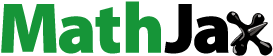
Abstract
Multidisciplinary approach is used to evaluate concrete with recycled concrete aggregates (RCA) from technical, environmental impacts and product circularity perspectives. Two RCA replacements investigated, RAC50: fine aggregates; RAC100: both coarse, fine aggregates. Reference, recycled concretes have same cement content, similar workability and compressive strength requirement, proven experimentally. RCA is sourced from pre-fab element discards of a Swedish plant, the logistical alternatives requiring environmental impact analysis. Alternatives are RCA crushing at plant and crushing at a different location including transportation. LCA shows transportation is second largest contributor after cement in all impact categories. RAC alternatives show lower total impact than reference concrete due to RCA replacement. A circularity index for concrete based on economic value of recirculated aggregates; supplements LCA for sustainability reporting. Circularity index results: RAC100 > RAC50 > RC. Combining circularity index with LCA helps optimize recycling process with regard to amount of recycled material and logistics respectively.
1. Introduction
Natural aggregates such as the sand used in concrete and other road infrastructure applications is an essential part of high quality water reservoirs, is a critical resource in Sweden. A tax imposition of 16 SEK/ton according to Swedish legislation (1995:1667) [Citation1] and an associated environmental goal has limited exploitation to a maximum of 1-3 million ton/year until 2020. The total use of natural sand has decreased by 22% in last 20 years [Citation2]. However, the amount of natural sand delivered to concrete industries in the same period has increased due to increased construction volumes [Citation3]. Recently there is a strong trend in the concrete industry to replace natural sand with crushed rock aggregates, alternatively, techniques to use crushed concrete currently being developed.
Construction and demolition waste, CDW as of 2016, accounts for approximately 32% of the total waste produced in Sweden and in most cases is contaminated with hazardous waste [Citation4]. Mineral waste including concrete rubble is one of the most increasing CDW fractions in Sweden, comprising more than 30% of the total CDW [Citation5]. The concrete waste is crushed to produce recycled concrete aggregates (RCA) used primarily as filling material or in road construction as unbounded application, the focus being on landfill mitigation and freeing-up urban land. At present, the technical feasibility of using RCA in bound applications such as in new structural concrete is being investigated for possible commercial implementation in Sweden [Citation6]. In addition to the production of recycled aggregate concrete (RAC) from RCA, efforts to valorize RCA waste not fetching use in new concrete is being researched. For example, subjecting the cement mortar adhered to RCA for CO2 sequestration [Citation7] or re-using it in clinker to produce new cement [Citation8]. This ensures that all concrete waste is recycled and may further incentivize a concrete recycling supply chain whose major product is RCA.
The environmental impacts of RCA concrete in comparison with natural aggregate concrete is the same when the transportation distance of RCA is lesser than natural aggregate, there is a clear gain in environmental benefits from minimizing resource depletion [Citation9]. However, on an urban scale, reductions in environmental impacts from RCA recycling depend largely on the transportation distances involved, unless the positive environmental impacts from landfill avoidance are also considered [Citation10]. Acknowledging landfill avoidance in impact assessments, results in RAC outperforming natural aggregate concrete [Citation11, Citation12]. The extraction of natural aggregate causes major environmental impacts such as geologic erosion, modifications to landscape and biodiversity [Citation13]. Cement however contributes most to the environmental impact in concrete [Citation12, Citation14]. Roughly, 5-7% of global CO2 emissions arise from cement production, which requires the calcination of clinker and fossil fuels to increase kiln temperature to facilitate calcination [Citation15]. Previous research has shown that RAC requires more cement to achieve alike strength and workability as a reference concrete with natural aggregates [Citation11, Citation16]. This results in a recycled concrete having higher environmental impacts arising from increased cement content challenging the environmental benefits coming from natural aggregate replacement [Citation11]. On the contrary, the feasibility of producing RAC likening a reference concrete in strength and workability with the same cement content is demonstrated on a lab scale by the authors in their previous research [Citation6, Citation17]. The novelty in their experiments is that RCA replacement percentages as high as 50,100% is investigated for the same cement content as reference concrete, therefore eliminating additional environmental impacts arising from cement alone. Compressive strength and workability similar to the reference concrete is reached either through the densification of RCA, paste densification or both [Citation6, Citation17]. Therefore, this article seeks to prove the environmental viability of technical developments achieved in our experiments using a life cycle analysis.
The production of concrete using RCA is still at a nascent stage in Sweden; however, commercial production of crushed rock aggregates for concrete is established. The rock-crushing infrastructure maybe used to upscale RCA production for structural concrete, demonstrated successfully on a laboratory scale involving three successive crushing stages combined with sieving [Citation6]. The crushing infrastructure for rock in Sweden has undergone constant improvement to optimize energy demand, dust and noise pollution for example, by the electrification of crushing processes [Citation18]. The crushing process brings forth 0.002386 kg CO2,eq/kg, value corresponding to the commercial production of crushed rock [Citation19]. Environmental impacts surrounding crushed aggregates is more centered on optimizing transportation distances between quarry site and concrete producers, as shown by a regional study [Citation20]. Therefore, the commencement of commercial RCA production in Sweden requires that logistical aspects related to continuous availability of waste and the the transportation of waste to crusher be resolved [Citation21]. Due to the lack of actual examples, this study analyzes hypothetical logistical scenarios developed in consultation with Swedish aggregate and concrete producers.
LCA does not always show favorable environmental impacts for closed-loop recycling as seen in literature [Citation22, Citation23]. Therefore, there is a need for tools, which can evaluate benefits of material circularity. Indicators capable of evaluating the resource potential of recycled materials, based on a framework that integrates amount of virgin material, unrecoverable waste and utility for a given product are found in research [Citation22]. The resource potential is also calculated using the economic value of the recirculated material in ratio to the economic value of the product, called circularity index [Citation24]. Evaluating resource management from the perspectives of economic value maybe a driving factor to implement concrete recycling on a commercial scale in Sweden where the economic feasibility needs to be justified. The circularity index of a product is indicative of the amount of recirculated material contained in the product. Therefore, this index may work as a product identifier enabling companies to easily identify and choose circular products in the design and construction phase of construction; two of the key parts of a construction value chain [Citation25]. The EU directive 2014/95/EU requires that large Swedish companies including those from the construction sector produce periodical sustainability reports. Also reported are environmental considerations relating to their businesses such as waste management, emissions including resource management [Citation26]. In such a situation, the circularity index may stand as a measurable indicator to account how the company has integrated circular resource management strategies into their activities. The LCA results can supplement the circularity index regarding the environmental impacts resulting from the inclusion of circular materials in the product.
The aim of this study is to combine LCA and circularity index to assess the environmental impacts and product circularity respectively, of a reference concrete compared to when the same concrete is produced with 50%, 100% aggregates replaced with RCA. The concrete recipes have the same amount of cement, water and admixtures, which is also the novelty of this study. Reference concrete, noted as RC, consists of natural sand for fine fractions and crushed stone in the coarse fraction. The RC is currently used to produce wall elements at a local company. Concrete with 100% RCA, denoted RAC100, assumes from a logistics point of view that there is continuous supply of concrete waste. In the alternative 50%, denoted as RAC50, only the fine fractions are replaced by recycled concrete aggregate. From a logistics point of view, this is interpreted as a scarcity of concrete waste for continuous replacement of all aggregates therefore replacing just 50%. Thus, two logistic scenarios are studied for 50%, 100% alternatives, which determines the distance to the crusher. Scenario 1 implies transporting concrete waste to a crushing plant and scenario 2 uses in-situ crushing by installing a mobile crusher on concrete production site. The practical feasibility of scenario 2 depends on the continuous availability of concrete waste. These two scenarios are chosen for being the most realistic given the existing conditions in most of the pre-fab element plants in Sweden [Citation21].
The environmental impacts arising from the logistical scenarios investigated in this study may be combined with crushing processes in RCA production in future research to acquire a comprehensive understanding. The environmental impacts from cement are considered in this investigation to enable comparisons in future studies with other cement types and replacements such as blast furnace slag, milled-glass to enhance RAC concrete properties; investigated separately [Citation6].
2. Methodology
Methods used for this study are life cycle assessment, LCA and circularity indicator, c. This chapter describes these methods and the results of technical tests that has been performed on the different concrete recipes.
2.1. Experimental study
Three mixes are investigated in this study: the reference concrete, reference concrete with 100% replacement and reference concrete with replacement of fine aggregates, denoted as RC, RAC100 and RAC50 respectively. All the three mixes have same w/c ratio 0.5, same quantities of other constituents such as cement content, see . The cement used in the recipes is type: CEM II/A- LL 42.5 R in accordance with EN 197-1 containing 10% of finely ground limestone.
Table 1. Recipe constituents of the concrete mixes compared.
The grading curves for the reference concrete aggregates with natural sand 0/8 mm, crushed stone 8/11.2 mm and for the 100% replacement alternative, RCA 0/4, 0.5/4 and 8/11.2 mm are shown in . Sieve analysis performed according to SS EN 933-1. RCA 0/8 mm has a coarser grading than natural sand 0/8 mm. Matching the grading curve of RCA to that of natural sand 0/8 mm is achieved by gap grading. Following this, the water absorption of RCA in the 50%, 100% concrete mixes is determined for a mixed aggregate fraction by the modified pycnometer method [Citation27]. The water corresponding to this measurement is added right before concrete mixing at the for momentarily pre-soaking the aggregates. The water absorption of aggregates in RAC50, RAC100 correspond to 1.7% and 2.8% of aggregate mass, determined in previous investigations relating to this study [Citation6].
Previous studies related to this research conducted densification of RCA by mechanical pre-processing [Citation17], densification of paste using blast furnace slag [Citation6] to improve concrete properties. This is not pursued in this study.
The concrete mixing uses step-wise addition of mixing water and superplasticizer where 70% of the total amount succeeded by remaining 30% is added with momentary mixing in between. This promotes coating of wetted cement on the already pre-soaked RCA; shown in literature to strengthen RCA-cement paste interface (also called ITZ) while the mixing is on-going [Citation28]. The pre-soaking water amount, mixing process are not considered in the LCA inventory; however, is proof of technical feasibility of concrete recycling as made in our lab investigations [Citation6, Citation17, Citation27].
The compressive strength is measured on three cylinder specimens presented with mean and standard deviation. The differences in strength of the RAC to reference concrete is related to the difference in densities, shown in , depending mostly on the differences between coarse aggregate densities. RAC has aggregates with lower densities as it is based on crushed concrete aggregates having adhered mortar on the surface. The slump flow is measured for the fresh concrete in order to assess workability according to SS-EN 12350-5. It is found that for RAC50 and RAC100, the slump flow decreases placing the new concrete in the immediate lower workability class than the reference concrete. Reduced workability is due to the porous mortar attached to the RCA causing it to absorb a part of the mixing water [Citation27].
Table 2. Compressive strength at 28 days and slump flow of the RC, RAC50 and RAC100.
Using the same amount of cement in each recipe is a deliberate choice to keep the environmental impact of the cement constant. Instead, a lower compressive strength is acknowledged but is still adequate for structural application.
2.2. Life cycle assessment
The goal of this LCA is to compare the environmental impact of RC, RAC50 and RAC100 in the stages shown by dotted line in the concrete lifecycle shown in . This represents product stages A1, A2 according to the standard SS-EN 15978, where A1-raw material supply and A2-transport. The product stage A3 representing manufacture of concrete is considered the same irrespective of aggregate type.
The geographical limit is set to Sweden. Most subcontractors are within the 60 km radius of the supposed production site.
Assumptions, limitations of the LCA study are:
Functional unit is 1m3 solid concrete
Concrete spill is assumed equal for all alternatives and thus not accounted for in this study.
The production of recycled aggregates is not investigated in this study, therefore the environmental impacts arising from RCA production is assumed the same as crushed rock production.
Energy demands of the crusher and economical aspects related to the hiring, operation of crusher is not accounted. [Citation19]
The following categories are studied in this LCA study: Global warming potential (GWP), Ozone depletion potential (ODP) Acidification potential (AP), Eutrophication Potential (EP) Photochemical ozone creation potential (POCP) and Abiotic resources depletion potential (ADP).
2.3. Flowchart and data collection
There are three concrete mixes, RC; RAC50 and RAC100 are studied where two scenarios apply only to RAC50 and RAC100, described in detail in section 2.4. A practical representation of the lifecycle flowchart shown in is shown in , applies for the concrete mix constituents representing RC, RAC50, RAC100 investigated in this study. The stages of aggregate production and mobile crusher represent scenario 1 and 2 available for both RCA alternatives 50%, 100%.
The cement in this study is manufactured by Cementa AB in Skövde. The distance between the cement factory in Skövde and concrete production plant in Hedared is determined using Google Maps to 128 km. The transport takes place with Euro 5 truck of 45 tons load capacity. It is also confirmed that the truck drives back empty. For the cement, the environmental impact of the selected categories is retrieved from EPD [Citation29]. The mixing water comes from own well within the site. There is no EPD for this specific case. It has therefore been assumed that it is equivalent to ordinary Swedish tap water.
Admixture used in all concrete mixes is superplasticizer (Sika HD-100). It is manufactured by Sika Sverige AB in Spånga, Stockholm. The distance between Sika's factory and the plant in Hedared is 433 km. According to the company, the transport takes place with Euro 5 truck. The environmental impacts are received from the EPD for Sika HD-100 [Citation30].
Crushed stone is produced in a stationary crushing plant 18 km away from the concrete plant transported with Euro 4 truck. Environmental impacts of the production of this material is gathered from EPD [Citation31]. The EPD includes the environmental impacts of clearing the vegetation, blasting the rock, the internal transport, crushing and sieving, and loading for transport. The natural sand is extracted in Örsås in Svenljunga quarry which is located 59 km from the concrete manufacturing plant in Hedared. At the time of writing this paper, no EPD for this product was developed, therefore results are taken from global average in LCA software.
Whether RCA is produced using stationary or mobile crushing plant, it is assumed that the process of crushing and sieving the concrete waste is similar to the process for crushed stone except the blasting component. Therefore, LCA in this study uses data from crushed stone. During the impact analysis of RCA production, a cut-off rule is applied such that the impacts from the production of parent concrete is not carried over to RCA production, also made in previous research [Citation9]. There is no impact allocation method used since the RCA is the sole product involved in the processes analyzed in this study. The environmental impacts are retrieved from the SimaPro 8 software with the Ecoinvent 3.0 database. The impact assessment method is derived from EPD in accordance with SS-EN 15804:2012, standard prescribing rules for EPD of construction products.
2.4. Two different transport scenarios for RCA
In this study, two scenarios are studied those found realistic for most pre-fab element sites in Sweden. Scenario 1 considers that the concrete waste stored in the pre-fab element site, is transported to the aggregate crushing plant, also producing RCA. The ready RCA fractions are then transported back to the pre-fab element site. It is estimated that 1 m3 of concrete waste gives 0.62 m3 aggregates of the fractions 0/100 mm [Citation32]. Recovery factor of 0.62 is therefore used to calculate the amount of concrete waste needed to produce desired fractions. Scenario 1 for RAC100 and RAC50 are shown in and respectively denoted by RAC100-1, RAC50-1. This is a scenario corresponding to moderate and small amount of concrete waste, mostly suitable for partial RCA replacement such as RAC50.
Figure 4. Flowchart for RAC100 Scenario 1 (RAC100-1) The production of RCA takes place at crushing and 233 recycling plant.
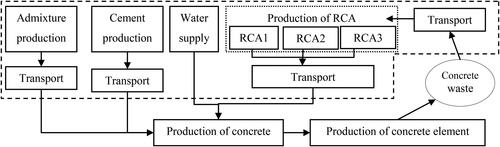
Scenario 2, considers a mobile crushing plant installed on site to conduct in-situ crushing, see and , denoted by RAC100-2, RAC50-2. In this scenario, the transports of concrete waste and RCA is avoided. The transport of mobile crushing plant (MCP) to concrete factory is not included in the study. Using MCP is only economically viable for crushing large waste quantities. One major Swedish company suggests that using MCP is cost efficient for quantities of at least 30 000 metric ton per month [Citation33]. The rent, energy and capacity of the mobile crushing plant used for is assumed as same as for crushed stone from data available in EPD [Citation31].
2.5. Circularity indicator, c
This study evaluates a circularity indicator for RC, RAC50 and RAC100. For this purpose, there is a need for a method to calculate the circularity of these three products. There is, however, no standardized method for calculating product circularity; a review of different circularity indices at product level is made and resulted in a choice of a circular index recommended by Linder et al [Citation24]. This product circularity, denoted c, is based on the economic value of materials of the product. A product tree for RAC100 has been created to illustrate how c is applied to our case, see . The total value of the product vtotal and circularity cRAC is derived from the values contributed by materials constituting the product such as cement, aggregates constituting concrete product. This indicates a bottom-up procedure for calculating vtotal and cRAC of a concrete product. All the costs that arise from processing a material of a product are called in this paper value of activities, Δv. After a material is processed, it gets new value. The new value is equal to the value of activities, Δv added to the original value, v, (new value = v + Δv).
Figure 8. Product tree for RAC100, v: value of material, Δv: value of activities and c: circularity index.
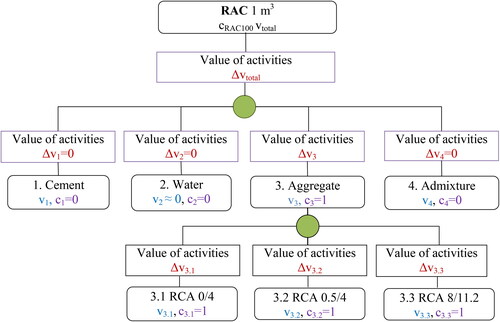
Circularity index of RAC, cRAC is calculated aaccording to Equationequation 1(1)
(1)
(1)
(1)
Where
(2)
(2)
(3)
(3)
The value of cement, natural sand, crushed stone and admixture are received from the company, see . The value of RCA is estimated using current market price. The value of water is estimated from the costs from the pumping of water, in this case well water pumped by electric pump. The pump at full effect (0.4 kW) has the capacity to supply 270 l water per minute, current electricity price in Borås region is 0.57 SEK per kWh, giving total cost of less than one hundredth of SEK and is therefore neglected from the calculation.
Table 3. Value of material and activities are expressed SEK/m3 concrete.
Cement is delivered directly to a silo and as no cement replacement happens, no activity is registered on the cement itself. Aggregates are transported with conveyor belt and machine to the mixing station taking 2.5 min to transport aggregates required for 1 m3 of concrete. The company has estimated the cost of this activity to be 5.56 SEK/min. The only activity when all materials are combined is the mixing of the concrete. The mixing of the concrete takes 15 min per m3. Basic wage of 333.80 SEK/h gives a cost of 83.45 SEK per one m3 concrete. This value represents the Δvtotal for both RAC and NRAC.
Cement, natural sand, crushed stone, admixture and water are assumed to contain no recycled parts, thus their c = 0. That in turn means reference concrete RC has a circularity index zero. The aggregate fractions in the recycled concrete alternatives are all originating from re-circulated concrete except for the coarse fractions in the RAC50. Therefore, these aggregates get a circularity index 1 as shown in .
The price of RCA fractions are high compared to the crushed stone. This is due to the fact that there is no regular supply chain for recycling of concrete in Sweden. It is also a scarcity of continuous supply of concrete waste to produce RCA, thus the production cost per m3 becomes high.
3. Results
From a logistics point of view, Scenario 1 is considered for moderate and small amount of concrete waste to be transported for crushing as in the case of RAC50. Scenario 2 with mobile crusher requires continuous supply of concrete waste and is therefore more suitable for RAC100. LCA has been assessed though for both alternatives and both scenarios to account for real-cases when the cost for short transportation distance is equivalent with the rent costs of a mobile crusher. Thus, by cross-examining alternatives and scenarios it makes possible to vary transport distances and mobile crusher costs correspondingly to real life situations.
3.1. Life cycle impact assessment
Environmental impacts of RC, RAC50 and RAC100 are discerned for the product stages raw materials and transportation respectively for all impact categories. For example, the calculation of total GWP arising from the raw materials stage is shown for the concrete mixes in acquired by multiplying GWP/kg of constituent with corresponding amount as per mix recipe in . The data on GWP of individual constituents are acquired from relevant EPDs, databases mentioned in section 2.3.
Table 4. Calculation of GWP for reference, RAC mixes with 50%, 100% replacement – Raw material stage.
The calculation of total GWP from transportation stage is shown for the RC, RAC concrete mixes in . The GWP of each mix constituent represented as kg CO2,eqv per tonne of weight and per kilometer is based on the transportation vehicle used, described in section 2.3; for example, 0.0847 kg CO2 eq/(t.km) for cement for Euro5 truck, 45 tons capacity. By multiplying this constant with the transportation distance 128 km and recipe amount 490 kg/m3, gives 5.31 kg CO2 eq/m3. The transportation distances for RCA, concrete waste are varied based on scenario 1,2 which represents transport to crushing, crushing on-site respectively.
Table 5. Calculation of GWP for reference, RAC mixes with 50%, 100% replacement – Transportation stage.
The results for all impact categories highlighting influence of aggregate, transportation with total including cement, water and admixtures is shown in and . This is calculated for reference and recycled concrete of 50%, 100% replacements and for both logistics scenarios of RCA crushing- scenario 1 with transportation to crushing location and scenario 2 with mobile crusher on-site.
Table 6. Result of Life cycle impact assessment, LCIA – Scenario 1.
Table 7. Result of Life cycle impact assessment, LCIA – Scenario 2.
In all environmental impact categories, all RAC alternatives for scenario 2 are more environmentally beneficial. For larger volumes of concrete waste such as in RAC100 it is more environmentally beneficial to own a mobile crusher than transport to a crushing site even if it is at a reasonable distance of 18 km from pre-fab element site. That is because RAC50-2 has shorter transport of aggregates, owing to the use of mobile crushing plant. Thus, RCA replacement amount and transportation distance that influences the total environmental impact for the RAC alternatives.
The impacts of RAC50 and RAC100 for both scenarios in categories GWP and ADP are similar to the impacts of RC, see . Of all categories, significant differences between RAC alternatives and reference concrete are only seen in the ODP, because of dominating impact from transport. Generally observed that RAC alternatives independent of scenarios give lesser total impacts on all categories.
Figure 9. Total LCIA of RAC100-1, RAC100-2, RAC50-1 and RAC50-2 expressed relative to reference concrete RC.
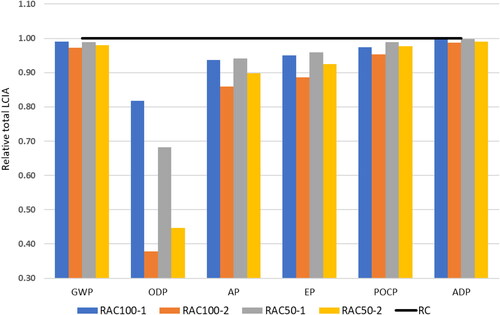
The cement contributes to over 90% to five of six impact categories assessed in this study for global warming potential and abiotic depletion potential. A replacement of cement with secondary cementitious materials such as glass or slag would reduce the cement contribution and beneficially influence the total impact of each alternative. The authors in their previous research have investigated the same recycled concrete recipe with cement replacement such as blast furnace slag replacing 30% of the Portland cement by weight [Citation34]. For a concrete with 100% RCA, the results showed the same compressive strength and workability as reference concrete. With the technical feasibility demonstrated on a lab scale, secondary cement materials maybe regarded as the input that can significantly influence the environmental impact results. Due to the lack of sufficient data on the blast furnace slag investigated, this study analyzes the influence on GWP impact category alone. The Portland cement stands for 704 kg CO2,eqv/ton, slag comparatively has a more modest climate footprint of 30 kg CO2,eqv/ton [Citation35]. The CO2,eqv emissions from cement alone per cubic meter of reference concrete with 100% Portland cement is 344 kg CO2,eqv/m3 (corresponding to cement content 490 kg/m3). When slag replaces 30% of the cement weight, the GWP reduces to 255 kg CO2,eqv/m3; implying at least 25% reduction in GWP resulting in a climate optimized concrete.
Reference concrete and RAC alternatives have same water-cement ratio, constant amounts of water, cement and admixture. This means that the reduction in impacts of RAC compared to reference arises from aggregate, transport activities, seen in for categories GWP, ADP. Thus, RCA substitution in both scenarios reduces the contribution of aggregates to environmental impacts GWP and ADP, interpreted as less CO2 emissions and less extraction of raw material thus conserving nature.
Figure 10. Contribution of aggregates, transportation to a) Global warming potential b) Abiotic resources depletion potential.
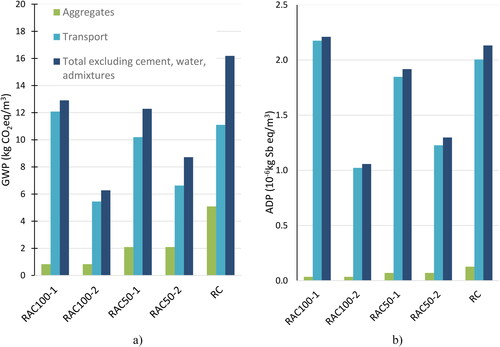
and show contribution of aggregates and transports respectively for different scenarios of RAC100 and RAC50 expressed relative to the contribution of RC. Aggregates contribution to environmental impact are less for RAC alternatives for all categories, RAC100 showing the least impact.
Figure 11. Aggregates contribution to the environmental impacts of different categories expressed relative to RC.
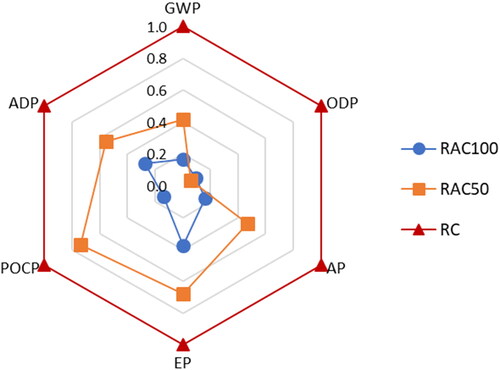
The contribution of transport to all RAC alternatives for all categories in scenario 2 are the lowest as a mobile crusher is placed at the pre-fab element site and there is only internal transportation. The most impact from transport in all categories comes from RAC100 scenario 1 even compared to reference concrete, RC. This is because to replace RCA in reference concrete the recipe was modified to contain 3 aggregate fractions instead of 2. As these fractions are transported separately to site it means that transportation has to be arranged for an extra fraction compared to the reference concrete. This maybe the case for many recipes where aggregate replacement happens with RCA due to experimental results showing that for same workability modification of fractions is required [Citation27, Citation36].
3.2. Product circularity
Circularity of RC is equal to zero as no recycled material is included. The circularity of RAC100 and RAC50 is calculated to 0.6 and 0.5 respectively according to equations in chapter 2.4.
In research related to concrete recycling, efforts are made to keep cement content constant in recipes while improvements in concrete quality are brought forth by quality improvements of recycled aggregates or by partly replacing cement with supplementary cementitious materials. Of this reason, the results of LCIA for all categories excepting for ODP are close to each other. For a better discernment of different concrete alternatives, the circularity index is related to the LCIA results.
3.3. Relation between LCIA and c
Results of this study show that the environmental impacts have noticeably reduced for impact categories such as GWP, ODP and AP when recycled aggregates completely replace virgin aggregates in concrete. Especially when the transportation distances are minimum, as in scenario 2. Besides the influence of transportation, which is a well-recognized environmental hotspot for concrete recycling [Citation37], our results show that the concrete mix generally presents reductions in environmental impact when the share of recycled materials increases. This is apparent in for RAC100-2 (scenario 2); the figures show relationship between circularity indicator and impacts for GWP, ADP respectively.
Figure 13. Relation between circularity index, LCI categories a) GWP b) ADP for reference and RAC mixes.
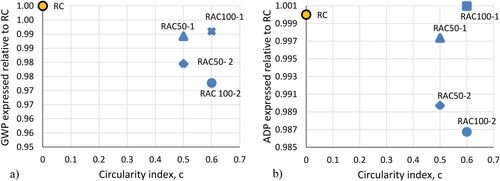
This understanding is consistent with the findings of Linder et.al [Citation38] who study 18 different Swedish products to show a statistically significant relationship between circular materials and LCI categories. They show that products with circular materials have reduced environmental impact compared to a reference linear product across all impact categories. There is a visible trend in where the differences in environmental impacts between logistical scenarios 1,2 are reducing as circularity index reduces. Since the circularity index with increasing RCA replacement percentages, this means that differences in environmental impacts between alternative scenarios are more distinguishable for higher RCA replacements. The differences would diminish further in the case of lower RCA replacement percentages, making it more challenging to determine most favorable scenario. In such a case, the circularity index is a useful tool with a resolution of 0.1 compared to GWP which has 0.01, see .
Combination of LCIA and circularity index may help optimize recycling solutions with regard to logistics, as the LCA categories are largely influenced by transportation. For each raise of the circularity index, it will have a positive impact on the environment as it leads to less extracted material, less waste and landfilling but maybe counteracted by larger transportation distances. Further investigations made on different concrete recipes may show that c can be used as a reliable proxy in place of tedious LCA evaluations.
4. Conclusions
This study analyses concrete recycling from the perspectives of technical, environmental impacts and product circularity. LCA is used to evaluate environmental impacts arising from alternative logistical scenarios related to the crushing and transportation of RCA. The circularity index calculated for concrete with different RCA replacement ratios effectively measures amount of recirculated material in a product usable in sustainability reporting.
The cement and transportation are dominant factors for all categories GWP, AP, EP, POCP and ADP. Cement amount is constant for reference concrete, RC and recycled aggregate concrete, RAC 50% and 100% replacement alternatives. As cement is the driving factor in environmental impacts in concrete production, a constant cement content in RAC concrete sets focus on the positive effects of concrete recycling.
After cement, it is the transport, which contributes largest to the total GWP; however, with RCA replacements the contribution of aggregate reduces by half.
Experimental results show possibility of achieving recycled concrete with 100% RCA when 30% of the Portland cement by weight is replaced with secondary cementitious material- blast furnace slag. Recycled concrete shows significant reduction in GWP compared to reference where CO2,eqv emissions reduced by 25% due to cement replacement.
Transport has significant contribution to the total impact in ODP category for all alternatives RC, RAC50 and RAC100. By replacing aggregates with RCA 50% or 100%, ODP contribution from aggregates significantly decreases. However, replacing natural aggregates with RCA leads to longer transport distances, the environmental benefits of RAC over RC will be lost.
Setting up mobile crushing plant is a viable solution for shortening transport distance given constant supply and large volumes of concrete waste. Sending the concrete waste to stationary plant is a viable solution for small quantities of wastes but generates higher environmental impacts for longer transportation distances. Therefore, the amount of waste available is pointing out best scenario.
Independent of the amount of concrete waste, RAC concrete combined with the inclusion of supplementary cementitious materials, which originate from other industrial supply chains like glass waste and slag can bring on improvements in both environmental and circularity aspects.
Increasing circularity index with increased replacement of aggregates will lead to environmental benefits by reducing raw material extraction, reducing waste and landfilling maybe counteracted by longer transportation distances.
Combining circularity index with LCA will make possible optimization of recycling process with regard to amount of recycled material and logistics respectively.
Acknowledgements
We would like to thank Hedared Sand och Betong AB and Gunnar Ivarson’s Foundation.
Disclosure statement
No potential conflict of interest was reported by the authors.
References
- Riksdagen. Dokument & lagar. 2017. [Online]. Available: https://www.riksdagen.se/sv/dokument-lagar/dokument/svensk-forfattningssamling/lag-19951667-om-skatt-pa-naturgrus_sfs-1995-1667. [Accessed 20. 03. 2018].
- Göransson M. Ersättningsmaterial för naturgrus - kunskapssammanställning och rekommendationer för användningen av naturgrus. SGU: Sveriges geologiska undersökning, Uppsala, December 2015.
- SGU Geological Survey of Sweden. Grus, sand och krossberg 2018. Elanders Sverige AB; 2019.
- SMED, Avfall i Sverige 2014 (Swedish). Swedish Environmental Protection Agency, Stockholm; 2016.
- Sundqvist J.-O. Återvinningsgrad för byggavfall. ASP, Stockholm; 2014.
- Sadagopan M. Recycling of concrete in new structural concrete (PhD thesis), Swedish Centre for Resource Recovery, University of Borås; 2021.
- Fang Y, Chang J. Microstructure changes of waste hydrated cement paste induced by accelerated carbonation. Constr Build Mater. 2015;76:360–365.
- Gastaldi D, Canonico F, Capelli L, et al. An investigation on the recycling of hydrated cement from concrete demolition waste. Cement Concrete Compos. 2015;61
- Marinkovic SB, Ignjatovic I, Radonjanin V. Life-cycle assessment (LCA) of concrete with recycled (RAs). In Handbook of recycled concrete and demolition waste, Woodhead publishing limited; 2013. p. 35.
- Yazdanbakhsh A, Bank L C, Baez T, et al. Comparative LCA of concrete with natural and recycled coarse aggregate in the New York city area. Int J Life Cycle Assess. 2018;23(6):1163–1173.
- Knoeri C, Sanye-Mengual E, Althaus H-J. Comparative LCA of recycled and conventional concrete for structural application. Int J Life Cycle Assess. 2013;18(5):909–918.
- Marinković S, Radonjanin V, Malešev M, et al. Comparative environmental assessment of natural and recycled aggregate concrete. Waste Manag. 2010;30(11):2255–2264. no
- Estanqueiro B, Silvestre J, de Brito J, et al. Environmental life cycle assessment of coarse natural and recycled aggregates for concrete. Euro J Environ Civil Eng. 2018;22(4):429–449.
- Ding T, Xiao J, Tam VWY. A closed-loop life cycle assessment of recycled aggregate concrete utilization in China. Waste Manag. 2016;56(2016):367–375.
- Benhelal E, Zahedi G, Shamsaei E, et al. Global strategies and potentials to curb CO2 emissions in cement. J Cleaner Prod. 2013;51(2013):142–161.
- Hansen TC. Recycling of demolished concrete and masonry. CRC Press; 1992.
- Sadagopan M, Malaga K, Nagy A. Improving recycled aggregate quality by mechanical pre-processing. Materials. 2020;13(19):4342.
- Lee C. How can environmental impacts be evaluated in aggregate production?. Gothenburg, Sweden: University of Gothenburg; 2021.
- M G. Energy and CO2, distribution of flows with crushing. Sweden: Luleå university of technology; 2019.
- Nagy A, Ringsberg H, Sadagopan M. Regional recycling of concrete: pre study. Sweden: University of Borås; 2021.
- Sadagopan M, Malaga K, Nagy A. RE:Concrete- study on recycling of concrete in Sweden. Nordic Concrete Res. 2017;56(1):83–99.
- Cottafava D, Ritzen M. Circularity indicator for residential buildings: addressing the gap between embodied impacts and design aspects. Resour Conserv Recycl. 2021;164:105120.
- Haupt M, Zschokke M. How can LCA support the circular economy?. In 63rd discussion forum on life cycle assessment, Zurich, Switzerland; 2016.
- Linder M, Sarasini S, van L P. A metric for quantifying product-level circularity. J Ind Ecol. 2017;21(3):545–558.
- Wahlström M, zu Castell-Rüdenhausen M, Fruergaard Astrup T, et al. Strategies and methods for implementing CE in construction activities in the Nordic countries: supporting cases. Copenhagen: Nordic council of ministers; 2021.
- Swedish Construction Federation, Corporate sustainability reporting - Guidance for small and medium industries in construction sector. Swedish Construction Federation, Stockholm, 2018.
- Sadagopan M, Malaga K, Nagy A. Modified pycnometer method to measure the water absorption of crushed concrete aggregates. J Sustain Cement-Based Mater. 2020;9(5):259–269.
- Tam VWY, Gao X, Tam C, et al. New approach in measuring water absorption of recycled aggregates. Constr Build Mater. 2008;22(3):364–369.
- Cementa AB. HeidelbergCement Group. Byggcement Sk;vde, 29. 10. 2014; 2014 [Online]. Available: https://www.cementa.se/sv/byggcement-skovde. [Accessed 10 11 2018].
- Institute for Construction and Environment in Germany (IBU). Sika EPD, 14. 9. 2015. [Online]; 2015. Available: https://swe.sika.com/sv/group/sustainability/ladda-ner-dokument/EPD.html?page=5. [Accessed 10 11 2018].
- International EPD® System. Environmental Product Declaration for aggregates from the stationary crushing plant Ramnaslätt. NCC Industry AB, Stockholm; 2017.
- Erlandsson M. Miljödata för krossprodukter och naturgrus. Stockholm: IVL Swedish Environmental Research Institute; 2010.
- NCC. Mobila krossar; 2019. [Online]. Available: https://www.ncc.se/ballast/mobila-krossar/. [Accessed 15 02 2019].
- Sadagopan M, Malaga K, Lundin M, et al. Effects of slag addition and mechanical pre-Processing on the properties of recycled concrete in terms of compressive strength and workability. Nordic Concrete Res. 2021;64(1):11–29.
- Thomas Cement. Technical information - Slag Bremen; 2014. [Online]. Available: https://docplayer.se/16583877-Teknisk-information-slagg-bremen-historik-och-ursprung.html. [Accessed 2021].
- M NA. Properties of concrete. 5th ed. Pearson Education; 2013.
- De Schepper M, Van den Heede P, Van Driessche I, et al. Life cycle assessment of completely recyclable concrete. Materials (Basel). 2014;7(8):6010–6027.
- Linder M, Boyer RH, Dahllöf L, et al. Product-level inherent circularity and its relationship to environmental impact. J Cleaner Prod. 2020;260:121096.