Abstract
Metabolic engineering allows the production of a variety of high-value chemicals in heterologous hosts. For example, itaconic acid (IA) has been produced in several microorganisms, such as Escherichia coli, Aspergillus niger, and Synechocystis sp. through the expression of cis-aconitate decarboxylase gene (cad) from Aspergillus terreus. Recently, we showed that inactivation of the isocitrate dehydrogenase gene and overexpression of the aconitase gene dramatically enhanced the production levels of IA in E. coli expressing cad. Furthermore, we demonstrated that it is possible to produce IA directly from starch by engineered E. coli that additionally expresses the α-amylase gene from Streptococcus bovis. In this study, we sum up our findings regarding the challenges of IA production in E. coli.
Metabolic engineering has opened a novel avenue for producing chemicals of interest, including biofuels and high-value commodities in heterologous hosts.Citation1 In particular, itaconic acid (IA), a promising vinyl monomer that is industrially produced by Aspergillus terreus,Citation2 has recently been produced in Escherichia coli,Citation3-6Aspergillus niger,Citation7–9 and Synechocystis sp,Citation10 which were engineered to express the cis-aconitate decarboxylase gene (cad)Citation11 from A. terreus. Vinyl monomers such as IA are promising building blocks for synthetic polymers, because of their radical polymerizability.Citation12-14 In general, it takes a relatively long time to cultivate fungi for itaconic acid production. Compared to fungi, E. coli seems to be a more suitable producer of itaconic acid because of its high growth rate; furthermore, gene manipulation of E. coli is easier than that of fungi.Citation6 Additionally, E. coli has the potential to produce dicarboxylic acids, such as succinic acid, malic acid, and itaconic acid.Citation6 Li et al. demonstrated that E. coli cells containing the cad gene produced 0.08 g/L of IA after overnight growth in Luria–Bertani (LB) medium.Citation3 More recently, Vuoristo et al. introduced the citrate synthase and the aconitase genes (acn) from Corynebacterium glutamicum in E. coli expressing cad and inactivating the phosphate acetyltransferase and the lactate dehydrogenase genes.Citation5 Cells thus modified produced up to 0.69 g/L of IA when grown in mineral medium supplemented with glucose, in an aerobic bioreactor.
Recently, we have reported similar efforts. Okamoto et al. overexpressed acn and inactivated the isocitrate dehydrogenase gene (icd) in E. coli expressing cad. The engineered E. coli produced 4.34 g/L of IA when grown for 105 h in LB medium supplemented with glucose, under pH-stat conditions.Citation4 Similarly to what observed by Vuoristo et al., we did not detect Cad activity in the engineered E. coli at 37°C.Citation5 In our study, cad was cloned into the IPTG-inducible pETHis vectorCitation15 containing the PT7 promoter, and protein expression was carried out in E. coli BW25113 (DE3) pLysS cells at 20°C or at 37°C. Cells were induced with IPTG for 18 h in 2 mL of LB medium. The presence of the pLysS plasmid considerably improved the expression levels of Cad; other expression systems bearing the inducible promoters PLAC and PBAD were unable to yield acceptable quantities of Cad. Although cells grew slower at 20°C than at 37°C, we obtained approximately 1.0 g of cells from each liter of culture. HPLC analysis revealed that cells grown for 18 h at 20°C produced 0.03 g/L of IA, suggesting that cad expression in a heterologous host promote production of IA. However, cells grown at 37°C did not produce IA. To further investigate this result, we performed SDS-PAGE. While the Cad protein (54.0 kDa) was present in the soluble fraction of cells grown at 20°C, it was mainly found in the insoluble fraction when expression was performed at 37°C (). Soluble Cad purified from recombinant E. coli shows activity at 37°C, but it is rather unstable.Citation11,16 It is likely that the protein has low stability in vivo and that it forms insoluble aggregates during expression at 37°C, thus, losing its activity. The result shown in suggests that expression of Cad at a moderate temperature, such as 20°C to 30°C, might be suitable for IA production in E. coli. To facilitate protein folding at 37°C, we co-expressed Cad with the molecular chaperons DnaKJ, GrpE, and GroESL, following a published protocolCitation17; however, this attempt was unsuccessful.
Figure 1. SDS-PAGE analysis of the Cad protein in E. coli. E. coli BW25113 (DE3) pLysS harboring pETHis (control) or pETHis-cad (cad) were grown at 20°C or 37°C in 2 mL of LB medium after 0.1 mM IPTG induction. After 18 h of incubation, cells were harvested, disrupted, and separated into soluble (S) and insoluble (IS) fractions. SDS-PAGE analysis was performed on a 12.5% polyacrylamide gel. 10 μg of sample from the soluble and from the insoluble fraction (one-fourth of precipitants) were loaded. The corresponding molecular masses (in kDa) of protein markers (M) are indicated on the left. The expressed Cad protein (54.0 kDa) is indicated by the arrow.
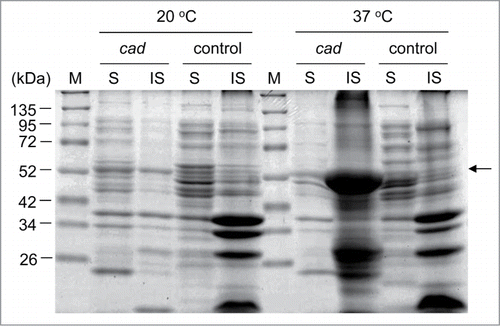
Figure 2. Plot of growth temperature (°C) versus itaconic acid (IA) production (g/L). The overnight cultures of recombinant E. coli (open squares, BW25113 (DE3) (pLysS, pETHis-cad); closed squares, BW25113 (DE3) (Δicd, pLysS, pETHis-cad)) cultivated in LB medium at 37°C were inoculated in fresh 2 mL of LB medium to an OD600 of 0.1. The cultures were subsequently incubated at 10, 20, 25, 28, 30, 33, 35, or 37°C for 18 h. When the cultures reached an OD600 of 0.4–0.6, IPTG was added at a final concentration of 0.1 mM to induce cad expression. The IA production in the resulting strains was analyzed after growth with IPTG induction.
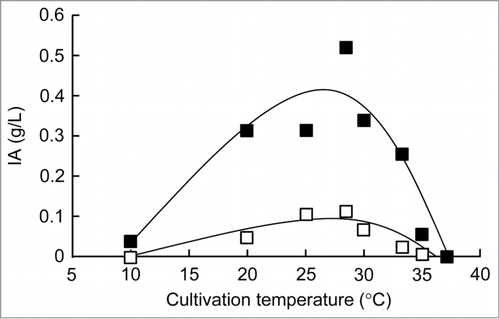
In our study, we also observed an increase of the concentration of acetic acid in the cell culture upon acn overexpression (9.04 g/L after 105 h of growth).Citation4 Overexpression of acn likely suppresses the activity of the glyoxylate shunt, resulting in an increase of acetic acid concentration derived from acetyl-CoA. To further optimize the production of IA, we inactivated both the acetate kinase gene (ack) and the pyruvate oxidase gene (pox), which are involved in acetic acid production, in E. coli expressing cad. In this study, we introduced pJAK12-cadCitation10 into the engineered E. coli to express cad. Cells bearing these modifications grew slower than the control cells (), but we observed similar Cad activity in E. coli BW25113 (pJAK12-cad) (9.9 U/g-protein) and in BW25113 (Δack, Δpox, pJAK12-cad) (10.3 U/g-protein). As expected, inactivation of ack and pox resulted in nearly 1.5-times higher levels of IA compared to the control (). These findings indicate that ack and pox inactivation enhances IA production in cad-expressing E. coli.
Figure 3. (A) Cell growth and (B) production of itaconic acid (IA) in engineered E. coli. Recombinant E. coli cells (closed circles, BW25113 (pJAK12-cad); closed squares, BW25113 (Δack, Δpox, pJAK12-cad)) grown in LB for 18 h at 37°C,were inoculated in 2 mL of fresh LB medium supplemented with 3% glucose to an OD600 of 0.1. The cultures were subsequently incubated at 28°C for an appropriate time. When the cultures reached an OD600 of 0.4–0.6, IPTG was added at a final concentration of 0.1 mM to induce cad expression. Induction was carried out for 24 h before analyzing the IA production in the resulting strains. Each experiment was performed in duplicates, and the average was represented with error bars indicating standard deviations.
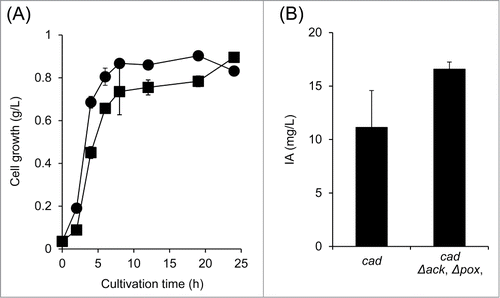
If IA could be produced by E. coli using starch as a substrate instead of glucose, production costs would be considerably lower. To reach this goal, Okamoto et al. expressed the α-amylase gene (amy) from Streptococcus bovis in E. coli expressing cad and inactivated icd.Citation6 In this report, we constructed a new expression vector, pGV3, which is suitable for cell surface display of heterologous proteins in E. coli. Cell surface display represents a unique expression system to decorate protein on the cell surface.Citation18 pGV3 consists of a fragment of the pVUB3 expression vector,Citation19 which contains lacIq’, PTRC, oprI’, and rrnBT1T2, and a fragment of the pGBM1 cloning vector,Citation20 which contains the Spcr marker and the pSC101 origin. The amy gene was cloned into pGV3, resulting pGV3-SBA.Citation6 The OprI’-based fusion proteins were anchored into the outer membrane of E. coli. pGV3 is compatible with plasmids containing the origins for ColE1 and p15A. Similar to pGV3, we constructed pAV3 from a fragment of pVUB3 and from a fragment of the pACYC184 cloning vector.Citation21 The pAV3 plasmid contained the Cmr marker and the p15A origin. The amy gene was cloned into pAV3, resulting in pAV3-SBA. When E. coli cells containing pAV3-SBA were induced, they expressed the OprI’-Amy fusion protein, as confirmed by SDS-PAGE. Amylase activity was also confirmed in the E. coli cells expressing amy. These results suggest that pAV3 is suitable for cell surface display of heterologous proteins in E. coli, and that it is compatible with plasmids containing ColE1 and pSC101. The technique constructed herein for direct production of value-added chemicals from biomass by E. coli expressing biomass-degrading enzymes may reduce total incurred costs, including the production costs due to carbon source.
In our study, we also showed that E. coli cells inactivating icd, which are auxotrophic for glutamate, showed no growth in a minimal medium lacking glutamate, but that they recovered upon addition of 0.5% glutamate.Citation6 shows cell growth and production levels of IA in E. coli BW25113 (DE3) (Δicd, pLysS, pETHis-cad, pGV3-SBA) grown for 78 h in 2 mL of M9 minimal medium with and without 0.5% glutamate, 0.4% glucose, or 0.4% starch. Despite the fact that the engineered E. coli showed hardly any growth without glutamate, the levels of IA increased, indicating that cad-expressing E. coli showed non-growth associated production of IA. This suggests that carbon yield during IA production in E. coli can be improved by using resting E. coli cells.
Table 1. Numbers of cells yield (X), itaconic acid (IA) production (P), and specific product yield (YP/X) from engineered E. coli grown for 78 h in different culture mediaa
The engineered E. coli produces IA less efficiently than A. terreus. However, itaconic acid production in E. coli can be further enhanced by metabolic approach, such as inactivation of undesired metabolic pathways involved in ethanol and lactate production. Further research and a deeper understanding of the metabolic properties of E. coli might indicate a novel approach to improve IA production in this organism.
Disclosure of Potential Conflicts of Interest
No potential conflict of interest was disclosed.
References
- Hollinshead W, He L, Tang YJ. Biofuel production: an odyssey from metabolic engineering to fermentation scale-up. Front Microbiol 2014; 5:344; PMID:25071754; http://dx.doi.org/10.3389/fmicb.2014.00344
- Okabe M, Lies D, Kanamasa S, Park EY. Biotechnological production of itaconic acid and its biosynthesis in Aspergillus terreus. Appl Microbio Biotechnol 2009; 84:597-606; PMID:19629471; http://dx.doi.org/10.1007/s00253-009-2132-3
- Li A, van Luijk N, ter Beek M, Caspers M, Punt P, van der Werf M. A clone-based transcriptomics approach for the identification of genes relevant for itaconic acid production in Aspergillus. Fungal Genet Biol 2011; 48:602-11; PMID:21324422; http://dx.doi.org/10.1016/j.fgb.2011.01.013
- Okamoto S, Chin T, Hiratsuka K, Aso Y, Tanaka Y, Takahashi T, Ohara H. Production of itaconic acid using metabolically engineered Escherichia coli. J Gen Appl Microbiol 2014; 60:191-7; PMID:25420424; http://dx.doi.org/10.2323/jgam.60.191
- Vuoristo KS, Mars AE, Sangra JV, Springer J, Eggink G, Sanders JP, Weusthuis RA. Metabolic engineering of itaconate production in Escherichia coli. Appl Microbiol Biotechnol 2015; 99:221-8; PMID:25277412; http://dx.doi.org/10.1007/s00253-014-6092-x
- Okamoto S, Chin T, Nagata K, Takahashi T, Ohara H, Aso Y. Production of itaconic acid in Escherichia coli expressing recombinant α-amylase using starch as substrate. J Biosci Bioeng 2015; 119:548-53; PMID:25468427; http://dx.doi.org/10.1016/j.jbiosc.2014.10.021
- Li A, Pfelzer N, Zuijderwijk R, Punt P. Enhanced itaconic acid production in Aspergillus niger using genetic modification and medium optimization. BMC Biotechnol 2012; 12:57; PMID:22925689; http://dx.doi.org/10.1186/1472-6750-12-57
- Blumhoff ML, Steiger MG, Mattanovich D, Sauer M. Targeting enzymes to the right compartment: Metabolic engineering for itaconic acid production by Aspergillus niger. Metab Eng 2013; 19:26-32. PMID:23727192; http://dx.doi.org/10.1016/j.ymben.2013.05.003
- van der Straat L, Vernooij M, Lammers M, van den Berg W, Schonewille T, Cordewener J, van der Meer I, Koops A, de Graaff LH. Expression of the Aspergillus terreus itaconic acid biosynthesis cluster in Aspergillus niger. Microb Cell Fact 2014; 13:11. PMID: 24438100; http://dx.doi.org/10.1186/1475-2859-13-11
- Chin T, Sano M, Takahashi T, Ohara H, Aso Y. Photosynthetic production of itaconic acid in Synechocystis sp. PCC6803. J Biotechnol 2015; 195:43-5; PMID: 25554635; http://dx.doi.org/10.1016/j.jbiotec.2014.12.016
- Kanamasa S, Dwiarti L, Okabe M, Park EY. Cloning and functional characterization of the cis-aconitic acid decarboxylase (CAD) gene from Aspergillus terreus. Appl Microbiol Biotechnol 2008; 80:223-9; PMID:18584171 http://doi.org/10.1007/s00253-008-1523-1
- Ishimoto K, Arimoto M, Okuda T, Yamaguchi S, Aso Y, Ohara H, Kobayashi S, Ishii M, Morita K, Yamashita H, Yabuuchi N. Biobased polymers: synthesis of graft copolymers and comb polymers using lactic acid macromonomer and properties of the product polymers. Biomacromolecules 2012; 13:3757-68; PMID:22984930 http://doi.org/10.1021/bm301212a
- Okuda T, Ishimoto K, Ohara H, Kobayashi S. Renewable biobased polymeric materials: facile synthesis of itaconic anhydride-based copolymers with poly(L-lactic acid) grafts. Macromolecules 2012; 45:4166-74; http://dx.doi.org/10.1021/ma300387j
- Sano M, Chin T, Takahashi T, Ohara H, Aso Y. A simple TLC-densitometric method for the quantification of acrylic acid in aqueous solutions. J Planar Chromatogr 2015; 28:12-16; http://dx.doi.org/10.1556/JPC.28.2015.1.2
- Chen BP, Hai T. Expression vectors for affinity purification and radiolabeling of proteins using Escherichia coli as host. Gene 1994; 139:73-5. PMID: 8112591; http://dx.doi.org/10.1016/0378-1119(94)90525-8
- Dwiarti L, Yamane K, Yamatani H, Kahar P, Okabe M. Purification and characterization of cis-aconitic acid decarboxylase from Aspergillus terreus TN484-M1. J Biosci Bioeng 2002; 94:29-33; PMID:16233265; http://dx.doi.org/10.1016/S1389-1723(02)80112-8
- Nishihara K, Kanemori M, Yanagi H, Yura T. Overexpression of trigger factor prevents aggregation of recombinant proteins in Escherichia coli. Appl Environ Microbiol 2000; 66:884-9; PMID:10698746; http://dx.doi.org/10.1128/AEM.66.3.884-889.2000
- Lee SY, Choi JH, Xu Z. Microbial cell-surface display, Trends Biotechnol 2003; 21:45-52; PMID: 12480350; http://dx.doi.org/10.1016/S0167-7799(02)00006-9
- Cote-Sierra J, Jongert E, Bredan A, Gautam DC, Parkhouse M, Cornelis P, De Baetselier P, Revets H. A new membrane-bound OprI lipoprotein expression vector. High production of heterologous fusion proteins in gram (-) bacteria and the implications for oral vaccination. Gene 1998; 221:25-34; PMID:9852946; http://dx.doi.org/10.1016/S0378-1119(98)00437-5
- Manen D, Pougeon M, Damay P, Geiselmann J. A sensitive reporter gene system using bacterial luciferase based on a series of plasmid cloning vectors compatible with derivatives of pBR322. Gene 1997; 186:197-200; PMID:9074496; http://dx.doi.org/10.1016/S0378-1119(96)00702-0
- Chang AC, Cohen SN. Construction and characterization of amplifiable multicopy DNA cloning vehicles derived from the P15A cryptic miniplasmid. J Bacteriol 1978; 134:1141-56; PMID:149110