recombinant-DNA plants. Codex Alimentarius Commission. Rome (Italy): Joint FAO/WHO Food Standards Programme, Food and Agriculture Organization of the United Nations; 2003.
- Codex Alimentarius. Foods derived from modern biotechnology. Codex Alimentarius Commission. Rome (Italy): Joint FAO/WHO Food Standards Programme, Food and Agriculture Organization of the United Nations; 2009.
- Codex Alimentarius. Foods derived from modern biotechnology. Codex Alimentarius Commission. Rome (Italy): Joint FAO/WHO Food Standards Programme, Food and Agriculture Organization of the United Nations; 2004.
- EFSA. Safety and nutritional assessment of GM plants and derived food and feed: the role of animal feeding trials. Food ChemToxicol. 2008;46:S2–S70. doi:10.1016/j.fct.2008.02.008.
- FAO-WHO. Safety aspects of genetically modified foods of plant origin. Report of a joint FAO/WHO expert consultation on foods derived from biotechnology. Geneva (Switzerland), Food and Agriculture Organization of the United Nations, World Health Organization, 2000.
- Bartholomaeus A, Parrott W, Bondy G, Walker K. The use of whole food animal studies in the safety assessment of genetically modified crops: limitations and recommendations. Crit Rev Toxicol. 2013;43 (Suppl 2):1–24. doi:10.3109/10408444.2013.842955.
- Zeljenková D, Alácˇová R, Ondrejková J, Ambrušová K, Bartušová M, Kebis A, Kovrižnych J, Rollerová E, Szabová E, Wimmerová S, et al. One‑year oral toxicity study on a genetically modified maize MON810 variety in Wistar Han RCC rats (EU 7th Framework Programme project GRACE). Arch Toxicol. 2016;90:2531–62. doi:10.1007/s00204-016-1798-4.
- Zeljenková D, Ambrušová K, Bartušová M, Kebis A, Kovrižnych J, Krivošíková Z, Kuricová M, Líšková A, Rollerová E, Spustová V, et al. Ninety‑day oral toxicity studies on two genetically modified maize MON810 varieties in Wistar Han RCC rats (EU 7th Framework Programme project GRACE). Arch Toxicol. 2014;88:2289–314. doi:10.1007/s00204-014-1374-8.
- FAO-WHO. Joint FAO/WHO expert consultation on biotechnology and food safety. Rome (Italy): Food and Agriculture Organization of the United Nations, World Health Organization, 1996.
- OECD. Safety evaluation of foods derived by modern biotechnology: concepts and principles. Paris (France): Organisation for Economic Co-operation and Development; 1993.
- Sanders PR, Lee TC, Groth ME, Astwood JD, Fuchs RL. Safety assessment of insect-protected corn. In: Thomas JA, editor. Biotechnology and safety assessment. New York,NY: Taylor and Francis; 1998. p. 241–56.
- EPA, U.S. Biopesticides Registration Action Document - Bt Plant-Incorporated Protectants. 2001. https://www3.epa.gov/pesticides/chem_search/reg_actions/pip/bt_brad2/2-id_health.pdf
- EFSA. EFSA panel on genetically modified organisms. Scientific opinion on the annual post-market environmental monitoring (PMEM) report from Monsanto Europe S.A. on the cultivation of genetically modified maize MON 810 in 2010. EFSA Jl. 2012;10:2610.
- Hammond BG, Koch MS. A review of the food safety of Bt crops. In: Sansinenea E, editor. Bacillus thuringiensis Biotechnology. 2012. p. 305–25. Dordrecht: Springer. doi: 10.1007/978-94-007-3021-2_16.
- Koch MS, Ward JM, Levine SL, Baum JA, Vicini JL, Hammond BG. The food and environmental safety of Bt crops. Front Plant Sci. 2015;6:283. doi:10.3389/fpls.2015.00283.
- Huber HE, Lüthy P. Bacillus thuringiensis δ-endotoxin: composition and activation. In: Davidson EW, editor. Pathogenesis of invertebrate microbial diseases. Totowa (New Jersey): Allanheld, Osman & Co. Publishers.; 1981. p. 242–55.
- Shukla R, Cheryan M. Zein: the industrial protein from corn. Ind Crops Prod. 2001;13:171–92. doi:10.1016/S0926-6690(00)00064-9.
- Bravo A, Gomex I, Conde J, Munoz-Garay C, Sanchez J, Miranda R, Zhuang M, Gill SS, Soberón M. Oligomerization triggers binding of a Bacillus thuringiensis Cry1Ab pore-forming toxin to aminopeptidase N receptor leading to insertion into membrane microdomains. Biochim Biophys Acta. 2004;1667:38–46. doi:10.1016/j.bbamem.2004.08.013.
- Fernandez LE, Gomez I, Pacheco S, Arenas I, Gilla SS, Bravo A, Soberón M. Employing phage display to study the mode of action of Bacillus thuringiensis Cry toxins. Pept. 2008;29:324–29. doi:10.1016/j.peptides.2007.07.035.
- Pardo-Lopez L, Gomez I, Munoz-Garay C, Jimenez-Juarez N, Soberon M, Bravo A. Structural and functional analysis of the pre-pore and membrane-inserted pore of Cry1Ab toxin. J Invertebr Pathol. 2006;92:172–77. doi:10.1016/j.jip.2006.02.008.
- Pena-Cardena A, Grande R, Sanchez J, Tabashnik BE, Bravo A, Soberon M, Gomez, I. The C-terminal protoxin region of Bacillus thuringiensis Cry1Ab toxin has a functional role in binding to GPI-anchored receptors in the insect midgut. J Biol Chem. 2018;293:20263–72. doi:10.1074/jbc.RA118.005101.
- Flannagan RD, Cao-Guo Y, Mathis JP, Meyer TE, Shi X, Siqueira HAA, Siegfried BD. Identification, cloning and expression of a Cry1Ab cadherin receptor from European corn borer, Ostrinia nubilalis (Hubner) (Lepidoptera: crambidae). Insect Biochem Mol Biol. 2005;35:33–40. doi:10.1016/j.ibmb.2004.10.001.
- Gomez I, Arenas I, Benitez I, Miranda-Rios J, Becerril B, Grande R, Almagro JC, Bravo A, Soberón M. Specific epitopes of domains II and III of Bacillus thuringiensis Cry1Ab toxin involved in the sequential interaction with cadherin and aminopeptidase-N receptors in Manduca sexta. J Biol Chem. 2006;281:34032–39. doi:10.1074/jbc.M604721200.
- Noteborn HPJM, Bienenmann-Ploum ME, van Den Berg JHJ, Alink GM, Zolla L, Reynaerts A, et al. Safety assessment of bacillus thuringiensis insecticidal protein CRY1A(b) expressed in transgenic tomato. In: Engel K-H, Takeoka GR, Teranishi Reditors. Genetically modified foods: safety issues American Chemical Society. Washington (D.C.); 1995. p. 134–47.
- Altschul SF, Madden TL, Schaffer AA, Zhang J, Zhang Z, Miller W, Lipman DJ. Gapped BLAST and PSI-BLAST: A new generation of protein database search programs. Nucleic Acids Res. 1997;25:3389–402. doi:10.1093/nar/25.17.3389.
- Dantzig AH, Hoskins JA, Tabas LB, Bright S, Shepard RL, Jenkins IL, Duckworth DC, Sportsman JR, Mackensen D, Rosteck PR. Association of intestinal peptide transport with a protein related to the cadherin superfamily. Sci New York, N.Y. 1994;264:430–33. doi:10.1126/science.8153632.
- U.S. EPA. Biopesticides registration action document: cry1Ab and Cry1F Bacillus thuringiensis (Bt) corn plant-incorporated protectants. Washington (D.C.): U.S. Environmental Protection Agency; 2010.
- Liang R, Fei YJ, Prasad PD, Ramamoorthy S, Han H, Yang-Feng TL, Hediger MA, Ganapathy V, Leibach FH. Human intestinal H+/peptide cotransporter. Cloning, functional expression, and chromosomal localization. J Biol Chem. 1995;270:6456–63. doi:10.1074/jbc.270.12.6456.
- Adibi SA. The oligopeptide transporter (Pept-1) in human intestine: biology and function. Gastroenterol. 1997;113:332–40. doi:10.1016/s0016-5085(97)70112-4.
- Wang CY, Liu S, Xie XN, Tan ZR. Regulation profile of the intestinal peptide transporter 1 (PepT1). Drug Des Devel Ther. 2017;11:3511–17. doi:10.2147/dddt.s151725.
- Andreassen M, Bøhn T, Wikmark O.G, Bodin J, Traavik T, Løvik M, Nygaard UC. Investigations of immunogenic, allergenic and adjuvant properties of Cry1Ab protein after intragastric exposure in a food allergy model in mice. BMC Immunol. 2016;17:10. doi:10.1186/s12865-016-0148-x.
- Batista R, Nunes B, Carmo M, Cardoso C, Jose HS, de Almeida AB, Manique A, Bento L, Ricardo CP, Oliveira MM. Lack of detectable allergenicity of transgenic maize and soya samples. J Allergy Clin Immunol. 2005;116:403–10. doi:10.1016/j.jaci.2005.04.014.
- Betz FS, Hammond BG, Fuchs RL. Safety and advantages of Bacillus thuringiensis-protected plants to control insect pests. Regul Toxicol Pharmacol. 2000;32:156–73. doi:10.1006/rtph.2000.1426.
- McClintock JT, Schaffer CR, Sjoblad RD. A comparative review of the mammalian toxicity of Bacillus thuringiensis-based pesticides. Pestic Sci. 1995;45:95–105. doi:10.1002/ps.v45:2.
- ILSI. A review of the environmental safety of the Cry1Ab protein. Washington (D.C): ILSI Research Foundation; 2011.
- Rehout V, Kadlec J, Citek J, Hradecka E, Hanusova L, Hosnedlova B, Lád F. The influence of genetically modified Bt maize MON 810 in feed mixtures on slaughter, haematological and biochemical indices of broiler chickens. J Anim Feed Sci. 2009;18:490–98. doi:10.22358/jafs/66423/2009.
- Swiatkiewicz S, Swiatkiewicz M, Koreleski J, Kwiatek K. Nutritional efficiency of genetically-modified insect resistant corn (MON 810) and glyphosate-tolerant soybean meal (Roundup Ready) for broilers. J Vet Res. 2010;54:43–48.
- Taylor ML, Hartnell GF, Riordan SG, Nemeth MA, Karunanandaa K, George B, Astwood JD. Comparison of broiler performance when fed diets containing grain from YieldGard (MON810), YieldGard x Roundup Ready (GA21), nontransgenic control, or commercial corn. Poult Sci. 2003;82:823–30. doi:10.1093/ps/82.5.823.
- Donkin SS, Velez JC, Totten AK, Stanisiewski EP, Hartnell GF. Effects of feeding silage and grain from glyphosate-tolerant or insect-protected corn hybrids on feed intake, ruminal digestion, and milk production in dairy cattle. J Dairy Sci. 2003;86:1780–88. doi:10.3168/jds.S0022-0302(03)73763-1.
- Steinke K, Guertler P, Paul V, Wiedemann S, Ettle T, Albrecht C, Meyer HHD, Spiekers H, Schwarz FJ. Effects of long-term feeding of genetically modified corn (event MON810) on the performance of lactating dairy cows. J Anim Physiol Anim Nutr (Berl). 2010;94:e185–e193. doi:10.1111/j.1439-0396.2010.01003.x.
- Buzoianu SG, Walsh MC, Rea MC, Cassidy JP, Ross RP, Gardiner GE, Lawlor PG. Effect of feeding genetically modified Bt MON810 maize to similar to 40-day-old pigs for 110 days on growth and health indicators. Anim. 2012;6:1609–1619. doi:10.1017/s1751731112000249.
- Buzoianu SG, Walsh MC, Rea MC, Cassidy JP, Ryan TP, Ross RP, Gardiner GE, Lawlor PG. Transgenerational effects of feeding genetically modified maize to nulliparous sows and offspring on offspring growth and health. J Anim Sci. 2013;91:318–30. doi:10.2527/jas.2012-5360.
- Sartowska KE, Korwin-Kossakowska A, Sender G. Genetically modified crops in a 10-generation feeding trial on Japanese quails–evaluation of its influence on birds’ performance and body composition. Poult Sci. 2015;94:2909–16. doi:10.3382/ps/pev271.
- Korwin-Kossakowska A, Sartowska K, Tomczyk G, Prusak B, Sender. G. Health status and potential uptake of transgenic DNA by Japanese quail fed diets containing genetically modified plant ingredients over 10 generations. Br Poult Sci. 2016;57:415–23. doi:10.1080/00071668.2016.1162281.
- Hammond BG, Dudek R, Lemen JK, Nemeth MA. Results of a 90-day safety assurance study with rats fed grain from corn borer-protected corn. Food ChemToxicol. 2006;44:1092–99. doi:10.1016/j.fct.2006.01.003.
- Ricroch AE, Boisron A, Kuntz M. Looking back at safety assessment of GM food/feed: an exhaustive review of 90-day animal feeding studies. Int J Biotechnol. 2014;13:230–56. doi:10.1504/IJBT.2014.068940.
- Van Eenennaam AL, Young AE. Prevalence and impacts of genetically engineered feedstuffs on livestock populations. J Anim Sci. 2014;92:4255–78. doi:10.2527/jas.2014-8124.
- Snell C, Bernheim A, Bergé J-B, Kuntz M, Pascal G, Paris A, Ricroch AE. Assessment of the health impact of GM plant diets in long-term and multigenerational animal feeding trials: A literature review. Food ChemToxicol. 2012;50:1134–48. doi:10.1016/j.fct.2011.11.048.
- Tufarelli V, Selvaggi M, Dario C, Laudadio V. Genetically modified feeds in poultry diet: safety, performance, and product quality. Crit Rev Food Sci Nutr. 2015;55:562–69. doi:10.1080/10408398.2012.667017.
- OECD. Test no. 408: Repeated dose 90-day oral toxicity study in rodents. Paris (France): Organization of Economic Cooperation and Development; 1998.
- EFSA. Scientific Opinion: guidance on conducting repeated-dose 90-day oral toxicity study in rodents on whole food/feed. Parma (Italy): European Food Safety Authority; 2011. EFSA Journal 9(12): 2438.
- OECD. Test No. 452: Chronic toxicity studies. Paris (France): Organization of Economic Cooperation and Development; 2009.
- Coumoul X, Servien R, Juricek L, Kaddouch-Amar Y, Lippi Y, Berthelot L, Naylies C, Morvan M-L, Antignac J-P, Desdoits-Lethimonier C, et al. The GMO90+ project: absence of evidence for biologically meaningful effects of genetically modified maize based-diets on Wistar rats after 6-months feeding comparative trial. Toxicol Sci. 2019;168:315–38. doi:10.1093/toxsci/kfy298.
- Delaney B, Astwood JD, Cunny H, Conn RE, Herouet-Guicheney C, MacIntosh S, Meyer LS, Privalle L, Gao Y, Mattsson J, et al. Evaluation of protein safety in the context of agricultural biotechnology. Food ChemToxicol. 2008;46:S71–S97. doi:10.1016/j.fct.2008.01.045.