ABSTRACT
Liquorice (Glycyrrhiza uralensis Fisch.) is one of the most widely used plants in food production, and it can also be used as an herbal medicine or for reclamation of salt-affected soils. Under salt stress, inhibition of plant growth, nutrient acquisition and symbiotic interactions between the medicinal legume liquorice and rhizobia have been observed. We recently evaluated the interactions between rhizobia and root-colonizing Pseudomonas in liquorice grown in potting soil and observed increased plant biomass, nodule numbers and nitrogen content after combined inoculation compared to plants inoculated with Mesorhizobium alone. Several beneficial effects of microbes on plants have been reported; studies examining the interactions between symbiotic bacteria and root-colonizing Pseudomonas strains under natural saline soil conditions are important, especially in areas where a hindrance of nutrients and niches in the rhizosphere are high. Here, we summarize our recent observations regarding the combined application of rhizobia and Pseudomonas on the growth and nutrient uptake of liquorice as well as the salt stress tolerance mechanisms of liquorice by a mutualistic interaction with microbes. Our observations indicate that microbes living in the rhizosphere of liquorice can form a mutualistic association and coordinate their involvement in plant adaptations to stress tolerance. These results support the development of combined inoculants for improving plant growth and the symbiotic performance of legumes under hostile conditions.
Introduction
Salt-affected arid lands negatively impact agricultural production and the livelihood of rural populations in many regions of the world, making them a major environmental problem. Phytoremediation of saline soils with nitrogen-fixing legumes such as liquorice has the potential to become a promising technique for the restoration of soil fertility because legumes can increase nitrogen content, improve organic matter quality, stimulate biological activity, and improve the water-holding capacity of soils. Citation1 In regards to their medicinal use, liquorice roots have been used since ancient times for the treatment of lung diseases, cardiovascular ailments, gastrointestinal disease, and various infection illnesses. Citation2,3,4 Essentially, salinity (i) inhibits root development, thus decreasing the ability of plants to absorb soil minerals, and (ii) affects rhizobia - host symbioses and the process of nodule initiation. Citation5,6,7 Plants rely on their microbiome for specific traits and activities, including growth promotion, nutrient acquisition, induced systemic resistance, and tolerance to abiotic stress factors. Citation8,9 Living in the rhizosphere or inside plants, microbes deliver metabolites that can be used as nutrients for partner organisms and are beneficial for plant growth. Citation10 We recently showed that liquorice tolerates salt stress under conditions of 75 mM NaCl, but nodulation is inhibited. The disturbance in nodule formation was linked to a reduced population size and survival rate of rhizobia in the rhizosphere of liquorice. However, combined inoculation with P. extremorientalis and the symbiotic Mesorhizobium significantly enhanced plant growth, nutrient acquisition, and nodulation compared to inoculation with the rhizobial strain alone. Synergistic associations between root-colonizing beneficial bacteria and rhizobia are beneficial to plants by synthesising biologically active compounds, such as phytohormones, antifungal compounds, osmoprotectants and siderophores, thus improving the availability of nutrients to plants and inducing a plant defense against various stress factors, including drought and salinity.Citation11,12
Despite many reports on the growth, nutrition and physiology of liquorice, studies concerning the associated microbes in the rhizosphere, particularly the physiological interactions between the host and microbes under natural field conditions, are not yet understood.Citation13 This knowledge is important for our understanding of the relationship between root-colonizing bacteria, rhizobia and their hosts under hostile environments, such as salinization, and for developing the best management practices for restoring degraded lands. In this Addendum, we provide an addition to our observations on the beneficial synergistic effects of rhizobia and root-colonizing bacteria on Glycyrrhiza uralensis, their potential to improve the stress tolerance of the plant and their symbiotic performance under salt-affected arid field conditions.
Plant growth and nutrient acquisition
We recently evaluated the effect of dual inoculation of liquorice with the Mesorhizobium sp. strain NWXJ31 and root-colonizing Pseudomonas extremorientalis strain TSAU20 on the growth, nodulation and N uptake of salt-stressed liquorice grown in potting soil.Citation14 Here we report that also under open-field conditions in Uzbekistan, the growth and nutrient acquisition of liquorice responded positively to microbial inoculation in saline soil. The soil was selected from salt-affected fields with an electrical conductivity of 7.9 dS m−1, Nt 0.01, P 1.2, Ct 0.25, Mg 21.0, Ca 63.5, K 6.2, Cl 0.1 g/kg. Bacterial inoculants were prepared, and the germinated seeds were inoculated by immersing seeds in cell suspensions, as described by Egamberdieva et al.Citation14 The plants inoculated with Mesorhizobium sp alone showed slower growth compared to co-inoculated plants. The shoot and root dry weights of the plants co-inoculated with Mesorhizobium sp. and P. extremorientalis were 27% higher compared to uninoculated plants (). A decline in plant growth regulators under salt stress has been reported,Citation15 which has been shown to result in an inhibition of root growth, root hair formation and a disturbance of nutrient acquisition from soil. According to López-Bucio et al.,Citation16 the modulation of the root system architecture by root-colonizing bacteria is related to the production of plant growth–regulating substances due to the extensive supply of substrates exuded from the roots. When colonizing the root system, the Pseudomonas strain produces the plant growth regulator auxin as well as fungal cell wall-degrading enzymes and may deliver metabolites directly to the rhizosphere of plants. The enlarged root system contains more available niches in the rhizosphere for the colonization by rhizobia.Citation17
Figure 1. Effect of P. extremorientalis TSAU20 and Mezorhizobium sp NWXJ31 inoculation on liquorice dry weight. Plants were grown under an open-field condition for 3 months in pots with saline soil. Columns represent the means of 4 plants (n = 5), with error bars showing standard deviation. Columns marked with an asterisk differed significantly from uninoculated plants at P < 0.05 (Student's t test).
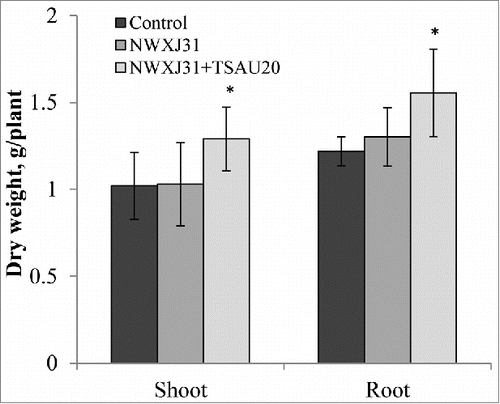
These properties, either together or alone, may explain the capacity of the inoculant P. extremorientalis to improve root growth and nutrient acquisition. Generally, salt stress inhibits the acquisition of nutrients by plants, such as nitrogen (N), phosphorus (P), potassium (K), and magnesium (Mg), through an antagonistic relationship of sodium.Citation18 In our study, the combined inoculation of Pseudomonas with rhizobia resulted in higher N, P, K, and Mg contents in liquorice by 24, 19, 27, and 9%, respectively, indicating that increased nodulation and growth correlate with N acquisition (). Liu et al.Citation19 observed increased plant biomass and phosphorus concentrations in the leaves and roots of co-inoculated liquorice with AM fungi compared to uninoculated plants. In another study, dual inoculation with Sinorhizobium ciceri and phosphate-solubilizing Pseudomonas was reported to increase the concentration of P in chickpea.Citation20 The phosphate-solubilizing activity of P. extremorientalis TSAU20 was determined by the method of Sperber Citation21; we found that the strain was able to solubilize phosphate, thus providing more phosphorus to liquorice.
Figure 2. Effect of P. extremorientalis TSAU20 and Mezorhizobium sp. NWXJ31 inoculation on nitrogen (N), phosphorus (P), potassium (K), and magnesium (Mg) contents in liquorice. Plants were grown in open-field conditions for 3 months in pots with saline soil. Columns represent the means of 4 plants (n = 5), with error bars showing standard deviation. Columns marked with an asterisk differed significantly from uninoculated plants at P < 0.05 (Student's t test).
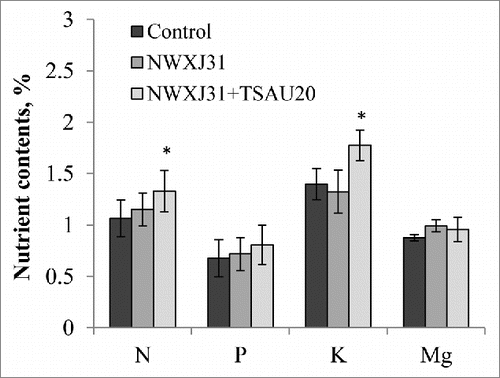
Physiological parameters
Some reports have suggested that the mutualistic interaction between root-associated microbes modifies the physiological status of host plants and induced systemic tolerance (IST) in plants through elevated antioxidant responses.Citation22
Salt stress inhibits the production of photosynthetic pigments due to changes in the synthesis of chlorophyll-related proteins and therefore reduces plant growth and development.Citation23 The chlorophyll contents in the leaves of liquorice as measured by the method of Richardson et al.Citation24 showed that chlorophyll a and b were lower compared to plants inoculated with microbes (). Compared to single inoculation, the combination of P. extremorientalis TSAU20 and Mesorhizobium sp NWXJ31 increased chlorophyll a and b content by 39 and 36%, respectively, which may be a mutual result of positive modifications in the plants. Enhanced chlorophyll synthesis as well as photosynthetic electron transport due to Pseudomonas inoculation under water stress conditions have been reported for the medicinal plant Ocimum basilicum. Citation25
Table 1. Effect of microbial inoculants on chlorophyll contents and hydrogen peroxide levels, as well as superoxide dismutase, peroxidase, glutathione reductase, and catalase activities in Glycyrrhiza uralensis. Values are shown as the mean ± SE (n = 5). Columns marked with an asterisk differed significantly from uninoculated plants at P < 0.05 (Student's t test).
We also elucidated the salt stress defense and tolerance mechanisms of liquorice by the mutualistic interaction between Mesorhizobium sp. NWXJ31 and P. extremorinetalis TSAU20. The hydrogen peroxide (H2O2) content of leaf samples was measured as described by Mukherjee and Choudhuri.Citation26 Uninoculated liquorice grown in saline soil contained a higher level of H2O2 in its leaves compared to inoculated plants. The enhanced membrane leakage by salt stress was reported to be correlated with H2O2 production, which causes a disturbance in cellular homeostasis.Citation27 Plants co-inoculated with P. extremorientalis TSAU20 and Mesorhizobium sp NWXJ31 showed a 35% reduction in H2O2 production, whereas single inoculation with NWXJ31 decreased H2O2 production only by 10% (). Our present observations support the mutualistic benefits of rhizobia and root-colonizing bacteria in protecting membrane lipids from peroxidation.
The antioxidant defense system in plants is important for counteracting salt stress-induced oxidative damage.Citation22,23 The antioxidant enzymes in plant tissues, namely peroxidase (POD), catalase (CAT), superoxide dismutase (SOD) and glutathione reductase (GR), are also affected by bacterial inoculation under salt stress. The activities of SOD (EC 1.15.1.1) were determined by Giannopolitis and RiesCitation28; POD (EC 1.11.1.7) by procedures of Kar and MishraCitation29; CT (EC 1.11.1.6) as reported by Chance and MaehlyCitation30; and GR (EC 1.6.4.2) as described by Carlberg and Mannervik.Citation31 Antioxidant enzyme activities (SOD, POD, GR, and CAT) increased following the combined inoculation of liquorice with TSAU20 and NWXJ31 compared to single inoculation with NWXJ31 and uninoculated control plants. Dual inoculation led to significant increases in SOD and CT activities (26% above untreated plants), whereas POD and GR activities were only slightly but not significantly improved. Similar observations have been reported for chickpeaCitation32 and Indian mustard.Citation27 It has been concluded that SOD reduces the formation of hydroxyl (OH−) radicals, and CAT mediates the quick removal of H2O2, thereby assisting in the normal functioning of membranes.Citation33 An overview of the beneficial properties of PGPR on plant growth, symbiotic performance and stress tolerance is provided in .
Figure 3. Schematic illustration of the main effects of plant growth-promoting rhizobacteria (PGPR) on the growth and stress tolerance of plants. The root-colonizing PGPR strain stimulates root growth, nutrient uptake and nodule formation through supplying additional phytohormones, mobilizes minerals and alleviates the adverse effects of oxidative stress. IAA: indole-3 acetic acid, ROS: reactive oxygen species.
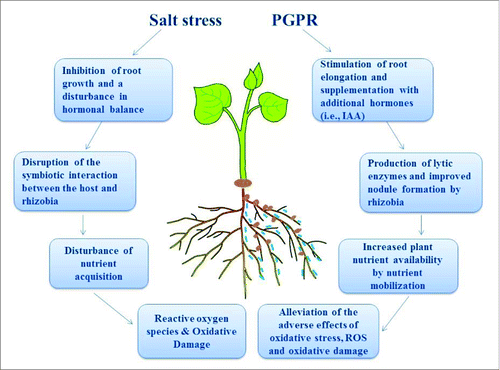
Conclusion
As shown in our previous study, the combined inoculation of liquorice with P. extremorientalis TSAU20 and Mesorhizobium sp. mitigated salt stress and increased nitrogen acquisition as well as nodule numbers compared with single-inoculated liquorice. Our observations in this study indicate that root-colonizing Pseudomonas and symbiotic Mesorhizobium strains are both involved in promoting specific tolerance mechanisms in response to salt stress. Mutualistic interactions between microbes in the rhizosphere enhanced the activities of antioxidant enzymes SOD and CT, thereby preventing reactive oxygen species (ROS)-induced oxidative damage. Furthermore, we showed enhanced plant growth, nutrient acquisition and the production of photosynthetic pigments of liquorice by combined inoculation under saline soil conditions. In conclusion, we suggest that the application of an efficient consortium of root-colonizing bacteria and rhizobia in the cultivation of liquorice under hostile environmental conditions may help farmers to increase plant production in a sustainable way.
Disclosure of potential conflicts of interest
No potential conflicts of interest were disclosed.
Funding
This work was supported by the Alexander von Humboldt Fellowship. The authors would like to extend their sincere appreciation to the Deanship of Scientific Research at King Saud University for its funding this research group NO. (RG-1435-014).
References
- Kushiev H, Noble AD, Abdullaev I, Toshbekov V. Remediation of abandoned saline soils using Glycyrrhiza glabra: A study for the Hungry Steppes of Central Asia. Inter J Agric Sustain 2005; 3:102-13; https://doi.org/10.1080/14735903.2005.9684748
- Armanini D, Fiore C, Mattarello MJ, Bielenberg J, Palermo M. History of the endocrine effects of licorice. Exp Clin Endocrinol Diabetes 2002; 110:257-61; PMID:12373628; https://doi.org/10.1055/s-2002-34587
- Nomura T, Fukai T, Akiyama T. Chemistry of phenolic compounds of licorice (Glycyrrhiza species) and their estrogenic and cytotoxic activities. Pure Appl Chem 2002; 74 (7):1199-206; https://doi.org/10.1351/pac200274071199
- Sharma V, Agrawal RC, Pandey S. Phytochemical screening and determination of anti-bacterial and anti-oxidant potential of Glycyrrhiza glabra root extracts. J Envir Res Devel 2013; 7(4):1552-8.
- Bouhmouch I, Souad-Mouhsine B, Brhada F. Influence of host cultivars and Rhizobium species on the growth and symbiotic performance of Phaseolus vulgaris under salt stress. J Plant Physiol 2005; 162:1103-13; PMID:16255168; https://doi.org/10.1016/j.jplph.2004.12.003
- Egamberdieva D, Berg G, Lindström K, Räsänen LA. Alleviation of salt stress of symbiotic Galega officinalis L. (goat's rue) by co-inoculation of Rhizobium with root-colonizing Pseudomonas. Plant Soil 2013; 369:453–465. https://doi:10.1007/s11104-013-1586-3
- Egamberdieva D, Jabborova D, Berg G. Synergistic interactions between Bradyrhizobium japonicum and the endophyte Stenotrophomonas rhizophila and their effects on growth, and nodulation of soybean under salt stress. Plant Soil 2016; 405:35–45. https://doi:10.1007/s11104-015-2661-8
- Egamberdieva D, Wirth S, Behrendt U, Abd-Allah EF, Berg G. Biochar treatment resulted in a combined effect on soybean growth promotion and a shift in plant growth promoting rhizobacteria. Front Microbiol 2016; 7:209; PMID:26941730; https://doi.org/10.3389/fmicb.2016.00209
- Malfanova N, Kamilova F, Validov S, Shcherbakov A, Chebotar V, Tikhonovich I, Lugtenberg B. Characterization of Bacillus subtilis HC8, a novel plant-beneficial endophytic strain from giant hogweed. Microb Biotech 2011; 4:523-32; https://doi.org/10.1111/j.1751-7915.2011.00253.x
- Sessitsch A, Kuffner M, Kidd P, Vangronsveld J, Wenzel W, Fallmann K, Puschenreiterd M. The role of plant-associated bacteria in the mobilization and phytoextraction of trace elements in contaminated soils. Soil Biol Biochem 2013; 60:182-94; PMID:23645938; https://doi.org/10.1016/j.soilbio.2013.01.012
- Vacheron J, Desbrosses G, Bouffaud ML, Touraine B, Loccoz YM, Muller D, Legendre L, Wisniewski-Dyé F, Prigent-Combaret C. Plant growth-promoting rhizobacteria and root system functioning. Front Plant Science 2013; 4:356; https://doi.org/10.3389/fpls.2013.00356
- Van der Heijden MAG, de Bruin S, Luckerhoff L, van Logtestijn RSP, Schlaeppi K. A widespread plant-fungal-bacterial symbiosis promotes plant biodiversity, plant nutrition and seedling recruitment. ISME J 2016; 10:389-99; PMID:26172208; https://doi.org/10.1038/ismej.2015.120
- Köberl M, Schmidt R, Ramadan EM, Bauer R, Berg G. The microbiome of medicinal plants: diversity and importance for plant growth, quality and health. Front Microbiol 2013; 4:400; PMID:24391634
- Egamberdieva D, Li Li, Lindström K, Räsänen L. A synergistic interaction between salt tolerant Pseudomonas and Mezorhizobium strains improves growth and symbiotic performance of liquorice (Glycyrrhiza uralensis Fish.) under salt stress. Appl Microb Biotech 2015; 100(6):2829-41; https://doi.org/10.1007/s00253-015-7147-3
- Debez A, Chaibi W, Bouzid S. Effect of NaCl and growth regulators on germination of Atriplex halimus L. Cah Agric 2001; 10:135-8.
- López-Bucio J, Campos-Cuevas JC, Hernández-Calderón E, Velásquez-Becerra C, Farías-Rodríguez R, Macías-Rodríguez LI, Valencia Cantero E. Bacillus megaterium rhizobacteria promote growth and alter root system architecture through an auxin and ethylene-independent signaling mechanism in Arabidopsis thaliana. Mol Plant Microbe Inter 2002; 20:207-17; https://doi.org/10.1094/MPMI-20-2-0207
- Cho S-T, Chang H-H, Egamberdieva D, Kamilova F, Lugtenberg B, Kuo C-H. Genome Analysis of Pseudomonas fluorescens PCL1751: A Rhizobacterium that Controls Root Diseases and Alleviates Salt Stress for Its Plant Host 2015; PLoS ONE 10(10):e0140231. https://doi.org/10.1371/journal.pone.0140231
- Näsholm T, Kielland K, Ganeteg U. Uptake of organic nitrogen by plants. New Phytologist 2009; 182(1):31-48; https://doi.org/10.1111/j.1469-8137.2008.02751.x
- Liu J, Wu L, Wei S, Xiao X, Su C, Jiang P, Song Z, Wang T, Yu Z. Effects of arbuscular mycorrhizal fungi on the growth, nutrient uptake and glycyrrhizin production of licorice (Glycyrrhiza uralensis Fisch). Plant Growth Regul 2007; 52:29-39; https://doi.org/10.1007/s10725-007-9174-2
- Messele B, Pant LM. Effects of inoculation of Sinorhizobium ciceri and phosphate solubilizing bacteria on nodulation, yield and nitrogen and phosphorus uptake of Chickpea (Cicer arietinum L.) in Shoa Robit Area. J Biofertilizers Biopesticides 2012; 3:129.
- Sperber JI. Solution of apatite by soil microorganisms producing organic acids. Austr J Agricultural Res 1958; 9:778-81; https://doi.org/10.1071/AR9580778
- Hashem A, Abd_Allah EF, Alqarawi AA, Aldebasi A, Egamberdieva D. Arbuscular mycorrhizal fungi enhances salinity tolerance of Panicum turgidum Forssk by altering photosynthetic and antioxidant pathways. J Plant Inter. 10: 230–242. https://doi.org/10.1080/17429145.2015.1052025
- Hashem A, Abd_Allah EF, Alqarawi AA, Egamberdieva D. Induction of salt stress tolerance in cowpea (Vigna unguiculata L. Walp) by arbuscular mycorrhizal fungi. Legume Res 2014; 38:579-88.
- Richardson AD, Duigan SP, Berlyn GP. An evaluation of noninvasive methods to estimate foliar chlorophyll content. New phytologist 2002; 153:185-94; https://doi.org/10.1046/j.0028-646X.2001.00289.x
- Heidari M, Golpayegani A. Effects of water stress and inoculation with plant growth promoting rhizobacteria (PGPR) on antioxidant status and photosynthetic pigments in basil (Ocimum basilicum L.). J Saudi Society Agri Sci 2012; 11:57-61.
- Mukherjee SP, Choudhuri MA. Implication of water stress-induced changes in the levels of endogenous ascorbic acid and hydrogen peroxide in Vigna seedlings. Physiol Plant 1983; 58:166-70; https://doi.org/10.1111/j.1399-3054.1983.tb04162.x
- Ahmad P, Hashem A, Abd-Allah EF, Alqarawi AA, John R, Egamberdieva D, Gucel S. Role of Trichoderma harzianum in mitigating NaCl stress in Indian mustard (Brassica juncea L) through antioxidative defense system. Front Plant Sci 2015; 6:868; PMID:26528324
- Giannopolitis CN, Ries SK. Superoxide Dismutases: II. Purification and Quantitative Relationship with Water-soluble Protein in Seedlings. Plant Physiol 1977; 59(2):315–318.
- Kar M, Mishra D. Catalase, peroxidase, polyphenyl oxidase activities during rice leaf senescence. Plant Physiol 1976; 57:315-319; PMID:16659474; https://doi.org/10.1104/pp.57.2.315
- Chance B, Maehly C. Assay of catalase and peroxidases. Methods Enzymol 1955; 11:764-75; https://doi.org/10.1016/S0076-6879(55)02300-8
- Carlberg I, Mannervik B. Glutathione reductase. Methods Enzymol 1985; 113:484-90; PMID:3003504; https://doi.org/10.1016/S0076-6879(85)13062-4
- Rasool S, Ahmad A, Siddiqi TO, Ahmad P. Changes in growth, lipid peroxidation and some key antioxidant enzymes in chickpea genotypes under salt stress. Acta Physiol Plant 2013; 35:1039-50; https://doi.org/10.1007/s11738-012-1142-4
- Wu QS, Ying-Ning Z, Abd-Allah EF. Mycorrhizal Association and ROS in Plants. In: P. Ahmad (Ed): Oxidative Damage to Plants 2014; pp. 453–475. https://doi.org/10.1016/B978-0-12-799963-0.00015-0