ABSTRACT
Cardiovascular disorders are on the rise worldwide due to alcohol abuse, obesity, hypertension, raised blood lipids, diabetes and age-related risks. The use of classical antiplatelet and anticoagulant therapies combined with surgical intervention helped to clear blood clots during the inceptive years. However, the discovery of streptokinase and urokinase ushered the way of using these enzymes as thrombolytic agents to degrade the fibrin network with an issue of systemic hemorrhage. The development of second generation plasminogen activators like anistreplase and tissue plasminogen activator partially controlled this problem. The third generation molecules, majorly t-PA variants, showed desirable properties of improved stability, safety and efficacy with enhanced fibrin specificity. Plasmin variants are produced as direct fibrinolytic agents as a futuristic approach with targeted delivery of these drugs using liposome technlogy. The novel molecules from microbial, plant and animal origin present the future of direct thrombolytics due to their safety and ease of administration.
Introduction
Thrombolytic therapy plays a pivotal role in cardiovascular disease management. A thrombus or a blood clot is a solid mass, made up of the blood constituents, that forms within the vascular system. The pieces of such thrombi can break free and may be carried to a different location via the blood stream. Such an “embolus” that blocks the vessels gives rise to the condition known as an embolism. These blockages obstruct the oxygen supply to the surrounding tissue resulting in its degradation and death. Depending upon the location of such thrombi and emboli, they may manifest themselves in the form of varied thromboembolic disorders like stroke, pulmonary embolism, deep vein thrombosis, arterial thrombosis, acute myocardial infarction (AMI) and retinal artery occlusion.Citation1 With 17.5 million deaths in 2012 and an estimated rate of approximately 23.6 million by 2030, such cardiovascular diseases are progressively becoming the leading cause of morbidity and mortality worldwide.Citation2 The majority of these cases occur in the low and middle-income countries due to socio-economic conditions and inaccessibility of efficient healthcare services. Three major variables predisposing to thrombosis include: alterations in the vascular wall, reduction in blood stream velocity and an increased tendency of blood coagulation (Virchow's triad).Citation3 The emerging trends of alcohol abuse, obesity, physical inactivity, unhealthy diet and lifestyle, tobacco use, hypertension, raised blood lipids, diabetes, air pollution, high salt intake combined with age-related risks further increase the risk of developing such cardiovascular complications.Citation2
An effective therapy should be based on rapid clot dissolution so as to minimize tissue damage and boost survival rates. In general, there are 4 treatment options available for thrombolytic therapy. The classical therapy relies upon the administration of anticoagulants and antiplatelet drugs. The anticoagulants, like warfarin (Coumadin®, Bristol-Myers Squibb Pharma Company) and heparin, act by blocking steps in the fibrin formation cascade. Warfarin interferes with the synthesis of vitamin K-dependent coagulation factors and heparin blocks factor X activation and thrombin formation in the coagulation cascade.Citation4 The antiplatelet drugs prevent thrombosis from occurring or getting bigger, and blocking the vessel. Aspirin inhibits platelet aggregation while clopidogrel and ticlopidine inhibit platelet activation. Surgical intervention to remove the thrombus is performed under conditions of massive vessel occlusions where drug treatments have failed or are contra-indicated. Fibrinolytic enzymes, on the other hand, are used to lyse pre-existing thrombi.Citation4,5
Enzymes are used as anti-inflammatory agents, digestive aids, mucolytics, thrombolytics, anticoagulants, oncolytics, and antimicrobials for the clinical management of several disorders.Citation4 Thrombolytic enzymes are mainly serine or metalloproteases having a direct or indirect mode of action for fibrin lysis. The former includes plasmin-like enzymes such as mini-plasmin, micro-plasmin, delta-plasmin, nattokinase (NK), serrapeptase (SP), some snake-venom thrombin-like enzymes (SVTLEs), and some earthworm fibrinolytic enzymes (EFEs), capable of degrading fibrin directly while the latter group is composed of the plasminogen activators that include streptokinase (SK), staphylokinase (SAK), urokinase (u-PA) and tissue-plasminogen activator (t-PA) etc ().
Table 1. An overview of different thrombolytic agents.
Plasminogen activators mediate thrombolysis via activation of the inactive zymogen present in the circulation, i.e. plasminogen into the active serine protease plasmin that can degrade the fibrin blood clot. The plasminogen activators mediate fibrin lysis via a direct or an indirect mechanism. The direct plasminogen activators are serine proteases that mediate a direct action on plasminogen to catalyze its activation eg: u-PA, pro-urokinase (pro-uPA), t-PA, reteplase (r-PA), tenecteplase (TNK-tPA) etc. The indirect plasminogen activators (SK, SAK), on the other hand, do not have any enzymatic activity of their own. They form a 1:1 stoichiometric complex with plasmin or plasminogen. This activator complex then activates the plasminogen molecules present in the circulation ().
While the plasminogen activators of human origin (t-PA and u-PA) are still in use for thrombolytic therapy, their high cost and side effects have led researchers to look for alternate sources of fibrinolytic enzymes. In this scenario, microbial fibrinolytic enzymes have gained attention due to their low cost of production and reduced side effects.Citation6 Microbes from food and non-food sources have been found to be promising producers of such thrombolytic enzymes with Bacillus being the most important genus.Citation4 Fermented Asian foods have been found to provide a good habitat for the production of such bacterial enzymes.Citation6 Furthermore, apart from humans and microbes, thrombolytic enzymes have also been reported in animals like vampire bats, snakes and earthworms and more recently in plant lattices.Citation7,8,9,10 In addition, recombinant DNA technology has helped to produce thrombolytic variants with different pharmacokinetic and pharmacodynamic properties. In the current review, the authors have described the current status of thrombolytic therapy with an insight into the mechanism of coagulation and thrombolysis. The physical and biochemical characteristics of the different thrombolytic enzymes have been discussed in detail with an emphasis on the future state of thrombolytic therapies.
Mechanism of coagulation and thrombolysis
The physiological balance between the coagulation and thrombolytic processes in the human body maintains a healthy circulatory system. A schematic representation of the coagulation and thrombolytic cascade has been shown in . During normal circulation, the coagulation cascade is in the favor of anticoagulation.Citation11 While coagulation is essential for minimizing blood loss during an injury (hemostasis), it is also involved in the formation of a fibrin clot that may block the blood vessels. Thus, any perturbations in the hemostatic balance may lead to bleeding or thrombotic disorders.
Figure 1. Overview of the coagulation (extrinsic and intrinsic) and thrombolytic cascades. In the extrinsic pathway (red arrow), membrane associated tissue factor (TF) binds to activated factor VII (VIIa) already present in circulation. This binary complex further activates factor X to factor Xa. Factor Xa then activates factor V to Va forming the prothrombinase complex that catalyzes activation of prothrombin to thrombin. Thrombin cleaves fibrinogen to fibrin that forms a mesh in which the RBCs, WBCs and platelets are entrapped to form the blood clot. In the intrinsic pathway (blue arrow), factor XII is activated via contact with negatively charged surfaces (collagen). Factor XIIa activates factor XI to XIa which catalyzes the activation of factor IX. Activated factor IXa forms a complex with factor VIIIa and mediates activation of factor X. The extrinsic and intrinsic pathways of blood coagulation merge at factor X activation (black arrow). The propagation of the fibrin clot is limited by the action of the serine protease, plasmin (green arrow). It is formed via the activation of plasminogen by plasminogen activators such as u-PA, t-PA, SK, APSAC etc. Plasmin cleaves fibrin into fibrin degradation products that are then removed by macrophages. A detailed mechanism has been described in the text.
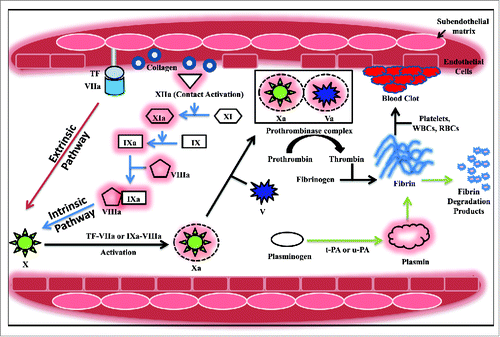
All enzymes and substrates of the coagulation cascade are vitamin-K-dependent proteins that interact with the phospholipid membrane via γ-carboxy glutamic acid residues located in their amino-terminal domains. These residues are involved in binding to calcium that is essential for the proper folding of the γ-carboxy glutamic acid domain.Citation11 Blood coagulation may be initiated through 2 pathways: the extrinsic pathway or the intrinsic pathway. The extrinsic pathway, where tissue factor plays a critical role, is the mechanism of trauma-initiated coagulation in vivo. It is triggered in response to the exposure of blood from a ruptured endothelial layer to the extravascular tissue.Citation11 This causes the release of tissue factor (TF, thromboplastin), a membrane protein that is constitutively expressed by cells surrounding the vascular bed.Citation12 It is a receptor for both zymogen and activated forms of factor VII i.e., factor VIIa. A fraction of factor VII circulates in the blood in the active form. Binding of active factor VIIa to the tissue factor promotes coagulation by catalyzing the activation of factors IX and X to IXa and Xa in the presence of Ca2+ ions.Citation11,12 Subsequently, the prothrombinase complex is formed that is responsible for activating prothrombin to thrombin. This complex, assembled on the negatively charged phospholipid membrane in the presence of Ca2+ ions, is composed of factor Xa and its co-factor Va. Factor V activation is catalyzed by factor Xa on the phospholipid surface as well as by thrombin in plasma and on the phospholipid membrane. In addition, thrombin activates factor VIII and XI resulting in feedback amplification of the system. In circulation, the inactivated factor VIII is attached to von Willebrand factor, an adhesive protein necessary for the primary platelet plug formation. Once activated, the factor VIIIa dissociates and forms a complex with factor IXa on the platelet surface called the tenase complex.Citation11 This complex then activates factor X which can then activate thrombin using factor Va as the co-factor. The extrinsic pathway is so named due to the requirement of an exogenous agent, the TF, which is exposed upon breakage of the endothelial barrier.Citation12 In an alternate mechanism, coagulation may also be carried out via the intrinsic pathway. This pathway is named so since all the components required for this pathway are present in the blood. In this pathway, factor XII (Hageman factor) gets activated and forms factor XIIa by contact activation via negatively charged surfaces like glass, collagen, kaolin or even membranes of some microorganisms.Citation12,13 Factor XIIa can further activate plasma prekallikrein to plasma kallikrein which in turn catalyzes the activation of factor XII in a positive feedback loop.Citation12 Active factor XIIa, along with high molecular weight kininogen as a co-factor, subsequently activates factor XI to factor XIa. In the presence of Ca2+ ions, factor XIa converts factor IX to factor IXa.Citation13 The activation of factor X to factor Xa is catalyzed by factor IXa along with factor VIIIa. Further, factor Xa can convert prothrombin to thrombin in the presence of calcium and factor Va.
Regulation of the coagulation cascade occurs via the action of several anticoagulants. The enzyme antithrombin inactivates several coagulation factors. The tissue factor pathway inhibitor (TFPI) blocks the TF:VIIa complex formation by complexing with factor Xa. Another regulator, activated protein C is responsible for proteolytic inactivation of factor Va and VIIIa.Citation11,12
Once thrombin has been produced, it converts the soluble fibrinogen into insoluble fibrin, which forms the blood clot. During thrombus formation, the circulatory erythrocytes, leukocytes, and platelets are incorporated into its structure. The detailed mechanism of fibrinogen activation by thrombin has been described in the next section.
The thrombus so formed is lysed through the action of proteolytic enzymes. Thrombolysis is the term given to the enzyme mediated degradation of the fibrin clot. In the mammalian circulatory system, plasmin plays a central role during thrombolysis. This protease is generated from the circulatory zymogen plasminogen through the action of plasminogen activators, u-PA, and t-PA in vivo. Plasmin restores the vascular patency by proteolysis of insoluble fibrin into fibrin degradation products leading to the dissolution of blood clots.Citation14
The thrombolytic system
The human thrombolytic system is an enzyme-based proteolytic system whose main function is to limit the intravascular thrombosis and restore blood flow through the occluded vessels. It is composed of the plasma zymogen plasminogen and its activated form plasmin, fibrinogen and its active product fibrin, as well as plasminogen activators that activate plasminogen to plasmin. Furthermore, it also includes the inhibitors of the thrombolytic system that mediate the regulation of thrombolysis. These act at the level of plasmin and plasminogen activators. In the following section, we describe the structure of plasminogen and fibrinogen and their conversion into the activated forms plasmin and fibrin respectively as well as various inhibitors responsible for thrombolysis regulation.
(i) Human plasminogen (Pg, profibrinolysin)
The gene for human plasminogen (52 kb) is located on chromosome 6 and consists of 19 exons and 18 introns. Human plasminogen (92 kDa) is synthesized as a single polypeptide chain of 810 amino acids while the mature protein is 791 amino acids (2373 bp) having a leader sequence of 19 residues (57 bp) that is cleaved during secretion. The primary source of plasminogen is the liver, however, other sources like the adrenal glands, thymus, lung, brain, gut, uterus, kidney, spleen, testis and heart have also been identified. Its concentration in the plasma is 2.2 µM. The protein is glycosylated at Asn289, Thr346, Ser248, Thr339 and phosphorylated at Ser578.Citation15 Plasminogen activators catalyze the activation of plasminogen to plasmin (Pm) by inducing a proteolytic cleavage at the Arg561-Val562 bond. Plasmin is a 2-chain molecule held together by 2 disulfide linkages. The N-terminal heavy chain of human plasmin (Glu1-Arg561) carries 5 kringle (K) domains that are triple-disulfide linked protein modules involved in interaction with lysine. Domains K1 and K4 exhibit the strongest affinity with lysine, while K2 has the weakest affinity.Citation15 Binding of plasmin to fibrin is mediated via the lysine-binding sites on these kringle domains. Kringle 1 is also involved in plasmin binding to the α2-antiplasmin inhibitor, one of the fastest protein-protein interactions (rate of the inhibition reaction is ∼107 M−1 s−1).Citation16 Consequently, plasmin bound to fibrin impedes inactivation by this inhibitor. The C-terminal light chain carries the serine protease domain with His603, Asp646 and Ser741 as the catalytic triad.Citation16 The heavy chain also accommodates a 77 residues N-terminal peptide region, which is cleaved during in vitro activation of plasminogen by autolysis. The Glu1-Pm and Lys78-Pm can catalyze the hydrolysis of the N-terminus 77 amino acids of Glu1-Pg or Glu1-Pm, converting Glu1-Pg to Lys78-Pg and Glu1-Pm to Lys78-Pm. Such a plasmin or plasminogen molecule having an N-terminal glutamic acid residue has a closed conformation while that with lysine as the N-terminal residue has a more open conformation making it the preferred substrate for activation by plasminogen activators.Citation15 Plasmin degrades fibrin as well as fibrinogen, and other coagulation factors including factor V, VIII, IX, XI, XII, insulin and growth hormones.Citation17 Various cell types such as monocytes, lymphocytes, granulocytes and endothelial cells express plasminogen receptors, which interact with plasminogen to accelerate its conversion to plasmin. The surface bound plasmin has an enhanced activity and is also protected from inactivation via inhibitors compared to plasmin in the blood plasma. Cell surface associated plasmin can also mediate the degradation of extracellular matrix and basement membrane molecules.Citation18
(ii) Fibrinogen
It is the most abundant plasma protein (2–4 g/L) synthesized mainly by the hepatocytes and in smaller amounts by the epithelium of the intestine, cervix, and lungs with a half-life of approximately 4 d.Citation19 The FGA, FGB and FGG genes, coding for 3 fibrinogen chains namely Aα, Bβ and γ, are located on chromosome 4 in a ∼50 kb region.Citation20 The first step in fibrinogen assembly is the formation of 2 chain complexes Aα-γ and Bβ-γ. In the next step, a third chain is added to form a 3 chain complex Aα-Bβ-γ. Finally, 2 of the 3 chain complexes join at the N-termini to form the dimeric hexamer (Aα-Bβ-γ)2 of 340 kDa held together by 29 disulfide bonds.Citation19 The central portion of this glycoprotein (domain E) comprises of the N-termini of the 6 chains. The domain D is a globular structure composed of the carboxy terminal of the Bβ and γ chains. The coiled coils of the α-helices of the 3 chains connect these domains. The spontaneous polymerization of the fibrinogen molecule is prevented due to the presence of negative charges at the N-terminus of the Aα and Bβ chains. Thrombin catalyzes the hydrolysis across the Arg16-GlyCitation17 and Arg14-GlyCitation15 peptide bonds in the N-terminal of the Aα and Bβ chains, respectively, due to which fibrinopeptides A and B are cleaved from fibrinogen. This partially degraded form of fibrin is called the fibrin monomer. Cleavage of fibrinopeptides A and B unmasks positively charged end groups which are the sites for polymerization termed as EA (Gly-Pro-Arg-Val) and EB (Gly-His-Arg-Pro). They interact non-covalently with negatively-charged complementary polymerization sites Da and Db in the C-terminal portion of the γ and β chains.Citation21 The fibrin monomers interact with each other in a half-staggered manner to form protofibrils, a 2 stranded fibrin polymer, leading to the polymerization process. Alongside, thrombin catalyzes the conversion of factor XIII to active factor XIIIa, a transglutaminase that stabilizes the fibrin structure by cross-linking. The cross-linked fibrin is mechanically stronger and more resistant to proteolysis by plasmin.
During degradation, plasmin mediates cleavage in the C-terminal portion of the Aα chain and attacks the amino and carboxy terminal part of the Bβ chain. The γ chain hydrolysis is relatively slow.Citation22 The first degradation product termed fragment X (∼260–300 kDa) is formed after the cleavage and removal of the C-terminal region of the Aα chain at Lys208-Met209, Lys221-Ser222 or Lys232-Ala233. This fragment is clottable by thrombin with an increased affinity toward plasmin and has a faster lysis rate compared to clots formed by intact fibrin.Citation22 Fragment X is further hydrolyzed giving rise to the fragments Y (∼150 kDa) and D (∼94 kDa). Fragment Y is further degraded producing another fragment D and fragment E (∼50 kDa). Degradation of cross-linked fibrin produces D-dimers instead of D fragments.Citation23
(iii) Fibrinolytic system inhibitors
Regulation of the fibrinolytic system occurs via the action of inhibitors both at the level of plasminogen activation and plasmin action. They are specific, fast-acting inactivators of the serine protease inhibitor (SERPIN) superfamily.Citation14 The main physiological inhibitors of the plasminogen activators are plasminogen activator inhibitor-1 (PAI-1) and plasminogen activator inhibitor-2 (PAI-2).Citation18 The primary plasmin inhibitor in circulation is α2-antiplasmin (α2-PI). This single chain glycoprotein (452 amino acids, 70 kDa) forms a 1:1 stoichiometric complex with plasmin by binding to the kringle domains of plasmin. α2-macroglobulin (a non-serpin inhibitor) (726 kDa tetramer composed of 4 identical subunits), is the most abundant plasmin inhibitor. It is a broad-spectrum, slow but effective inhibitor of plasmin that acts only when the concentration of α2-PI is decreased.Citation17 Upon activation by thrombin, the thrombin-activated fibrinolysis inhibitor (TAFI) (a non-serpin inhibitor) downregulates thrombolysis by removing the C-terminal lysine residues essential for plasminogen binding to fibrin.Citation14
Plasminogen activators
First generation plasminogen activators
Early treatment for myocardial infarction was palliative involving the use of morphine (analgesic), aspirin (anti-platelet), atropine, papaverine and nitroglycerin (antispasmodics to relieve coronary spasm) combined with anti-coagulants to prevent reinfarction. The use of intravenous heparin was also found to be no more efficient than the oral anticoagulants.Citation24 Hence, some of the initial studies on thrombolytic therapies were aimed at reducing mortality and evaluating the route of administration and dosing regimens. Persistent efforts paved the way for an entirely new approach to thrombolytic therapy, i.e., the use of thrombolytic enzymes. The first generation plasminogen activators, namely streptokinase and urokinase (or urokinase-type plasminogen activator), activate free circulatory plasminogen to plasmin but also degrade fibrinogen and other clotting factors in addition to fibrin. Since the addition of such plasminogen activators results in the degradation of the α2-PI inhibitor, free plasmin is no longer inhibited by α2-PI. Therefore, it causes a systemic fibrinolytic state leading to bleeding complications.Citation23
The choice of thrombolytic therapy depends on several factors, including the cost of the drug, side effects, stability, half-life as well as specificity toward fibrin and immunogenicity ().Citation17 Thus, till today in the developing countries of the world the first generation plasminogen activator, streptokinase, remains the mainstay of thrombolytic therapy due to its cost-effectiveness (US $200 per treatment).Citation1
Table 2. Characteristics of FDA approved plasminogen activators.
(i) Streptokinase
Streptokinase (SK) is a microbial plasminogen activator secreted by several strains of β-hemolytic streptococci. It is a single chain polypeptide that exhibits an indirect fibrinolytic action by activating the circulatory zymogen plasminogen. Discovered serendipitously by Dr. William Smith Tillett in 1933, this wonder drug laid the foundation for an entirely new approach in the field of cardiovascular medicine.Citation24 Indeed, thrombolytic therapy has transformed the treatment of cardiovascular disorders by overcoming the need for surgical intervention. Its amino acid sequence was determined by Jackson and Tang using the Edman degradation method. It is a 47 kDa monomeric protein (414 amino acids) with a maximum activity at a pH of ∼7.5 and an isoelectric point of 4.7. The protein is devoid of cystine, cysteine, phosphorus, conjugated carbohydrates and lipids.Citation1
The group C strain S. equisimilis H46A (ATCC 12449) isolated from a human source in 1945 was selected from several fibrinolytic isolates for streptokinase production since it yielded the most active form of this plasminogen activator. Moreover, it is less fastidious than most of the group A streptococci and can be grown on semisynthetic media.Citation1
On the basis of structural studies, streptokinase has been classified as an α+β protein. The protein consists of 3 structurally autonomous domains, i.e α, β and γ, with varied functional properties.Citation25 The 3 domains are interconnected by 2 flexible linkers bearing sequence homology whose primary structural requirements were discussed by Yadav and Sahni.Citation26 Teuten and co-workers (1993) suggested that each of these 3 domains have independent stability and the protein can have a number of partially folded states.Citation27 Welfle and coworkers (1992) demonstrated the 2-domain structure of streptokinase by differential scanning calorimetry with the less thermostable domain located in the N-terminal region of the molecule while the C-terminal region houses the more thermostable domain.Citation28 Streptokinase can activate plasminogen via a fibrin-dependent as well as a fibrin-independent mechanism.Citation1 The protein binds preferentially to the extended conformation of plasminogen via the lysine binding sites to trigger a conformational change in plasminogen leading to its activation.Citation29 Several studies involving the use of truncated fragments have been carried out to determine the regions responsible for plasminogen binding and activation. A ∼150–200 residues central core region has been found to be associated with high-affinity plasminogen binding.Citation30 The N-terminal region (1–59 residues) was found to complement the low plasminogen activation ability of the remaining molecule.Citation25,30 Furthermore, this region has multiple functional roles without which the protein has an unstable secondary structure and the activity of the remaining fragment is considerably reduced.Citation1 The protein contains 2 independent plasminogen binding sites (residues 1–59 and residues 143–293).Citation30 The Asp41-HisCitation48 region plays an important role in substrate binding. The C-terminal domain of streptokinase Met369-Pro373 is essential for recognition and activation of substrate. The coiled-coil region in the γ domain seems to be necessary for plasminogen activation.Citation17 The SK β domain is required in substrate docking and activator complex formation. Further, the complex utilizes long range protein-protein interactions with the substrate to maximize catalytic turnover.Citation1 The kringle 4 domain of plasminogen plays a critical role in catalysis of plasminogen by the activator complex.Citation31 Recently, a model of streptokinase action has been described where higher catalytic rates occur via co-operation of several exosites distributed across all 3 domains of streptokinase.Citation32
Attempts have been carried out to modify streptokinase in order to increase its half-life, reduce its immunogenicity and improve the biological efficacy. However, a major drawback of these approaches is the overlapping of the domains responsible for the activity, stability, and immunogenicity of streptokinase.Citation1 Mutants with improved stability were produced by Shi and co-workers (1998) via K59E mutations.Citation33 Similarly, Wu and coworkers (1998) mutated LysCitation59 and Lys386 residues (sites susceptible to proteolytic action by plasmin) to generate plasmin-resistant muteins in the protease deficient B. subtilis WB600 with upto 21 fold higher half-life without compromising its kinetic parameters for plasminogen activation.Citation34 Furthermore, glycosylation of P. pastoris produced recombinant streptokinase appears to make the molecule resistant to proteolysis.Citation35 PEGylation of streptokinase has been attempted as a strategy to extend its half-life and reduce immunogenicity.Citation1,36 However, such modifications compromised its plasminogen activation ability.Citation1 Recently, PEGylated mutants of streptokinase have been created with clot specificity.Citation36 Streptokinase derivatives with platelet glycoprotein binding domains have been developed that produced a higher localized concentration of plasmin in vivo.Citation1 Recombinant SK with minimized immunoreactivity has been produced. The deletion of 42 residues of streptokinase from C-terminus has been shown to reduce its immunogenicity.Citation37
The pathogenic nature of the natural isolate led co-workers to subclone the gene in several heterologous expression platforms. Extensive work has been carried out on recombinant streptokinase expression in the E. coli host with upto 25,000 IU/mL of the recombinant protein.Citation38 The secretion of glycosylated streptokinase has been reported in P. pastoris (3200 IU/mL) with an improved proteolytic resistance and stability.Citation35 Recently, 4.25 g/L of bioactive streptokinase was produced in P. pastoris with a volumetric productivity of 3727 IU/mL/h.Citation39
In the GISSI trial (Gruppo Italiano per la Sperimentazione della Streptochinasi nell'Infarto Miocardico) a reduction in mortality rate was seen when AMI patients were administered 1.5 million IU of streptokinase over 60 min.Citation40 The drug is available under the trade names Streptase® and Kabikinase® and is obtained from β-hemolytic streptococci. In India, Shankinase (Shantha Biotech), a similar biologic produced in E. coli has been approved. The drug is approved by the FDA for use in acute evolving myocardial infarction, pulmonary embolism, deep vein thrombosis and arterial thrombosis. Streptase is also used in occlusion of arteriovenous cannulae.Citation5
(ii) Urokinase-type plasminogen activator
The urokinase-type plasminogen activator (u-PA) or urokinase is primarily involved in cell migration, tissue remodeling, complement activation, pro-hormone conversion and wound healing. It is a serine protease that converts plasminogen into plasmin and thus finds clinical applications as a thrombolytic agent, but also plays a significant role as a prognostic marker in carcinogenesis and metastasis. This plasminogen activator was discovered and isolated from human urine where it was produced by the kidney cells. The vascular endothelial cells, smooth muscle cells, epithelial cells, fibroblasts, monocytes/macrophages and several malignant tumors can also produce urokinase.Citation41,42 Mammalian urine has a very low concentration of urokinase (10–15 ng/mL) making enzyme purification a tedious and expensive proposition. Cultured renal cells secrete large quantities of u-PA in vitro (50–100 ng/mL). The human plasma also has a urokinase concentration of 10 µg/L.Citation41,42
The 6.4 kb gene coding for u-PA is located on chromosome 10 and is composed of 11 exons and 10 introns.Citation41 The protein is synthesized in vivo as a 53 kDa single chain pro-enzyme (411 amino acids) (scu-PA or pro-uPA). Pro-uPA is a true zymogen with very low intrinsic activity as compared to u-PA.Citation18 However, unlike other zymogens, fibrin potentiates pro-uPA induced plasminogen activation to the level of u-PA. Pro-uPA is more fibrin specific than u-PA, which lowers the risk of bleeding complications upon its application.Citation41 Upon its secretion from the cell surface, pro-uPA binds to the uPAR (urokinase-type plasminogen activator receptor) and is converted to a 2-chain active state, i.e. tcu-PA or u-PA) via the cleavage at Lys158-Ile159 bond by neighboring membrane-bound plasmin or other proteases such as kallikrein. The receptor-bound pro-uPA is activated by plasmin faster than when it is free in the plasma.Citation18 Active uPA, then converts the neighboring membrane-bound plasminogen to plasmin. The two chains of the active u-PA (A and B) are linked by a disulfide bond. There are 3 functional domains in the molecule: a serine protease domain (residues 159–411) containing the catalytic triad His204, Asp255 and Ser356, a kringle domain (residues 45–134) that interacts with PAI-1 and an epidermal growth factor-like domain (residues 9–45) that is responsible for specific interaction with the uPAR/CD87 receptor.Citation42,43 The A chain harbors the non-catalytic kringle domain and epidermal growth factor-like domain while the B chain carries the catalytic serine-protease domain. Only the disulfide bridges in the catalytic domain are necessary for the amidolytic and fibrinolytic activities of this molecule. uPA is N-glycosylated at Asn302 in the catalytic domain and O-glycosylated at ThrCitation18 located in the epidermal growth factor like-domain.Citation42
A low molecular weight form of 2-chain urokinase (33 kDa) is created upon plasmin-mediated hydrolysis of the mature scu-PA across the Lys158-Ile159 bond and subsequently at the Lys135-Lys136 bond.Citation18,43 In urokinase isolated from urine, a higher fraction of the high molecular weight form is present while the urokinase from cultured kidney cells contains a major portion of the low molecular weight form. Further, a low molecular weight (32 kDa) form of scu-PA could be generated by the hydrolysis of the Glu143-Leu144 bond which showed similar properties as uPA.Citation43
Attempts have been carried out for the heterologous production of recombinant urokinase and pro-uPA in different expression platforms. Nelles and coworkers (1987) generated pro-uPA mutants with the Lys158 residue substituted with Gly158 (rscu-PA-Gly158) or Glu158 (rscu-PA-Glu158) and expressed them in CHO cell line.Citation44 The mutants had lower specific activity than the wild-type rscu-PA and were not converted to the 2 chain form by plasmin. However, they could still activate plasminogen following Michaelis-Menten kinetics but with a lower stability of the Michaelis complex. The same group created t-PA/u-PA hybrids (using either scu-PA or tcu-PA) which improved the fibrin-mediated plasminogen activation.Citation45 Expression of recombinant pro-uPA and its deletion mutants in S. cerevisiae using the GAL7 promoter and the prepeptide sequence of Mucor pusillus rennin led to their intracellular accumulation within the endoplasmic reticulum.Citation46 They were inactive in their native state and had to be solubilized and refolded for biological activity. Glycosylated and non-glycosylated variants of pro-urokinase were produced in the yeast Pichia pastoris.Citation47 The non-glycosylated form was less stable due to proteolysis but had a catalytic activity comparable to mammalian-derived recombinant pro-uPA. However, glycosylation of pro-urokinase by P. pastoris interfered with its fibrinolytic activity. Therefore, mammalian cells are the preferred system for recombinant urokinase production. Subcultured human umbilical vein endothelial cells produced single-chain uPA with low fibrinolytic activity, no fibrin specificity, but high plasminogen affinity.Citation48 Avgerinos and co-workers (1990) produced 51 g of rscu-PA in 1000 L medium in 31 d of CHO culture using a 20 L stirred tank fermentor.Citation49 Currently, urokinase (Abbokinase®, Kinlytic™) is commercially produced using the human neonatal kidney cells.Citation5 It contains the low molecular weight form of u-PA as the active ingredient. The drug was first approved by the FDA in 1978. However, due to problems of viral contamination upon inspection, FDA discontinued the drug from december 1998 due to deviations from Current Good Manufacturing Practices. Since 2002, it has been reapproved for use in cases of pulmonary embolism. Clinical studies showed that a 12-hour infusion of urokinase followed by heparin had better resolution of pulmonary embolism in the first 24 h of therapy compared to heparin alone.Citation50,51 A dosing regimen of 2,000 IU/lb (4,400 IU/kg) of Abbokinase® is administered at 90 mL/h over 10 min, followed by a continuous infusion of 2,000 IU/lb/hr (4,400 IU/kg/hr) at 15 mL/h for 12 h.Citation5
Second generation plasminogen activators
The driving force behind the development of the second generation plasminogen activators was targeted thrombolysis since the first generation plasminogen activators showed non-specific fibrin degradation and caused systemic fibrinolysis with the concomitant destruction of the hemostatic proteins leading to hemorrhage.
(i) Anistreplase (Anisoylated plasminogen-streptokinase activator complex, APSAC, Eminase)
It is an equimolar complex of streptokinase and human lys-plasminogen (131 kDa) where the active site of plasminogen is acylated using the reversible acylating agent p-amidinophenyl p′anisate hydrochloride (APAN).Citation52 The acylation process renders the complex catalytically inactive, allowing for single bolus administration and also protects it from neutralization by plasmin inhibitors.Citation53 Upon intra-venous administration, the p-anisoyl group is hydrolyzed and the deacylation occurs. Thus, the anistreplase is converted to an active lys-plasminogen-streptokinase complex starting the process of thrombolysis. This strategy allows the drug to be maintained in circulation for a much longer period. In comparison with streptokinase, anistreplase possesses the advantages of enhanced stability in plasma, increased half-life (90–105 min), improved fibrin-binding and a reduced time of administration from 60 min to 2–5 min.Citation52 In particular, its single bolus dosing regimen is ideal for pre-hospital therapy in patients with AMI.Citation53,54 Its safety and efficacy as a thrombolytic drug has been established and is found to be comparable to streptokinase. In the Third International Study of Infarct Survival (ISIS-3) (1992), a significant difference among mortality rate and reinfarction was not observed between streptokinase and APSAC.Citation55 However, being derived from streptokinase, the drug is antigenic and can also induce systemic fibrinogenolysis.Citation52,53 The drug is being manufactured by Wulfing Pharma GmbH under the brand name Eminase® for acute myocardial infarction. In cases of adults suffering from AMI, 30 units of APSAC is administered intravenously over 2–5 min.Citation5
(ii) Pro-urokinase (r-scuPA, saruplase, pro-uPA)
Saruplase is the unglycosylated form of recombinant scu-PA (411 amino acids) produced in E. coli. It was developed as a fibrinolytic agent since scu-PA can mediate clot specific lysis in the presence of fibrin, unlike tcu-PA which does not possess fibrin specificity. Furthermore, it can be cleaved in-vivo to the 2 chain form of urokinase resulting in plasmin generation.Citation56 A recombinant pro-uPA (Prolyse®, Abbot laboratories) has been developed for the treatment of thromboembolic disorders. However, the drug has not yet been approved by the FDA.Citation41 The PROACT trial (Prolyse in Acute Cerebral Thromboembolism) suggested that pro-urokinase combined with heparin enhanced the recanalization efficiency in acute thromboembolic strokes.Citation57 Further, the PROACT II study showed that r-prouPA (intra-arterial) improved clinical outcome at 90 d when administered within 6 h of onset of acute ischemic stroke.Citation58 Further, the COMPASS (Comparative Trial of Saruplase Versus Streptokinase) trial demonstrated that the 30-day mortality rate was similar in the case of saruplase and streptokinase therapy given to patients with AMI.Citation59 The Practical Applications of Saruplase Study (PASS) in Europe assessed that saruplase, in combination with aspirin and intravenous heparin, is safe and effective to be administered to patients with AMI.Citation60 The standard dosing regimen is composed of 20 mg of an initial bolus followed by an infusion consisting of 60 mg over 1 h and preceded by a heparin bolus of 5000 U.Citation56,60 In 1997, the SESAM (Study in Europe with Saruplase and Alteplase in Myocardial Infarction) study suggested that saruplase was as safe and effective as recombinant t-PA during AMI.Citation61 According to the Liquemin in Myocardial Infarction During Thrombolysis With Saruplase (LIMITS) study, the administration of heparin bolus before therapy with saruplase results in a better patency rate without any significant increase in bleeding.Citation62 The PRIMI (Pro-Urokinase in Myocardial Infarction Trial) trial showed no major difference between the activity of saruplase and streptokinase in patients with AMI.Citation63
(iii) Tissue plasminogen activator (t-PA)
t-PA was first identified as an agent derived from animal tissues that could activate plasminogen and was named “fibrinokinase.”Citation64 In 1979, Rijken and coworkers isolated the first highly purified form of t-PA (1 mg) from human uterine tissue.Citation65 The same group further established the fact that t-PA was synthesized by the vascular endothelial cells and is secreted into the blood stream.Citation64 The release is of both types, i.e., constitutive and receptor-mediated occurring in response to factors of the coagulation cascade like factor Xa and thrombin. Further, the rate of synthesis can be enhanced by bradykinin, substance P, TNF-α, desmopressin and is reduced in the presence of plasmin.Citation23 Rijken and Collen (1981) purified and characterized t-PA secreted by a human melanoma cell line (Bowes).Citation66 This cell line efficiently secreted t-PA. The scale-up of purification was done and ∼2 g of t-PA was produced.Citation64 The same cell line was used to clone the t-PA cDNA (2,530 bp) for the first time and it was expressed under the control of the trp promoter in E. coli.Citation67 An efficient, properly processed and glycosylated form of recombinant t-PA (rt-PA) was produced using mammalian cells with properties similar to the Bowes cell line t-PA.Citation68 Majidzadeh-A and co-workers (2010) expressed the gene encoding full-length human tPA under the control of the AOX1 promoter and S. cerevisiae α-mating factor signal sequence in P. pastoris GS115 with an amidolytic activity of 1,650 IU/ml.Citation69
Human t-PA (located on chromosome 8) was first isolated as a 70 kDa (527 amino acids) single chain polypeptide that is converted to the active 2-chain form via plasmin-mediated cleavage across the Arg275-Ile276 bond.Citation43,67 Unlike scuPA, single-chain tPA has significant enzymatic activity and is not a true zymogen.Citation18 The molecule has 35 Cys residues forming 17 disulfide bonds with the remaining free cysteine residue at position 83.Citation70 The t-PA molecule is composed of 4 functional domains: a fibronectin-like domain or finger domain (F-domain) (residues 4–50), an epidermal growth factor-like domain (E-domain) (residues 50–87), 2 kringle domains (K1: residues 87–176; K2: residues 176–256) and a catalytic serine protease domain (P-domain) (276–527) with the catalytic triad His322, Asp371, and Ser478.Citation67,70 The amino-terminal chain houses the E, F and kringle domains while the catalytic domain is C-terminally located. The K2 and F domains mediate the high fibrin affinity of tPA.Citation64 The molecule has 3 potential N-glycosylation sites at residues 117, 184 and 448 and also has an O-linked fucose residue at ThrCitation61. The E and F domains and the carbohydrate side chain residues are responsible for its fast clearance in the hepatocytes with an initial half-life of 6 min.Citation64 The t-PA clearance rate from the hepatocytes may be divided into 2 phases: a first rapid clearance due to the interaction of E and F domains and a second phase due to the presence of glycosylation sites.Citation71 The AsnCitation117 residue in the kringle domain (K1) is recognized by mannose receptors in the liver, leading to its rapid clearance. Further, t-PA lacking the E domain showed an improved half-life in vivo. The replacement of TyrCitation67 in the E-domain reduced its clearance rate. Amino acid alterations in the F domain between residues 42–49 also played a major role in drug clearance.Citation23 The sequence Lys296-His297-Arg298-Arg299 is required for rapid inhibition by PAI-1.Citation72 An advantage of t-PA over other plasminogen activators is its specificity toward fibrin. The presence of fibrin greatly enhances its enzymatic activity. Upon analysis of its kinetic activity, it was revealed that t-PA and plasminogen bind sequentially to the fibrin clot yielding a ternary complex where t-PA has enhanced affinity for its substrate fibrin.Citation64
Several clinical trials have compared the efficacy and safety of rt-PA in stroke.Citation73,74 Administration of intravenous or intracoronary rt-PA induced recanalization of occluded coronary arteries without significant fibrinogenolysis.Citation64 The GISSI-2 (1990) and the ISIS-3 trials (1992) showed no significant differences in terms of effectiveness and safety for the thrombolytic use of rt-PA for AMI patients.Citation55,75 A 100 mg infusion of recombinant t-PA consisting of a 15 mg intravenous bolus, a 50 mg infusion over 30 min and a 35 mg infusion over the next 60 min induced early patency rate of the coronary artery thrombi compared to a 3 h infusion without any adverse reactions and increase in reocclusion rate.Citation76,77 The GUSTO I (Global Utilization of Streptokinase and Tissue Plasminogen Activator to Open Occluded Coronary Arteries, 1993) trial showed reduction in 30 day mortality rate of 14.6 % with the administration of rt-PA using the above-mentioned accelerated dosing regimen combined with intravenous heparin in comparison with streptokinase, the previous standard therapy during myocardial infarction.Citation78,79 During the COBALT (Continuous Infusion versus Double-Bolus Administration of Alteplase) trial, a double bolus of rt-PA was administered (2 boluses given 30 min apart) and was not found to be as effective as the accelerated infusion regimen due to a higher incidence of intracranial hemorrhage during AMI.Citation80
The recombinant t-PA (alteplase) is produced for commercial purposes in CHO cell lines under the trade name Activase® (Genentech, Inc.) and has been approved by FDA for the treatment of AMI, acute ischemic stroke, and pulmonary embolism patients. The drug, under the trade name of Actilyse® (Boehringer Ingelheim GmbH), has been approved by the EMA (European Medicines Agency) for the same purpose. In the developing countries like India, alteplase is being used for intervention during the acute ischemic stroke and acute pulmonary embolism. For acute ischemic stroke, a dose of 0.9 mg/kg is administered (not to exceed 90 mg total dose), with 10% of the total dose given intravenously over 1 min and the rest was infused over 60 min. In the case of acute myocardial infarction, 2 regimens may be allowed. In the 3 h infusion regimen, for patients weighing ≥ 65 kg, a total of 100 mg t-PA is administered as 60 mg in the first 60 min (6–10 mg administered as a bolus), 20 mg in the second hour, and 20 mg in the third hour while for patients weighing < 65 kg, a dose of 1.25 mg/kg is administered over 3 h. In the accelerated infusion regimen: for patients weighing > 67 kg, a dose of 100 mg t-PA is administered as an intravenous bolus of 15 mg followed by 50 mg infusion over the first 30 min and 35 mg in the next 60 min while for patients weighing ≤ 67 kg, an initial 15 mg intravenous bolus is given followed by 0.75 mg/kg for the first 30 min and 0.50 mg/kg for the next 60 min. The recommended dose for pulmonary embolism is 100 mg administered by intravenous infusion over 2 h.Citation5
Third generation plasminogen activators
This category includes t-PA variants that have been engineered to improve the structural and functional properties like longer half-life, resistance to inhibitors, safety and improved efficacy along with enhanced fibrin specificity. Their long clearance rates allow easy administration in one or 2 boluses. This class of plasminogen activators also includes new molecules of bacterial (staphylokinase) and animal (desmoteplase) origin.
(i) Reteplase (recombinant plasminogen activator, r-PA, BM 06.022)
It is an unglycosylated single chain t-PA deletion variant produced in E. coli K12 in the form of insoluble inclusion bodies. It consists of the residues 1–3 and 176–527 of t-PA. The deletion of the Val4-GluCitation175 region indicates that r-PA is devoid of the finger, epidermal growth factor and kringle 1 domains of t-PA. Thus, the molecule is composed only of the kringle 2 and the catalytic protease domains of t-PA and has a molecular weight of 39 kDa (355 residues).Citation81,82 The kringle 1 domain of t-PA is responsible for its rapid clearance in the liver. Thus, the loss of the kringle 1 domain in r-PA increased the half-life of this molecule from 4 to ∼15 min with mainly renal form of elimination.Citation23,83 Further, other characteristics, like inhibition by PAI-1, activity in the absence of fibrin and conversion between single-chain and 2-chain forms seems to be similar to alteplase.Citation81,82 However, its fibrin binding affinity is 5-fold lesser than alteplase because of the absence of the fibrin-binding finger domain.Citation81,84 Despite of the presence of the kringle 2 domain in the molecule that is known to interact with fibrin and stimulate the activity, the fibrinolytic activity of r-PA is 4-fold lower than alteplase. A double bolus regimen (10 + 10 mega units after 30 min) of r-PA administered to patients with AMI helped in complete, speedy, and sustained thrombolysis in comparison with the standard dose of t-PA (front-loaded accelerated infusion or standard infusion).Citation85,86 This strategy helped to overcome the necessity of a continuous intravenous infusion. The double bolus regimen of r-PA was found to be as effective as the standard dosage of streptokinase (1.5 million units given intravenously over 60 min) for the treatment of AMI (The INJECT Trial, 1995).Citation87 Furthermore, in the GUSTO III (The Global Use of Strategies to Open Occluded Coronary Arteries) trial, the mortality rate and frequency of hemorrhagic stroke after 30 d seems to be comparable in alteplase and reteplase (The GUSTO III Trial, 1997).Citation88
In the US and Europe, reteplase is marketed under the trade name of Retavase® (Centocor, Inc.) and Rapilysin® (Roche) respectively, for the treatment of AMI condition where it is given as 2 intravenous boluses of 10 units each with a gap of 30 min.Citation5
(ii) Tenecteplase (TNK-tPA)
It is a variant of t-PA with 3 point mutations {(i) ThrCitation103 with Asn, (ii) AsnCitation117 with Gln and (iii) Lys296-His297-Arg298-Arg299 each replaced with alanine} and a molecular weight of 65 kDa (527 residues).Citation89 The first mutation at position 103 introduces a glycosylation site while the second mutation deletes the glycosylation site within the kringle 1 domain which also prolongs its half-life to about 10–24 min since it is partly responsible for t-PA elimination.Citation90 Owing to its slow clearance rate, a single bolus regimen can be administered simplifying its application outside of a hospital setting.Citation91 The third point mutation with alanine, from residues 296–299, provides 80-fold higher resistance against inhibition by PAI-1. The molecule also possesses 14-fold enhanced fibrin specificity than t-PA but comparable fibrin-binding affinity and plasma clot lysis activity in vivo.Citation89 Clinical trials showed no difference between t-PA and TNK-tPA in reducing infarct mortality during AMI, however, there was a decrease in major bleeding rate in comparison with t-PA.Citation92 In the TIMI 10B (Thrombolysis in Myocardial Infarction) trial a single bolus administration of TNK-tPA (40 mg) produced the same patency rate at 90 min of thrombolytic therapy as the accelerated regimen of alteplase.Citation93 In the ASSENT-1 (Assessment of Safety and Efficacy of a New Thrombolytic agent) trial, a single bolus (30 to 50 mg) administration of tenecteplase was found to be as safe as the accelerated alteplase administration regimen in the treatment of patients with AMI.Citation94 Similarly, in the phase III ASSENT-2 trial, t-PA and TNK-tPA showed a comparable mortality rate after 30 d during treatment of AMI patients.Citation95 The drug is produced in the CHO cell line and is commercially available in the US and European market under the trade names of TNKase™ (Genentech, Inc.) and Metalyse® (Boehringer Ingelheim GmbH) respectively. It is used for the treatment of AMI where a single intravenous weight-based bolus dose of ∼0.5 mg/kg is administered over 5 s.Citation5
(iii) Duteplase (met-t-PA)
This two-chain form of t-PA produced in CHO cells has a single amino acid substitution at position 245 where valine is replaced by methionine. It has a lower in vitro specific activity in comparison with alteplase. For AMI, a weight adjusted infusion of duteplase combined with oral aspirin and intravenous heparin was found to be as effective and safe as alteplase in the ESPRIT study.Citation96
(iv) Monteplase (E6010)
Monteplase is a modified t-PA molecule in which the CysCitation84 in the epidermal growth factor domain is substituted with serine. It has a prolonged half-life (23 min) which in turn allows its administration as a single intra-venous bolus dose.Citation97,98 TIMI (Thrombolysis in Myocardial Infarction)-3 flow rates of 69% were obtained after 60 min in patients with AMI.Citation23 In a trial, comparing E6010 with tisokinase (native t-PA version of Japan), E6010 produced a higher rate of early recanalization without fatal bleeding complications when given as a single-bolus (0.22 mg/kg) injection over 2 min during the treatment of AMI.Citation98 In 2000, its usefulness was shown in a canine model of acute pulmonary thromboembolism.Citation99 The clinical usefulness of this molecule has been seen in studies where pretreatment with monteplase with a timely transfer to a tertiary center for coronary angioplasty could be used as an effective approach for the treatment of AMI.Citation100 Similar studies conducted earlier using the conventional thrombolytic agents did not provide any benefits.Citation101 This may be due to PAI-1 independent kinetics of monteplase.Citation102 The PAI-1 activity is enhanced during the use of conventional thrombolytic agents leading to re-occlusion. In a rat model, monteplase was shown to be a promising thrombolytic for the treatment of cerebral ischemia.Citation103 In 2010, its effective use for deep vein thrombosis treatment was demonstrated.Citation104
(v) Lanoteplase (novel plasminogen activator, n-PA)
This molecule lacks the finger and epidermal growth factor domains (Cys6-IleCitation86) along with the substitution of AsnCitation117 in the kringle 1 domain with Gln resulting in the deletion of the glycosylation site. These modifications have helped in increasing the molecule's residence time to 37–45 min, thus allowing a single-bolus regimen.Citation71 n-PA is a single chain, 53.6 kDa molecule produced in CHO cells.Citation23 A single bolus regimen of n-PA (120 kU/kg) increased coronary patency rate at 90 min in comparison with an accelerated alteplase regimen with a comparable mortality at 30 d.Citation105,106 However, n-PA showed more mild bleeding with a higher rate of hemorrhagic stroke than alteplase.Citation23
(vi) Pamiteplase (YM866, Solinase)
This is another derivative of t-PA with a deletion of the kringle 1 domain (residues 92–173) and a substitution of Arg274 in the cleavage site of sct-PA with Glu preventing its cleavage by plasmin.Citation107 Its half-life is about 30–47 min and is dose-dependent. Its in vitro fibrin affinity is more pronounced and the specific activity in vitro is the same as of t-PA.Citation23 An intravenous bolus injection of YM866 administered in a canine model of coronary thrombosis exerted a superior thrombolytic effect than t-PA without systemic activation of fibrinolysis in comparison with t-PA.Citation108 A clinical trial conducted in Japan comparing palmiteplase with t-PA showed that palmiteplase had TIMI-3 flow rates of 25% at 30 min (16% for t-PA) and 50% after 60 min (48% for t-PA), with similar thrombolytic effects.Citation23
(vii) Amediplase (CGP 42935, K2tu-PA, MEN 9036)
It is a hybrid plasminogen activator composed of the kringle 2 domain of t-PA (1–3+ 176–275) and the catalytic protease domain of scu-PA (159–411). The molecule has a longer plasma half-life of over 30 min.Citation109 It has a molar mass of 39.9 kDa (365 amino acids) and is produced in CHO cells.Citation110 Amediplase binding to fibrin is significantly lower than alteplase, however, it shows better clot penetration.Citation111 It was found to be more active than TNK-tPA and scu-PA in an external plasma clot lysis model.Citation112
(viii) Desmoteplase (Bat-PA, DSPAα1, v-PA)
A 477 amino acid single chain enzyme, desmoteplase, was isolated from the saliva of the vampire bat Desmodus rotundus in 1991. It is one among a group of 4 plasminogen activators found in bats collectively named Desmodus salivary plasminogen activators (DSPAS).Citation8 DSPAα1 is the largest (50 kDa) and most potent of the 4 plasminogen activators with a half-life of 2.8 h. It has been recombinantly produced using mammalian (CHO) and insect cells.Citation23 It is composed of a finger, EGF and a kringle domain.Citation8 Its kringle domain is similar to the kringle 1 domain of t-PA without any lysine binding site. Further, it exists in the single chain form due to the absence of any plasmin cleavage site in the protease domain.Citation113 The molecule is almost inactive in the absence of fibrin. However, the presence of fibrin and fibrinogen enhances its activity by 12,900 fold, which is much higher than that for t-PA.Citation113 Furthermore, bat-PA is inhibited by tranexamic acid, a synthetic lysine analog, that can be used to control any bleeding complications upon its administration.Citation114 In a canine model of arterial thrombosis, the bat-PA promoted effective, rapid and sustained reperfusion without systemic fibrinogenolysis in comparison with t-PA.Citation115 The use of bat-PA in the treatment of acute ischemic stroke does not promote excitotoxic injury which has been implicated during the administration of t-PA.Citation116 Desmoteplase administered after 3–9 h of symptom onset during the treatment of acute ischemic stroke showed a higher rate of reperfusion and better clinical outcomes compared with placebo.Citation117 125µg/kg was the most effective dose of desmoteplase for efficient reperfusion. The DIAS-2 (The Desmoteplase in Acute Ischemic Stroke) study, however, did not show any benefit of desmoteplase.Citation118 The phase III clinical trials of desmoteplase (DIAS-3) showed that desmoteplase is safe, but did not improve functional outcomes when administered to patients after 3–9 h of ischemic stroke.Citation119
(ix) Staphylokinase (SAK)
It is a 15.5 kDa (136 residues) single chain polypeptide that is secreted by certain strains of Staphylococcus aureus. The molecule does not possess any disulfide bond. Similar to streptokinase, SAK forms a 1:1 stoichiometric complex with plasmin or plasminogen to activate other plasminogen molecules. However, unlike SK, plasminogen in the complex has to be first activated to plasmin by other plasminogen activators in order to form an active complex, which then activates remaining plasminogen molecules in an exponentially kinetic manner. There is a 4-fold enhancement of plasminogen activation in the presence of fibrin.Citation120 SAK binds preferentially to plasminogen, which is bound to partially degraded fibrin.Citation121 Unlike SK, the Pg-SAK complex is rapidly neutralized in the absence of fibrin by α 2-antiplasmin thereby preventing systemic activation of plasminogen. This inhibition is overcome in the presence of fibrin due to the occupancy of the lysine-binding sites of the Pg-SAK complex, thereby allowing preferential plasminogen activation at the fibrin surface.Citation120 The SAK-plasmin complex formation is favored since SAK has 160 times higher affinity for plasmin than plasminogen.Citation121 SAK-plasmin modifies SAK by cleaving it at the Lys10-LysCitation11 peptide bond.Citation122 The amino acid at position 26 i.e. methionine seems to be important for plasminogen activation.Citation123 Deletion or substitution of the LysCitation11 residue abolished its activator function.Citation124 Substitution of LysCitation11, AspCitation13, and AspCitation14 with Ala results in a mutated SAK that binds plasmin(ogen) but does not activate soluble plasminogen. Similarly, substitutions of GluCitation46, LysCitation50, GluCitation65 and AspCitation69 with alanine yielded mutants with impaired activation ability.Citation125 Szemraj and coworkers (2005) created a staphylokinase variant, SAK-RGD-K2-Hir by introducing the K2 domain of t-PA, the Arg-Gly-Asp tripeptide for preventing aggregation of platelets and the thrombin inhibitor hirudin to the C-terminus of staphylokinase.Citation126 Recently, another variant SAK-RGD-Hirulog has been developed with thrombin inhibition and antiplatelet aggregation abilities.Citation127 The recombinant fusion protein was more potent and faster-acting thrombolytic agent than rSAK (recombinant staphylokinase) with antithrombin and antiplatelet activities. Administration of recombinant staphylokinase via the intra-arterial route restores patency in cases of peripheral arterial occlusion without fibrinogen degradation.Citation128 The STAR trial showed that recombinant staphylokinase seems to be as effective as rt-PA for early coronary recanalization during AMI.Citation129 During the trial, 100 patients were administered either of the 2 staphylokinase doses (10 mg or 20 mg given intravenously over 30 min) or accelerated t-PA. It was found that staphylokinase treatment was not associated with systemic fibrinogenolysis. A double-bolus administration of recombinant staphylokinase was found to be safe and effective for coronary artery recanalization.Citation130 Double bolus staphylokinase (15 mg + 15 mg given 30 min apart) was found to be more efficient and fibrin-specific than an accelerated regimen of rt-PA. Further, it did not induce any allergic reactions.Citation131 The therapeutic potential of staphylokinase has also been confirmed by the CAPTORS I and II trials (Collaborative Angiographic Patency Trial of Recombinant Staphylokinase).Citation132,133
Other Thrombolytics/Plasminogen activators
Plasminogen activators from snakes
Snake venoms contain a great variety of proteases exhibiting fibrinolytic properties. Isolated from the venom of the Chinese green tree viper snake Trimeresurus stejnejeri, the TSV-PA is the prototypical plasminogen activator obtained from snakes. The venom also contains other fibrinogenolytic enzymes, namely stejnefibrase-1, 2 and 3 and the fibrin clotting enzyme stejnobin.Citation9 TSV-PA is a single-chain glycoprotein of 234 amino acids (33 kDa) with a pI of 5.2 and possesses a single potential N-glycosylation site at AsnCitation161. The molecule bears 21–23% sequence similarity with the u-PA and t-PA catalytic domains. It contains 6 disulfide bonds and HisCitation41, AspCitation86 and SerCitation180 form the catalytic triad in TSV-PA. Further, TSV-PA lacks the sequences responsible for interaction of t-PA (KHRR; Lys-His-Arg-Arg) and u-PA (RRHR; Arg-Arg-His-Arg) with PAI-1. The recombinant form of this molecule has been produced using E. coli.Citation134 Haly-PA is a 234 amino acids plasminogen activator isolated from the venom of Agkistrodon halys which shows 82% similarity to TSV-PA. It has 30-fold lesser activity than u-PA. The molecule has been expressed as a 32 kDa glycoprotein using the baculovirus expression system. Similar to TSV-PA, it lacks domains for interaction with PAI-1 and thus has a prolonged half-life.Citation135 The LV-PA plasminogen activator (33 kDa) was isolated from the bushmaster snake, Lachesis muta muta with 90% sequence identity with TSV-PA and 85% with Haly-PA.Citation136
Chimeric thrombolytic agents
(i) GHRP-scu-PA-32K
A chimeric plasminogen activator was created by fusing the GHRP (Gly-His-Arg-Pro) tetrapeptide to the 32 kDa low molecular weight form of scu-PA. It was expressed in CHO cells with a specific activity of 150,000 IU/mg. The molecule showed 2.5-fold enhanced fibrin binding, higher thrombolytic potency and less fibrinogen depletion in plasma compared with the native low molecular weight scu-PA (144–411).Citation137
(ii) GHRP-SYQ-K2S
A novel, chimeric truncated t-PA variant (394 amino acids) was developed which contains the K2 kringle domain and the serine protease domain (K2S). SYQ, the first 3 residues of t-PA are retained as they are essential for protein activity. Further, to compensate for the loss of fibrin affinity due to the deletion of the finger domain the chimeric tetrapeptide (GHRP, Gly-His-Arg-Pro) was introduced upstream of SYQ-K2S. It possesses 86% of the fibrin binding capacity of t-PA while reteplase has only 30%. The recombinant expression of the chimera in CHO cells yielded 752 IU/mL (566,917 IU/mg) of recombinant protein.Citation138
The future of thrombolytic therapy
Direct thrombolytic agents
The intravenous (systemic) administration of plasminogen activators for thrombolysis has been found to be associated with an unavoidable bleeding risk since it exceeds the inhibitory capacity of PAI-1. On the other hand, catheter-based (local) administration of plasminogen activators helps in achieving thrombolysis faster, but bleeding complications may still result since the local concentration of the plasminogen activator may exceed the PAI-1 inhibitory capacity.Citation16 In such a scenario, the attributes of direct-acting thrombolytics can be exploited. Direct thrombolytic agents are proteolytic enzymes that do not require the intermediate step of plasminogen activation and degrade fibrin directly. Plasmin is the prototypical form of such direct acting thrombolytic agents. When administered systemically, plasmin is rapidly inhibited by α2-antiplasmin and does not dissolve the thrombus while also not inducing hemorrhagic complications. Thus, plasmin can be safely administered systemically but is not effective.Citation139 In contrast, catheter-based delivery of plasmin allows it to remain shielded from α2-antiplasmin and carry out thrombolysis. Subsequently, upon its release from the thrombus and entry into the circulation, the plasmin may be rapidly neutralized by α2-antiplasmin thereby preventing the risk of hemorrhage. Thus, direct acting thrombolytics have potential for improved thrombolysis and enhanced hemostatic safety.Citation16,139
In a porcine model, plasmin was found to possess superior characteristics than t-PA in dissolving arteriovenous graft thrombi.Citation140 Several studies conducted in animal models indicate the effectiveness and safety of plasmin administration for thrombolytic therapy.Citation141,142 In a phase I human trial, 24 mg plasmin (Talecris Biotherapeutics, Inc.) effectively induced thrombolysis of hemodialysis graft occlusions.Citation143
Several plasmin derivatives have also been created for application as direct thrombolytics. Mini-plasminogen is a truncated form of plasminogen derived from a limited proteolysis of plasminogen by the action of porcine pancreatic elastase. This gives rise to 3 functional fragments: kringles 1 to 3, kringle 4 and mini-plasminogen (digestion at Val441-Val442 peptide bond). The molecule is composed of the catalytic protease domain and the kringle 5 domain of plasminogen.Citation16 Mini-plasminogen has been expressed in E. coli as inclusion bodies (500–600 mg/L) using the T7 expression system. The inclusion bodies were refolded, purified and activated to mini-plasmin using urokinase.Citation144 The kinetic properties of mini-plasmin were comparable to that of native plasmin. Moroz (1981) showed that mini-plasmin exhibited almost twice the fibrinolytic activity of plasmin.Citation145 However, in a different study, the fibrinolytic catalytic efficiency (Kcat/Km) for miniplasmin was found to be 1.9 × 105 M−1s−1 in comparison with 4.3 × 105 M−1s−1 for plasmin.Citation146 The molecule is efficiently inhibited by α2-macroglobulin but reacts slowly with α2-antiplasmin. It effects fibrin binding via the kringle 5 domain.Citation14 In a canine model of femoral artery thrombosis, the use of miniplasmin showed a higher incidence of reperfusion and faster thrombolysis than rt-PA without the hemorrhagic complications associated with the latter.Citation147
Another plasmin derivative, micro-plasmin (∼29 kDa) is a 2 chain variant generated upon autocatalytic cleavage of plasmin at the Arg529-Lys530 bond in an alkaline solution (pH 11.0).Citation148 Micro-plasminogen has been produced in E. coli as inclusion bodies (500–600 mg/L) from where it was isolated, purified and activated to micro-plasmin using urokinase.Citation144 The molecule has been recombinantly expressed in E. coli, Pichia pastoris, and insect cells.Citation149,150,151 It showed 100 times lesser inhibition by α2-antiplasmin than plasmin due to the absence of the lysine binding sites in micro-plasmin.Citation150 It does not have any kringle domain and thus lacks fibrin affinity. Its main inhibitor is α2-macroglobulin.Citation16 It appears to have a comparable potency to full-length plasmin in vivo.Citation150 Micro-plasmin has been shown to reduce cerebral ischemic damage in animal models with lesser hemorrhage than t-PA.Citation152,153 During clinical trials too, intravenous microplasmin was generally well tolerated in patients with acute ischemic stroke.Citation154 Catheter malfunction due to thrombotic occlusion was overcome safely and effectively using microplasmin without any bleeding complications.Citation155 Several studies showed the usefulness of intravitreal microplasmin administration to induce detachment of the vitreous cortex from the retina.Citation156,157 The drug has been produced in P. pastoris and is marketed commercially under the trade name of Jetrea® (ocriplasmin, ThromboGenics, Inc.). It was approved by FDA in 2012 for use in symptomatic vitreomacular adhesion.Citation5
In 2008, a deletion mutant of plasminogen was developed called delta plasminogen (TAL6003; Talecris Biotherapeutics, Inc.) composed of the kringle 1 and serine protease domain of plasminogen. It has been recombinantly expressed in E. coli using the T7 expression system at a level of 200 mg/L in the form of inclusion bodies. The molecule showed similar properties to full-length plasminogen. Delta plasmin exhibited fibrinolytic potency comparable to plasmin and was rapidly inhibited by α2-antiplasmin and α2-macroglobulin.Citation158
The venom of the southern copperhead snake Agkistrodon contortrix contortrix contains a direct acting single chain fibrinolytic zinc metalloprotease called fibrolase (203 residues). Alfimeprase, a recombinant fibrolase derivative is 6 times more active than t-PA and urokinase and does not induce systemic fibrinolysis.Citation159 In alfimeprase, the 3 N-terminal residues of fibrolase (EQR) have been replaced by a single S (Ser) residue without any effect on the fibrinolytic activity. The molecule was developed by Amgen Inc., Thousand Oaks, CA and is being produced by Nuvelo for commercialization. Alfimeprase mediates fibrinolysis via cleavage of the Aα chain of fibrin at Lys413-Leu414 bond. It is inhibited by α2-macroglobulin.Citation160 In a canine model of arterial thrombosis, intracoronary administration of alfimeprase administered (0.5 mg/kg over 1 min) to the left circumflex coronary artery of dogs induced rapid reperfusion without any myocyte reperfusion injury in comparison with t-PA.Citation161 Alfimeprase is safe for human administration and upto 0.5 mg/kg has been seen to be well tolerated in patients with chronic peripheral arterial occlusion.Citation162 1 or 3 mg of alfimeprase had the potential to restore the function of occluded central venous access devices.Citation163 In a phase 2 multicenter, randomized, double-blind study (NuCath), alfimeprase (1 mg and 3 mg) restored 50% catheter patency in comparison with 0% for Cathflo®Activase® (Genentech) at 15 min without any hemorrhagic events.Citation164
From the venom of the snake Vipera lebetina, a direct fibrinolytic enzyme with low hemorrhagic activity, Lebetase, has been isolated.Citation165 A fibrinolytic enzyme (lumbrokinase) from the earthworm Lumbricus rubellus was isolated in 1991.Citation7 Another direct acting fibrinolytic, thrombinase, is derived from Bacillus sphaericus sero type H5a and 5b. This thrombolytic enzyme (32 kDa) acts directly like plasmin and can dissolve blood clots faster than u-PA, t-PA or SK.Citation23 In 2009, Wu and co-workers isolated Fu-P (chymotrypsin-like serine metalloprotease), a novel fibrinolytic enzyme from Fusarium sp. CPCC 480097 from the stems of chrysanthemums.Citation166 Several direct acting fibrinolytic enzymes have also been isolated from the genus Bacillus including Subtilisin DJ-4 (Bacillus amyloliquefacien), subtilisin DFE (B. amyloliquefacines) and subtilisin CK (Bacillus sp strain CK-11-4).Citation23
Serrapeptase (SP), a proteolytic enzyme produced by Serratia sp. E-15, is isolated from the intestine of the silkworm Bombyx mori.Citation4 It is a Zn2+ metalloprotease with a molecule weight of ∼60 kDa. It helps the silkworms to digest their cocoons but does not affect the living tissue. Thus, it is useful in the degradation and removal of cholesterol, fibrin and cellular waste products in the arteries. The enzyme can also dissolve blood clots, necrotic tissue, inflammatory factors and arterial plaques. It acts synergistically with antibiotics by liquefying bio-films produced by bacteria to protect themselves from the action of antibiotics indicating its role as a mucus liquefying agent.Citation4 It was found that in patients with chronic airway diseases, 30 mg of serrapeptase daily for 4 weeks reduced sputum weight and neutrophil count as well as its viscosity and elasticity.Citation167
Fermented boiled soya beans (natto, part of Japanese diet) release a fibrinolytic substance called nattokinase (NK) (EC 3.4.21.62). NK has been found to have the highest fibrinolytic activity among all food-based fibrinolytic agents.Citation4 It is a single chain 27.7 kDa polypeptide (275 amino acids) with a pI of 8.6. It shows maximum activity in the pH range of 6 to 12 and the activity drops beyond the temperature of 60°C. About 50 g of natto produces the same effect as a urokinase therapy costing 200,000 Japanese yen. Further, NK maintains activity up to 12 h while the effectiveness of urokinase last for only about 4 to 20 min.Citation168 NK may help in preventing conditions leading to blood clot with a daily oral dose of as low as 2,000 FU (50 g) of natto.Citation4 Unlike other molecules, nattokinase improves the body's ability to fight the development of clots. Further, it possesses advantages of efficacy, oral administration, prolonged effects, low price, stability in the alimentary tract, direct fibrinolytic action and enhances the body's production of plasmin and physiological plasminogen activators like u-PA and t-PA. The molecule not only cleaves fibrin directly, but it also induces production of t-PA that results in an increased plasmin generation. Furthermore, NK cleaves and inactivates PAI-I.Citation6 Blood clots induced in the major leg vein of male dogs were dissolved and normal circulation was restored within 5 h of administration of 4 capsules (250 mg/ capsule) of nattokinase.Citation169 NK was found to have a higher thrombolytic activity than plasmin or elastase in vivo (rat model).Citation4,168
Plant proteases: the natural sources of fibrinolytic enzymes
Natural products may be good alternatives to the currently used thrombolytic agents.Citation10 The herbal preparations have been in use since ancient times to treat diseases with minimal side effects. Being a normal part of many diet routines, their ease of administration is their most attractive feature. Plant lattices are also a rich source of hydrolytic enzymes that play a key role in host-pathogen interactions.Citation170 The majority of these enzymes belong to the serine and cysteine protease family. Using an in vitro model of thrombosis, the herb Fagonia arabica exhibited 75.6% clot lysis in comparison with streptokinase (86.2%).Citation171 In a similar study conducted by Rahman and coworkers (2013) Clausena suffruticosa, Leea indica, and Leucas aspera were shown to possess effective thrombolytic properties.Citation172 In 2015, Emran and co-workers showed the potential of Trema orientalis L., Bacopa monnieri L., and Urena sinuate L. in clot lysis using streptokinase as a control.Citation173 A 34 kDa serine protease, hirtin, purified from the latex of the medicinal herb Euphorbia hirta showed fibrinolytic and fibrinogenolytic activities.Citation174 Late of the plant genus Euphorbia is known to have serine proteases.Citation175 Rajesh and coworkers (2007) describe the clot-inducing and dissolving properties of proteases present in plant latex. Extracts from the latex of Wrightia tinctoria R. Br. (Apocynaceae) and Synadenium grantii Hook. f. (Euphorbiaceae) contain serine proteases that exhibited procoagulant and fibrinolytic activity.Citation176 Such activity was also demonstrated in the cysteine protease-containing lattices of Calotropis gigantea R.Br., Cynanchum puciflorum R.Br., Pergularia extensa R.Br. and Asclepias curassavica L. belonging to the Asclepiadaceae family.Citation176,177 Eumiliin, from the latex of Euphorbia milii var. hislopii (Euphorbiaceae), showed fibrinogen hydrolysis in vitro.Citation178 Cysteine protease rich extracts of fruits like Pseudananas macrodontes, Bromelia balansae, and B. hieronymi showed fibrinolytic activity.Citation179 The fibrinolytic effect of Litchi chinensis Sonn. extract (Sapindaceae) has been discussed by Sung and co-workers.Citation180 A serine protease, AMP48, isolated from the jackfruit (Artocarpus heterophyllus) latex showed maximum fibrinogenolytic activity at pH 8.0 between 55 and 60°C.Citation181 Similarly, cysteine proteases from the lattices of Cryptostegia grandiflora and Plumeria rubra exhibited thrombin and plasmin-like activities.Citation182 EuP-82, a serine protease from Euphorbia cf. lactea latex could digest fibrinogen.Citation183 Such studies support the conventional usage of plant latex for wound healing and also as potential sources to treat various hemostatic disorders. With more advanced research the fibrinolytic efficacy and safety of these natural products may be tested for their use in therapy.
Liposome-based plasminogen activators
The use of plasminogen activators has issues of stability, immunogenicity, drug toxicity and non-specific fibrinolysis during administration in the conventional manner. Delivery of plasminogen activators via nanoparticles could be used to increase their therapeutic efficacy by overcoming such limitations. Liposome based nanocarriers are non-toxic spherical vesicles resembling membranes that can be used for encapsulation or adsorption of the plasminogen activator. Furthermore, the liposomal encapsulated plasminogen activators may be targeted to the sites of thrombi via the intravenous route preventing their degradation by plasma components due to their limited exposure.Citation184 The liposomally entrapped streptokinase when used in a rabbit model of thrombolysis showed a 47.4 +/− 1.4% of clot lysis against 36.3 +/− 3.4% for free streptokinase. The streptokinase immunized rabbits containing anti-SK antibodies also showed a slightly reduced thrombolytic activity of 33.8 ± 1.5%. However, it was still comparable to free SK in non-immunized rabbits. Thus liposomal streptokinase can be used as an alternate strategy to overcome the limitations of anti-SK antibodies in vivo.Citation185 The biological half-life of PEGylated liposomal SK increased 16-fold compared with that of free SK.Citation186 A liposomal encapsulated formulation of streptokinase administered to a canine model of myocardial infarction, reduced the reperfusion time by 50% in comparison to free streptokinase, moreover, the total dose of liposomal streptokinase used was also lower.Citation187 Platelets play an important role in blood coagulation by formation of the primary platelet plug. The αIIbβ3 integrin composed of the glycoproteins IIb/IIIa (GPIIb/IIIa) is the major integrin present on platelets and its activation allows high affinity platelet binding to fibrinogen in the plasma. Thus αIIbβ3 integrin receptor-specific peptides (RGD, Arg-Gly-Asp) can be used for targeted delivery of thrombolytic agents. The use of RGD-PEGylated liposomal SK and PEGylated liposomal SK prolonged the circulation of SK by 5- and 5.5-fold, respectively, compared with free SK. Furthermore, the RGD peptide conjugated liposomes were targeted specifically to the site of the blood clot where a higher thrombolytic activity was achieved.Citation188 A PEGylated liposomal formulation of streptokinase was also found to enhance its systemic circulation.Citation189 The PEGylated t-PA loaded liposomes had an enhanced half-life of 141 min. Further, attachment of the peptide sequence, i.e CQQHHLGGAKQAGDV of the fibrinogen γ chain that binds to the GPIIb/IIIa integrin expressed on activated platelets caused a 35% increase in clot lysis and 4.3-fold less fibrinogenolysis.Citation190 Liposomal formulation of urokinase enhanced its half-life and promoted targeted delivery.Citation191 An RGD-PEGylated liposomal formulation of urokinase enhanced thrombolysis in a rat carotid artery model in comparison with free urokinase.Citation192 A urokinase liposome incorporated with the D-dimer monoclonal antibody (DDmAb) was developed and its efficacy was studied in a rabbit model of pulmonary embolism. At 80 min post administration, the right ventricular pressure was restored to the normal value with reduction in emboli size.Citation193 Thus, monoclonal antibody-based liposomal urokinase can be an effective approach for targeted thrombolysis. Further, temperature-sensitive liposomes have been developed that release their contents following hyperthermia. SAK, u-PA and t-PA were loaded into such thermosensitive vesicles and their thrombolytic efficacy was analyzed after hyperthermia. The SAK formulation showed maximum clot lysis in comparison with the other plasminogen activators.Citation194 Encapsulation of plasmin in echogenic liposomes that release their contents at the clot site in response to ultrasound have been developed so as to protect plasmin from its inhibitors in the plasma. Clot lysis was carried out using such echogenic liposomes loaded with plasmin in the presence and absence of ultrasound. A 31% higher clot lysis was obtained during ultrasound-mediated thrombolysis.Citation195 The liposomal formulation of plasminogen activators has been reviewed recently.Citation184
Conclusions
Thrombolytic agents are life-saving drugs that can dissolve thrombi and achieve reperfusion. However, the use of such agents is determined by several factors like immunogenicity, fibrin-specificity, ease of administration, drug's half-life and cost of treatment. Hence, there is a constant urge of developing novel molecules at lower cost to address these problems. Tissue plasminogen activator (t-PA) is popular in the developed nations, however, due to its higher cost, streptokinase remains the drug of choice for thrombolytic therapy in the developing countries. The recombinant DNA technology and bioprocess optimization strategies are helping in providing these drugs to patients at reduced cost. These drugs are produced in different expression platforms such as bacterial, yeast to mammalian cells to mimic their biological activity. Recently, the development of recombinant thrombolytics majorly focuses on enhancing their efficacy, stability and half-life so as to allow their administration in one or 2 bolus injections. Therefore, third generation molecules are developed to have an effective administration regimen that allows a pre-hospital therapy thus reducing the mortality rates. The emergence of direct fibrinolytic agents has shown exciting outcomes with effective results in clinical settings. The oral administration of nattokinase also presents a viable and cost effective alternate as a potent thrombolytic therapy. The targeted thrombolysis using liposomes is an attractive concept toward directed dissolution of blood clots. However, its rigorous clinical testing needs to be done to understand its futuristic implications.
Disclosure of potential conflicts of interest
No potential conflicts of interest were disclosed.
Funding
This work was financially supported by R&D grant of University of Delhi. Adivitiya is the recipient of senior research fellowship from Council of Scientific and Industrial Research (CSIR), Govt. of India, New Delhi.
References
- Banerjee A, Chisti Y, Banerjee UC. Streptokinase–a clinically useful thrombolytic agent. Biotechnol Adv 2004; 22(4):287-307; PMID:14697452; https://doi.org/10.1016/j.biotechadv.2003.09.004
- Global status report on non-communicable diseases, 2014. World Health Organization; http://www.who.int/cardiovascular_diseases/en/
- Esmon CT. Basic mechanisms and pathogenesis of venous thrombosis. Blood Rev 2009; 23(5):225-9; PMID:19683659; https://doi.org/10.1016/j.blre.2009.07.002
- Kotb E. The biotechnological potential of fibrinolytic enzymes in the dissolution of endogenous blood thrombi. Biotechnol Prog 2014; 30(3):656-72; PMID:24799449; https://doi.org/10.1002/btpr.1918
- US Food and Drug Administration home page. www.fda.gov. Accessed 13 October 2016.
- Kotb E. Activity assessment of microbial fibrinolytic enzymes. Appl Microbiol Biotechnol 2013; 97(15):6647-65; PMID:23812278; https://doi.org/10.1007/s00253-013-5052-1
- Mihara H, Sumi H, Yoneta T, Mizumoto H, Ikeda R, Seiki M, Maruyama M. A novel fibrinolytic enzyme extracted from the earthworm, Lumbricus rubellus. Jpn J Physiol 1991; 41(3):461-72; PMID:1960890; https://doi.org/10.2170/jjphysiol.41.461
- Kratzschmar J, Haendler B, Langer G, Boidol W, Bringmann P, Alagon A, Donner P, Schleuning WD. The plasminogen activator family from the salivary gland of the vampire bat Desmodus rotundus: cloning and expression. Gene 1991; 105(2):229-37; PMID:1937019
- Gao R, Zhang Y, Meng QX, Lee WH, Li DS, Xiong YL, Wang WY. Characterization of three fibrinogenolytic enzymes from Chinese green tree viper (Trimeresurus stejnegeri) venom. Toxicon 1998; 36(3):457-67; PMID:9637365; https://doi.org/10.1016/S0041-0101(97)00150-5
- Islam MA, Alam F, Khalil MI, Sasongko TH, Gan SH. Natural Products Towards the Discovery of Potential Future Antithrombotic Drugs. Curr Pharm Des 2016; 22(20):2926-46; PMID:26951101
- Dahlback B. Blood coagulation. Lancet 2000; 355(9215):1627-32; PMID:10821379; https://doi.org/10.1016/S0140-6736(00)02225-X
- Smith SA, Travers RJ, Morrissey JH. How it all starts: Initiation of the clotting cascade. Crit Rev Biochem Mol Biol 2015; 50(4):326-36; PMID:26018600; https://doi.org/10.3109/10409238.2015.1050550
- Renne T, Schmaier AH, Nickel KF, Blomback M, Maas C. In vivo roles of factor XII. Blood 2012; 120(22):4296-303; PMID:22993391; https://doi.org/10.1182/blood-2012-07-292094
- Chapin JC, Hajjar KA. Fibrinolysis and the control of blood coagulation. Blood Rev 2015; 29(1):17-24; PMID:25294122; https://doi.org/10.1016/j.blre.2014.09.003
- Castellino FJ, Ploplis VA. Structure and function of the plasminogen/plasmin system. Thromb Haemost 2005; 93(4):647-54; PMID:15841308; https://doi.org/10.1160/TH04-12-0842
- Marder VJ, Novokhatny V. Direct fibrinolytic agents: biochemical attributes, preclinical foundation and clinical potential. J Thromb Haemost 2010; 8(3):433-44; PMID:19943877; https://doi.org/10.1111/j.1538-7836.2009.03701.x
- Kunamneni A, Abdelghani TT, Ellaiah P. Streptokinase–the drug of choice for thrombolytic therapy. J Thromb Thrombolysis 2007; 23(1):9-23; PMID:17111203; https://doi.org/10.1007/s11239-006-9011-x
- Irigoyen JP, Munoz-Canoves P, Montero L, Koziczak M, Nagamine Y. The plasminogen activator system: biology and regulation. Cell Mol Life Sci 1999; 56(1-2):104-32; PMID:11213252
- Tennent GA, Brennan SO, Stangou AJ, O'Grady J, Hawkins PN, Pepys MB. Human plasma fibrinogen is synthesized in the liver. Blood 2007; 109(5):1971-4; PMID:17082318; https://doi.org/10.1182/blood-2006-08-040956
- Kant JA, Fornace AJ Jr, Saxe D, Simon MI, McBride OW, Crabtree GR. Evolution and organization of the fibrinogen locus on chromosome 4: gene duplication accompanied by transposition and inversion. Proc Natl Acad Sci U S A 1985; 82(8):2344-8; PMID:2986113
- Mosesson MW, Siebenlist KR, Meh DA. The structure and biological features of fibrinogen and fibrin. Ann N Y Acad Sci 2001;936:11-30; PMID:11460466; https://doi.org/10.1111/j.1749-6632.2001.tb03491.x
- Gaffney PJ. Structure of fibrinogen and degradation products of fibrinogen and fibrin. Br Med Bull 1977; 33(3):245-51; PMID:143980
- Flemmig M, Melzig MF. Serine-proteases as plasminogen activators in terms of fibrinolysis. J Pharm Pharmacol 2012; 64(8):1025-39; PMID:22775207; https://doi.org/10.1111/j.2042-7158.2012.01457.x
- Sikri N, Bardia A. A history of streptokinase use in acute myocardial infarction. Tex Heart Inst J 2007; 34(3):318-27; PMID:17948083
- Parrado J, Conejero-Lara F, Smith RA, Marshall JM, Ponting CP, Dobson CM. The domain organization of streptokinase: nuclear magnetic resonance, circular dichroism, and functional characterization of proteolytic fragments. Protein Sci 1996; 5(4):693-704; PMID:8845759; https://doi.org/10.1002/pro.5560050414
- Yadav S, Sahni G. Probing the primary structural determinants of streptokinase inter-domain linkers by site-specific substitution and deletion mutagenesis. Biochim Biophys Acta 2010; 1804(9):1730-7; PMID:20417732; https://doi.org/10.1016/j.bbapap.2010.04.003
- Teuten AJ, Broadhurst RW, Smith RA, Dobson CM. Characterization of structural and folding properties of streptokinase by n.m.r. spectroscopy. Biochem J 1993; 290 Pt 2):313-9; PMID:8452517; https://doi.org/10.1042/bj2900313
- Welfle K, Pfeil W, Misselwitz R, Welfle H, Gerlach D. Conformational properties of streptokinase–differential scanning calorimetric investigations. Int J Biol Macromol 1992; 14(1):19-22; PMID:1596467
- Boxrud PD, Bock PE. Streptokinase binds preferentially to the extended conformation of plasminogen through lysine binding site and catalytic domain interactions. Biochemistry 2000; 39(45):13974-81; PMID:11076540; https://doi.org/10.1021/bi000594i
- Nihalani D, Sahni G. Streptokinase contains two independent plasminogen-binding sites. Biochem Biophys Res Commun 1995; 217(3):1245-54; PMID:8554583; https://doi.org/10.1006/bbrc.1995.2902
- Joshi KK, Nanda JS, Kumar P, Sahni G. Substrate kringle-mediated catalysis by the streptokinase-plasmin activator complex: critical contribution of kringle-4 revealed by the mutagenesis approaches. Biochim Biophys Acta 2012; 1824(2):326-33; PMID:22056293; https://doi.org/10.1016/j.bbapap.2011.10.010
- Aneja R, Datt M, Yadav S, Sahni G. Multiple exosites distributed across the three domains of streptokinase co-operate to generate high catalytic rates in the streptokinase-plasmin activator complex. Biochemistry 2013; 52(49):8957-68; PMID:23919427; https://doi.org/10.1021/bi400142s
- Shi GY, Chang BI, Su SW, Young KC, Wu DH, Chang LC, Tsai YS, Wu HL. Preparation of a novel streptokinase mutant with improved stability. Thromb Haemost 1998; 79(5):992-7; PMID:9609235
- Wu XC, Ye R, Duan Y, Wong SL. Engineering of plasmin-resistant forms of streptokinase and their production in Bacillus subtilis: streptokinase with longer functional half-life. Appl Environ Microbiol 1998; 64(3):824-9; PMID:9501422
- Pratap J, Rajamohan G, Dikshit KL. Characteristics of glycosylated streptokinase secreted from Pichia pastoris: enhanced resistance of SK to proteolysis by glycosylation. Appl Microbiol Biotechnol 2000; 53(4):469-75; PMID:10803905; https://doi.org/10.1007/s002530051643
- Sawhney P, Katare K, Sahni G. PEGylation of Truncated Streptokinase Leads to Formulation of a Useful Drug with Ameliorated Attributes. PLoS One 2016; 11(5):e0155831; PMID:27192220; https://doi.org/10.1371/journal.pone.0155831
- Torrens I, Ojalvo AG, Seralena A, Hayes O, de la Fuente J. A mutant streptokinase lacking the C-terminal 42 amino acids is less immunogenic. Immunol Lett 1999; 70(3):213-8; PMID:10656677; https://doi.org/10.1016/S0165-2478(99)00151-0
- Ramalingam S, Gautam P, Mukherjee KJ, Jayaraman G. Effects of post-induction feed strategies on secretory production of recombinant streptokinase in Escherichia coli. Biochem Eng J 2007; 33(1):34-41; https://doi.org/10.1016/j.bej.2006.09.019
- Adivitiya, Dagar VK, Devi N, Khasa YP. High level production of active streptokinase in Pichia pastoris fed-batch culture. Int J Biol Macromol 2016;83:50-60; PMID:26631635; https://doi.org/10.1016/j.ijbiomac.2015.11.062
- Rovelli F, De Vita C, Feruglio GA, Lotto A, Selvini A, Tognoni G. GISSI trial: early results and late follow-up. Gruppo Italiano per la Sperimentazione della Streptochinasi nell'Infarto Miocardico. J Am Coll Cardiol 1987; 10(5 Suppl B):33B-39B; PMID:3312371; https://doi.org/10.1016/S0735-1097(87)80426-6
- Roychoudhury PK, Khaparde SS, Mattiasson B, Kumar A. Synthesis, regulation and production of urokinase using mammalian cell culture: a comprehensive review. Biotechnol Adv 2006; 24(5):514-28; PMID:16822639; https://doi.org/10.1016/j.biotechadv.2006.05.002
- Bansal V, Roychoudhury PK. Production and purification of urokinase: a comprehensive review. Protein Expr Purif 2006; 45(1):1-14; PMID:16055345; https://doi.org/10.1016/j.pep.2005.06.009
- Collen D, Lijnen HR. Basic and clinical aspects of fibrinolysis and thrombolysis. Blood 1991; 78(12):3114-24; PMID:1742478
- Nelles L, Lijnen HR, Collen D, Holmes WE. Characterization of recombinant human single chain urokinase-type plasminogen activator mutants produced by site-specific mutagenesis of lysine 158. J Biol Chem 1987; 262(12):5682-9; PMID:3106341
- Nelles L, Lijnen HR, Collen D, Holmes WE. Characterization of a fusion protein consisting of amino acids 1 to 263 of tissue-type plasminogen activator and amino acids 144 to 411 of urokinase-type plasminogen activator. J Biol Chem 1987; 262(22):10855-62; PMID:2956260
- Hiramatsu R, Horinouchi S, Beppu T. Isolation and characterization of human pro-urokinase and its mutants accumulated within the yeast secretory pathway. Gene 1991; 99(2):235-41; PMID:1902432
- Wang P, Zhang J, Sun Z, Chen Y, Liu JN. Glycosylation of prourokinase produced by Pichia pastoris impairs enzymatic activity but not secretion. Protein Expr Purif 2000; 20(2):179-85; PMID:11049742; https://doi.org/10.1006/prep.2000.1310
- Booyse FM, Lin PH, Traylor M, Bruce R. Purification and properties of a single-chain urokinase-type plasminogen activator form produced by subcultured human umbilical vein endothelial cells. J Biol Chem 1988; 263(29):15139-45; PMID:3170576
- Avgerinos GC, Drapeau D, Socolow JS, Mao JI, Hsiao K, Broeze RJ. Spin filter perfusion system for high density cell culture: production of recombinant urinary type plasminogen activator in CHO cells. Biotechnology (N Y) 1990; 8(1):54-8; PMID:1367426
- Urokinase pulmonary embolism trial. Phase 1 results: a cooperative study. JAMA 1970; 214(12):2163-72; PMID:5536580
- Bell WR, Simon TL, Stengle JM, Sherry S. The urokinase-streptokinase pulmonary embolism trial (phase II) results. Circulation 1974; 50(6):1070-1; PMID:4430106; https://doi.org/10.1161/01.CIR.50.6.1070
- Sherry S. Pharmacology of anistreplase. Clin Cardiol 1990; (Suppl 5):V3-10; PMID:2182238
- Munger MA, Forrence EA. Anistreplase: a new thrombolytic for the treatment of acute myocardial infarction. Clin Pharm 1990; 9(7):530-40; PMID:2198125
- Rawles JM. Quantification of the benefit of earlier thrombolytic therapy: five-year results of the Grampian Region Early Anistreplase Trial (GREAT). J Am Coll Cardiol 1997; 30(5):1181-6; PMID:9350912; https://doi.org/10.1016/S0735-1097(97)00299-4
- ISIS-3 (Third International Study of Infarct Survival) Collaborative Group. ISIS-3: a randomised comparison of streptokinase vs tissue plasminogen activator vs anistreplase and of aspirin plus heparin vs aspirin alone among 41,299 cases of suspected acute myocardial infarction. Lancet 1992; 339(8796):753-70; PMID:1347801
- Moser M, Bode C. Pharmacology and clinical trial results of saruplase (scuPA) in acute myocardial infarction. Expert Opin Investig Drugs 1999; 8(3):329-35; PMID:15992082; https://doi.org/10.1517/13543784.8.3.329
- del Zoppo GJ, Higashida RT, Furlan AJ, Pessin MS, Rowley HA, Gent M. PROACT: a phase II randomized trial of recombinant pro-urokinase by direct arterial delivery in acute middle cerebral artery stroke. PROACT Investigators. Prolyse in Acute Cerebral Thromboembolism. Stroke 1998; 29(1):4-11; PMID:9445320; https://doi.org/10.1161/01.STR.29.1.4
- Furlan A, Higashida R, Wechsler L, Gent M, Rowley H, Kase C, Pessin M, Ahuja A, Callahan F, Clark WM, et al. Intra-arterial prourokinase for acute ischemic stroke. The PROACT II study: a randomized controlled trial. Prolyse in Acute Cerebral Thromboembolism. JAMA 1999; 282(21):2003-11; PMID:10591382; https://doi.org/10.1001/jama.282.21.2003
- Tebbe U, Michels R, Adgey J, Boland J, Caspi A, Charbonnier B, Windeler J, Barth H, Groves R, Hopkins GR, et al. Randomized, double-blind study comparing saruplase with streptokinase therapy in acute myocardial infarction: the COMPASS Equivalence Trial. Comparison Trial of Saruplase and Streptokinase (COMASS) Investigators. J Am Coll Cardiol 1998; 31(3):487-93; PMID:9502624; https://doi.org/10.1016/S0735-1097(97)00553-6
- Vermeer F, Bosl I, Meyer J, Bar F, Charbonnier B, Windeler J, Barth H. Saruplase is a safe and effective thrombolytic agent; observations in 1,698 patients: results of the PASS study. Practical Applications of Saruplase Study. J Thromb Thrombolysis 1999; 8(2):143-50; PMID:10436145
- Bar FW, Meyer J, Vermeer F, Michels R, Charbonnier B, Haerten K, Spiecker M, Macaya C, Hanssen M, Heras M, et al. Comparison of saruplase and alteplase in acute myocardial infarction. SESAM Study Group. The Study in Europe with Saruplase and Alteplase in Myocardial Infarction. Am J Cardiol 1997; 79(6):727-32; PMID:9070549; https://doi.org/10.1016/S0002-9149(97)89274-0
- Tebbe U, Windeler J, Boesl I, Hoffmann H, Wojcik J, Ashmawy M, Rüdiger Schwarz E, von Loewis P, Rosemeyer P, Hopkins G, et al. Thrombolysis with recombinant unglycosylated single-chain urokinase-type plasminogen activator (saruplase) in acute myocardial infarction: influence of heparin on early patency rate (LIMITS study). Liquemin in Myocardial Infarction During Thrombolysis With Saruplase. J Am Coll Cardiol 1995; 26(2):365-73; PMID:7608436
- Spiecker M, Windeler J, Vermeer F, Michels R, Seabra-Gomes R, vom Dahl J, Kerber S, Verheugt FW, Westerhof PW, Bar FW, et al. Thrombolysis with saruplase versus streptokinase in acute myocardial infarction: five-year results of the PRIMI trial. Am Heart J 1999; 138(3 Pt 1):518-24; PMID:10467203
- Collen D, Lijnen HR. The tissue-type plasminogen activator story. Arterioscler Thromb Vasc Biol 2009; 29(8):1151-5; https://doi.org/10.1161/ATVBAHA.108.179655
- Rijken DC, Wijngaards G, Zaal-de Jong M, Welbergen J. Purification and partial characterization of plasminogen activator from human uterine tissue. Biochim Biophys Acta 1979; 580(1):140-53; PMID:121055
- Rijken DC, Collen D. Purification and characterization of the plasminogen activator secreted by human melanoma cells in culture. J Biol Chem 1981; 256(13):7035-41; PMID:6787058
- Pennica D, Holmes WE, Kohr WJ, Harkins RN, Vehar GA, Ward CA, Bennett WF, Yelverton E, Seeburg PH, Heyneker HL, et al. Cloning and expression of human tissue-type plasminogen activator cDNA in E. coli. Nature 1983; 301(5897):214-21; PMID:6337343
- Collen D, Stassen JM, Marafino BJ Jr, Builder S, De Cock F, Ogez J, Tajiri D, Pennica D, Bennett WF, Salwa J, et al. Biological properties of human tissue-type plasminogen activator obtained by expression of recombinant DNA in mammalian cells. J Pharmacol Exp Ther 1984; 231(1):146-52; PMID:6541693
- Majidzadeh-A K, Khalaj V, Fatemeh D, Mahdi H, Farzaneh B, Ahmad A, Mahboudi F. Cloning and expression of functional full-length human tissue plasminogen activator in Pichia pastoris. Appl Biochem Biotechnol 2010; 162(7):2037-48; PMID:20455033; https://doi.org/10.1007/s12010-010-8979-z
- Collen D, Lijnen HR. Tissue-type plasminogen activator: a historical perspective and personal account. J Thromb Haemost 2004; 2(4):541-6; PMID:15102005; https://doi.org/10.1111/j.1538-7933.2004.00645.x
- Larsen GR, Metzger M, Henson K, Blue Y, Horgan P. Pharmacokinetic and distribution analysis of variant forms of tissue-type plasminogen activator with prolonged clearance in rat. Blood 1989; 73(7):1842-50; PMID:2496774
- Lijnen HR, Collen D. Strategies for the improvement of thrombolytic agents. Thromb Haemost 1991; 66(1):88-110; PMID:1926054
- Wahlgren N, Ahmed N, Dávalos A, Ford GA, Grond M, Hacke W, Hennerici MG, Kaste M, Kuelkens S, Larrue V, et al. Thrombolysis with alteplase for acute ischaemic stroke in the Safe Implementation of Thrombolysis in Stroke-Monitoring Study (SITS-MOST): an observational study. Lancet 2007; 369(9558):275-82; PMID:17258667; https://doi.org/10.1016/S0140-6736(07)60149-4
- Davis SM, Donnan GA, Parsons MW, Levi C, Butcher KS, Peeters A, Barber PA, Bladin C, De Silva DA, Byrnes G, et al. Effects of alteplase beyond 3 h after stroke in the Echoplanar Imaging Thrombolytic Evaluation Trial (EPITHET): a placebo-controlled randomised trial. Lancet Neurol 2008; 7(4):299-309; PMID:18296121; https://doi.org/10.1016/S1474-4422(08)70044-9
- GISSI-2: a factorial randomised trial of alteplase versus streptokinase and heparin versus no heparin among 12,490 patients with acute myocardial infarction. Gruppo Italiano per lo Studio della Sopravvivenza nell'Infarto Miocardico. Lancet 1990; 336(8707):65-71; PMID:1975321
- Neuhaus KL, Feuerer W, Jeep-Tebbe S, Niederer W, Vogt A, Tebbe U. Improved thrombolysis with a modified dose regimen of recombinant tissue-type plasminogen activator. J Am Coll Cardiol 1989; 14(6):1566-9; PMID:2509531; https://doi.org/10.1016/0735-1097(89)90399-9
- Neuhaus KL, von Essen R, Tebbe U, Vogt A, Roth M, Riess M, Niederer W, Forycki F, Wirtzfeld A, Maeurer W, et al. Improved thrombolysis in acute myocardial infarction with front-loaded administration of alteplase: results of the rt-PA-APSAC patency study (TAPS). J Am Coll Cardiol 1992; 19(5):885-91; PMID:1552106
- The GUSTO investigators. An international randomized trial comparing four thrombolytic strategies for acute myocardial infarction. N Engl J Med 1993; 329(10):673-82; PMID:8204123; https://doi.org/10.1056/NEJM199309023291001
- The GUSTO Angiographic Investigators. The effects of tissue plasminogen activator, streptokinase, or both on coronary-artery patency, ventricular function, and survival after acute myocardial infarction. N Engl J Med 1993; 329(22):16 15-22; PMID:8232430; https://doi.org/10.1056/NEJM199311253292204
- The Continuous Infusion versus Double-Bolus Administration of Alteplase (COBALT) Investigators. A comparison of continuous infusion of alteplase with double-bolus administration for acute myocardial infarction. N Engl J Med 1997; 337(16):1124-30; PMID:9340504; https://doi.org/10.1056/NEJM199710163371604
- Kohnert U, Rudolph R, Verheijen JH, Weening-Verhoeff EJ, Stern A, Opitz U, Martin U, Lill H, Prinz H, Lechner M, et al. Biochemical properties of the kringle 2 and protease domains are maintained in the refolded t-PA deletion variant BM 06.022. Protein Eng 1992; 5(1):93-100; PMID:1321420; https://doi.org/10.1093/protein/5.1.93
- Sturzebecher J, Neumann U, Kohnert U, Kresse GB, Fischer S. Mapping of the catalytic site of CHO-t-PA and the t-PA variant BM 06.022 by synthetic inhibitors and substrates. Protein Sci 1992; 1(8):1007-1013; PMID:1304379; https://doi.org/10.1002/pro.5560010806
- Martin U, von Mollendorff E, Akpan W, Kientsch-Engel R, Kaufmann B, Neugebauer G. Pharmacokinetic and hemostatic properties of the recombinant plasminogen activator bm 06.022 in healthy volunteers. Thromb Haemost 1991; 66(5):569-574; PMID:1725068
- Martin U, von Mollendorff E, Akpan W, Kientsch-Engel R, Kaufmann B, Neugenauer G. Dose-ranging study of the novel recombinant plasminogen activator BM 06.022 in healthy volunteers. Clin Pharmacol Ther 1991; 50(4):429-436; PMID:1914379; https://doi.org/10.1038/clpt.1991.160
- Smalling RW, Bode C, Kalbfleisch J, Sen S, Limbourg P, Forycki F, Habib G, Feldman R, Hohnloser S, Seals A. More rapid, complete, and stable coronary thrombolysis with bolus administration of reteplase compared with alteplase infusion in acute myocardial infarction. RAPID Investigators. Circulation 1995; 91(11):2725-2732; PMID:7758177; https://doi.org/10.1161/01.CIR.91.11.2725
- Bode C, Smalling RW, Berg G, Burnett C, Lorch G, Kalbfleisch JM, Chernoff R, Christie LG, Feldman RL, Seals AA, et al. Randomized comparison of coronary thrombolysis achieved with double-bolus reteplase (recombinant plasminogen activator) and front-loaded, accelerated alteplase (recombinant tissue plasminogen activator) in patients with acute myocardial infarction. The RAPID II Investigators. Circulation 1996; 94(5):891-898; PMID:8790022; https://doi.org/10.1161/01.CIR.94.5.891
- International Joint Efficacy Comparison of Thrombolytics. Randomised, double-blind comparison of reteplase double-bolus administration with streptokinase in acute myocardial infarction (INJECT): trial to investigate equivalence. Lancet 1995; 346(8971):329-336; PMID:7623530; https://doi.org/10.1016/S0140-6736(95)92224-5
- The Global Use of Strategies to Open Occluded Coronary Arteries (GUSTO III) Investigators. A comparison of reteplase with alteplase for acute myocardial infarction. N Engl J Med 1997; 337(16):1118-1123; PMID:9340503; https://doi.org/10.1056/NEJM199710163371603
- Keyt BA, Paoni NF, Refino CJ, Nerleau L, Nguyen H, Chow A, Lai J, Pena L, Pater C, Ogez J, et al. A faster-acting and more potent form of tissue plasminogen activator. Proc Natl Acad Sci USA 1994; 91(9):3670-3674; PMID:8170967
- Cannon CP, McCabe GH, Gibson CM, Ghali M, Sequeira RF, McKendall GR, Breed J, Modi NB, Fox NL, Tracy RP, et al. TNK-tissue plasminogen activator in acute myocardial infarction. Results of the Thrombolysis in Myocardial Infarction (TIMI) 10A dose-ranging trial. Circulation 1997; 95(2):351-356; PMID:9008448; https://doi.org/10.1161/01.CIR.95.2.351
- Wallentin L, Goldstein P, Armstrong PW, Granger CB, Adgey AA, Arntz HR, Bogaerts K, Danays T, Lindahl B, Makijarvi M, et al. Efficacy and safety of tenecteplase in combination with the low-molecular-weight heparin enoxaparin or unfractionated heparin in the prehospital setting: the Assessment of the Safety and Efficacy of a New Thrombolytic Regimen (ASSENT)-3 PLUS randomized trial in acute myocardial infarction. Circulation 2003; 108(2):135-142; PMID:12847070; https://doi.org/10.1161/01.CIR.0000081659.72985.A8
- Zeymer U, Neuhaus KL. Clinical trials in acute myocardial infarction. Curr Opin Cardiol 1999; 14(5):392-402; PMID:10500901
- Cannon CP, Gibson CM, McCabe CH, Adgey AA, Schweiger MJ, Sequeira RF, Grollier G, Giugliano RP, Frey M, Mueller HS, et al. TNK-tissue plasminogen activator compared with front-loaded alteplase in acute myocardial infarction: results of the TIMI 10B trial. Thrombolysis in Myocardial Infarction (TIMI) 10B Investigators. Circulation 1998; 98(25):2805-2814; PMID:9860780; https://doi.org/10.1161/01.CIR.98.25.2805
- Van de Werf F, Cannon CP, Luyten A, Houbracken K, McCabe CH, Berioli S, Bluhmki E, Sarelin H, Wang-Clow F, Fox NL, et al. Safety assessment of single-bolus administration of TNK tissue-plasminogen activator in acute myocardial infarction: the ASSENT-1 trial. The ASSENT-1 Investigators. Am Heart J 1999; 137(5):786-791; PMID:10220625
- Van De Werf F, Adgey J, Ardissino D, Armstrong PW, Aylward P, Barbash G, Betriu A, Binbrek AS, Califf R, Diaz R, et al. Single-bolus tenecteplase compared with front-loaded alteplase in acute myocardial infarction: the ASSENT-2 double-blind randomised trial. Assessment of the Safety and Efficacy of a New Thrombolytic (ASSENT-2) Investigators. Lancet 1999; 354(9180):716-22; PMID:10475182; https://doi.org/10.1016/S0140-6736(99)07403-6
- Malcolm AD, Keltai M, Walsh MJ. ESPRIT: a European study of the prevention of reocclusion after initial thrombolysis with duteplase in acute myocardial infarction. Eur Heart J 1996; 17(10):1522-31; PMID:8909909; https://doi.org/1522-1531
- Suzuki S, Saito M, Suzuki N, Kato H, Nagaoka N, Yoshitake S, Mizuo H, Yuzuriha T, Yui Y, Kawai C. Thrombolytic properties of a novel modified human tissue-type plasminogen activator (E6010): a bolus injection of E6010 has equivalent potency of lysing young and aged canine coronary thrombi. J Cardiovasc Pharmacol 1991; 17(5):738-46; PMID:1713988
- Kawai C, Yui Y, Hosoda S, Nobuyoshi M, Suzuki S, Sato H, Takatsu F, Motomiya T, Kanmatsuse K, Kodama K, et al. A prospective, randomized, double-blind multicenter trial of a single bolus injection of the novel modified t-PA E6010 in the treatment of acute myocardial infarction: comparison with native t-PA. E6010 Study Group. J Am Coll Cardiol 1997; 29(7):1447-53; PMID:9180103; https://doi.org/10.1016/S0735-1097(97)00074-0
- Tagami H, Utoh J, Sun LB, Okamoto K, Moriyama S, Kunitomo R, Kitamura N. Effects of recombinant tissue-type plasminogen activator on life-threatening acute pulmonary thromboembolism in a canine model: a comparative study of e6010 and alteplase. Ann Thorac Cardiovasc Surg 2000; 6(5):299-303; PMID:11173335
- Inoue T, Nishiki R, Kageyama M, Node K. Therapeutic potential of monteplase in acute myocardial infarction as a powerful thrombolytic agent for pretreatment of coronary intervention. Cardiovasc Drug Rev 2004; 22(4):320-33; Review. PMID:15592577; https://doi.org/10.1111/j.1527-3466.2004.tb00149.x
- Widimsky P, Groch L, Zelizko M, Aschermann M, Bednar F, Suryapranata H. Multicentre randomized trial comparing transport to primary angioplasty vs immediate thrombolysis vs combined strategy for patients with acute myocardial infarction presenting to a community hospital without a catheterization laboratory. The PRAGUE study. Eur Heart J 2000; 21(10):823-31; PMID:10781354; https://doi.org/10.1053/euhj.1999.1993
- Inoue T, Yaguchi I, Takayanagi K, Hayashi T, Morooka S, Eguchi Y. A new thrombolytic agent, monteplase, is independent of the plasminogen activator inhibitor in patients with acute myocardial infarction: initial results of the Combining Monteplase with Angioplasty (COMA) trial. Am Heart J. 2002; 144(4):E5; PMID:12360174; https://doi.org/10.1067/mhj.2002.124864
- Muramatsu H, Igarashi H, Okubo S, Katayama Y. Monteplase reduces infarct volume and hemorrhagic transformation in rat model of embolic stroke. Neurol Res 2002; 24(3):311-6; PMID:11958428; https://doi.org/10.1179/016164102101199800
- Yamagami T, Yoshimatsu R, Tanaka O, Miura H, Nishimura T. Endovascular thrombolysis using monteplase for non-chronic deep venous thrombosis. Cardiovasc Intervent Radiol 2010; 33(6):1223-9; PMID:20411388; https://doi.org/10.1007/s00270-010-9856-4
- den Heijer P, Vermeer F, Ambrosioni E, Sadowski Z, Lopez-Sendon JL, von Essen R, Beaufils P, Thadani U, Adgey J, Pierard L, et al. Evaluation of a weight-adjusted single-bolus plasminogen activator in patients with myocardial infarction: a double-blind, randomized angiographic trial of lanoteplase versus alteplase. Circuation 1998; 98(20):2117-25; PMID:9815865; https://doi.org/10.1161/01.CIR.98.20.2117
- InTIME-II Investigators. Intravenous NPA for the treatment of infarcting myocardium early; InTIME-II, a double-blind comparison of single-bolus lanoteplase vs accelerated alteplase for the treatment of patients with acute myocardial infarction. Eur Heart J 2000; 21(24):2005-13; PMID:11102251; https://doi.org/10.1053/euhj.2000.2498
- Ishikawa A, Ohata T, Imamura K, Iwasaki M, Sakai T, Matsuzawa T, Okazaki S. Single and repeated intravenous toxicity studies of pamiteplase (genetical recombination) in rats and monkeys. J Toxicol Sci 1997; 22(2):117-33; PMID:9198009
- Kawasaki T, Katoh M, Kaku S, Gushima H, Takenaka T, Yui Y, Kawai C. Thrombolytic activity of a novel modified tissue-type plasminogen activator, YM866, in a canine model of coronary artery thrombosis. Jpn J Pharmacol 1993; 63(1):9-16; PMID:8271535; https://doi.org/10.1254/jjp.63.9
- Agnelli G, Pascucci C, Nenci GG, Mele A, Burgi R, Heim J. Thrombolytic and haemorrhagic effects of bolus doses of tissue-type plasminogen activator and a hybrid plasminogen activator with prolonged plasma half-life (K2tu-PA: CGP 42935). Thromb Haemost 1993; 70(2):294-300; PMID:8236138
- Muller D, Domon B, Karas M, van Oostrum J, Richter WJ. Characterization and direct glycoform profiling of a hybrid plasminogen activator by matrix-assisted laser desorption and electrospray mass spectrometry: correlation with high-performance liquid chromatographic and nuclear magnetic resonance analyses of the released glycans. Biol Mass Spectrom 1994; 23(6):330-8; PMID:8038226; https://doi.org/10.1002/bms.1200230606
- Rijken DC, Barrett-Bergshoeff MM, Jie AF, Criscuoli M, Sakharov DV. Clot penetration and fibrin binding of amediplase,a chimeric plasminogen activator (K2 tu-PA). Thromb Haemost 2004; 91(1):52-60; PMID:14691568; https://doi.org/10.1160/TH03-07-0435
- Guimaraes AH, Barrett-Bergshoeff MM, Criscuoli M, Evangelista S, Rijken DC. Fibrinolytic efficacy of Amediplase, Tenecteplase and scu-PA in different external plasma clot lysis models: sensitivity to the inhibitory action of thrombin activatable fibrinolysis inhibitor (TAFI). Thromb Haemost 2006; 96(3):325-30; PMID:16953274; https://doi.org/10.1160/TH06-04-0197
- Bringmann P, Gruber D, Liese A, Toschi L, Kratzchmar J, Schleuning WD, Donner P. Structural features mediating fibrin selectivity of vampire bat plasminogen activators. J Biol Chem 1995; 270(43):25596-603; PMID:7592732; https://doi.org/10.1074/jbc.270.43.25596
- Niego B, Horvath A, Coughlin PB, Pugsley MK, Medcalf RL. Desmoteplase-mediated plasminogen activation and clot lysis are inhibited by the lysine analogue tranexamic acid. Blood Coagul Fibrinolysis 2008; 19(4):322-4; PMID:18469556; https://doi.org/10.1097/MBC.0b013e3282f54568
- Mellott MJ, Stabilito II, Holahan MA, Cuca GC, Wang S, Li P, Barrett JS, Lynch JJ, Gardell SJ. Vampire bat salivary plasminogen activator promotes rapid and sustained reperfusion without concomitant systemic plasminogen activation in a canine model of arterial thrombosis. Arterioscler Thromb 1992; 12(2):212-21; PMID:1371932; https://doi.org/10.1161/01.ATV.12.2.212
- Reddrop C, Moldrich RX, Beart PM, Farso M, Liberatore GT, Howells DW, Petersen KU, Schleuning WD, Medcalf RL. Vampire bat salivary plasminogen activator (desmoteplase) inhibits tissue-type plasminogen activator-induced potentiation of excitotoxic injury. Stroke 2005; 36(6):1241-6; PMID:15879331; https://doi.org/10.1161/01.STR.0000166050.84056.48
- Hacke W, Albers G, Al-Rawi Y, Bogousslavsky J, Davalos A, Eliasziw M, Fischer M, Furlan A, Kaste M, Lees KR, et al. The Desmoteplase in Acute Ischemic Stroke Trial (DIAS): a phase II MRI-based 9-hour window acute strokethrombolysis trial with intravenous desmoteplase. Stroke. 2005; 36(1):66-73; PMID:15569863; https://doi.org/10.1161/01.STR.0000149938.08731.2c
- Hacke W, Furlan AJ, Al-Rawi Y, Davalos A, Fiebach JB, Gruber F, Kaste M, Lipka LJ, Pedraza S, Ringleb PA, et al. Intravenous desmoteplase in patients with acute ischaemic stroke selected by MRI perfusion-diffusion weighted imaging or perfusion CT (DIAS-2): a prospective, randomised, double-blind, placebo-controlled study. Lancet Neurol 2009; 8(2):141-50; PMID:19097942; https://doi.org/10.1016/S1474-4422(08)70267-9
- Albers GW, von Kummer R, Truelsen T, Jensen JK, Ravn GM, Grønning BA, Chabriat H, Chang KC, Davalos AE, Ford GA, et al. Safety and efficacy of desmoteplase given 3–9 h after ischaemic stroke in patients with occlusion or high-grade stenosis in major cerebral arteries (DIAS-3): a double-blind, randomised, placebo-controlled phase 3 trial. Lancet Neurol 2015; 14(6):575-84; PMID:25937443; https://doi.org/10.1016/S1474-4422(15)00047-2
- Lijnen HR, Van Hoef B, De Cock F, Okada K, Ueshima S, Matsuo O, Collen D. On the mechanism of fibrin-specific plasminogen activation by staphylokinase. J Biol Chem 1991; 266(18):11826-32; PMID:2050679
- Sakharov DV, Lijnen HR, Rijken DC. Interactions between staphylokinase, plasmin(ogen), and fibrin. Staphylokinase discriminates between freeplasminogen and plasminogen bound to partially degraded fibrin. J Biol Chem 1996; 271(44):27912-8; PMID:8910391; https://doi.org/10.1074/jbc.271.44.27912
- Schlott B, Guhrs KH, Hartmann M, Röcker A, Collen D. NH2-terminal structural motifs in staphylokinase required for plasminogen activation. J Biol Chem 1998; 273(35):22346-50; PMID:9712854; https://doi.org/10.1074/jbc.273.35.22346
- Schlott B, Hartmann M, Gührs KH, Birch-Hirschfeld E, Gase A, Vettermann S, Collen D, Lijnen HR. Functional properties of recombinant staphylokinase variants obtained by site-specific mutagenesis ofmethionine-26. Biochim Biophys Acta 1994; 1204(2):235-42; PMID:8142464
- Jespers L, Vanwetswinkel S, Lijnen HR, Van Herzeele N, Van Hoef B, Demarsin E, Collen D, De Maeyer M. Structural and functional basis of plasminogen activation by staphylokinase. Thromb Haemost 1999; 81(4):479-85; PMID:10235424
- Silence K, Hartmann M, Gührs KH, Gase A, Schlott B, Collen D, Lijnen HR. Structure-function relationships in staphylokinase as revealed by “clustered charge to alanine” mutagenesis. J Biol Chem 1995; 270(45):27192-8; PMID:7592976; https://doi.org/10.1074/jbc.270.45.27192
- Szemraj J, Walkowiak B, Kawecka I, Janiszewska G, Buczko W, Bartkowiak J, Chabielska E. A new recombinant thrombolytic and antithrombotic agent with higher fibrin affinity–a staphylokinase variant. I. In vitro study. J Thromb Haemost 2005; 3(10):2156-65; PMID:16150047; https://doi.org/10.1111/j.1538-7836.2005.01480.x
- Kumar A, Pulicherla KK, Mayuren C, Kotra S, Rao KR. Evaluation of a multifunctional staphylokinase variant with thrombin inhibition and antiplatelet aggregation activities produced from salt-inducible E. coli GJ1158. Can J Physiol Pharmacol 2013; 91(10):839-47; PMID:24144055; https://doi.org/10.1139/cjpp-2012-0467
- Vanderschueren S, Stockx L, Wilms G, Lacroix H, Verhaeghe R, Vermylen J, Collen D. Thrombolytic therapy of peripheral arterial occlusion with recombinant staphylokinase. Circulation 1995; 92(8):2050-7; PMID:7554181; https://doi.org/10.1161/01.CIR.92.8.2050
- Vanderschueren S, Barrios L, Kerdsinchai P, Van den Heuvel P, Hermans L, Vrolix M, De Man F, Benit E, Muyldermans L, Collen D, et al. A randomized trial of recombinant staphylokinase versus alteplase for coronary artery patency in acute myocardial infarction. The STAR Trial Group. Circulation 1995; 92(8):2044-9; PMID:7554180; https://doi.org/10.1161/01.CIR.92.8.2044
- Vanderschueren S, Collen D, van de Werf F. A pilot study on bolus administration of recombinant staphylokinase for coronary artery thrombolysis. Thromb Haemost 1996; 76(4):541-4; PMID:8902993
- Vanderschueren S, Dens J, Kerdsinchai P, Desmet W, Vrolix M, De Man F, Van den Heuvel P, Hermans L, Collen D, Van de Werf F. Randomized coronary patency trial of double-bolus recombinant staphylokinase versus front-loaded alteplase in acute myocardial infarction. Am Heart J 1997; 134(2 Pt 1):213-9; PMID:9313600; https://doi.org/10.1016/S0002-8703(97)70127-3
- Armstrong PW, Burton JR, Palisaitis D, Thompson CR, Van de Werf F, Rose B, Collen D, Teo KK. Collaborative angiographic patency trial of recombinant staphylokinase (CAPTORS). Am Heart J 2000; 139(5):820-3; PMID:10783215; https://doi.org/10.1016/S0002-8703(00)90013-9
- Armstrong PW, Burton J, Pakola S, Molhoek PG, Betriu A, Tendera M, Bode C, Adgey AA, Bar F, Vahanian A, et al. Collaborative Angiographic Patency Trial Of Recombinant Staphylokinase (CAPTORS II). Am Heart J 2003; 146(3):484-8; PMID:12947367; https://doi.org/10.1016/S0002-8703(03)00312-0
- Zhang Y, Wisner A, Xiong Y, Bon C. A novel plasminogen activator from snake venom. Purification, characterization, and molecular cloning. J Biol Chem 1995; 270(17):10246-55; PMID:7730329; https://doi.org/10.1074/jbc.270.17.10246
- Park D, Kim H, Chung K, Kim DS, Yun Y. Expression and characterization of a novel plasminogen activator from Agkistrodon halys venom. Toxicon 1998; 36(12):1807-19; PMID:9839665; https://doi.org/10.1016/S0041-0101(98)00090-7
- Sanchez EF, Santos CI, Magalhaes A, Diniz CR, Figueiredo S, Gilroy J, Richardson M. Isolation of a proteinase with plasminogen-activating activity from Lachesis muta muta (bushmaster) snake venom. Arch Biochem Biophys 2000; 378(1):131-41; PMID:10871053; https://doi.org/10.1006/abbi.2000.1781
- Jiao J, Yu M, Ru B. Characterization of a recombinant chimeric plasminogen activator with enhanced fibrin binding. Biochim Biophys Acta 2001; 1546(2):399-405; PMID:11295444; https://doi.org/10.1016/S0167-4838(01)00161-3
- Davami F, Sardari S, Majidzadeh-A K, Hemayatkar M, Barkhrdari F, Omidi M, Azami M, Adeli A, Davoudi N, Mahboudi F. Expression of a novel chimeric truncated t-PA in CHO cells based on in silico experiments. J Biomed Biotechnol 2010;2010:108159; PMID:20885932; https://doi.org/10.1155/2010/108159
- Marder VJ. Historical perspective and future direction of thrombolysis research: the re-discovery of plasmin. J Thromb Haemost 2011; 9(Suppl 1):364-73; https://doi.org/10.1111/j.1538-7836.2011.04370.x
- Hoefer IE, Stroes ES, Pasterkamp G, Levi MM, Reekers JA, Verhagen HJ, Meijers JC, Humphries JE, Rotmans JI. Locally applied recombinant plasmin results in effective thrombolysis in a porcine model of arteriovenous graft thrombosis. J Vasc Interv Radiol 2009; 20(7):951-8; PMID:19481472; https://doi.org/10.1016/j.jvir.2009.03.043
- Sadeghi S, Marder VJ, Stewart D, Kong M, Humphries J, Baumbach GA, Jesmok G. Safety of plasmin in the setting of concomitant aspirin and heparin administration in an animal model of bleeding. J Thromb Haemost 2003; 1(12):2621-5; PMID:14675099; https://doi.org/10.1046/j.1538-7836.2003.00441.x
- Jahan R, Stewart D, Vinters HV, Yong W, Vinuela F, Vandeberg P, Marder VJ. Middle cerebral artery occlusion in the rabbit using selective angiography: application for assessment of thrombolysis. Stroke 2008; 39(5):1613-5; PMID:18340097; https://doi.org/10.1161/STROKEAHA.107.507376
- Shlansky-Goldberg RD, Matsumoto AH, Baumbach GA, Siegel JB, Raabe RD, Murphy TP, Deng C, Ray Dawkins J, Marder VJ. A first-in human phase I trial of locally delivered human plasmin for hemodialysis graft occlusion. J Thromb Haemost 2008; 6(6):944-50; PMID:18384651; https://doi.org/10.1111/j.1538-7836.2008.02969.x
- Medynski D, Tuan M, Liu W, Wu S, Lin X. Refolding, purification, and activation of miniplasminogen and microplasminogen isolated from E. coli inclusion bodies. Protein Expr Purif 2007; 52(2):395-402; PMID:17126563; https://doi.org/10.1016/j.pep.2006.10.012
- Moroz LA. Mini-plasminogen: a mechanism for leukocyte modulation of plasminogen activation by urokinase. Blood 1981; 58(1):97-104; PMID:7016219
- Kolev K, Komorowicz E, Owen WG, Machovich R. Quantitative comparison of fibrin degradation with plasmin, miniplasmin, neurophil leukocyte elastase andcathepsin G. Thromb Haemost. 1996; 75(1):140-6; PMID:8713793
- Fu J, Ren J, Zou L, Bian G, Li R, Lu Q. The thrombolytic effect of miniplasmin in a canine model of femoral artery thrombosis. Thromb Res 2008; 122(5):683-90; PMID:18328540; https://doi.org/10.1016/j.thromres.2008.01.007
- Shi GY, Wu HL. Isolation and characterization of microplasminogen. A low molecular weight form of plasminogen. J Biol Chem 1988; 263(32):17071-5; PMID:2972717
- Ma Z, Lu W, Wu S, Chen J, Sun Z, Liu JN. Expression and characterization of recombinant human micro-plasminogen. Biotechnol Lett 2007; 29(4):517-23; PMID:17206368; https://doi.org/10.1007/s10529-006-9290-5
- Nagai N, Demarsin E, Van Hoef B, Wouters S, Cingolani D, Laroche Y, Collen D. Recombinant human microplasmin: production and potential therapeutic properties. J Thromb Haemost 2003; 1(2):307-13; PMID:12871505; https://doi.org/10.1046/j.1538-7836.2003.00078.x
- Wang J, Brdar B, Reich E. Structure and function of microplasminogen: I. Methionine shuffling, chemical proteolysis, and proenzymeactivation. Protein Sci 1995; 4(9):1758-67; PMID:8528074; https://doi.org/10.1002/pro.5560040911
- Suzuki Y, Nagai N, Collen D. Comparative effects of microplasmin and tissue-type plasminogen activator (tPA) on cerebral hemorrhage in a middle cerebral artery occlusion model in mice. J Thromb Haemost 2004; 2(9):1617-21; PMID:15333039; https://doi.org/10.1111/j.1538-7836.2004.00889.x
- Chen F, Suzuki Y, Nagai N, Sun X, Wang H, Yu J, Marchal G, Ni Y. Microplasmin and tissue plasminogen activator: comparison of therapeutic effects in rat stroke model at multiparametric MR imaging. Radiology 2007; 244(2):429-38; PMID:17581889; https://doi.org/10.1148/radiol.2442061316
- Thijs VN, Peeters A, Vosko M, Aichner F, Schellinger PD, Schneider D, Neumann-Haefelin T, Rother J, Davalos A, Wahlgren N, Verhamme P. Randomized, placebo-controlled, dose-ranging clinical trial of intravenous microplasmin in patients with acute ischemic stroke. Stroke 2009; 40(12):3789-95; https://doi.org/10.1161/STROKEAHA.109.560201
- Verhamme P, Jerome M, Goossens G, Devis J, Maleux G, Stas M. A pilot trial of microplasmin in patients with long-term venous access catheter thrombosis. J Thromb Thrombolysis 2009; 28(4):477-81; PMID:19225865; https://doi.org/10.1007/s11239-009-0310-x
- Chen W, Huang X, Ma XW, Mo W, Wang WJ, Song HY. Enzymatic vitreolysis with recombinant microplasminogen and tissue plasminogen activator. Eye (Lond) 2008; 22(2):300-7; PMID:17704761; https://doi.org/10.1038/sj.eye.6702931
- de Smet MD, Valmaggia C, Zarranz-Ventura J, Willekens B. Microplasmin: ex vivo characterization of its activity in porcine vitreous. Invest Ophthalmol Vis Sci 2009; 50(2):814-9; PMID:18806295; https://doi.org/10.1167/iovs.08-2185
- Hunt JA, Petteway SR Jr, Scuderi P, Novokhatny V. Simplified recombinant plasmin: production and functional comparison of a novel thrombolytic molecule withplasma-derived plasmin. Thromb Haemost 2008; 100(3):413-9; PMID:18766256; https://doi.org/10.1160/TH08-04-0225
- Deitcher SR, Funk WD, Buchanan J, Liu S, Levy MD, Toombs CF. Alfimeprase: a novel recombinant direct-acting fibrinolytic. Expert Opin Biol Ther 2006; 6(12):1361-9; PMID:17223743; https://doi.org/10.1517/14712598.6.12.1361
- Toombs CF. Alfimeprase: pharmacology of a novel fibrinolytic metalloproteinase for thrombolysis. Haemostasis 2001; 31(3–6):141-7; PMID:11910179; https://doi.org/48057
- Hong TT, Huang J, Lucchesi BR. Effect of thrombolysis on myocardial injury: recombinant tissue plasminogen activator vs. alfimeprase. Am J Physiol Heart Circ Physiol 2006; 290(3):H959-67; PMID:16243915; https://doi.org/10.1152/ajpheart.00649.2005
- Ouriel K, Cynamon J, Weaver FA, Dardik H, Akers D, Blebea J, Gruneiro L, Toombs CF, Wang-Clow F, Mohler M, et al. A phase I trial of alfimeprase for peripheral arterial thrombolysis. J Vasc Interv Radiol 2005; 16(8):1075-83; PMID:16105919; https://doi.org/10.1097/01.RVI.0000167863.10122.2A
- Moll S, Kenyon P, Bertoli L, De Maio J, Homesley H, Deitcher SR. Phase II trial of alfimeprase, a novel-acting fibrin degradation agent, for occluded central venous access devices. J Clin Oncol. 2006 Jul 1; 24(19):3056-60; PMID:16809729; https://doi.org/10.1200/JCO.2006.05.8438
- Deitcher SR, Moll S, Homesley HD, Bertoli L, Kenyon P, Swischuk P, Pena L. Safety and Efficacy of Alfimeprase for Restoring Function in Occluded Central Venous Catheters: Interim Results of a Phase 2, Multicenter, Randomized, Double-Blind Study (NuCath). Blood 2004; 104(11):1768-1768.
- Siigur E, Siigur J. Purification and characterization of lebetase, a fibrinolytic enzyme from Vipera lebetina (snake) venom. Biochim Biophys Acta 1991; 1074(2):223-9; PMID:2065076; https://doi.org/10.1016/0304-4165(91)90156-B
- Wu B, Wu L, Chen D, Yang Z, Luo M. Purification and characterization of a novel fibrinolytic protease from Fusarium sp. CPCC 480097. J Ind Microbiol Biotechnol 2009; 36(3):451-9; PMID:19142678; https://doi.org/10.1007/s10295-008-0516-5
- Nakamura S, Hashimoto Y, Mikami M, Yamanaka E, Soma T, Hino M, Azuma A, Kudoh S. Effect of the proteolytic enzyme serrapeptase in patients with chronic airway disease. Respirology 2003; 8(3):316-20; PMID:12911824; https://doi.org/10.1046/j.1440-1843.2003.00482.x
- Fujita M, Hong K, Ito Y, Fujii R, Kariya K, Nishimuro S. Thrombolytic effect of nattokinase on a chemically induced thrombosis model in rat. Biol Pharm Bull 1995; 18(10):1387-91; PMID:8593442
- Sumi H, Hamada H, Nakanishi K, Hiratani H. Enhancement of the fibrinolytic activity in plasma by oral administration of nattokinase. Acta Haematol 1990; 84(3):139-43; PMID:2123064
- Domsalla A, Melzig MF. Occurrence and properties of proteases in plant latices. Planta Med 2008; 74(7):699-711; PMID:18496785; https://doi.org/10.1055/s-2008-1074530
- Prasad S, Kashyap RS, Deopujari JY, Purohit HJ, Taori GM, Daginawala HF. Effect of Fagonia Arabica (Dhamasa) on in vitro thrombolysis. BMC Complement Altern Med 2007;7:36; PMID:17986325; https://doi.org/10.1186/1472-6882-7-36
- Rahman MA, Sultana R, Bin Emran T, Islam MS, Rahman MA, Chakma JS, Rashid HU, Hasan CM. Effects of organic extracts of six Bangladeshi plants on in vitro thrombolysis and cytotoxicity. BMC Complement Altern Med 2013;13:25; PMID:23363538; https://doi.org/10.1186/1472-6882-13-25
- Emran TB, Rahman MA, Uddin MM, Rahman MM, Uddin MZ, Dash R, Layzu C. Effects of organic extracts and their different fractions of five Bangladeshi plants on in vitro thrombolysis. BMC Complement Altern Med 2015;15:128; PMID:25902818; https://doi.org/10.1186/s12906-015-0643-2
- Patel GK, Kawale AA, Sharma AK. Purification and physicochemical characterization of a serine protease with fibrinolytic activity from latex of a medicinal herb Euphorbia hirta. Plant Physiol Biochem 2012;52:104-11; PMID:22305073; https://doi.org/10.1016/j.plaphy.2011.12.004
- Domsalla A, Gorick C, Melzig MF. Proteolytic activity in latex of the genus Euphorbia–a chemotaxonomic marker? Pharmazie 2010; 65(3):227-30; PMID:20383946
- Rajesh R, Shivaprasad HV, Gowda CD, Nataraju A, Dhananjaya BL, Vishwanath BS. Comparative study on plant latex proteases and their involvement in hemostasis: a special emphasis on clot inducing and dissolving properties. Planta Med 2007; 73(10):1061-7; PMID:17691056; https://doi.org/10.1055/s-2007-981575
- Shivaprasad HV, Riyaz M, Venkatesh Kumar R, Dharmappa KK, Tarannum S, Siddesha JM, Rajesh R, Vishwanath BS. Cysteine proteases from the Asclepiadaceae plants latex exhibited thrombin and plasmin like activities. J Thromb Thrombolysis 2009; 28(3):304-8; PMID:18979066; https://doi.org/10.1007/s11239-008-0290-2
- Fonseca KC, Morais NC, Queiroz MR, Silva MC, Gomes MS, Costa JO, Mamede CC, Torres FS, Penha-Silva N, Beletti ME, et al. Purification and biochemical characterization of Eumiliin from Euphorbia milii var. hislopii latex. Phytochemistry 2010; 71(7):708-15; PMID:20206951; https://doi.org/10.1016/j.phytochem.2010.02.009
- Errasti ME, Prospitti A, Viana CA, Gonzalez MM, Ramos MV, Rotelli AE, Caffini NO. Effects on fibrinogen, fibrin, and blood coagulation of proteolytic extracts from fruits of Pseudananas macrodontes, Bromelia balansae, and B. hieronymi (Bromeliaceae) in comparison with bromelain. Blood Coagul Fibrinolysis 2016; 27(4):441-9; PMID:26886361; https://doi.org/10.1097/MBC.0000000000000531
- Sung YY, Yang WK, Kim HK. Antiplatelet, anticoagulant and fibrinolytic effects of Litchi chinensis Sonn. extract. Mol Med Rep 2012; 5(3):721-4; PMID:22200762; https://doi.org/10.3892/mmr.2011.735
- Siritapetawee J, Thumanu K, Sojikul P, Thammasirirak S. A novel serine protease with human fibrino(geno)lytic activities from Artocarpus heterophyllus latex. Biochim Biophys Acta 2012; 1824(7):907-12; PMID:22579962; https://doi.org/10.1016/j.bbapap.2012.05.002
- Viana CA, Oliveira JS, Freitas CD, Alencar NM, Carvalho CP, Nishi BC, Ramos MV. Thrombin and plasmin-like activities in the latices of Cryptostegia grandiflora and Plumeria rubra. Blood Coagul Fibrinolysis 2013; 24(4):386-92; PMID:23314383; https://doi.org/10.1097/MBC.0b013e32835d540b
- Siritapetawee J, Sojikul P, Klaynongsruang S. Biochemical characterization of a new glycosylated protease from Euphorbia cf. lactea latex; Plant Physiol Biochem 2015;92:30-8; PMID:25900422; https://doi.org/10.1016/j.plaphy.2015.04.012
- Koudelka S, Mikulik R, Masek J, Raska M, Turanek Knotigova P, Miller AD, Turanek J. Liposomal nanocarriers for plasminogen activators. J Control Release 2016;227:45-57; PMID:26876783; https://doi.org/10.1016/j.jconrel.2016.02.019
- Perkins WR, Vaughan DE, Plavin SR, Daley WL, Rauch J, Lee L, Janoff AS. Streptokinase entrapment in interdigitation-fusion liposomes improves thrombolysis in an experimental rabbit model. Thromb Haemost 1997; 77(6):1174-8; PMID:9241753
- Kim IS, Choi HG, Choi HS, Kim BK, Kim CK. Prolonged systemic delivery of streptokinase using liposome. Arch Pharm Res 1998; 21(3):248-52; PMID:9875439
- Nguyen PD, O'Rear EA, Johnson AE, Patterson E, Whitsett TL, Bhakta R. Accelerated thrombolysis and reperfusion in a canine model of myocardial infarction by liposomal encapsulation of streptokinase. Circ Res 1990; 66(3):875-8; PMID:2306811; https://doi.org/10.1161/01.RES.66.3.875
- Vaidya B, Agrawal GP, Vyas SP. Platelets directed liposomes for the delivery of streptokinase: development and characterization. Eur J Pharm Sci 2011; 44(5):589-94; PMID:22009110; https://doi.org/10.1016/j.ejps.2011.10.004
- Jin SE, Kim IS, Kim CK. Comparative effects of PEG-containing liposomal formulations on in vivo pharmacokinetics of streptokinase. Arch Pharm Res 2015; 38(10):1822-9; PMID:25851624; https://doi.org/10.1007/s12272-015-0594-7
- Absar S, Nahar K, Kwon YM, Ahsan F. Thrombus-targeted nanocarrier attenuates bleeding complications associated with conventional thrombolytic therapy. Pharm Res 2013; 30(6):1663-76; PMID:23468049; https://doi.org/10.1007/s11095-013-1011-x
- Erdogan S, Ozer AY, Bilgili H. In vivo behaviour of vesicular urokinase. Int J Pharm 2005; 295(1-2):1-6; PMID:15847986; https://doi.org/10.1016/j.ijpharm.2005.01.021
- Wang XT, Li S, Zhang XB, Hou XP. [Preparation of thrombus-targeted urokinase liposomes and its thrombolytic effect in model rats]. Yao Xue Xue Bao 2003; 38(3):231-5; PMID:12830724
- Lu CP, Yang H, Wang J, Dong XL. [Thrombolysis of rabbit's pulmonary embolism with thrombus-targeted urokinase immune liposome]. Zhonghua Xin Xue Guan Bing Za Zhi 2009; 37(11):1035-8; PMID:20137334
- Saxena V, Gacchina Johnson C, Negussie AH, Sharma KV, Dreher MR, Wood BJ. Temperature-sensitive liposome-mediated delivery of thrombolytic agents. Int J Hyperthermia 2015; 31(1):67-73; PMID:25766387; https://doi.org/10.3109/02656736.2014.991428
- Kandadai MA, Meunier JM, Hart K, Holland CK, Shaw GJ. Plasmin-loaded echogenic liposomes for ultrasound-mediated thrombolysis. Transl Stroke Res 2015; 6(1):78-87; PMID:25411015; https://doi.org/10.1007/s12975-014-0376-4