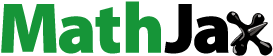
ABSTRACT
58s bioactive glass shows great potential for bone defects repair. However, at early repairing stage, the degradation rate of 58s glass is too fast due to the fast ion-exchange. At later repairing stage, the degradation rate of 58s glass is too slow due to the high dense mineral layer. In this work, Zinc oxide (ZnO) and β-tricalcium phosphate (β-TCP) were introduced into 58s glass bone scaffolds to improve the degradability. The results showed that ZnO could decrease the degradation rate and promote the stability of 58s glass at early repairing stage. Moreover, the presence of β-TCP appeared to increase the degradation rate at a later stage of repairing. Furthermore, in vitro biocompatibility study, carried out using human osteoblast-like cells (MG63), demonstrated that ZnO and β-TCP enhanced cell attachment and proliferation. The study provided a reference for further research in bone tissue engineering.
Introduction
Bioactive glass (BG, SiO2–CaO–P2O5 system) is one of the most appealing biomaterials for bone defects repair owing to its good biocompatibility and excellent bone affinity.Citation1,2 It is beneficial for the formation of strong bonding with bone tissue.Citation3 Besides, recent researches show that soluble ions released from BG can stimulate osteogenic genes expression and promote angiogenesis.Citation4,5 58s glass, as a new generation of BG, has received special attention as a promising bone scaffold material.Citation6-8 However, the degradability of 58s glass is undesirable. At early repairing stage, the degradation rate of this glass is too fast due to the fast ion-exchange. At later repairing stage, the degradation rate of this glass is too slow due to the high dense mineral layer.Citation9,10 Thus, the urgent question is to improve the biological stability at the early repair and accelerate the degradation rate at the late repair of 58s glass.
Recent studies indicated that ZnO can promote the stability of bone substitute,Citation11 which could be a good solution to slow down the degradation rate at the early immersion stage and promote the stability of the 58s scaffolds. In addition, Zinc elements play a crucial role in human body due to the stimulatory effect on new bone formation by inhibiting the osteoclast growth and promoting the osteoblasts proliferation.Citation12,13 Ikeuchi et al reported that Zn could stimulate the osteogenic differentiation of bone marrow cells.Citation14 Bandyopadhyay et al discovered that ZnO-containing calcium phosphate ceramics exhibited higher the stability and densification compared with pure calcium phosphate ceramics.Citation15
It was well known that the mineral layer on β-TCP is loose after soaking in body fluid.Citation16 β-TCP was also used as a bioabsorbable filler to improve the degradation properties of bioinert materials and have been widely recognized. Moreover, β-tricalcium phosphate (β-TCP) is considered as an ideal bioactive material, which has been studied extensively and used in clinical due to the excellent osteoconductivity.Citation17,18 Yeo et al reported that β-TCP could increase the degradation rate of polycaprolactone (PCL) scaffolds implanted in rabbit calvaria defects.Citation19 Therefore, β-TCP is also expected to accelerate the degradation rate of 58s glass at the late stage of repair.
The aim of this study was to improve the degradability of 58s glass via mixing with ZnO and β-TCP. 58s glass/ZnO/β-TCP composite scaffolds were manufactured by selective laser sintering (SLS). The biological properties were evaluated by Fourier-transform infrared (FTIR) and scanning electron microscope (SEM) equiped with energy dispersed spectroscopy (EDS). The compressive strength of the scaffolds after different immersion days in SBF was also measured with universal testing machine. Moreover, the biocompatibility was evaluated by culturing osteoblast-like cells (MG63) on the scaffolds.
Results and discussion
Phase identification
The XRD spectra of the 58s scaffolds and 58s glass/β-TCP/ZnO composite scaffolds was shown in . The spectra of 58s glass scaffolds did not show any characteristic diffraction peaks. Meanwhile, a broad band at 20° ∼ 30°, a typical characteristic of amorphous glass, was observed in XRD pattern.Citation20 This indicated that the amorphous state of 58s glass was maintained during densification. The characteristic peaks of 58s glass/β-TCP/ZnO composite scaffolds were in good agreement with JCPDS # 09–0169 (β-TCP) and no α-TCP peaks were detected in composite scaffolds.Citation21 Furthermore, the addition of ZnO promoted the phase stability of β-TCP during sintering, which was reported by other researchers as well.Citation22
In vitro bioactivity evaluation
FTIR spectra of 58s glass scaffolds surfaces after cultivation in SBF at different time-points were shown in . As can be seen, some absorption peaks at around 800 and 464 cm−1 was ascribed to the silicate networks (Si-O-Si) which were exhibited in all the scaffolds.Citation23 The spectrum of 58s glass scaffolds before soaking in SBF showed absorption peaks at around 1039 cm−1, which was correspondence to v3 PO43−.Citation24 The bands in the vicinity of 3500 and 1621 cm−1 belonged to O–H in adsorbed water.Citation25 The band appeared at around 1498 cm−1 assigned to v3 IR absorption of carbonate as a result of water and CO2 mixed in glass powders.Citation26 With increasing the immersion time of the 58s glass scaffolds, the intensity of the silicate absorption peaks decreased and carbonate absorption peaks increased. In addition, a couple of well-defined new bands formed at 601 and 566 cm−1, which were correspondence to v4 PO43−.Citation27 The results indicated that the apatite crystals appeared on the scaffolds were hydroxyl-carbonate apatite (HCA). FTIR spectra of 58s glass/β-TCP/ZnO composite scaffolds before and after immersing in SBF were shown in . With increasing the immersion time, the intensity of FTIR absorption peaks got stronger, which may be attributed to the existence of HCA on the composite scaffolds. However, in comparison with 58s glass scaffolds, the FTIR spectra of composite scaffolds showed stronger absorption peaks after 28 d immersion, which indicated a higher amount of HCA was deposited on the composite scaffolds.
Degradability evaluation
The weight losses for 58s glass scaffolds and 58s glass/β-TCP/ZnO composite scaffolds after soaking in SBF at given time-points were shown in . The result presented that the weight of 58s scaffolds decreased rapidly in the initial 3 d immersion, and reached a steady value, limiting weight above ∼14 d. The highest mass loss of 58s glass scaffolds was 8.10 ± 0.27%. Moreover, the weight of 58s glass/β-TCP/ZnO composite scaffolds decreased slowly during the initial 14 d. With the soaking time up to 28 d, composite scaffolds showed a continued degradation in weight from 4.75 ± 0.12% to 6.13 ± 0.34%. The weight losses in composite scaffolds are primarily affected by 2 mechanisms: (i) the matrix dissolution or degradation, and (ii) HCA formation in SBF.Citation28,29 Our results revealed that dissolution was dominated over the formation of HCA for both scaffolds. Based on the results of , it could be concluded that the degradation rate of composite scaffolds was optimal and caused a higher amount of HCA formed on the scaffolds. In general, the excellent formation ability of apatite precipitation on scaffolds depends on optimal degradation properties of bioactive material.Citation30 The different apatite-formation ability and degradation rate of scaffolds were further illustrated using microstructural examination.
Figure 3. Weight losses for 58s glass scaffolds and 58s glass/β-TCP/ZnO composite scaffolds after soaking in SBF at different time.
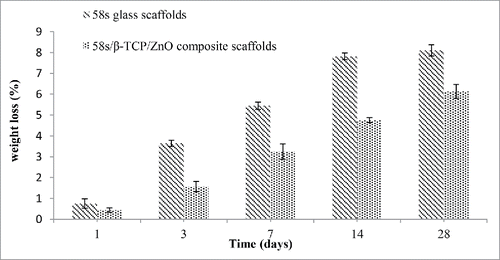
The compressive strength of 58s glass scaffolds and 58s glass/β-TCP/ZnO composite scaffolds after cultivation in SBF for selected point-in-time was shown in . The initial compressive strengths of 23.66 ± 3.9 MPa and 30.28 ± 2.79MPa were observed for 58s glass scaffolds and 58s glass/β-TCP/ZnO composite scaffolds, respectively. The mechanical improvement of composite scaffolds was dependent on the pinning effect of ZnO. A significant drop in strength from 23.66 ± 3.9 MPa to 15.26 ± 1.26 MPa was observed for 58s glass scaffolds during the first 7 d followed by a slightly decrease to 10.97 ± 1.85 MPa till 28 d of SBF immersion. However, composite scaffolds showed a slow and gradual decrease in strength from 30.28 ± 2.79 MPa to 23.45 ± 2.17 MPa over the 28 d period. Studies have shown that zinc has a positive effect on stability of matrix,Citation31 which lead to the slow strength degradation of composite scaffolds.
Microstructure of the formed apatite
To better illustrate the effects of β-TCP and ZnO on bioactivity and degradability of 58s composite scaffolds, the surface morphology and EDS of 58s glass and 58s glass/β-TCP/ZnO composite scaffolds after cultivation in SBF at different point-in-time were shown in . The surface morphology of 58s glass scaffolds soaked in SBF for 3, 14 and 28 d were presented in , and , respectively, at both high and low magnification. As can be seen in , some unusual crystallites with cauliflower shape were observed on the surfaces of 58s glass scaffolds after 3 d cultivation in SBF. According to the EDS analysis, the elements detected were mainly Ca and P. The presence of Si in EDS could be ascribed to the 58s glass. After 14 d of soaking (), significant growth of the apatite layer was detected on the 58s glass scaffolds. EDS spectrum confirmed the large amount of Ca and P in this precipitations. After the soaking time extended to 28 d (), a densely packed mineral layer appeared on the surface of 58s glass scaffolds. At a higher magnification, the mineral layer seemed to be composed of a dense structure of nanometer-sized particles. EDS spectrum indicated that the newly formed mineral layer contained mainly calcium, phosphorus and small proportions of silicon. The calcium/phosphorus mole ratio of the precipitations (1.37) was close to that of HA (1.67),Citation32 indicating that apatite formed on the 58s glass scaffolds. Also, the Na element coming from the SBF was detected in EDS spectrum.
Figure 5. Microstructures and EDS of (A, C, and E) 58s glass scaffolds and (B, D, and F) 58s glass/β-TCP/ZnO composite scaffolds after cultivation in SBF for (A, B) 3 d, (C, D) 14 d and (E, F) 28 d.
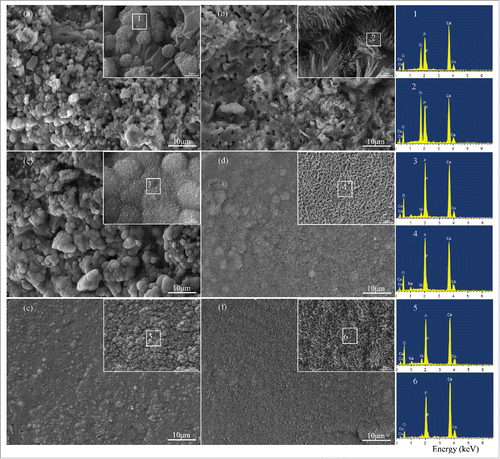
58s glass/β-TCP/ZnO composite scaffolds were cultivated in SBF for various time. , and presented the surface morphology of composite scaffolds after cultivation in SBF for 3, 14, and 28 d, respectively. The results showed that some small precipitations with needle-like shape were observed on the composite scaffolds after 3 d soaking in SBF (). The increased in Ca and P with the decreasing Si contents of the newly formed layer were also presented in EDS spectrum. After 14 d immersion (), a mineral layer with nanosheet-like shape and had heaped pores structures covered the whole surface of the composite scaffolds. With the cultivation time further increasing to 28 d (), the morphology of the mineral layer changed from nanosheet-like to nearly rod-like and a higher amount of apatite (Ca/P atomic ratio of 1.64) seemed to form at this stage. Considering those results, it could be suggested that the apatite formation ability of 58s glass/β-TCP/ZnO composite scaffolds were higher than those of 58s glass scaffolds. Besides, the improved degradation of composite scaffolds was also attributed to highly porosity microstructure of the mineral layer.
Biocompatibility
The morphological properties of the MG63 cells culture on the scaffolds for 1, 3 and 5 d were shown in , respectively. After the first day culture, a few MG-63 cells with a globular appearance were observed on both scaffolds, which indicated that all the scaffolds were non-poisonous to the cells. After 3 d, MG-63 cells exhibited well attachment on both scaffolds with numerous lamellipodia. In addition, cells showed better penetration on the 58s glass/β-TCP/ZnO composite scaffolds than the 58s glass scaffolds. Extracellular matrix (ECM) was actively released from the seeded MG-63 cells, which could be observed on the surfaces of cells and scaffolds. As the culture time extended to 5 d, a variety of adherent cells were observed. On 58s glass scaffolds, cells presented fusiform in shape with some lamellipodia extension. In contrast, more dense growth of cells and more connections among cells were observed on the composite scaffolds. Furthermore, wide distribution of the ECM on composite scaffolds was also observed, which indicated the good cellular migration.
Figure 6. The morphology of MG-63 cell seeded on (A-C) 58s glass scaffolds and (E-F) 58s glass/β-TCP/ZnO composite scaffolds after different days of culture.
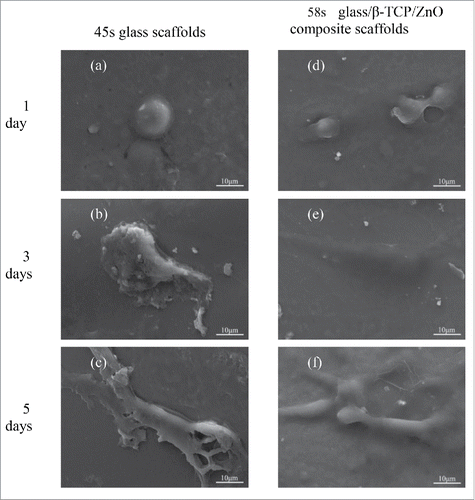
showed fluorescence images of MG-63 cell cultured on the surface of scaffolds after different days. It could be seen that viable cells were blue, which attached fine and exhibited ordinary growth on both scaffolds at the early time point (1 d). The quantity of cells increased with the culture time from 1 to 5 d. Meanwhile, an increase in cell proliferation was found on the composite scaffolds due to the higher cell density, compared with the 58s glass scaffolds. The magnified images further illustrated that cells grow and propagate well on composite scaffolds which accorded with the development as shown in . These results suggested that the presence of β-TCP and ZnO enhanced cellular attachment and proliferation. Generally speaking, the continuous degradation of composite scaffolds could provide more meshes to promote cells adhesion compared to the 58s glass scaffolds. Meanwhile, ZnO could accelerate cells proliferation and differentiation, which were vital to the applications in tissue engineering and consistent with recent reports by other researchers.Citation33
Materials and methods
Materials and fabrication
The micrographs of 58s glass, β-TCP and ZnO powders were expressed in . 58s glass and β-TCP were provided by the KunShan Chinese Technology New Materials Co. Ltd. 58s glass with the nominal chemical composition (in mol) 60% SiO2-36% CaO- 4% P2O5 was prepared by the sol-gel method. It has an irregular shape and size of particles is 5–15μm (). β-TCP is a crystal of calcium phosphate, with a Ca/P radio of 1.50. Its particles are rhombus-like with average particle size of 500 nm (). High-purity ZnO powder (99.8% purity) was provided by Nanjing Emperor Nano Material Co. Ltd (China). The average particle size of ZnO is 50 nm ().
The composite powders were prepared by adding 20 wt % β-TCP and 2 wt % ZnO into 58s glass powders. The amounts of compositions were chosen based on our previous studies.Citation34,35 The powders were mixed using the following procedure: appropriate amounts of β-TCP and ZnO powders were first dispersed in ethanol and sonicated using ultrasonic cleaning device for 1 h to minimize the agglomerates. Then, 58s glass was added to the β-TCP/ZnO suspension and ball-milled for 12h using ZrO2 balls as the grinding media to increase the homogeneity of the powders. Subsequently, the mixed powders were kept in a dryer at 60°C to remove the liquid phases.
A homemade SLS system, as previously reported,Citation36 was used to prepare the scaffolds. It is made up of a CO2 laser with a focus system, a 3-dimensional motion worktable and a corresponding control system. Briefly, the process starts with paving a thin layer of powders in the working platform. Then, the laser beam selectively scanned over the powders based on CAD pattern. After each layer is sintered, the working platform is moved down by the thickness of monolayer. The process is repeated, layer by layer, until the sintering job is done. The optimal process parameters was selected at laser power of 6.0 W, beam diameter of 1.2 mm, scanning speed of 100 mm·min−1.
Microstructure and phase analysis
The microtopography of the scaffolds were viewed under a scanning electron microscope (SEM) (TESCAN MIRA3 LMU, CO., Czech) operated at 20 kV acceleration. Before the SEM observations, the scaffolds were sputtered with a thin layer of Pt using a sputter coater in vacuum (JFC-1600, JEOL CO., Japan). In addition, Energy dispersive spectroscopy analyzer (EDS) attached to SEM was used to define the elemental constitution of the deposits on the scaffolds after cultivation in SBF.
Phase analysis of 58s scaffolds and 58s glass/β-TCP/ZnO composite scaffolds was carried out by a X-ray Rigaku D/MAX-2500 diffractometer (XRD, Rigaku Co., Japan). Cu-Kα radiation, λ = 1.540600 Å. The operating voltage and current were 40 kV and 250 mA, respectively. For qualitative analysis, the dates were recorded from 10° to 60° with 0.02° 2θ step size.
The functional group analyses were performed via Fourier-transform infrared (FTIR) using Nicolet™ 6700 spectrometer (Thermo Scientific Co. American). For IR analysis, samples scraped from the scaffolds were carefully mixed with KBr) (IR grade) and pressed to a disc. The sample to KBr (in weight) was 0.004. Then, the disc was analyzed in the mid-infrared range (4000–400 cm−1).
Immersion in SBF
The in vitro bioactivity and degradation behavior of 58s scaffolds and 58s glass/β-TCP/ZnO composite scaffolds were evaluated by immersing the scaffolds in SBF solution which has similar ion concentrations to those in human fluid environment. The SBF solution was prepared by blending highly purified reagents (NaCl, NaHCO3, KCl, K2HPO4•3H2O, MgCl2•6H2O, CaCl2 and Na2SO4) with distilled water and buffered at a pH of 7.40 with trimethyl aminomethane-HCl.
The scaffolds were exposed to the SBF solution and kept at 36.5 ± 0.5°C in a shaking incubator after 1, 3, 7, 14 and 28 d, respectively. Over the course of the study, the SBF solutions were refreshed every alternate day. After immersing in the SBF for scheduled time, scaffolds were removed of the solution and washed gently with deionized water, followed by drying at atmospheric temperature and weighing. The weight loss was calculated using the equation (Equation1(1)
(1) ):
(1)
(1)
Where W0 is the weight of the scaffolds before soaking in SBF and Wt is the weight at time t.
The microstructure of the converted scaffolds was examined by FTIR and SEM equipped with EDS microanalysis using the scheduled conditions.
Mechanical response
The compressive strength of the scaffolds, as-prepared or after scheduled immersion time in SBF, was measured by using a universal testing machine (Shanghai Zhuoji instruments Co. LTD, China) at a loading speed of 0.5 mm per min. The results was calculated based on the maximum load recorded and scaffolds dimension. At least 4 tests for each group were measured and compressive strength was finally presented by mean ± standard deviation (SD)
Cell culture studies
The biocompatibility of 58s scaffolds and 58s glass/β-TCP/ZnO composite scaffolds were studied using MG63 cells (human osteogenic sarcoma) (ATCC, Rochville, MD). Cells were preserved and propagated in Dulbecco's modified Eagle's medium (DMEM) supplementing with 100 μg/ml streptomycin, 100 U/ml penicillin, 0.25 mg/ml amphotericin and 10% fetal bovine serum (FBS, ATCC) at 36.5 ± 0.5°C in a moist environment containing 5% of CO2. The medium was renewed every 2 d. Before confluence, cells were trypsinized, centrifuged and resuspended in complete nutrient solution. At the same time, the scaffolds were disinfected by 75% ethanol and washed with sterile phosphate-buffered saline (PBS) to remove all residues.
The cells were transplanted on the pre-wetted scaffolds at a concentration of 5 × 103 cells ml−1 in 12-well culture plates. Subsequently, the cell-scaffolds were saved at 36.5 ± 0.5°C in a moist incubator under standard cultivating environment for 1, 3 and 5 d, respectively. Scaffolds were fetched out from the incubator and washed 2 times with deionised water to remove fine and adhered impurities after the scheduled incubation time. Cells were fixed on the scaffolds with 2.5% glutaraldehyde for 90 mins, followed by 2 washes with warm PBS to eliminate unattached cells. Following this, fixed scaffolds were dried with progressive concentrations of graded ethanol/water solutions (30, 40, 50, 60, 70, 90, 95 and 100%) and further dried using hexamethyldisilazane (HMDS). Dried scaffolds were then mounted on aluminum stubs, gold coated and observed under electron microphotography to characterize cell attachment.
Fluorescence techniques
To obtain the cell proliferation, 58s glass scaffolds and 58s glass/β-TCP/ZnO composite scaffolds were analyzed using fluorescence microscopy after 1, 3 and 5 d cell culture. After the scheduled incubation period, scaffolds washed with PBS solution and fixed with buffered ice-cold paraformaldehyde (4%), and then dyed with DAPI (blue) (4′, 6-diamidino-2-phenylindole) for 5 min for cell nuclei staining. Imaging was completed using fluorescence microscope (BX51, Olympus Optical Co., Japan) with a CCD camera (DP72, Olympus Optical Co., Japan).
Conclusions
In this paper, the influences of β-TCP and ZnO on the bioactivity and biodegradability of 58s glass scaffolds were studied. The results showed that the 58s glass/β-TCP/ZnO composite scaffolds exhibited high bioactivity and improved degradability compared to the 58s glass scaffolds. The initial compressive strength of the 58s glass scaffolds and 58s glass/β-TCP/ZnO composite scaffolds were 30.28 ± 2.79 MPa and 23.66 ± 3.9 MPa, respectively. The different bioactivity and biodegradability were further illustrated by microstructural examination. Furthermore, cell culture studies clearly exhibited improved cell attachment and proliferation on the 58s glass/β-TCP/ZnO composite scaffolds as compared with 58s glass scaffolds. The results indicated that β-TCP and ZnO are viable reinforcements to improve the bioactivity and degradability of 58s glass scaffolds.
Disclosure of potential conflicts of interest
No potential conflicts of interest were disclosed.
Funding
This work was supported by the following funds: (1) The Natural Science Foundation of China (51575537, 81572577, 81472058); (2) High Technology Research and Development Program of China (2015AA033503); (3) Overseas, Hong Kong & Macao Scholars Collaborated Researching Fund of National Natural Science Foundation of China (81428018); (4) Hunan Provincial Natural Science Foundation of China (14JJ1006); (5) The Open-End Fund for the Valuable and Precision Instruments of Central South University; (6) The faculty research grant of Central South University (2013JSJJ011, 2013JSJJ046); (7) The Fundamental Research Funds for the Central Universities of Central South University; (8) Innovation Foundation of Sunward Intelligent (2014scxjj03).
References
- Jones JR. Review of bioactive glass: from Hench to hybrids. Acta Biomater 2013; 9:4457-86; PMID:22922331; http://dx.doi.org/10.1016/j.actbio.2012.08.023
- Scarber RE, Salaam AD, Thomas V, Janowski GM, Dean D. Direct sol-gel electrospinning of fibrous bioglass scaffolds for bone tissue engineering. J Biomater Tissue Eng 2013; 3:440-7; http://dx.doi.org/10.1166/jbt.2013.1101
- Aguiar H, Serra J, González P. Nanostructural transitions in bioactive sol-gel silicate glasses. Int J Appl Ceram Technol 2011; 8:511-22; http://dx.doi.org/10.1111/j.1744-7402.2011.02633.x
- Hoppe A, Güldal NS, Boccaccini AR. A review of the biological response to ionic dissolution products from bioactive glasses and glass-ceramics. Biomaterials 2011;32:2757-74; PMID:21292319; http://dx.doi.org/10.1016/j.biomaterials.2011.01.004
- Cao H, Liu X. Plasma-Sprayed Ceramic Coatings for Osseointegration. Int J Appl Ceram Technol 2013;10:1-10; http://dx.doi.org/10.1111/j.1744-7402.2012.02770.x
- Bosetti M, Cannas M. The effect of bioactive glasses on bone marrow stromal cells differentiation. Biomaterials 2005;26:3873-9; PMID:15626435; http://dx.doi.org/10.1016/j.biomaterials.2004.09.059
- Bielby RC, Pryce RS, Hench LL, Polak JM. Enhanced derivation of osteogenic cells from murine embryonic stem cells after treatment with ionic dissolution products of 58S bioactive sol-gel glass. Tissue Eng 2005;11:479-88; PMID:15869426; http://dx.doi.org/10.1089/ten.2005.11.479
- Zhu H, Hu C, Zhang F, Feng X, Li J, Liu T, Chen J, Zhang J. Preparation and antibacterial property of silver-containing mesoporous 58S bioactive glass. Mater Sci Eng 2014;42:22-30; PMID:25063087; http://dx.doi.org/10.1016/j.msec.2014.05.004
- Fu Q, Rahaman MN, Fu H, Liu X. Silicate, borosilicate, and borate bioactive glass scaffolds with controllable degradation rate for bone tissue engineering applications. I. Preparation and in vitro degradation. J Biomed Mater Res A 2010;95:164-71; PMID:20544804; http://dx.doi.org/10.1002/jbm.a.32824
- Chen QZ, Thompson ID, Boccaccini AR. 45S5 Bioglass-derived glass-ceramic scaffolds for bone tissue engineering. Biomaterial 2006;27:2414-25; PMID:16336997; http://dx.doi.org/10.1016/j.biomaterials.2005.11.025
- Rezaee A, Rangkooy H, Khavanin A, Jafari AJ. High photocatalytic decomposition of the air pollutant formaldehyde using nano-ZnO on bone char. Environ Chem Lett 2014;12:353-7; http://dx.doi.org/10.1007/s10311-014-0453-7
- Chou J, Hao J, Kuroda S, Bishop D, Ben-Nissan B, Milthorpe B, Otsuka M. Bone regeneration of rat tibial defect by zinc-tricalcium phosphate (Zn-TCP) synthesized from porous Foraminifera carbonate macrospheres. Mar Drugs 2013;11:5148-58; PMID:24351911; http://dx.doi.org/10.3390/md11125148
- Carbajal L, Serena S, Caballero A, Saínz MA, Detsch R, Boccaccini AR. Role of ZnO additions on the β/α phase relation in TCP based materials: Phase stability, properties, dissolution and biological response. J Eur Ceram Soc 2014;34:1375-85; http://dx.doi.org/10.1016/j.jeurceramsoc.2013.11.010
- Ikeuchi M, Ito A, Dohi Y, Ohgushi H, Shimaoka H, Yonemasu K, Tateishi T. Osteogenic differentiation of cultured rat and human bone marrow cells on the surface of zinc-releasing calcium phosphate ceramics. J Biomed Mater Res A 2003;67:1115-22; PMID:14624496; http://dx.doi.org/10.1002/jbm.a.10041
- Bandyopadhyay A, Withey EA, Moore J, Bose S. Influence of ZnO doping in calcium phosphate ceramics. Mater Sci Eng 2007;27:14-17; http://dx.doi.org/10.1016/j.msec.2005.11.004
- Eder C, Meissner J, Bretschneider W, Schildböck S, Ogon M. Analysis of a β-TCP bone graft extender explanted during revision surgery after 28 months in vivo. Eur Spine J 2014;23:157-60; PMID:23636843; http://dx.doi.org/10.1007/s00586-013-2802-9
- Diogo GS, Gaspar VM, Serra IR, Fradique R, Correia IJ. Manufacture of β-TCP/alginate scaffolds through a Fab@ home model for application in bone tissue engineering. Biofabrication 2014;6:025001; PMID:24657988; http://dx.doi.org/10.1088/1758-5082/6/2/025001
- Jin HB, Oktar FN, Dorozhkin S, Agathopoulos S. Sintering behavior and properties of reinforced hydroxyapatite/TCP biphasic bioceramics with ZnO-whiskers. J Compos Mater 2011;45:1435-45; http://dx.doi.org/10.1177/0021998310383728
- Yeo A, Wong WJ, Teoh SH. Surface modification of PCL‐TCP scaffolds in rabbit calvaria defects: Evaluation of scaffold degradation profile, biomechanical properties and bone healing patterns. J Biomed Mater Res A 2010;93:1358-67; PMID:19911382
- Fu Q, Rahaman MN, Bal BS, Brown RF, Day DE. Mechanical and in vitro performance of 13–93 bioactive glass scaffolds prepared by a polymer foam replication technique. Acta Biomater 2008;4:1854-64; PMID:18519173; http://dx.doi.org/10.1016/j.actbio.2008.04.019
- Ribeiro G, Trommer RM, dos Santos LA, Bergmann CP. Novel method to produce β-TCP scaffolds. Mater Lett 2011;65:275-7; http://dx.doi.org/10.1016/j.matlet.2010.09.066
- Fielding GA, Bandyopadhyay A, Bose S. Effects of silica and zinc oxide doping on mechanical and biological properties of 3D printed tricalcium phosphate tissue engineering scaffolds. Dental Mater 2012;28:113-22; PMID:22047943; http://dx.doi.org/10.1016/j.dental.2011.09.010
- Ma J, Chen CZ, Wang DG, Meng XG, Shi JZ. In vitro degradability and bioactivity of mesoporous CaO-MgO-P2O5-SiO2 glasses synthesized by sol–gel method. J Sol-gel Sci Technol 2010;54:69-76; http://dx.doi.org/10.1007/s10971-010-2159-z
- Mohandes F, Salavati-Niasari M. In vitro comparative study of pure hydroxyapatite nanorods and novel polyethylene glycol/graphene oxide/hydroxyapatite nanocomposite. J Nanoparticle Res 2014;16:1-12; http://dx.doi.org/10.1007/s11051-014-2604-y
- Madejová J. FTIR techniques in clay mineral studies. Vib Spectrosc 2003;31:1-10; http://dx.doi.org/10.1016/S0924-2031(02)00065-6
- Groust JF, Costentin G, Krafft JM, Massiani P. Mechanism and deactivation process of the conversion of methylbutynol on basic faujasite monitored by operando DRIFTS. Phys Chem Chem Phys 2010;12:937-46; PMID:20066379; http://dx.doi.org/10.1039/B919733H
- Lemos AF, Rocha JHG, Quaresma SSF, Kannan S, Oktar FN, Agathopoulos S, Ferreira JMF. Hydroxyapatite nano-powders produced hydrothermally from nacreous material. J Eur Ceram Soc 2006;26:3639-46; http://dx.doi.org/10.1016/j.jeurceramsoc.2005.12.011
- Plewinski M, Schickle K, Lindner M, Kirsten A, Weber M, Fischer H. The effect of crystallization of bioactive bioglass 45S5 on apatite formation and degradation. Dental Mater 2013;29:1256-64; PMID:24157243; http://dx.doi.org/10.1016/j.dental.2013.09.016
- Xiao W, Fu H, Rahaman MN, Liu Y, Bal BS. Hollow hydroxyapatite microspheres: A novel bioactive and osteoconductive carrier for controlled release of bone morphogenetic protein-2 in bone regeneration. Acta Biomater 2013;9:8374-83; PMID:23747325; http://dx.doi.org/10.1016/j.actbio.2013.05.029
- Pan H, Zhao X, Darvell BW, Lu WW. Apatite-formation ability–Predictor of “bioactivity”? Acta Biomater 2010;6:4181-8; PMID:20493974; http://dx.doi.org/10.1016/j.actbio.2010.05.013
- Wu C, Ramaswamy Y, Chang J, Woods J, Chen Y, Zreiqat H. The effect of Zn contents on phase composition, chemical stability and cellular bioactivity in Zn-Ca-Si system ceramics. J Biomed Mater Res B 2008;87:346-53; PMID:18464251; http://dx.doi.org/10.1002/jbm.b.31109
- Jallot E, Nedelec JM, Grimault AS, Chassot E, Grandjean-Laquerriere A, Laquerriere P, Laurent-Maquin D. STEM and EDXS characterisation of physico-chemical reactions at the periphery of sol-gel derived Zn-substituted hydroxyapatites during interactions with biological fluids. Colloids Surf B Biointerfaces 2005;42:205-10; PMID:15893220; http://dx.doi.org/10.1016/j.colsurfb.2005.03.001
- Haimi S, Gorianc G, Moimas L, Lindroos B, Huhtala H, Räty S, Kuokkanen H, Sándora GK, Schmidb C, Miettinena S, et al. Characterization of zinc-releasing three-dimensional bioactive glass scaffolds and their effect on human adipose stem cell proliferation and osteogenic differentiation. Acta Biomater 2009;5:3122-31; PMID:19428318; http://dx.doi.org/10.1016/j.actbio.2009.04.006
- Shuai C, Li P, Liu J, Peng S. Optimization of TCP/HAP ratio for better properties of calcium phosphate scaffold via selective laser sintering. Mater Charact 2013;77:23-31; ; http://dx.doi.org/10.1016/j.matchar.2012.12.009
- Feng P, Wei P, Shuai C, Peng S. Characterization of mechanical and biological properties of 3-D scaffolds reinforced with zinc oxide for bone tissue engineering. PloS One 2014;9:e87755; PMID:24498185; http://dx.doi.org/10.1371/journal.pone.0087755
- Shuai C, Gao C, Nie Y, Hu H, Zhou Y, Peng S. Structure and properties of nano-hydroxypatite scaffolds for bone tissue engineering with a selective laser sintering system. Nanotechnology 2011;22:285703; PMID:21642759; http://dx.doi.org/10.1088/0957-4484/22/28/285703