ABSTRACT
The extract of Phyllodium (P.) elegans was investigated for its anti-cancer properties on brain astroglioma cells (U251-MG), colorectal carcinoma cells (HCT116), and malignant melanoma cells (A375). P. elegans methanolic extract (PeME) showed cytotoxicity on all three cancer cell lines tested. The cell viability assay revealed that PeME significantly reduced the viability of these cells. Clear apoptotic features such as cellular morphology, cell shrinkage, and augmentation of dead cells were observed. Flow cytometry and fluorescence staining techniques confirmed the apoptotic property of PeME. In vitro scratch invasion assay showed that cell migration rate was significantly reduced. Fluorescence microscopic studies using 4′,6-diamidino-2-phenylindole staining showed early and late signs of apoptosis after PeME treatment. Upon PeME stimulation, activation of caspase-3/-9 and Mu-2-related death-inducing gene (MUDENG, MuD) was observed by western blot analysis. JC-1 staining analysis by flow cytometry showed that PeME depolarized the mitochondria membrane potential (MMP). Collectively, these findings, for the first time, point to the fact that PeME has anti-cancer properties against brain, colon, and skin cancer cell lines by depolarizing the MMP and activating apoptotic signaling through the activation of caspase-3/-9 as well as MuD. This is the first report reporting the anticancer activity of this specific plant extract.
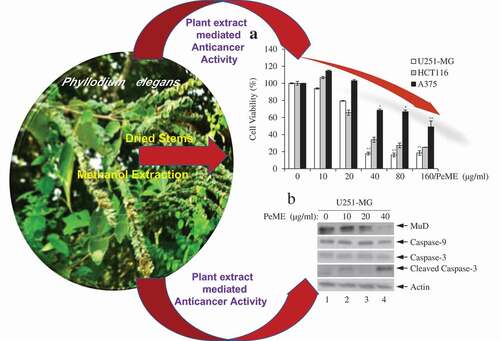
Introduction
In recent years, remarkable progress has been made toward the understanding of cancer and its treatment. However, in spite of improved state-of-the-art-medicines such as radiation therapy, surgery, and chemotherapy, the survival rate and life expectancy of cancer patients have not been extended owing to side effects and resistance to anti-cancer drugs [Citation1,Citation2]. Therefore, nature-based or nature-derived medicines are receiving increasing attention as better therapeutic options for the prevention of human cancers [Citation3,Citation4]. To date, a large part of chemotherapeutic drugs originates from naturally-derived products [Citation5]. The traditional use of plants for medicinal purposes has attracted extensive research attention to explore possible bioactive components for anti-cancer drugs [Citation6,Citation7]. Furthermore, numerous plant products are known to induce apoptosis in cancer cells but not in normal cells [Citation8].
Phyllodium (P) is a small genus within the tribe Desmodieae (Leguminosae-Papilionoideae) that is distributed in India, southeastern and eastern Asia, and northern Australia [Citation9]. P. elegans (Lour) Desv. (Taxon Name) is one of the six Phyllodium species, referred to as Desmodium blandum, D. elegans, Dicerma elegans, Hedysarum elegans as synonyms [Citation10]. The roots and leaves are used medicinally to reduce fever and as an antiphlogistic, carminative, and diuretic in humans [Citation10–Citation12]. According to the Editorial Committee of Zhonghua Bencao National Traditional Chinese Herb Administration [Citation13], a paste of the whole plant is also employed to stop bleeding and in the treatment of burn injuries, fractures, and bruises [Citation14]. The root is used in the treatment of rheumatic pain and chronic hepatitis [Citation14].
Apoptosis is a pivotal mode of action through intrinsic or extrinsic pathways by anti-tumor agents. In cells undergoing apoptosis, a family of proteases called caspases is activated. Activation of caspases appears to be directly responsible for many molecular and structural changes in the apoptotic pathway [Citation15,Citation16].
Mu-2-related death-inducing gene (MUDENG, MuD) protein contains a Mu homology domain found in adaptor proteins that play important roles in intracellular trafficking pathways. It is known to be involved in the initial stages of cell death in cytotoxic T cells [Citation17]. Furthermore, we have reported that MuD possesses anti-apoptotic functions [Citation18], and is involved in silver nanoparticle (AgNP)-induced astroglioma hormesis [Citation19]. In addition, we reported the correlation between graviola anti-cancer activity and MuD [Citation20].
Currently, no published reports exist reporting the anti-cancer activity of P. elegans (Lour.) Desv. Thus, this study was performed to investigate the cytotoxic activity of P. elegans methanolic extract (PeME) against three human cancer cell lines. This is the first time report that PeME treatment-induced cell viability reduction, morphological alteration, and cellular apoptosis through alleviation of mitochondrial membrane potential (MMP) on brain astroglioma cells, colorectal carcinoma cells, and human malignant melanoma cells. This less studied extract adds novel value in natural product research and is expected to trigger off sensitization for application toward versatile anticancer natural remedies.
Materials and methods
Chemicals and reagents
FITC-Annexin V and propidium iodide (PI) were bought from BD Biosciences (San Jose, CA, USA). JC-1 dye was purchased from Enzo Life Sciences (Farmingdale, NY, USA). 4′,6-diamidino-2-phenylindole (DAPI) was purchased from Sigma-Aldrich (Saint Louis, MO, USA). Primary antibody (Ab) for caspase-3 was from Cell Signaling Technology (Beverly, MA, USA). Caspase-9 Ab and actin Ab were from Santa Cruz Biotechnology (Dallas, TX, USA). Mouse MuD monoclonal Abs (MAb) were produced from C22B3 hybridoma culture medium [Citation21]. Horseradish peroxidase (HRP)-conjugated goat anti-mouse IgG Ab and goat anti-rabbit IgG Ab were purchased by the Jackson ImmunoResearch Lab (West Groove, PA, USA). 2-(4-iodophenyl)-3-(4-nitrophenyl)-5-(2,4-disulfophenyl)-2H-tetrazolium monosodium salt (WST-1) reagent was purchased from Roche Applied Science (Mannheim, Germany). Recombinant Human sTRAIL/Apo2 ligand was purchased from PeproTech (Seoul, Korea).
Preparation of P. elegans extract
Methanol extract of P. elegans stem was supplied by Foreign Plant Extract Bank (no. FBM118-023; Daejeon, Korea). The plant was collected by Sydara K (one of the authors of the paper) in Ham Ao, Laos in 2010 and authenticated by Institute of Traditional Medicine (ITM). A voucher specimen recoded as ‘Korea Research Institute of Bioscience and Biotechnology (KRIBB) 0033580ʹ was deposited in the herbarium of the Korea KRIBB. Briefly, the dried and refined aerial parts of P. elegans (92g) were extracted with 1,000 mL of 99.9% (v/v) methanol using a sonicator (SDN-900H, SD Ultrasonic Cleaner, Seoul, Korea) at 45°C for 3 days (15 min sonication at 1500 W and 40 kHz followed by 2 h standing; repeated 10 times per day). The resultant product was filtered with non-fluorescent cotton, condensed using a rotary evaporator (N-1000SWD, EYELA, Tokyo, Japan) under reduced pressure at 45°C, and lyophilized using a freeze dryer (Christ, Germany). Dimethyl sulfoxide (DMSO) was used as a solvent for lyophilized substances.
Liquid chromatography (LC)- mass spectrometry (MS) analysis of the methanolic extract
LC- electrospray ionization (ESI)-MS, LC- ESI-tandem mass spectrometric (MS/MS), and LC-ESI-MS/MS-multiple reaction monitoring (MRM) studies were performed for the identification of bioactive compounds in the methanolic extract of P. elegans. In the first step, methanolic extract was partitioned with ethyl acetate to minimize the background noise in the MS analysis. Briefly, 5 mg of isolated extract was dissolved in 3 ml water and partitioned with 5 ml ethyl acetate. The upper ethyl acetate fraction was collected the procedure was repeated three times. Subsequently, all the fractions were pooled, dried in a rotary vacuum evaporator, recovered in 1 ml methanol, and filtered through a Whatman (0.45 µm) filter.
The ESI-MS and MS/MS analyses were performed by SCIEX API 3200 triple quadrupole mass spectrometer (AB-SCIEX, USA) equipped with an Exion LC™ system and Turbo V™ interface with Turbo spray ion source. The ESI-MS and MS/MS analysis were performed in negative (-) mode, following the liquid chromatographic (LC)-separation in Luna® 5 µm C18(2) column (100 Å, 250 × 4.6 mm) with TMS end-capping. The column thermostat was maintained at 20°C. The solvent system consisting of 0.1% formic acid (v/v) in water (mobile phase A) and acetonitrile with 0.1% formic acid (Mobile phase B). The gradient elution was 10–50% B in 30 min, 50–100% B in next 15 min, hold at 100% B for 10 min, followed by 5-minute post-run at a flow rate of 1 ml/min.
The ESI-MS source parameters were optimized as follows: dry gas, N2; curtain gas (CUR), 30 psi; ion source gas (GAS1), 50 psi; GAS2, 50 psi; temperature, 700°C; and ion spray voltage, 4000 V. The Q1 scan was performed at delustring potential (DP) of −25 to −50 V and the mass spectra were acquired in the range of 60–1000 m/z at 1 s interval.
The LC-MS/MS-MRM based targeted metabolomics approach was employed for the identification of commonly occurring polyphenols in the extract. The optimized values of collision energy [Citation22], cell exit potential (CXP), decluttering potential (DP), entrance potential [Citation16] of selected MRM transition that were used for identification are listed in Table S1 (supplementary material).
Preparation of cell line and cell culture for testings
Human malignant astroglioma cell line U251-MG was obtained from Dr Benveniste EN (University of Alabama at Birmingham, Birmingham, AL, USA). Human colorectal carcinoma cell line HCT116 and human malignant melanoma cell line A375 were obtained from Korean Cell Line Bank (Seoul, Korea). U251-MG were cultured in Minimum Essential Medium (MEM) (Welgene, Daegu, Korea), HCT116 in McCoy’s 5A Medium (McCoy’s) (Welgene), and A375 cells in Dulbecco’s Modified Eagle Medium (DMEM) (Welgene). Media were supplemented with 10% (v/v) fetal bovine serum (FBS) (Gibco, Logan, Utah, USA), 1x antibiotic (Welgene).
Cell viability assay
2.0 × 104 cells were plated in a 96-well microplate and incubated overnight. The next day, PeME-containing media of specified concentrations were replenished in triplicates. After 24 h, cells were washed with phosphate buffer saline (PBS). Then, 10 µl WST solution (0.5 mg/ml) was added in each well and incubated for an additional hour in the dark. Cell viability was measured using a colorimetric assay with WST-1 reagent. The absorbance was determined at 450 nm using a microplate reader and read with Gen5 software (Bio-Tek, Winooski, VT, USA). Methanol in medium was used as a control.
Morphological observation
2 × 104 cells were plated in a six-well plate and incubated overnight. The next day, the cells were treated with PeME of specified concentration, and cellular morphological changes were examined and imaged at 24 h under an inverted phase-contrast microscope (Motic, Kowloon, Hong Kong).
In vitro scratch motility assay
2 × 105 cells were plated in a six-well plate and incubated overnight. A liner scratch wound was created after 24 h in 1% serum-containing medium with the help of a sterile 0.2 ml yellow tip. Cell debris was removed by PBS wash (done twice), followed by PeME treatment. The wound was analyzed and photographed at 12, 24, and 36 h under an inverted phase-contrast microscope and imaged by Motic Image Plus 2.0 ML (Motic).
Nuclear morphology study by 4′,6-diamidino-2-phenylindole (DAPI) staining
2 × 104 cells were seeded in a 96-well plate and incubated overnight. Cells were treated with a specified concentration of PeME. After 24 h, cells were fixed with 4% paraformaldehyde for 20 min and permeabilized with PBS containing 0.1% Tween 20 for 10 min. After washing by PBS, the cells were treated with 300 nM DAPI solution for 5 min. Immediately, the cells were analyzed under a fluorescence microscope and imaged using NIS-Elements BR (Nikon, Melville, NY, USA).
Immunoblotting assay
Prepared cells were washed in PBS and lysed in lysis buffer as shown previously [Citation21]. Protein concentrations were determined using the Lowry protein assay (Bio-Rad, Hercules, CA) and quantified by Microplate Spectrophotometer (Bio-tek) at 750 nm. Total proteins in each cell lysate were resolved at 10% concentration of sodium dodecyl sulfate-polyacrylamide gel electrophoresis (SDS-PAGE) and transferred onto a polyvinyl difluoride membrane. After blocking for 1 h by 5% nonfat milk at 21‒23℃, membranes were incubated with shaking overnight with specific Ab at 4°C. After incubating, PVDF membranes were carried out by incubation with HRP-conjugated secondary Ab at 21‒23°C for 2 h. Bands were visualized by enhanced chemiluminescence (Bio-Rad).
Flow cytometric analysis
Each cell was seeded into six-well plates at 2 × 105 cells/well and incubated overnight. PeME was treated into each well and incubated for 24 h. Then, the cells were washed twice and resuspended in 1 ml of 1x Annexin V binding buffer at a concentration of 1 × 106 cell/ml. Following this, 100 µl of the resuspended solution (1 × 105 cells) was transferred into a 1.5 ml microtube and 5 µl of FITC-Annexin V and 5 µl of PI were added. After incubation for 20 min at 21‒23°C in the dark, 400 µl of 1x Annexin V binding buffer was added to each sample. Then, cells were analyzed by flow cytometry using NovoCyte 1000 and visualized by NovoExpress (ACEA Biosciences, San Diego, CA, USA).
Measurement of mitochondria membrane potential (MMP)
2 × 105 cells/well were seeded in a six-well plate and incubated overnight. The next day, various concentrations of PeME were treated for 24 h, and then cells were harvested with trypsin-EDTA (Welgene) and transferred into 1.5 ml microtubes (1 × 104 cell/tube). JC-1 dyes were diluted to a final concentration of 2.5 µg/ml. The cells were incubated for 15 min at 21‒23°C in the dark. After incubation, cells were washed with PBS, and resuspended in 500 µl PBS. Flow cytometry analysis was performed immediately. The solutions were divided by NovoCyte 1000 and visualized by NovoExpress (ACEA biosciences).
Statistical analysis
Student’s t-test was used to assess significant differences among treatment groups compared to untreated group using Excel. The criterion for statistical significance was set at p < 0.01. Values of p < 0.01 were considered statistically significant.
Results and discussion
Liquid chromatography (LC)- mass spectrometry (MS) analysis of the methanolic extract
In the present study, a total of 32 compounds were chromatographed in the methanolic extract of P. elegans by LC-MS (Figure S1, supplementary material). The retention time and mass spectrum characteristics of 28 identified and 4 unidentified compounds are systematically listed in . Moreover, the MS/MS fragmentation of unidentified compounds are summarized in Figure S2 (Supplementary material). The flavan-3-ols (e.g. catechins and their derivatives) were found as the dominating flavonoid polyphenols in the extract. Specifically, (-)-epicatechin and epicatechin-epicatechin (Dimer; proanthocyanidin) are identified from singly charged negative ions with a [M − H]− at m/z 289.5 and 577.8, respectively (Figures S3 and S4, supplementary material). The retro-Diels-Alder (RDA) fragmentation of the epicatechin dimers produced typically [M − H − 152]− product ions at m/z 425.7. The m/z of 407.6 [M − H − 152 − 18]− resulted from the elimination of water molecule from m/z 425.7. The [M − H − 288] − ions at m/z 289.5 result from the loss of an epicatechin molecule [Citation23]. With the help of selective multiple reaction monitoring (MRM) transitions, several non-flavonoid polyphenols, comprising of hydroxybenzoic acids, such as gallic, protocatechuic, 4-hydroxybenzoic, syringic, vanillic, and salicylic, and hydroxycinnamic acids, such as chlorogenic, caffeic, p-coumaric, and ferulic acids were identified ().
Table 1. Identification of major polyphenols in the methanolic extract of P. elegans by Liquid chromatography (LC)-electrospray ionization (ESI) -tandem mass spectrometric (MS/MS), and LC-ESI-MS/MS-multiple reaction monitoring (MRM) analysis.
Cell viability assay
Firstly, to determine the method of action of the plant extract on cancer cells, cell viability assay was performed with PeME in U251-MG, HCT116, and A375 cells. PeME treatment was done at various concentrations (0, 10, 20, 40, 80, and 160 µg/ml) for 24 h. Our findings revealed that cell growth was significantly suppressed in a concentration-dependent manner. Interestingly, it was observed that U251-MG and HCT116 cells were more sensitive toward PeME treatment than A375 cells. The IC50 values of PeME treated U251-MG, HCT116, and A375 cells were 28.275, 32.396, and 117.2 µg/ml respectively ()).
Figure 1. Cytotoxic effects of P. elegans methanolic extract (PeME)-treated glioblastoma, colon, and melanoma cancer cells. Cells were incubated for 24 h with various concentrations of PeME (0, 10, 20, 40, 80 and 160 μg/ml). (a). Cell viability was determined using WST-1 assay. Experiments were done in triplicates (n = 3) and the results were statistically significant at *P < 0.05, **P < 0.01. (b). PeME treatment changed the cell morphology and inhibited the proliferation rate of glioblastoma, colon, and melanoma cancer cells. Cells were exposed to PeME at 0, 20, 40, and 80 µg/ml concentrations respectively. The cellular morphological changes were examined at 24 h of treatment and imaged under an inverted phase-contrast microscope (100x magnification).
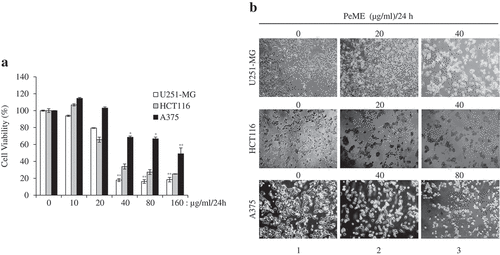
Morphological observation
The effect of PeME treatment on cell morphology in all three cell lines was studied. PeME treatment was done at various concentrations (0, 20, 40, 80 µg/ml) for 24 h. As shown in ), PeME-treated cells in a dose-dependent manner showed morphological features seen in the process of apoptosis (Lane 2–3), and not in the control (Lane 1). Treated cells clearly showed changes in their cellular morphology, cell shrinkage, and increased number of floating and dead cells. Proliferation rate in high concentration (40 and 80 µg/ml) of PeME was significantly reduced in all three cells. Subsequently, 40 µg/ml treatment of PeME in U251-MG and HCT116 cells showed that most of the cells were detached from the plate surface and floated in the media.
In vitro scratch motility assay
Tumor metastasis is a major contributor to the death of cancer patients [Citation24]. To determine whether PeME treatment induces anti-metastasis effect, in vitro scratch motility assay was performed. PeME was treated at various concentrations (0, 10, 20, 40, 80 µg/ml) for 0‒36 h. All three cells in the control wells migrated from the edges of the wound toward the centre of the scratched areas (), lane 4, the uppermost). However, it had been observed that the cell migration rate was significantly reduced in PeME-treated wells, in a time-dependent manner (), lane 2‒4, middle and bottom). Finally, these findings suggested that PeME showed potent cytotoxic, anti-metastatic, and anti-proliferative activity on cancer cells.
Figure 2. P. elegans methanolic extract (PeME) treatment showed the anti-metastatic effect and reduced the cell migration rate of the U251-MG, HCT116, and A375 cells. Wound closure ability of control and treated U251-MG (10 and 20 µg/ml), HCT116 (10 and 20 µg/ml), and A375 cells (40 and 80 µg/ml) were shown. The image of wounded wells was captured with the help of an inverted phase-contrast microscope at 0, 12, 24, 36 h (100x magnification).
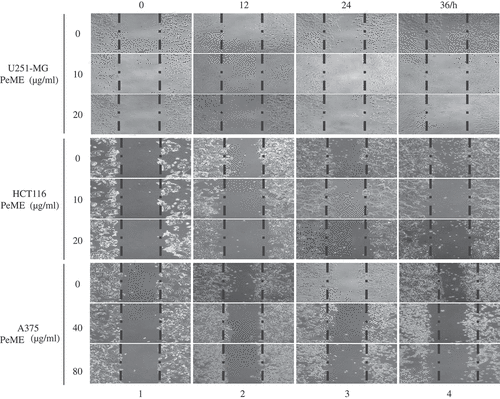
Nuclear morphology study by 4′,6-diamidino-2-phenylindole (DAPI) staining
To examine the morphological change of the cells after PeME treatment, DNA-specific fluorescent dye DAPI was used to stain cell nuclei. To do this, PeME was treated at various concentrations (0, 20, 40, 80, and 160 µg/ml) for 24 h. As shown in , all three cells showed normal intact nuclei and less bright blue fluorescence in the centre (a/d/g). In contrast, PeME-treated cells (b-c/e-f/h-I, arrow) showed bright blue fluorescent dots in the centre as well as condensed and fragmented nuclei. Moreover, at higher concentrations (c/f/i, arrow), cells with distorted nuclei were increased in number in all the treatments. Overall, our findings suggest that PeME treatment induces apoptotic features such as damaged and condensed nuclei in a dose-dependent manner.
Figure 3. Apoptotic features in P. elegans methanolic extract (PeME) treated glioblastoma, colon, and melanoma cancer cells. (a–c) showed control, 20 and 40 µg/ml treated U251-MG and (d–f) showed control, 20 and 40 µg/ml treated HCT116 incubated for 24 h. Changes in the morphology of the cancer cells were observed under fluorescence microscope (200x magnification).
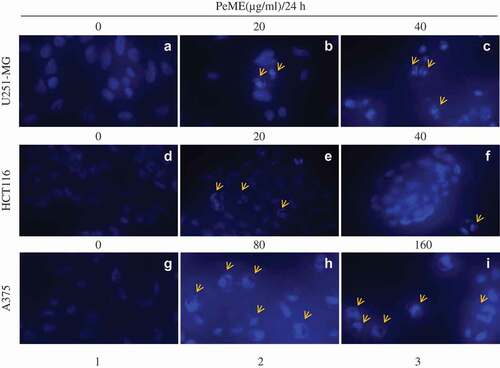
Annexin V staining for apoptosis detection
Induction of apoptosis is one of the most important markers of cytotoxic antitumor agents. It has been shown that some natural compounds including plants induce apoptotic pathways that are blocked in cancer cells [Citation3]. The apoptotic event following treatment of PeME on three cell lines was investigated. After treatment with concentrations of PeME for 24 h, cells were labeled with annexin V-FITC and PI and flow cytometry analysis was performed. These findings suggested that PeME treated U251-MG and HCT116 (20 and 40 µg/ml) cells and A375 (80 and 160 µg/ml) cells exhibited a change in nuclear morphology such as condensed and fragmented nuclei, and damaged and shrunk nuclear membrane as compared to control cells (data not shown). Apoptotic cell number was found to be significantly higher in a directly proportional dose-dependent manner in all three cell types following treatment. As shown in , apoptotic cell population was significantly increased in a dose-dependent manner in PeME treated cancer cells. U251-MG cells (Table A) were more sensitive to PeME treatment (, upper). These findings were already reaching 75% of apoptotic deaths at 20 µg/ml treated cells, compared to HCT116 (Table B: 18.2% death at 20 µg/ml) and A375 (Table C: 32.54% death at 40 µg/ml) cells. Early apoptosis occurred in the order of HCT116 (Table B: 37.5% death at 40 µg/ml), A375 (Table C: 20.62% death at 80 µg/ml), and U251-MG (Table A: 1.99% at 20 µg/ml). On the other hand, late apoptosis occurred in the order of U251-MG (Table A: 82.03% death at 40 µg/ml), HCT116 (Table B: 63.32% death at 80 µg/ml), and A375 (Table C: 54.76% death at 160 µg/ml). Early apoptosis occurred in the order of HCT116 (Table B: 37.5% death at 40 µg/ml), A375 (Table C: 20.62% death at 80 µg/ml), and U251-MG (Table A: 1.99% death at 20 µg/ml). On the other hand, late apoptosis occurred in the order of U251-MG (Table A: 82.03% death at 40 µg/ml), HCT116 (Table B: 63.32% death at 80 µg/ml), and A375 (Table C: 54.76% death at 160 µg/ml). These differences appear to be related to cancer cell type-specific effects.
Figure 4. Flow cytometry analysis of U251-MG, HCT116, and A375 cell lines after P. elegans methanolic extract (PeME) treatment for 24 h. All cells were labeled with annexin V-FITC and PI. U251-MG cells (a) treated at 0,10, 20, and 40 µg/ml concentration of PeME, HCT116 cells (b) were treated for 0, 20, 40, and 80 µg/ml. (b), A375 cells (c) were treated 0, 40, 80, and 160 µg/ml. FACs analysis was performed and analyzed. Data presented are representative of three experiments.
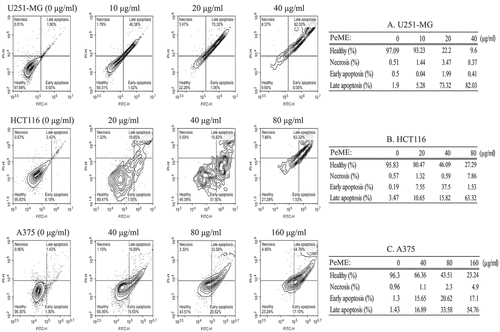
P. elegans methanolic extract (PeME)-induced cell death occurred via caspase-3/-9 and Mu-2-related death-inducing gene (MuD) activation
Next, the dynamics of key components involved in the extrinsic apoptosis pathway was examined. Various concentrations of PeME were treated in each cell for 24 h and then western blot was performed using specific target Abs. As shown in ), it was observed that PeME treatment induces proteolytic cleavage of caspase-3/-9 molecules at high concentration in each cell. Previously, it has been suggested that MuD may be a novel caspase-3 substrate in response to TRAIL stimulation in Jurkat T cells [Citation18,Citation25]. Thus, it was examined whether MuD expression in these cells could be modulated by PeME. As shown in ), constitutive expression of MuD protein was detected (lane 1). Interestingly, the amount of MuD protein began to decrease at low concentrations (lane 2‒3), reaching maximal reduction at high concentrations (lane 4). These results demonstrate that MuD and caspase-3/-9 activation were involved in PeME induced cell death.
Figure 5. Analysis of caspase and MuD activation in U251-MG, A375, and HCT116 cell lines. Cells were treated with various concentrations of PeME for 24 h; U251-MG were treated with 0, 10, 20 and 40 µg/ml (a), HCT116 cells were treated with 0, 10, 20, 40 and 80 µg/ml (b), A375 cell were treated with 0, 40, 80 and 160 µg/ml (c). The lysates were analyzed by immunoblotting using anti-caspase-3/-9 and MuD MAb. Actin was used for loading control.
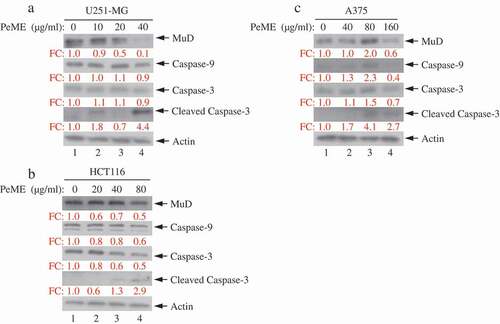
Measurement of mitochondria membrane potential (MMP)
Mitochondrial dysfunction has been shown to participate in the induction of apoptosis [Citation26]. Flow cytometric JC-1 staining was done to determine whether PeME treatment triggers apoptotic pathway by mitochondrial dysfunction. As shown in , we observed right-shifted green fluorescence profiles in a dose-dependent manner in PeME-treated cells was observed. Furthermore, through checking the MMP after PeME treatment, we observed a significant reduction in MMP in a dose-dependent manner. It is indicated that PeME triggers mitochondrial dysregulation, leading to apoptotic signaling pathway.
Figure 6. P. elegans methanolic extract (PeME) alleviated mitochondrial membrane potential (MMP). PeME induced a significant reduction in MMP in three cells. a-d showed control, 20, 40, and 80 µg/ml treated U251-MG; e-h showed control, 20, 40, and 80 µg/ml treated HCT116; i-l showed control, 40, 80, and 160 µg/ml treated A375 cells. Histogram profiles of JC-1 monomer (green fluorescence) were detected using flow cytometry. Peaks of treated concentration were shifted toward the right in the three treated cells compared to control, indicating a reduction of MMP.
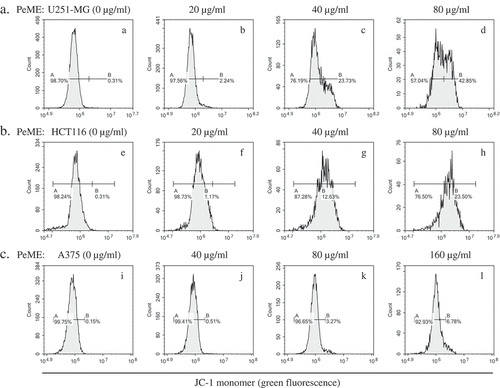
In spite of improved surgical techniques and advanced radio/chemotherapy, the survival rate and life expectancy of cancer patients have not been extended because of side effects and resistance to anti-cancer drugs. Therefore, the availability of natural products with higher effectiveness and lower side effects is desired [Citation4]. Medicinal herbs are important for cancer treatment as they possess multiple chemical compounds, which can be used to discover new active materials against cancer [Citation27]. P. elegans (Lour.) Desv. (taxon name) has an application in traditional medicinal in southeastern Asia. The roots and leaves are particularly used in folk medicine [Citation10,Citation12]. It was displayed in only one species of the Phyllodium genus that a substance isolated from P. pulchellum extracts inhibited the proliferation of the human hepatocyte carcinoma cell lines, in vitro [Citation28].
Recently Li et al reported for the first time that three triterpenoids (lupenone, lupeol, and botulin) are chemical constituents of P. elegans extracts [Citation22]. However, they did not provide additional information on the function of the triterpenoids in P. elegans extracts. Those triterpenoids have a wide range of pharmacological effects including anti-inflammatory, anti-diabetic and anti-cancer effects, and stimulation of various immune functions [Citation29]. For example, lupeol has been discovered as an active inhibitor of some cancers including prostate and skin cancers via downregulation of tumor necrosis factor (TNF)-α and VEGF-R2 [Citation30–Citation32]. Moreover, lupenone has been known to stimulate melanogenesis through the inhibition of Erk1/2 activation [Citation33].
Since then, there has been no report on the biological activity of P. elegans extracts. Therefore, this study to investigate whether PeME has anti-cancer activity against human astroglioma cells (U251-MG), human colorectal carcinoma cells (HCT116), and human malignant melanoma cells (A375) was undertaken. These findings revealed that PeME displayed cytotoxicity against all three cell lines. Clear apoptotic changes in these cells were observed after treatment. Cell migration and invasion rate of the cancer cells by in vitro scratch invasion assay were significantly reduced. JC-1 staining analysis by flow cytometry showed that PeME depolarized the MMP. Thus, these results enabled us to report that PeME has anti-tumoral potential against cancer cells by depolarization of MMP and activation of apoptotic signaling through the activation of caspase-3/-9 and MuD.
MuD, the novel anti-apoptotic protein is expressed and has a function in human glioblastoma cells [Citation18]. The function of this novel protein is inhibited upon TNF-related apoptosis-inducing ligand (TRAIL) stimulation in astroglioma cells [Citation18]. In this study, PeME induced activation of caspase-3/-9 and decreased MuD protein expression in a dose-dependent manner, suggesting that MuD is involved in PeME’s activities to suppress cell growth was observed. The novelty of this work is the very fact that this plant extract is seldom worked on and this is the first report on working out the anticancer activity and its effect on MuD. Thus, this naïve extract is not yet studied and it adds novel value toward natural product research. This research is expected to trigger off sensitization for application toward versatile anticancer natural remedies. More detailed future studies unraveling the exact mechanism and fundamental level of inhibitory mechanisms behind the anticancer activity of Phyllodium elegans extracts could result in meaningful and fruitful applications and natural remedies for cancer.
Conclusion
In conclusion, PeME anti-cancer activity against U251-MG, HCT116, and A375 cells in a time-/dose-dependent manner has been demonstrated. The U251-MG cells were most sensitive to PeME treatment compared to the other cells. The data also confirmed the apoptotic and anti-metastatic properties of PeME. This study, for the first time, confirmed that PeME triggered apoptotic cell death through significant reduction in MMP in cancer cells. This naïve extract is not yet studied, so it has novel value for natural product research.
Supplemental Material
Download MS Word (220.4 KB)Disclosure statement
No potential conflict of interest was reported by the authors.
Supplemental material
Supplemental data for this article can be accessed here.
References
- Arruebo M, Vilaboa N, Saez-Gutierrez B, et al. Assessment of the evolution of cancer treatment therapies. Cancers (Basel). 2011;3:3279–3330.
- Baskar R, Lee KA, Yeo R, et al. Cancer and radiation therapy: current advances and future directions. Int J Med Sci. 2012;9:193–199.
- Safarzadeh E, Sandoghchian Shotorbani S, Baradaran B. Herbal medicine as inducers of apoptosis in cancer treatment. Adv Pharm Bull. 2014;4:421–427.
- Kooti W, Servatyari K, Behzadifar M, et al. Effective medicinal plant in cancer treatment, part 2: review study. J Evid Based Complementary Altern Med. 2017;22:982–995.
- Madhuri S, Pandey G. Some anticancer medicinal plants of foreign origin. Curr Sci India. 2009;96:779–783.
- Houghton PJ. The role of plants in traditional medicine and current therapy. J Altern Complement Med. 1995;1:131–143.
- Chen Y, Xue G, Liu F, et al. Immunosuppressive effect of extracts from leaves of Fraxinus Mandshurica Rupr. Bioengineered. 2017;8:212–216.
- Taraphdar AK, Roy M, Bhattacharya RK. Natural products as inducers of apoptosis: implication for cancer therapy and prevention. Curr Sci India. 2001;80:1387–1396.
- Saisorn D, Chantaranothai P. Taxonomic studies on the genus Phyllodium Desv. (Leguminosae) in Thailand. Trop Nat History. 2015;15:23–40.
- Contu S Phyllodium elegans. The IUCN red list of threatened species 2012.
- Huang P, Hiroyoshi Ohashi H. PHYLLODIUM Desvaux. Flora China. 2010;10:265–266.
- Lopez Poveda L Desmodium elegans. The IUCN red list of threatened species. 2012.
- Administration ECoZBNTCH. Zhonghua bencao (Chinese Herbal Medicine). Shanghai: Shanghai Science and Technology Publishing House; 1996. p. 3120–3343.
- Ma X, Zheng C, Hu C, et al. The genus Desmodium (Fabaceae)-traditional uses in Chinese medicine, phytochemistry and pharmacology. J Ethnopharmacol. 2011;138:314–332.
- Thorburn A. Death receptor-induced cell killing. Cell Signal. 2004;16:139–144.
- Galluzzi L, Kepp O, Kroemer G. Mitochondria: master regulators of danger signalling. Nat Rev Mol Cell Biol. 2012;13:780–788.
- Lee MR, Shin JN, Moon AR, et al. A novel protein, MUDENG, induces cell death in cytotoxic T cells. Biochem Biophys Res Commun. 2008;370:504–508.
- Choi JH, Lim JB, Wickramanayake DD, et al. Characterization of MUDENG, a novel anti-apoptotic protein. Oncogenesis. 2016;5:e221.
- Choi JH, Min WK, Gopal J, et al. Silver nanoparticle-induced hormesis of astroglioma cells: A Mu-2-related death-inducing protein-orchestrated modus operandi. Int J Biol Macromol. 2018;117:1147–1156.
- Gopal J, Lee YM, Shin J, et al. The graviola impact on human astroglioma cells: functional significance of MUDENG. Rsc Adv. 2019;9:8935–8942.
- Wagley Y, Choi JH, Wickramanayake DD, et al. A monoclonal antibody against human MUDENG protein. Monoclon Antib Immunodiagn Immunother. 2013;32:277–282.
- Li YL, Fan X, Wang YL, et al. Studies on the triterpenoids constituents from Phyllodium elegans. Zhong Yao Cai. 2010;33:720–721.
- Rockenbach II, Jungfer E, Ritter C, et al. Characterization of flavan-3-ols in seeds of grape pomace by CE, HPLC-DAD-MSn and LC-ESI-FTICR-MS. Food Res Int. 2012;48:848–855.
- Steeg PS. Targeting metastasis. Nat Rev Cancer. 2016;16:201–218.
- Shin JN, Han JH, Kim JY, et al. MUDENG is cleaved by caspase-3 during TRAIL-induced cell death. Biochem Biophys Res Commun. 2013;435:234–238.
- Ly JD, Grubb DR, Lawen A. The mitochondrial membrane potential (deltapsi(m)) in apoptosis; an update. Apoptosis. 2003;8:115–128.
- Newman DJ, Cragg GM. Natural products as sources of new drugs over the 30 years from 1981 to 2010. J Nat Prod. 2012;75:311–335.
- Shen CC, Wang ST, Tsai SY, et al. Cinnamylphenols from Phyllodium pulchellum. J Nat Prod. 2005;68:791–793.
- Xu F, Huang X, Wu H, et al. Bene fi cial health effects of lupenone triterpene: a review. Biomed Pharmacother. 2018;103:198–203.
- Kangsamaksin T, Chaithongyot S, Wootthichairangsan C, et al. Lupeol and stigmasterol suppress tumor angiogenesis and inhibit cholangiocarcinoma growth in mice via downregulation of tumor necrosis factor-alpha. PLoS One. 2017;12:e0189628.
- Nigam N, Prasad S, Shukla Y. Preventive effects of lupeol on DMBA induced DNA alkylation damage in mouse skin. Food Chem Toxicol. 2007;45:2331–2335.
- Saleem M, Afaq F, Adhami VM, et al. Lupeol modulates NF-kappaB and PI3K/Akt pathways and inhibits skin cancer in CD-1 mice. Oncogene. 2004;23:5203–5214.
- Villareal MO, Han J, Matsuyama K, et al. Lupenone from Erica multiflora leaf extract stimulates melanogenesis in B16 murine melanoma cells through the inhibition of ERK1/2 activation. Planta Med. 2013;79:236–243.