ABSTRACT
2-Phenylethanol (2-PE) is a high value aromatic alcohol with a rose-like odor that is utilized in the cosmetics and other industries. Although the chemical routes of 2-PE production have been altered by some microbial transformation processes, the poor tolerance to organic solvents of these microorganisms has limited the 2-PE yield. In this study, the stress-tolerant yeast Candida glycerinogenes WL2002-5 showed a 2-PE tolerance to 4 g/l, which is the highest reported to date. Moreover, the 2-PE titer in a batch fermentation from L-phenylalanine reached 5g/l, which is the highest level achieved by fermentation without in situ product recovery. These results suggest C. glycerinogenes WL2002-5 is a robust strain for the bioproduction of 2-PE with potential for commercial exploitation.
Introduction
2-Phenylethanol (2-PE) is an important volatile aromatic alcohol with a rose-like odour.Citation1 It is wildly used in the pharmaceutical industry as a bactericide and also in the food, perfumery, and cosmetics industries to improve character.Citation2,3 At present, most commercial 2-PE is synthesized chemically via Friedel-Crafts acylation.Citation1 However, this produces toxic byproducts that are harmful to health, which is an obvious issue for use in the food and cosmetics industries, and only natural 2-PE extracted from the essential oils of flowers or plants is permitted. However, the scarcity of flowers and plants and the low efficiency of extraction limit the titer of 2-PE produced from natural sources.Citation4 The price of naturally sourced 2-PE is therefore 1000 US$/kg, compared with only 5 US$/kg for chemical synthesized 2-PE.Citation5 Much attention has therefore been paid to 2-PE biosynthesis.
Various microorganisms including bacteria, filamentous fungi and yeast possess the ability to synthesize 2-PE.Citation2,6-10 Among the reported 2-PE producers, yeasts such as Saccharomyces cerevisiae, Kluyveromyces marxianus, and Yarrowia lipolytica are the most efficient strains.Citation8,11, 12 The 2-PE titer of K. marxianus was improved from 0.39 to 0.5 g/l by culture optimization,Citation13 while wild-type Y. lipolytica NCYC3825 produced 1.98 g/l 2-PE in non-optimized batch culture.Citation8 To further increase 2-PE production, genetic strategies have been applied. Overexpressions of ARO8 and ARO10 in S. cerevisiae SPO810 increased the 2-PE titer from 0.85 to 1.1 g/lCitation14. Deletion of ALD3 in the recombinant S. cerevisiae W303-1B improved 2-PE production from 0.11 to 4.8 g/l.Citation15 Although much attention has been paid to the biosynthesis of 2-PE, the titer remains disappointingly low. Unfortunately, 2-PE is toxic to most microorganisms (log P = 1.36),Citation16 which limits its production.Citation1 To reduce the toxicity, in situ product recovery (ISPR) was applied to 2-PE production in K. marxianus CBS 600, and the titer was improved to 26.5 g/lCitation17. However, despite the increased yield, extraction of 2-PE from the organic phase proved uneconomical.Citation18 Bioproduction of 2-PE by a robust and stress-tolerant organism would be a more economically viable and practical approach, and the stress-tolerant yeast S. cerevisiae Ye9-612 has been tested for this purpose.Citation19
Candida glycerinogenes WL2002-5 is another stress-tolerant yeast that can tolerate NaCl to 15 % (w/v) and glucose to 55 % (w/v).Citation20,21 As a ‘generally recognized as safe’ industrial strain, C. glycerinogenes WL2002-5 has been used commercially for the production of glycerol from glucose for over 20 y in China.Citation22 In the present study, the tolerance of C. glycerinogenes WL2002-5 to 2-PE was investigated, and the potential for this organism for 2-PE production was assessed.
Materials and methods
Strains and culture conditions
C. glycerinogenes WL2002-5 and S. cerevisiae W303-1A were stored in our lab and routinely cultivated in yeast extract peptone dextrose (YPD) medium at 30°C. For 2-PE synthesis, overnight cultivated C. glycerinogenes WL2002-5 was incubated in 30 ml defined medium (50 g/l glucose, 5 g/l L-Phe, 5 g/l KH2PO4, 0.5 g/l MgSO4·7H2O, and 0.17 g/l yeast nitrogen base (YNB) without amino acids) in a 250 ml Erlenmeyer flask at 30°C for 50 h.
Analytical methods
The optical density (OD) of cells was determined using a spectrophotometer at 600 nm. For determination of the cell dry weight, fermentation broth was centrifuged at 12,000 × g for 5 min in weighed pre-dried tubes. Cell pellets were washed twice and dried at 105°C to constant weight. The weight difference is equivalent to the cell dry weight per ml. Identification of 2-PE was performed by gas chromatography-mass spectrometry (GC-MS). The culture supernatant was collected by centrifugation at 10,000 × g for 10 min. 2-PE was extracted from the supernatant with pentane:cyclohexane (2:1, v:v) and dried with Na2SO4. The extract was analyzed by GC-MS (Broker SCION SQ, USA) using a pulsed pressure injection of 0.2 μl onto a DB-wax column (30 m × 0.25 mm, 0.25 μm). The helium flow rate was 0.8 ml/min, and the inlet port temperature was 250°C. The oven program started at 80°C for 1 min, followed by an increase of 10°C/min to 230°C, and retention at 230°C for 5 min. Mass detector parameters were as follows: transfer line = 250°C, scan mode range = 33-400 m/z, electron impact ionization = 70 eV.
The 2-PE concentration was determined using 1200 series high-performance liquid chromatography (HPLC; Agilent, USA) equipped with a RP-C18 column (250 mm × 4.6 mm, 10 μm; Ailite, China). The mobile phase was methanol:water (50:50, v/v), the flow rate was 0.7 ml/min, the temperature was 30°C, and the detection wavelength was 260 nm. The glucose concentration was measured enzymatically using a SBA-40E Biosensor.
Batch fermentation
Fresh cultures of C. glycerinogenes WL2002-5 were inoculated into in a 5 l bioreactor (Boxing 5BG, China) containing 3 l of medium (90 g/l glucose, 5 g/l KH2PO4, 4 g/l yeast extract, 0.5 g/l MgSO4·7H2O, 7 g/l L-phenylalanine (L-Phe)). The bioreactor was aerated at 1.5 vvm and stirred at 500 rpm, 30°C for 50 h.
Results and discussion
2-PE tolerance of C. glycerinogenes WL2002-5
To study the tolerance of C. glycerinogenes WL2002-5 toward 2-PE, C. glycerinogenes WL2002-5 and S. cerevisiae W303-1A were cultivated in YPD agar containing various concentrations of 2-PE. As shown in , the cell growth of C. glycerinogenes WL2002-5 was not impacted when the 2-PE concentration was lower than 2 g/l, but it gradually decreased at higher 2-PE concentrations. In comparison, the cell growth of S. cerevisiae W303-1A was almost completely repressed at a 2-PE concentration of 2 g/l. Of note, C. glycerinogenes WL2002-5 colonies were still observed at 4 g/l 2-PE.
Figure 1. Comparison of C. glycerinogenes WL2002-5 and S. cerevisiae W303-1A for 2-PE tolerance on YPD agar. Cells were incubated in liquid YPD medium at 30°C for 18 h and diluted to an OD600 of 1. Cells (2 µL) at a dilution of 10−1, 10−2, 10−3, 10−4, and 10−5 were spotted on solid YPD agar containing different concentrations of 2-PE (0 (control), 1, 2, 3, 3, 4 g/l). Cells were incubated at 30°C.
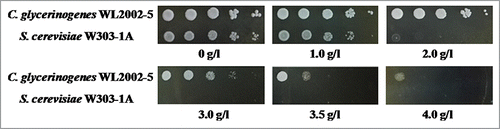
To quantify the difference in tolerance between the organisms, they were cultivated in liquid YPD medium containing 2-PE. As shown in , the cell growth of both strains was reduced slightly at low concentrations of 2-PE. The biomass of C. glycerinogenes WL2002-5 was reduced by 40% compared with a reduction of 75% for S. cerevisiae W303-1A at a 2-PE concentration of 3 g/l. The growth of S. cerevisiae W303-1A was completely repressed at a 2-PE concentration of 3.5 and 4.0 g/l, whereas the biomass of C. glycerinogenes WL2002-5 was 34% and 12% that of the control at these concentrations. These results indicated that C. glycerinogenes WL2002-5 was more tolerant to 2-PE, and was also higher than that previously reported for S. cerevisiae GIV2009, which did not grow at a 2-PE concentration of 4 g/l Citation[23]. As far as we know, C. glycerinogenes WL2002-5 is the most tolerant to 2-PE of any reported strain. To our surprise, the concentration of 2-PE in the broth remained stable at all time points (data not shown), even after 80 h, suggesting there might not be a 2-PE degradation pathway in C. glycerinogenes WL2002-5.
Figure 2. Comparison of C. glycerinogenes WL2002-5 (A) and S. cerevisiae W303-1A (B) for 2-PE tolerance in liquid culture. Cells were incubated in YPD medium at 30°C for 18 h, and diluted to the same concentration. Cells were inoculated into fresh YPD liquid medium containing various concentrations of 2-PE (0 (control), 1, 2, 3, 3.5, 4 g/l), and incubated at 30°C with shaking at 200 rpm. Open square, 0 g/l; solid circle, 1 g/l; open up-pointing triangle, 2 g/l; solid down-pointing triangle, 3 g/l; open diamond, 3.5 g/l; solid left-pointing triangle, 4 g/l.
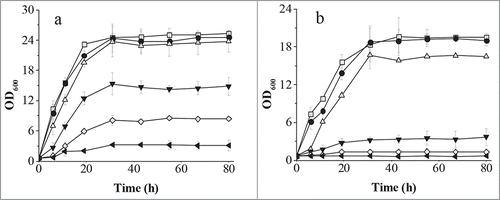
The organic solvent tolerance of yeast is reported to be due to changes in membrane fatty acids and the cell wall and some other factors,Citation24 but the exact mechanisms are complex and remain unknown.Citation25,26 Some global regulatory mechanisms appear to be responsible for organic solvent tolerance in yeast, such as the high-osmolarity glycerol (HOG) pathway.Citation27 The presence of glycerol in the medium also contributes to organic solvent tolerance in yeast.Citation28 We therefore speculate that a high glycerol synthesis capability and efficient HOG pathway in C. glycerinogenes WL2002-5Citation21,29 contribute to its excellent 2-PE tolerance.
Biosynthesis of 2-PE by C. glycerinogenes WL2002-5 using different substrates
In order to assess the 2-PE synthesis capability of C. glycerinogenes WL2002-5, the strain was cultivated in defined medium (see Material and Methods). As shown in , the 2-PE concentration in broth was 2.5 g/l, as determined by GC-MS, suggesting C. glycerinogenes WL2002-5 could synthesize 2-PE. In yeast, there are 2 routes for the biosynthesis of 2-PE from L-Phe: the phenylethylamine (PEA) pathway and the Ehrlich pathway. In the PEA pathway, L-Phe is decarboxylated to PEA by aromatic amino acid decarboxylase and then oxidatively deaminated to phenylacetaldehyde by monoamine oxidase, followed by reduction to 2-PE by alcohol dehydrogenase. In the Ehrlich pathway, L-Phe is transaminated to phenylpyruvate by aminotransferase and then decarboxylated to phenylacetaldehyde by phenylpyruvate decarboxylase, followed by reduction to 2-PE by alcohol dehydrogenase.Citation2 A previous study revealed a high degree of gene similarity (>99 %) between C. glycerinogenes WL2002-5 and Issatchenkia orientalis,Citation30 and genomic analysis indicates the Ehrlich pathway is present in I. orientalis. We therefore predict that the Ehrlich pathway is responsible for production of 2-PE in C. glycerinogenes WL2002-5, and we are currently cloning these genes in our laboratory. Interestingly, the 2-PE concentration was 75 mg/l following cultivation in defined medium without L-Phe (YNB as a sole nitrogen source), indicating the potential presence of a de novo pathway for the synthesis of 2-PE from glucose in C. glycerinogenes WL2002-5.
Culture optimization
In order to improve the production titer of 2-PE, the culture process was optimized based on the defined medium. As shown in , 2-PE accumulation and biomass were higher when the glucose concentration was between 30 and 90 g/l, but lower at a higher glucose concentration (). Increasing the L-Phe concentration from 3 to 11 g/l also diminished cell growth (). In contrast, the 2-PE titer peaked at a L-Phe concentration of 7 g/l but was decreased slightly at a higher L-Phe concentration. Yeast extract is cheap and was tested as a nitrogen source instead of YNB. As shown in , the biomass and 2-PE titer were increased slightly with an increasing concentration of yeast extract, and 2-PE production was improved from 3.2 to 3.6 g/l.
Figure 4. Effect of glucose (A), L-Phe (B), yeast extract (C) concentration, and temperature (D) on 2-PE production and cell growth. Biomass was measured using a spectrophotometer at 600 nm, and the 2-PE titer was determined by HPLC. Open square, 2-PE; solid triangle, OD600.
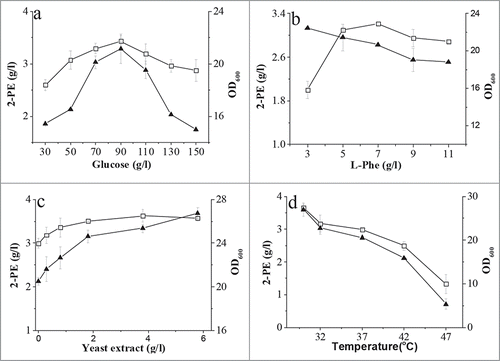
Figure 5. Batch fermentation in a 5 l bioreactor. Solid circle, 2-PE; open square, dry cell weight (DCW); open triangle, glucose.
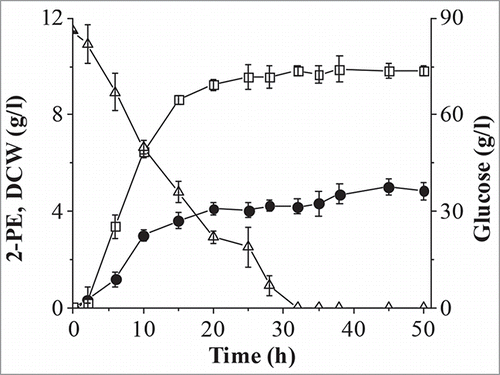
To investigate the effect of temperature on the biosynthesis of 2-PE, C. glycerinogenes WL2002-5 was incubated in optimized medium (90 g/l glucose, 5 g/l KH2PO4, 4 g/l yeast extract, 7 g/l L-Phe, 0.5 g/l MgSO4·7H2O) at different temperatures. As shown in , the biomass and 2-PE titer were significantly reduced with increasing temperature, especially at temperatures above 37°C, and the optimum temperature was 30°C. Even though cell growth was almost completely repressed at 47°C, a 1.5 g/l 2-PE (40% of the maximal titer) was detected in the broth (). In contrast, 2-PE production in the thermotolerant S. cerevisiae Ye9-596 and some other strains was almost completely repressed (<0 .01 g/l) at 40°C33. This result suggested that biosynthesis of 2-PE by C. glycerinogenes WL2002-5 is thermotolerant.
Batch culture
A batch fermentation was carried out to further enhance production of 2-PE from L-Phe (). Glucose was exhausted and the biomass reached stationary phase at 30 h, and accumulation of 2-PE was coupled with cell growth, as reported previously for other organisms.Citation19 The maximum titer of 2-PE was 5.0 g/l at 45 h, corresponding to a conversion rate of 0.71 g/g, which is close to the maximum theoretical bioconversion yield of 0.75 g/g1. This 2-PE titer and bioconversion rate are higher than that reported for the engineered S. cerevisiae W303-1B (4.8 g/l, 0.48 g/g),Citation15 for S. cerevisiae Giv 2009 (2.35 g/l, 0.39 g/g),Citation31 and for other strains.Citation26,31, 32 To the best of our knowledge, the 2-PE titer produced by C. glycerinogenes WL2002-5 is the highest reported to date for a batch culture method without the use of ISPR, indicating that C. glycerinogenes WL2002-5 is an excellent strain for the bioproduction of 2-PE. Attempts to further improve 2-PE production by genetic engineering are ongoing in our group.
Disclosure of potential conflicts of interest
No potential conflicts of interest were disclosed.
Funding
This work was supported by the National High Technology Research and Development Program of China (863 Program, No. 2012AA021201), Natural Science Foundation of China (No. 31570052, 31270080), Natural Science Foundation of Jiangsu Province (No. BK20140138), the Fundamental Research Funds for the Central Universities, and the 111 project (No. 111-2-06).
References
- Etschmann MM, Bluemke W, Sell D, Schrader J. Biotechnological production of 2-phenylethanol. Appl Microbiol Biotechnol 2002; 59:1-8; PMID:12073125; http://dx.doi.org/10.1007/s00253-002-0992-x
- Masuo S, Osada L, Zhou S, Fujita T, Takaya N. Aspergillus oryzae pathways that convert phenylalanine into the flavor volatile 2-phenylethanol. Fungal Genet Biol 2015; 77:22-30; PMID:25797315; http://dx.doi.org/10.1016/j.fgb.2015.03.002
- Stark D, Munch T, Sonnleitner B, Marison IW, Stockar UV. Extractive bioconversion of 2-phenylethanol from L-phenylalanine by Saccharomyces cerevisiae. Biotechnol Prog 2002; 18:514-23; PMID:12052068; http://dx.doi.org/10.1021/bp020006n
- Mei J, Min H, Lü Z. Enhanced biotransformation of l-phenylalanine to 2-phenylethanol using an in situ product adsorption technique. Process Biochem 2009; 44:886-90; http://dx.doi.org/10.1016/j.procbio.2009.04.012
- Hua D, Xu P. Recent advances in biotechnological production of 2-phenylethanol. Biotechnol Adv 2011; 29:654-60; PMID:21601630; http://dx.doi.org/10.1016/j.biotechadv.2011.05.001
- Zhang H, Cao M, Jiang X, Zou H, Wang C, Xu X, Xian M. De-novo synthesis of 2-phenylethanol by Enterobacter sp CGMCC 5087. Bmc Biotechnol 2014; 14:1-7; http://dx.doi.org/10.1186/1472-6750-14-30
- Wani MA, Sanjana K, Kumar DM, Lal DK. GC-MS analysis reveals production of 2-phenylethanol from Aspergillus niger endophytic in rose. J Basci Microb 2010; 50:110-4; http://dx.doi.org/10.1002/jobm.200900295
- Celinska E, Kubiak P, Bialas W, Dziadas M, Grajek W. Yarrowia lipolytica: the novel and promising 2-phenylethanol producer. J Ind Microbiol Biotechnol 2013; 40:389-92; PMID:23443834; http://dx.doi.org/10.1007/s10295-013-1240-3
- Etschmann MM, Huth I, Walisko R, Schuster J, Krull R, Holtmann D, et al. Improving 2-phenylethanol and 6-pentyl-alpha-pyrone production with fungi by microparticle-enhanced cultivation (MPEC). Yeast 2015; 32:145-57; PMID:24910400
- Gethins L, Guneser O, Demirkol A, Rea MC, Stanton C, Ross R P, et al. Influence of carbon and nitrogen source on production of volatile fragrance and flavour metabolites by the yeast Kluyveromyces marxianus. Yeast 2015; 32:67-76; PMID:25308412
- Etschmann MM, Sell D, Schrader J. Medium optimization for the production of the aroma compound 2-phenylethanol using a genetic algorithm. J Mol Catal B-Enzym 2004; 29:187-3; http://dx.doi.org/10.1016/j.molcatb.2003.10.014
- Fabre CE, Blanc PJ, Gerard G. Production of 2-phenylethyl alcohol by Kluyveromyces marxianus. Biotechnol Prog 1998; 14:270-4; PMID:9548779; http://dx.doi.org/10.1021/bp9701022
- Garavaglia J, Flôres SH, Pizzolato TM, Peralba MD, Ayub MA. Bioconversion of l-phenylalanine into 2-phenylethanol by Kluyveromyces marxianus in grape must cultures. World J Microb Biot 2007; 23:1273-9; http://dx.doi.org/10.1007/s11274-007-9361-3
- Yin S, Zhou H, Xiao X, Lang T, Liang J, Wang C. Improving 2-phenylethanol production via Ehrlich pathway using genetic engineered Saccharomyces cerevisiae strains. Curr Microbiol 2015; 70:762-7; PMID:25681107; http://dx.doi.org/10.1007/s00284-015-0785-y
- Kim B, Cho BR, Hahn JS. Metabolic Engineering of Saccharomyces cerevisiae for the Production of 2-Phenylethanol Via Ehrlich Pathway. Biotechnol Bioeng 2014; 111:115-24; PMID:23836015; http://dx.doi.org/10.1002/bit.24993
- Straathof AJ. Auxiliary phase guidelines for microbial biotransformations of toxic substrate into toxic product. Biotechnol Prog 2003; 19:755-762; PMID:12790635; http://dx.doi.org/10.1021/bp025750m
- Etschmann MM, Schrader J. An aqueous-organic two-phase bioprocess for efficient production of the natural aroma chemicals 2-phenylethanol and 2-phenylethylacetate with yeast. Appl Microbiol Biotechnol 2006; 71:440-443; PMID:16397768; http://dx.doi.org/10.1007/s00253-005-0281-6
- Hua D, Lin S, Li Y, Chen H, Zhang Z, Du Y, et al. Enhanced 2-phenylethanol production from L-phenylalanine viain situproduct adsorption. Biocatal Biotransfor 2010; 28:259-66; http://dx.doi.org/10.3109/10242422.2010.500724
- Eshkol N, Sendovski M, Bahalul M, Katz-Ezov T, Kashi Y, Fishman A. Production of 2-phenylethanol from L-phenylalanine by a stress tolerant Saccharomyces cerevisiae strain. J Appl Microbiol 2009; 106:534-42; PMID:19200319; http://dx.doi.org/10.1111/j.1365-2672.2008.04023.x
- Xie T, Fang HY, Zhuge B, Zhuge J. Promotional mechanism of high glycerol productivity in the aerobic batch fermentation of Candida glycerinogenes after feeding several amino acids. App Biochem Microbiol 2009; 45:303-308; http://dx.doi.org/10.1134/S0003683809030119
- Ji H, Lu X, Wang C, Zong H, Fang H, Sun J, et al. Identification of a novel HOG1 homologue from an industrial glycerol producer Candida glycerinogenes. Curr Microbiol 2014; 69:909-14; PMID:25119307; http://dx.doi.org/10.1007/s00284-014-0670-0
- Wang ZX, Zhuge J, Fang HY, Prior BA. Glycerol production by microbial fermentation: A review. Biotechnol Adv 2001; 19:201-23; PMID:14538083; http://dx.doi.org/10.1016/S0734-9750(01)00060-X
- Stark D, Zala D, Münch T, Sonnleitner B, Marison IW, Stockar UV. Inhibition aspects of the bioconversion of L-phenylalanine to 2-phenylethanol by Saccharomyces cerevisiae. Enzyme Microb Tech 2003; 32:212-23; http://dx.doi.org/10.1016/S0141-0229(02)00237-5
- Nicolaou SA, Gaida SM, Papoutsakis ET. A comparative view of metabolite and substrate stress and tolerance in microbial bioprocessing: From biofuels and chemicals, to biocatalysis and bioremediation. Metab Eng 2010; 12:307-31; PMID:20346409; http://dx.doi.org/10.1016/j.ymben.2010.03.004
- Zhao XQ, Bai FW. Mechanisms of yeast stress tolerance and its manipulation for efficient fuel ethanol production. J Biotechnol 2009; 144:23-30; PMID:19446584; http://dx.doi.org/10.1016/j.jbiotec.2009.05.001
- Ding J, Huang X, Zhang L, Zhao N, Yang D, Zhang K. Tolerance and stress response to ethanol in the yeast Saccharomyces cerevisiae. Appl Microbiol Biot 2009; 85:253-63; http://dx.doi.org/10.1007/s00253-009-2223-1
- Rep M, Krantz M, Thevelein JM, Hohmann S. The Transcriptional Response of Saccharomyces cerevisiae to Osmotic Shock. J Biol Chem 2000; 275:8290-300; PMID:10722658; http://dx.doi.org/10.1074/jbc.275.12.8290
- Yu KO, Ju J, Ramzi AB, Choe SH, Kim SW, Park C, Han SO. Increased ethanol production from glycerol by Saccharomyces cerevisiae strains with enhanced stress tolerance from the overexpression of SAGA complex components. Enzyme Microb Tech 2012; 51:237-43; http://dx.doi.org/10.1016/j.enzmictec.2012.07.003
- Zhuge J, Fang HY, Wang ZX, Chen DZ, Jin HR, Gu H L. Glycerol production by a novel osmotolerant yeast Candida glycerinogenes. Appl Microbiol Biot 2001; 55:686-92; http://dx.doi.org/10.1007/s002530100596
- Kitagawa T, Tokuhiro K, Sugiyama H, Kohda K, Isono N, Hisamatsu M, et al. Construction of a beta-glucosidase expression system using the multistress-tolerant yeast Issatchenkia orientalis. Appl Microbiol Biotechnol 2010; 87:1841-53; PMID:20467739; http://dx.doi.org/10.1007/s00253-010-2629-9
- Stark D, Münch T, Sonnleitner B, Marison IW, Stockar UV. Extractive bioconversion of 2-phenylethanol from L-phenylalanine by Saccharomyces cerevisiae. Biotechnol Prog 2001; 18:514-23; http://dx.doi.org/10.1021/bp020006n
- Etschmann MM, Sell D, Schrader J. Screening of yeasts for the production of the aroma compound 2-phenylethanol in a molasses-based medium. Biotechnol Lett 2003; 25:531-6; PMID:12882140; http://dx.doi.org/10.1023/A:1022890119847