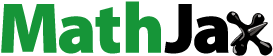
ABSTRACT
Thermal denaturation of lysozymes was studied as a function of protein concentration, phosphate buffer concentration, and scan rate using differential scanning calorimetry (DSC), which was then analyzed by the isoconversional method. The results showed that lysozyme thermal denaturation was only slightly affected by the protein concentration and scan rate. When the protein concentration and scan rate increased, the denaturation temperature (Tm) also increased accordingly. On the contrary, the Tm decreased with the increase of phosphate buffer concentration. The denaturation process of lysozymes was accelatated and the thermal stability was reduced with the increase of phosphate concentration. One part of degeneration process was not reversible where the aggregation occurred. The other part was reversible. The apparent activation energy (Ea) was computed by the isoconversional method. It decreased with the increase of the conversion ratio (α). The observed denaturation process could not be described by a simple reaction mechanism. It was not a process involving 2 standard reversible states, but a multi-step process. The new opportunities for investigating the kinetics process of protein denaturation can be supplied by this novel isoconversional method.
Introduction
In recent years, the denaturation of protein has become a research hot spot in life science. From the viewpoint of practical applications such as laser surgery, thermal therapy, and tissue engineering, its kinetics is of important interest.Citation1 Protein denaturation happens with a change of conditions including pH levels and high temperature or pressure. Lysozymes are a highly basic protein representing up to 4%, by weight, of chicken egg whites. Its stable structure makes it an ideal model to study the denaturation of protein.Citation2
Although a lot of research has been published on the experimental and theoretical aspects of denaturation, most focus primarily on the thermodynamic aspects; there are few articles on the kinetics of a lysozyme denaturation process using the isoconversional method.Citation3-12 Several reports used the lysozyme denaturation process for fitting first-order kinetic models.Citation3-5 However, some researchers believe there is an intermediate state and aggregation state instead of 2 state processes.Citation6,7 The lysozyme denaturation kinetics model is debatable. How to solve this dispute and find a model suitable for lysozyme denaturation kinetics is a quandary. The application of model-free approaches represented by a variety of isoconversional methods is one advisable option.
The integral “model-free” method is helpful, even if the mechanism of complex reaction is unclear. No model is essential to be pre-selected in the evaluations of kinetic parameters. The isoconversional method can provide new methods for exploring the kinetic process of protein denaturation. Many applications have been used by it in kinetic analysis of thermally stimulated processes in synthetic polymers. But the use of this method for the protein thermal denaturation has rarely been reported.Citation1 It can well describe the mechanism of a single process, also can get the relationship between conversion rate and activation energy for complex process. The apparent activation energy calculated by this method is not dependent on the mechanism of function, and can provide useful information for in-depth analysis of the mechanism.Citation13
The study of thermal kinetics analysis of protein denaturation has great significance in order to obtain a thorough understanding of protein folding and stretching, the denaturation mechanism, structural stability, and the metabolism of living organisms.
In this contribution, the isoconversional method was used to analyze thermal denaturation kinetics of different concentrations of lysozymes in different concentrations of phosphate buffer. The results confirmed that the isoconversional method opened a new avenue for quick and easy research in the multi-step or one-step kinetics processes of protein denaturation.
Materials and methods
Theoretical method
With reference to the literature,Citation14 the apparent activation energy is computed by the isoconversional method. The following formula is used.(1)
(1)
A simple reaction mechanism cannot be available to reaction process, if the computed apparent Ea decreases with the increase of the conversion ratio (α). Otherwise, kinetic triplets can be calculated.Citation14
Experimental
Instruments and materials
The instruments and materials used in this research included a Mettler Toledo calorimeter, model DSC 822e (Switzerland), Orion 828 pH meter (America, Thermo Orion), and Lysozyme (made in China, used without further purification).
All other chemicals were of analytical grade. pH 6.89 buffer [(0.020 M Na2HPO4, 0.020 M KH2PO4 and 0.150 M NaCl, pH6.89) and (0.010 M Na2HPO4, 0.010 M KH2PO4 and 0.150 M NaCl, pH6.89)] was used. The lysozyme was dissolved in phosphate buffer at different concentrations.
DSC
DSC of the protein solutions was performed under various experimental conditions of scan rate, protein concentration, and buffer concentration. A 40 μL medium pressure crucible was used with 30 μL of sample in sample cell and the corresponding buffer in a reference cell for each particular condition in the temperature range of 35–95° C. The instrument was calibrated with indium. Using the Star® evaluation program Calorimetric, measurements were carried out. DSC scans after subtraction of appropriate base lines and computer smoothing are shown in . In different conditions, the transition peak temperature (Tm) was determined graphically. By integration of the transition peak, the transition enthalpy △H was obtained. Measurements were implemented on 3 samples respectively (replicates), and the averages were reported.
Results and discussion
Reversibility and irreversibility of lysozyme thermal denaturation process
The unique ability of lysozymes to refold upon cooling after having been unfolded by heating make it possible to apply repeated heating and cooling cycles. The purpose of cycling is to check the reversibility and irreversibility of the lysozyme thermal denaturation process. An irreversible process locks the unfolded protein in a state from which it cannot refold over the time of the experiment. Any irreversibly denatured protein does not contribute to heat absorbed under the DSC peak in the second scan. shows that the endotherms decreased in the area after each cycle. And an obvious trend of lower △H values was observed as cycle scans increased. The 60 mg/mL protein samples, which drawn from the sample cell after the first rise scanning, presented a strong gelation state and meant an irreversible denaturated aggregation had occurred.
Figure 1. DSC circle curves of 60 mg/mL lysozyme in 0.010 M phosphate buffer with 0.150 M NaCl (pH 6.89). The heat-up rate was 2.5 K/min. The first heating scan, the following cooling scan, and the other heating scans are labeled as scan1, scan2, and scan3, respectively.
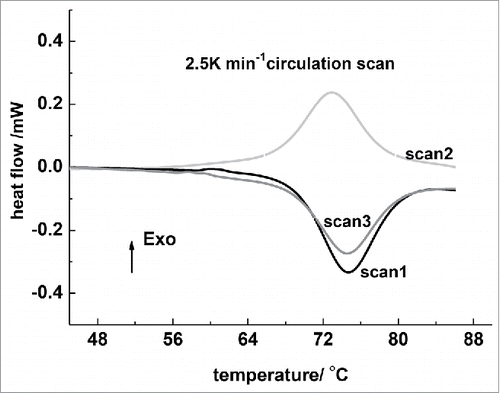
It is believed that processes leading to irreversibility involve aggregation, improper disulfide bond formation, or covalent alterations. In the course of refolding proteins from an unfolded state, aggregation leads to a decrease in the recovery of folded proteins. It is well-known that heating globular proteins in aqueous solutions induces aggregation which, if the concentration is sufficiently large, can lead to the formation of gel.Citation15 When a protein unfolds, parts of the interior are exposed at the molecular surface. This changes the affinity of the molecule for other molecules. Under conditions favoring inter-chain interactions, aggregation of protein molecules occurs and a gel is formed.Citation16 This aggregation progress is irreversible.Citation17
Impacts of protein and phosphate buffer concentrations on the lysozyme thermal denaturation process
The impact of protein and phosphate buffer concentrations on the lysozyme thermal denaturation process was investigated, and the DSC curves of lysozymes under different conditions at a heating rate of 2.0 K/min were studied, as displayed in . The corresponding unit enthalpy of denaturation (△H) and the Tm are listed in . Only one endothermic peak was found in each thermogram. Circle scans revealed that irreversible denaturation took place for part of the lysozyme. From and , it can be seen that as the protein concentration increased, an upward shift in Tm and a decrease in unit enthalpy occurred in the buffers of the same concentration. The shift in Tm indicated that the lysozyme concentration affected the denaturation process and aggregation affected by lysozyme concentration obviously occurred. The variations in unit enthalpy of the denaturation process as a function of lysozyme concentration likely indicated that aggregation occurred because denaturation was associated with intensive heat absorption while aggregation was an exothermic process that made the enthalpy decrease.Citation18 This result was consistent with Daisuk's reportCitation19 in which it was reported that aggregation occurs in the lysozyme denaturation process.
Figure 2. DSC curves of different concentrations lysozyme in phosphate buffer with different concentrations. The heat-up rate was 2.0 K/min. The light gray DSC curves denote lysozyme in 0.010 M buffer. From the top to the bottom, the lysozyme concentration was 10, 20, 30, 40, 60, and 80 mg/mL. Dark gray DSC curves denote lysozyme in 0.020 mol/L phosphate buffer. From the top to the bottom, the lysozyme concentration was 30, 60, and 150 mg/mL.
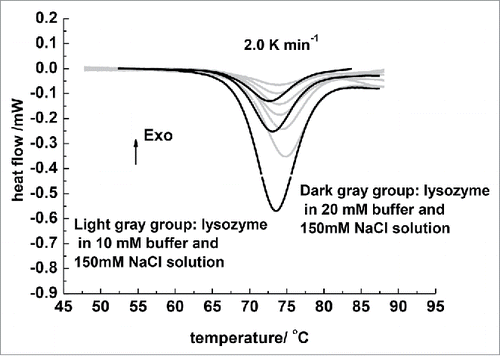
Table 1. Unit enthalpy of denaturation (△H) and Tm of different lysozyme concentrations in phosphate buffer of different concentrations at heat-up rate of 2.0 K/min.
An obvious influence of the buffer concentration on the lysozyme thermal denaturation was showed in . As the light gray DSC curves were compared to the dark gray curves, it was observed that the Tm of the lysozymes in 0.020 mol/L phosphate buffer distinctly moved to lower temperatures at the same scan rate and protein concentration. As shown in , the Tm of the same concentration lysozyme in higher-concentrated buffer markedly decreased, which suggested that the thermal stability of the lysozyme was in correlation with the buffer concentration. The lysozyme denaturation process was accelerated and its thermostability decreased with the increase of phosphate concentration. It is theoretically assumed that the bigger net charge molecule's stability presents a stronger dependence on salts.Citation20 The lysozyme that is a bigger net charge molecule has 6-7 net charges in pH 4-7. The changes of phosphate concentration caused an ion intensity change, which consequently affected the hydrophobic group exposure and the protein tertiary structure.Citation21 The phosphate reduced the thermal stability of lysozyme showed that the salts held the lysozyme denatured status over the nature ones.
Impact of scan rate on the lysozyme thermal denaturation process
Lysozyme thermal denaturation curves at different scan rates and different buffer concentrations are shown in . The corresponding Tm is provided in . Based on the Van 't Hoff equation, the DSC curve of a standard reversible 2-state protein denaturation process should be symmetric, and the Tm and △H should not change with the scan rate.Citation22 As displayed in , when the scan rate increased from 1.0 to 2.5 K/min, an increase of about 0.5° C for the Tm was observed. shows that the DSC curves were asymmetric, and the △H increased with the increase of scan rate. Based on these results, it was educed that the denaturation process of the lysozyme was not a standard reversible 2-state process.
Figure 3. (A) DSC curves of 30 mg/mL lysozyme in 0.010 M phosphate buffer at different heat-up rates. (B) DSC curves of 60 mg/mL lysozyme in 0.020 M phosphate buffer at different heat-up rates. (C) DSC curves of 150 mg/mL lysozyme in 0.020 M phosphate buffer. From top to bottom, the scan rates were 0.25, 0.5, 1.0, 1.5, 2.0, and 2.5 K/min.
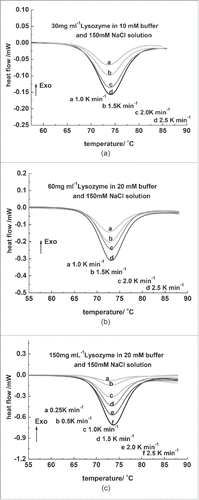
Table 2. The Tm of different concentrations of lysozyme in phosphate buffer of different concentrations at diverse heat-up rates.
Thermal denaturation of lysozyme analyzed by isoconversional method
From the DSC conversion plots, the α–T data were obtained. And from the isoconversional plot of ln(β/T1.894661) versus 1/T at diverse conversion ratios (α) in scope of 0.2–0.8, according to Eq. (Equation1(1)
(1) ), the lysozyme denaturation apparent Ea was calculated. All these plots had linear correlation coefficients larger than 0.99. The Ea decreased with the increase of conversion ratio was indicated in . The reaction process could not be described by a simple reaction mechanism, as the Ea depended on the extent of the conversion ratio. And the process kinetics could not be reduced to a single-step process. Therefore, it was determined that the denaturation process of the lysozyme was not a standard reversible 2-state process.
Figure 4. The apparent Ea - conversions (α) of 30, 60, and 150 mg/mL lysozyme in phosphate buffer of different concentrations.
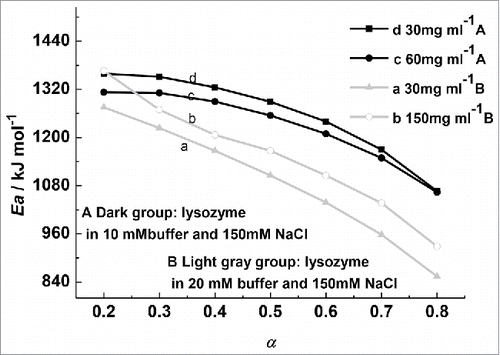
Using the isoconversional method, Citation1 studied collagen thermal denaturation. A powerful decrease of Ea with conversion was observe, which was in accord with the Lumry–Eyring model.Citation23 This kind of situation was believed ideally: Ea at α→1 should provide an estimate for the irreversible step activation energy, while Ea at α→0 should provide an estimate for Ea +△H, where Ea is the irreversible step activation energy and △H is the reversible step standard enthalpy. Therefore, the apparent activation energy calculated by the isoconversional method does not stay invariable at different conversion ratios (α), but decreases with the increase of α. However, it must be pointed out that in the lysozyme thermal transition, aggregation is most likely a main cause of irreversibility. As such, the irreversible step kinetics can be predicted to higher than the first order reaction.Citation24 The denaturation process of lysozyme cannot be depicted by the Lumry-Eyring model. As described by Nohara, et al.,Citation19 the apparent first-order reaction involves a second-order reaction that characterizes the aggregation of the denatured protein molecules. Our conclusion was in accordance with their results.
Conclusion
An extensive study of the thermal denaturation of lysozymes was conducted by DSC under various experimental conditions including scan rate, protein concentration, and buffer concentration. It was learned that the thermal denaturation of lysozymes was slightly affected by protein concentration and scan rate, and the phosphate reduced its thermal stability. The apparent activation energy of lysozyme thermal denaturation, calculated by the isoconversional method, decreased throughout the process; this proved the denaturation process of lysozymes was not standard reversible 2-state process. A simple reaction mechanism could not be applied to reaction progress, because the Ea depended on the extent of the conversion ratio. These results confirmed that the isoconversional method can be used as a new avenue for investigating multi-step or one-step kinetics processes of protein denaturation.
Disclosure of potential conflicts of interest
No potential conflicts of interest were disclosed.
Funding
The support for this project from the National Natural Science Foundation of China (30600116) and Nature Sciences Foundation of Hubei and China Postdoctoral Science Foundation is gratefully acknowledged.
References
- Vyazovkin S, Vincent L, Sbirrazzuoli N. Thermal denaturation of collagen analyzed by isoconversional method. Macromol Biosci 2007; 7(11):1181-6; PMID:17876859; http://dx.doi.org/10.1002/mabi.200700162
- Lindgren M, Sparrman T, Westlund PO. A combined molecular dynamic simulation and urea (14)N NMR relaxation study of the urea-lysozyme system. Spectrochim. Acta: Part A 2010; 75(3):953-9; http://dx.doi.org/10.1016/j.saa.2009.11.054
- Burovaa TV, Grinberga NV, Grinberga VY, Rariy RV, Klibanov AM. Calorimetric evidence for a native-like conformation of hen egg-white lysozyme dissolved in glycerol. Biochim Biophys Acta 2000; 1478(2):309-17; PMID:10825542; http://dx.doi.org/10.1016/S0167-4838(00)00028-5
- Petersen SB, Jonson V, Fojan P, Pedersen S. Sorbitol prevents the self-aggregation of unfolded lysozyme leading to and up to 13 degrees C stabilisation of the folded form. J Biotechnol 2004; 114(3):269-78; PMID:15522436; http://dx.doi.org/10.1016/j.jbiotec.2004.07.004
- Mohamed AA, Duarte PR, Kim S. Effect of starch on the thermal kinetics and transmittance properties of lysozyme. J Sci Food Agric 2005; 85:450-8; http://dx.doi.org/10.1002/jsfa.2001
- Hédoux A, Affouard F, Descamps M, Guinet Y, Paccou L. Microscopic description of protein thermostabilization mechanisms with disaccharides from Raman spectroscopy investigations. J Phys Condens Matter 2007; 19:205142-8; PMID:Can't; http://dx.doi.org/10.1088/0953-8984/19/20/205142
- Byrne N, Wang LM, Belieres JP, Angell CA. Reversible folding-unfolding, aggregation protection, and multi-year stabilization, in high concentration protein solutions, using ionic liquids. Chem Commun 2007; 45:2714-6; http://dx.doi.org/10.1039/B618943A
- Li XQ. Microcalorimetry study of lysozyme denaturation induced by the guanidine chloride and urea. Chemistry 2011; 74 (9):834-8.
- Alice B, Jennifer JM. Reversible and non-reversible thermal denaturation of lysozyme with varying pH at low ionic strength. Biochimica et Biophysica Acta 2013; 1834:2064-70; http://dx.doi.org/10.1016/j.bbapap.2013.06.001
- Li SJ, Wei YM, Fang YQ, Zhang W, Zhang B. DSC study on the thermal properties of soybean protein isolates/corn starch mixture. J Therm Anal Calorim 2014; 115:1633-8; http://dx.doi.org/10.1007/s10973-013-3433-4
- Anna M, Zofia D, Ewa SK, Barbara K. Calorimetric monitoring of the effect of endurance training and testosterone treatment on rat serum denaturation transition. J Therm Anal Calorim 2014; 115:2231-7; http://dx.doi.org/10.1007/s10973-013-3611-4
- Mallamace F, Corsaro C, Mallamace D, Cicero N Vasi, S, Dugo G, Stanley HE. Dynamical changes in hydration water accompanying lysozyme thermaldenaturation. Front Phys 2015; 10(5):106104-7; http://dx.doi.org/10.1007/s11467-015-0486-9
- Vyazovkin S, Wight CA. Model-free and model-fitting approaches to kinetic analysis of isothermal and nonisothermal data. Thermochim Acta 1999; 340/341:53-68; http://dx.doi.org/10.1016/S0040-6031(99)00253-1
- Tang, WJ, Wang CX, Chen DH. An investigation of the pyrolytic kinetics of some aliphatic amino acids. J Anal Appl Pyrolysis 2006; 75:49-53; http://dx.doi.org/10.1016/j.jaap.2005.04.003
- Maeda Y, Yamada H, Ueda T, Imoto T. Effect of additives on the renaturation of reduced lysozyme in the presence of 4 M urea. Protein Eng 1996; 9:461-5; PMID:8795046; http://dx.doi.org/10.1093/protein/9.5.461
- Baier S, McClements J. Impact of Preferential Interactions on Thermal Stability and Gelation of Bovine Serum Albumin in Aqueous Sucrose Solutions. J Agric Food Chem 2001; 49:2600-8; PMID:11368642; http://dx.doi.org/10.1021/jf001096j
- Bon CL, Nicolai T, Durand D. Kinetics of aggregation and gelation of globular proteins after heat-induced denaturation. Macromolecules 1999; 32:6120-7; http://dx.doi.org/10.1021/ma9905775
- Biliaderis CG. Differential scanning calorimetry in food research—A review. Food Chem 1983; 10:239-65; http://dx.doi.org/10.1016/0308-8146(83)90081-X
- Nohara D, Mizutani A, Sakai T. Kinetic study on thermal denaturation of hen egg-white lysozyme involving precipitation. J Biosci Bioeng 1999; 87:199-205; PMID:16232451; http://dx.doi.org/10.1016/S1389-1723(99)89013-6
- Shosheva A, Donchev A, Dimitrov M, Kostov G, Toromanov G, Getov V, Alexov E. Comparative study of the stability of poplar plastocyanin isoforms. Biochim Biophys Acta 2005; 1748:116-27; PMID:15752700; http://dx.doi.org/10.1016/j.bbapap.2004.12.012
- Ravindra BK, Vinod B. Ionic-strength-dependent transition of hen egg-white lysozyme at low pH to a compact state and its aggregation on thermal denaturation. Eur J Biochem 1997; 245:781-9.
- Sanchez-Ruiz JM. Theoretical analysis of Lumry-Eyring models in differential scanning calorimetr. Biophys J 1992; 61(4):921-935; PMID:19431826; http://dx.doi.org/10.1016/S0006-3495(92)81899-4
- Lumry R, Eyring H. Conformational changes of protein. J Phys Chem 1954; 58:110-20; http://dx.doi.org/10.1021/j150512a005
- Jaenicke R. Folding and association of proteins. Prog Biophys Mol Biol 1987; 49:117-237; PMID:3327098; http://dx.doi.org/10.1016/0079-6107(87)90011-3