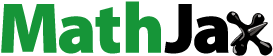
ABSTRACT
Rhizoremediation is one of the most accepted, cost-effective bioremediation techniques focusing on the application of rhizospheric microorganisms in combination with plants for the remediation of organic and inorganic pollutants from the contaminated sites. This work focuses on isolation and identification of metal resistant bacteria to grow on medium with the copper ion concentration of 1500 mg/L. The resistant isolate was identified as Pantoea dispersa by a 16S rRNA sequencing. The bioaccumulation of Cu(II) ions in plant is high at the concentration of Cu(II) ion is 125 mg/L in soil. In Sphaeranthus indicus the Cu(II) ion translocation factor has expanded with an expansion of grouping of Cu(II) ion in the soil and the most extreme TF factor was acquired at the centralization of Cu(II) ion is 150 mg/L in soil. Surface morphology of biochar was characterized by Scanning Electron Microscopy (SEM) analysis. The adsorption performance of biochar (Sphaeranthus indicus biomass) and mechanism for the removal of Cu(II) ion were investigated. This study resolves that pyrolysis is promising technology for the conversion of metal ion contaminated plant residues from phytoremediation into valuable products.
Introduction
The contamination of toxic heavy metals in the soil makes a severe risk to humans and environment on account of its non-biodegradability and poisonous quality [Citation1,Citation2]. The soil contamination by poisonous heavy metals has quickened significantly by the utilization of heavy metals, for example, chromium, cadmium, zinc, lead, mercury, copper and nickel [Citation3–Citation5]. These toxic heavy metals are directly introduced into the environment through the rapid development of different industries such as metal plating, fertilizer, battery, mining, paper, pesticides, electroplating, tannery, leather, iron and steel [Citation6–Citation8]. In this manner, remediation of toxic heavy metals is important to shield the earth from their lethal impacts. A few endeavors have been made to create manageable and environmental friendly advancements valuable to remove and expel dangerous heavy metals from water and soil [Citation9–Citation11].
These heavy metal contamination causes several health effects to humans such as may damage liver, lungs, kidney, nervous system, headache, anxiety and depression, digestive problems, autoimmune diseases, vomiting, muscle weakness [Citation12–Citation16]. Different treatment technologies have been used for the removal of toxic heavy metals such as ion exchange [Citation17], membrane separation [Citation10], reverse osmosis [Citation18], electro chemical treatment [Citation19], precipitation [Citation20], chelation [Citation21], electro dialysis [Citation22] and ultrafiltration [Citation23]. These ordinary advances utilized for the removal of toxic heavy metals from profluent wastewater have a few disadvantages, for example, high establishment cost, tedious running, costly upkeep, low productivity of execution at low metal concentration and issues with transfer of sludge. There is an important to stop the gathering of these natural poisons and expels them from the earth without influencing different assets [Citation24,Citation25]. In the substitute, organic approaches/bioremediation is considered as the best, eco-accommodating and minimal effort strategy for metal remediation without changing the physiochemical properties of the dirt.
The remediation procedures to beat the issues of environmental degradation ought to be permeated for its environmental effects. As of late, phytoremediation is viewed as a noticeable innovation for the expulsion of toxic contaminants. Phytoremediation is a pertinent strategy to a few recovering treatment, since it does not meddle with the biological system, it requires little manpower and accordingly is not extravagant contrasted with customary physicochemical techniques. Phytoremediation strategies could be applied for the recuperation of the modern destinations vigorously debased. Plant rhizosphere associations with contaminants, are the key factor in phytoremediation innovations. Plants take up the metals from the rhizosphere as pursues: Mobilization of heavy metals in soil and ensuing take-up by plant roots, translocation of the collected metals from roots to airborne tissues pursued by sequestration of the metals in plant tissues and resistance [Citation26–Citation31].
The achievement of phytoremediation is reliant on the capability of the plants to yield high biomass and withstand the metal pressure. Moreover, the metal bioavailability in rhizosphere soil is viewed as another basic factor that decides the productivity of metal translocation and phytostabilization process. Despite the fact that these changes increment the productivity of phytoextracton/phytostabilization, some concoction alterations are not just phytotoxic yet additionally dangerous to gainful soil microorganisms that assume significant job in plant development and advancement [Citation32–Citation34]. Bioremediation is characterized as the procedure whereby toxins naturally lessen under controlled conditions to a harmless state, which comprise of phytoremediation, biopile, windrows, bioreactor, land cultivating, bioventing, bioslurping, biosparging, penetrable responsive boundary, bioaugmentation, bioleaching and treating the soil. Among them, phytoremediation is the more dominant and broadly acknowledged system for metal remediation. A promising option in contrast to concoction changes could be the utilization of organism intervened forms, in which the microbial metabolites/forms in the rhizosphere influence plant metal take-up by changing the versatility and bioavailability [Citation35,Citation36].
When thinking about ways to deal with adjusts heavy metal mobilization, there are a few preferences to the utilization of favorable organisms as opposed to substance changes on the grounds that the microbial metabolites are biodegradable, less poisonous and it might be conceivable to create them in situ at rhizosphere soils [Citation37–Citation39]. Building pyrolysis innovation for feasible administration of contaminated biomass coming about because of phytoremediation and their use as the feedstock for synchronous generation of significant items requires examination of the biomass warm conduct. This examination meant to explore the impact of pyrolysis temperature (500–800°C) on the properties of polluted biomass resulted from phytoremediation and resulting pyrolytic products. Sphaeranthus indicus acquired after phytoremediation of Cu(II) ions smelter slags was utilized as a model biomass. The outcomes were additionally contrasted with an uncontaminated Sphaeranthus indicus biomass to decide the impact of heavy metals on the properties of the resultant pyrolytic products.
In this study, Sphaeranthus indicus plant has been chosen and the Cu(II) ion resistant bacteria—Pantoea dispersa was isolated from the tannery-effluent exposed area. This crop is being created in the zone adjoining the organizations where current effluents spoiled with significant metals is heading off to the field so the present assessment is grasped to think about the phytotoxic effects of Cu(II) ion on advancement parameters in Sphaeranthus indicus. With this foundation, the present investigation planned to recognize the phytoremediation capacity of Sphaeranthus indicus in Cu(II) ion tainted soil with the plant growth developing microorganism—Pantoea dispersa. The aim of the present study was (i) to identify the potential of Sphaeranthus indicus plant with the integrated approach for the enhanced phytoremediation of Cu(II) ions from the soil (ii) to isolate the Cu(II) ion-resistant bacteria and identifying the isolated bacteria by 16s rDNA sequencing and phylogenetic tree analysis (iii) to analyze the metal degrading ability of Pantoea dispersa by biodegradability assay (vi) to investigate the growth development of Sphaeranthus indicus plant coupled with Pantoea dispersa in the Cu(II) ion-contaminated soil (v) to estimate the translocation and bioaccumulation factor of Sphaeranthus indicus plant (vi) to characterize the Sphaeranthus indicus biomass proliferate with Cu(II) ions after phytoremediation (vii) to estimate the biosorptive applications of biochar (Sphaeranthus indicus biomass) for the removal of methylene orange dye from aqueous solution.
Materials and methods
Copper resistant bacteria
The soil samples were collected from the Vaniyambadi region in Vellore district, Tamil Nadu, India. This region has been exposed to the large number of toxic tannery industrial wastes. The soil samples were transferred to the laboratory by using sterile glass bottles and allowed for serial dilution (10−1 – 10−9) by using the distilled water. The dilutions of 10−4, 10−5 and 10−6 were plated (streak) by using Nutrient Agar (NA) for the isolation of heavy metal resistant bacteria. After plating they were allowed to incubate at 30°C for 48 h. After the incubation different morphological colonies were absorbed in the plate, from that specific colony were isolated and inoculated into another NA plate for the isolation of specific species from the group of colonies, this process will occurs until the homogenous growth phase is obtained. The isolated bacterial samples were allowed for sequencing study and phylogenetic analysis, which was performed in Yaazh Xenomics, Chennai.
Sequencing
For the sequencing study of isolated bacterial species, the DNA extraction was done by using the standard procedure. The concentration of DNA was measured by running aliquots on 1% agarose gel. Using PCR (Polymerase Chain Reaction) specific resistant gene was amplified after isolation process. PCR was executed using two different primers (Forward and Reverse primer) both are having 20 bp named as 25F and 1492R and their sequences are; 5ʹ AGAGTTTGATCMTGGCTCAG 3ʹ and 5ʹ TACGGYTACCTTGTTACGACTT 3ʹ respectively. 5µL of isolated DNA was mixed with 25 µl of PCR reaction mixture containing the primers, Taq Master Mix and deionized water. Using five different thermal conditions the reaction was achieved, the thermal conditions are; Initial Denaturation (94 °C, 3 min), Denaturation (94°C, 30 s), annealing (60°C, 30 s), extension (72°C, 1 min) and Final extension (72 °C, 10 min). Finally the mixture were hold at 4°C, using single-pass sequencing the developed PCR results were molecularly characterized (sequenced) and an amplified outcomes were subjected to electrophoresis at Yaazh Xenomics, Coimbatore, Tamil Nadu, India.
Phylogenetic analysis
The phylogenetic examination with other firmly related grouping was done trailed by various succession arrangements utilizing the program MUSCLE 3.7. The precise Phylogenetic investigation was performed utilizing the program PhyML 3.0.
Biodegradability assay
The isolated bacteria, Pantoea dispersa, was sub cultured in LB broth medium and kept in shaking incubator for the period of 24 h at the temperature of 37 °C and pH of 7.0. Biodegradability assay was performed by adding 100 mL of different concentration of Cu(II) ion into the 250 mL of Erlenmeyer flask containing LB broth medium. The flasks were kept in temperature controlled shaking incubator at the temperature of 37°C for about 24 h. After the mentioned time period, the culture was taken and centrifuged at 5000 rpm for the period of 15 min. Supernatant was isolated and blended in with equivalent amount of concentrated nitric acid followed by heating at the temperature of 100°C until introductory volume of supernatant was acquired. At that point the supernatant is filtered utilizing Whatman filter paper and the concentration of Cu(II) ion in the solution was examined utilizing Atomic Adsorption Spectroscopy (AAS).
Plant growth analysis and percentage removal of Cu(II) ions
The sieved soil was filled in the six distinct pots each as 500 g and washed with refined water. The various focuses (25–150 mg/L) of arranged CuSO4 solution were included into the five pots separately aside from the control. The bacterial detach was immunized in the Luria Broth (LB) and kept in incubator at 30°C for 24 h. The cell suspension were changed in accordance with Optical Density at 600 nm (OD600) between 1.4 and 2.0, after that the three sound, comparative size and shape seeds of Sphaeranthus indicus seeds per pot were planted and permitted to develop. Subsequent to planting the seeds 20 mL of brooded and upgraded bacterial culture Pantoea dispersa were vaccinated into the each pot. Following 45, 55 and 65 days of development the plants were collected and their wet and dry loads are noticed, the roots, shoots and leaves of the plant were ground.
The length and weight of the shoot (stem and leaf) and roots were evaluated. The plant materials were kept in oven at 70°C for 24 h and thereafter dried materials were ground using mortar and pestle. At that point the dried material was permitted to corrosive absorption by the accompanying method. The grounded plant matter was handled using 5 mL of destructive mixes (60% HCl and 85% HNO3) at medium-term. After the endorsed time interim, the examples were viewed as cooling and 2.5 mL of 20% HCl was added to the cooled tests, mixed inside and out and warmed at 80 °C for 30 min. The warmed examples were cooled and separated through Whatman 42 channel paper. The concentration of Cu(II) ion in the dirt was assessed when the phytoremediation and moreover in the plant materials (shoot and roots) using Atomic Absorption Spectroscopy (AAS).
Translocation factor and bioaccumulation factor
Translocation factor (TF) is the extent of centralization of metal in shoot to convergence of metal in root. It is an ability of a plant to translocate the metal from roots through shoots and leaves of a plant which is mindful of Phytoextraction, in like manner called as shoot-root remainder. Cu(II) ion trans-location from root to shoot was evaluated by
Where, Cshoot is concentration of metal in shoot of the plant, Croot is concentration of metals in root of the plant.
Bioaccumulation factor (BAF) is the extent of collection of metal in shoot to intermingling of metal in soil. Bioaccumulation is the technique where the poisonous metals are accumulated in different pieces of the plants like shoot and leaves as non-dangerous. Bioaccumulation of Cu(II) particle in plant was determined by
Where, Cshoot is concentration of metal in plant shoot, Csoil is concentration of metal in soil.
Methyl orange (MO) dye solution
In the present study, MO dye solution was used as adsorbate, which was procured from E. Merck, India. A stock solution of 1000 mg/L of MO dye solution was prepared by dissolving required quantity of methylene orange in 1000 mL of distilled water. Then the stock solution was diluted to required concentration (25 to 150 mg/L) using deionized water. The initial and final concentration of MO dye in the solution was analyzed using UV visible spectrophotometer. The pH of the solution was adjusted to neutral using 0.1 N NaOH or 0.1 N HCl.
Preparation of biosorbent—biochar
The Cu(II) ion contaminated Sphaeranthus indicus plant residues (SIPR) has been used as feedstock for the pyrolysis study. Sphaeranthus indicus stem were collected and cut into small pieces and washed using pure water to remove the dust particles. The collected stem particles were dried in hot air oven at the temperature of 80°C for about 1 h. The dried stem particles were grinded into fine powder. The grinded fine particles were pyrolyzed at 500, 600, 700, 800°C using a vacuum tube sintering furnace (Manish Scientific Instruments Company, Chennai, India) to produce biochar. The muffle furnace was kept on warming up at the warming pace of 10°C/min until the prescribed temperature was reached. Afterward, the obtained temperature was kept up for 2 h. All the pyrolysis forms were completed with presence of N2. After pyrolysis, the materials were cooled to air temperature and put away in a desiccator before use.
Characterization study
The newly synthesized biochar Sphaeranthus indicus plant residue at the pyrolysis temperature of 700°C (SIPR-700) has been utilized as adsorbent material for the removal of methylene orange (MO) dye from aqueous solution. The surface morphology of the adsorbent material plays an imperative role for the determination of adsorption characteristics. The surface morphology of newly synthesized pyrolytic biomass residues was characterized by Scanning Electron Microscopy (SEM) analysis.
Batch adsorption study
Adsorption of methylene orange (MO) dye was carried out by using the biosorbent material of SIPR-700. Batch experimental study for the removal of MO dye onto SIPR-700 was examined at different factor influencing parameters such as MO dye concentration (25 mg/L to 150 mg/L), contact time (10 to 90 min), pH (3.0 to 10.0), SIPR-700 dosage (0.5 to 2.5 g) and temperature (30 to 60 °C). MO dye adsorption experiments was performed at 100 mL of desired concentration of MO dye solution, pH of 3.0, temperature at 30 °C for about different time interval. After the prescribed time interval, flasks were withdrawn from the shaker and the MO dye solution was filtered using Whatman filter paper. The MO dye concentration in the supernatant was measured by using UV visible spectrophotometer. Every one of the analyses was done in triplicates and the reports were furnished with average of these qualities. The percentage removal of MO dye was determined utilizing the accompanying condition:
Where Ci is the initial concentration of MO dye and Cf is the final concentration of MO dye.
Results and discussion
Copper resistant bacteria isolation
The resistant bacteria were observed after 24–36 h of incubation. 27 isolates were obtained by serial dilution in the plates 10−2 and 10−3 with copper ion concentration of 100 mg/L. On further screening, these 27 isolates were grown on nutrient medium plates with high copper ion concentration of 1500 mg/L. Among the 27 isolates, one isolate was grown and shown high resistance at high copper ion concentration. The isolated strain was selected and sub cultured by streak plate method. The plate was incubated at 37°C for 24 h.
Sequencing
DNA was isolated and the PCR products were purified. Sanger method was used for sequencing the DNA strands. The isolate was found to be Pantoea dispersa. represents the 16s rRNA sequence of the isolate. MEGA 6.0 software was used for constructing phylogenetic tree. The 16s rDNA sequence of the bacterial isolate was submitted to NCBI Gene bank. The complete genome sequence of sequenced gene is available in NCBI GenBank under the accession number MH985357.1.
Phylogenetic tree construction
Phylogenetic tree was developed utilizing MEGA 6.0 programming for inducing the phylogeny or transformative relationship among other species and to develop branching tree dependent on their similitudes and contrasts in their characters. represents the phylogenetic tree of the resistant isolate. This phylogenetic tree helps in determining the similarity with other species and finding out their similarity and differences in the characters.
Biodegradability assay
The sample was incubated and centrifuged followed by the supernatant of isolate was mixed with concentrated HNO3. The sample mixture was warmed until essential volume was gotten. At that point the amount of desired Cu(II) ion in the treated supernatant estimated utilizing AAS and the results showed a decline in the Cu(II) ion concentration contrasted with initial Cu(II) ion concentration. The strain can lessen up to the Cu(II) ion concentration of 2100 mg/L. obviously portrays the metal debasing capacity of Pantoea dispersa. The bacterium doesn’t display any metal decrease after 2100 mg/L.
Plant growth analysis and phytoremediation study
The shoot and root weight were estimated when drying also, the outcomes are appeared in . The relationship between the Cu(II) ion concentration in soil and shoot weight and root weight was shown in , separately. It was indicated that, shoot weight and root weight (fresh and dry) has bit by bit diminished with an expansion of initial Cu(II) ion concentration from 25 to 150 mg/L. The purpose behind this conduct, Cu was intruding with a factor straight forwardly related with cell prolongation suggesting the specific commitment of synthetic substances in the divider and ATPase related with the plasmalemma.
Table 1. Shoot and root – fresh and dry weight of Sphaeranthus indicus in soil associated with Pantoea dispersa amended with Cu(II) ion concentration
Figure 4. (a) Relation between the shoot weight (Sphaeranthus indicus) and Cu(II) ion concentration in soil (b) Relation between the root weight (Sphaeranthus indicus) and Cu(II) ion concentration in soil (c) Relation between translocation factor and Cu(II) ion concentration in soil (d) Relation between bioaccumulation factor and Cu(II) ion concentration in soil
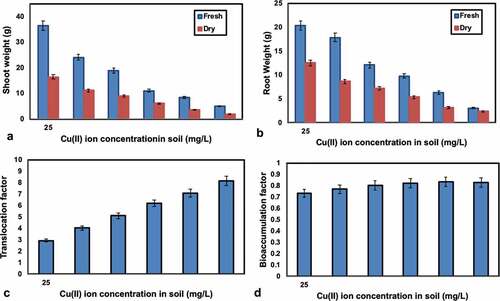
Phytoremediation of Cu(II) ion from the metal rich soil was done and the results have showed up in the . From the arrangement report, the results shows that Cu(II) ion in soil were about decreased. The Cu(II) ions were translocated from the dirt to the root and shoot of plant; here they are gathering in the vacuoles. In the vacuoles, they are put away in non-dangerous structure because of detoxification instrument in the vacuoles. From the , the centralization of Cu(II) ion was found in the root and shoot, in which the grouping of Cu(II) ion is more in shoot of the plant differentiated and root. In perspective on the Cu(II) ion concentration in soil they can be assemble in the shoot and root, when the centralization of Cu(II) ion constructs the collection of Cu(II) ion similarly augments straightforwardly in the shoot and establishment of the plant.
Table 2. Cu(II) ion concentration in soil sample and shoot, root (on a dry weight premise) of Sphaeranthus indicus associated with Pantoea dispersa.
Translocation factor and bioaccumulation factor
The translocation factor (TF) chooses the practicality of Cu(II) ion translocation from root to shoot and the bioaccumulation factor (BAF) arranges the plants as aggregators, hyper accumulators and excluders subject to the congregation of Cu(II) ion. , shows that Translocation and Bioaccumulation factor of Cu(II) ions of Sphaeranthus indicus and the results have showed up in . From ,, it was seen that Cu(II) ions are successfully translocated from the root to shoot of the plant. The bioaccumulation of Cu(II) ions in plant is high at the concentration of Cu(II) ion is 125 mg/L in soil. In Sphaeranthus indicus the Cu(II) ion translocation factor has expanded with an expansion of grouping of Cu(II) ion in the soil and the most extreme TF factor was acquired at the centralization of Cu(II) ion is 150 mg/L in soil. On the off chance that TF > 1, the translocation of metals from the root to shoot is successful. From the organization report, it was indicated that TF and BAF regard more conspicuous than 1, by then it is a great gatherer plant. Fittingly, in the present examination, Sphaeranthus indicus goes about as a convincing gatherer on account of its higher TF and its BAF being more unmistakable than 1. Higher gathering of heavy metals in plants shows cut down centralizations of metal, both in the water and the leftovers of the wetland. Thusly, Sphaeranthus indicus goes about as a progressively conspicuous phytoremediator of Cu(II) ions in light of its higher translocation limit.
Table 3. Translocation and Bioaccumulation factor of Cu(II) ions of Sphaeranthus indicus associated with Pantoea dispersa.
Characterization study
The surface structure and morphology of SIPR-700 was characterized by Scanning Electron Microscopy at different magnifications of 1300 x (100 µm), 2500 x (50 µm), 5000 x (20 µm – 11.4 mm) and 5000 x (20 µm – 11.2 mm) and the results were shown in . SEM analysis can be used to identify the pores present in the surface of the adsorbent material. shows that SIPR-700 has enormous cavities, more pores and non-permeable solid material as for its surface smoothness. Enormous cavities were formed on the surface of the SIPR-700 due to pyrolysis process. In addition to that, the water molecules present on the pores of the adsorbent material has also been removed by undergoing pyrolysis process. These characteristics indicated that SIPR-700 has more adequate qualities for the removal of MO dye from aqueous solution.
Batch adsorption study
Influence of MO dye concentration
The initial concentration of MO dye assumes a significant job in the adsorption procedure for deciding the adsorption limit of the adsorbent material. In the present investigation, the impact of initial MO dye concentration was examined by varying the MO dye concentration from 25 to 150 mg/L at the pH of 7.0 for 60 min and the biochar dose of 0.25 g at the temperature of 30°C. ) shows the impact of initial MO dye concentration on the adsorption of MO dye onto SIPR-700. It was seen from ), percentage removal of MO dye was diminished with expanding the MO dye concentration from 25 to 150 mg/L. This might be expected to, at lower concentration, MO dye in the fluid arrangement would conceivably bind with the surface dynamic locales of the adsorbent material and along these lines make conceivable practically complete adsorption. In the resistance of, at higher concentration, MO dye are left unadsorbed in the fluid arrangement which may be perhaps because of less accessibility of dynamic locales for example immersion of dynamic locales.
Figure 6. (a) Effect of MO dye concentration for the removal of MO dye onto biochar (b). Effect of pH for the removal of MO dye onto biochar (c). Effect of contact time for the removal of Cu(II) ion onto biochar (d). Effect of biochar dosage for the removal of MO dye onto biochar (e). Effect of temperature for the removal of MO dye onto biochar
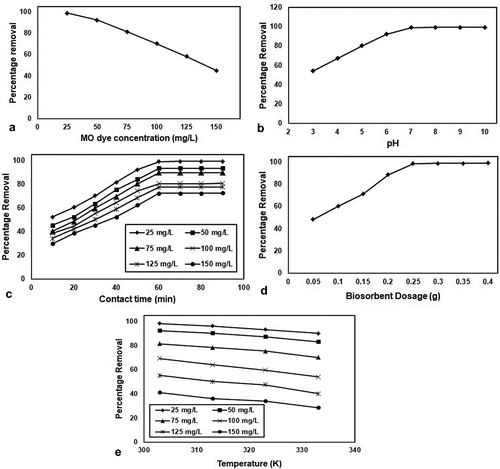
Influence of pH
) shows the impact of pH on the adsorption of MO dye onto SIPR-700 with a wide scope of pH from 3.0 to 9.0. The pH of the MO dye solution was balanced by utilizing 0.1 N HCl and 0.1 N NaOH and estimated by utilizing the pH meter. The adsorption procedure was completed with 100 mL of MO dye concentration (25 mg/L) with various pH (3.0 to 9.0) and biochar measurements (0.25 g) with a contact time of 60 min. As the outcomes from the ), the take-up of MO dye was seen as expanding with increment in the arrangement pH from 3.0 to 7.0. By and large, the MO dye displays the emphatically charged particles when broken down in water. In the pH 3.0 to 7.0, the adsorption of MO dye was expanded; the purpose behind this conduct may be because of essence of an acidic medium, the surface of the adsorbent may get positive charge which doesn’t draw in the decidedly charged MO dye in the solution. As the solution pH increments, the adsorbent surface accomplishes the negative charge which improves the adsorption limit of SIPR-700 due to the arrangement of electrostatic interaction between the adversely charged SIPR-700 and the decidedly charged MO dye. However, further expanding the pH to 9.0, result no change in the adsorption of MO dye on SIPR-700. This conduct can be clarified by at basic medium (pH 7.0) repugnance happens between the adsorbent material (SIPR-700) and the base mechanism of the MO dye solution, for the explanation that, at soluble pH the MO dye in the solution likewise goes to negative charge. Hence, the ideal pH for the evacuation of MO dye onto SIPR-700 was seen as 7.0.
Influence of contact time
To explore the contact time for the adsorption of MO dye onto SIPR-700 was performed at various contact times from 10 to 90 min for an underlying MO dye concentration of 25 mg/L at pH of 7.0 and the biochar dose of 0.25 g at the temperature of 30°C. ) shows the impact of contact time on the adsorption of MO dye onto the SIPR-700. ) shows that, removal of MO dye was expanded with an expansion of contact time from 10 to 60 min and accomplishes the equilibrium condition at 60 min. The plausible purpose behind fast adsorption at starting stage might be because of the accessibility of increasingly number of dynamic destinations in the SIPR-700. At that point, adsorption of MO dye kept a proceeded expanding inclination, at a more slow rate pursued by a more extended balance timespan from 10 to 60 min. Further increment in contact time from 60 to 90 min, the adsorption rate turned out to be slower furthermore, no further significant adsorption has been noted.
Influence of biochar dosage
The impact of biochar dosage on the adsorption of MO dye was considered by varying the biochar dose from 0.05 to 0.4 g in 100 mg/L of 25 mg/L centralization of MO color arrangement at pH = 7.0 for 60 min at 30°C. ) shows the impact of biochar dosage on the adsorption of MO dye onto SIPR-700. It could be seen from ), the percentage removal of MO dye was step by step expanded with an expanding the biochar measurements—SIPR 700 from 0.05 to 0.25 g. The purpose behind this conduct is because of the accessibility of increasingly number of surface dynamic locales and bigger surface zone. Further expanding the adsorbent portion from 0.25 to 0.4 g, the percentage removal was arrived at consistent. This may be potentially because of the accomplishment of balance between the adsorbate and the adsorbent material.
Influence of temperature
) show the percentage removal of MO dye onto SIPR-700. Adsorption study was performed by adding 0.25 g of SIPR-700 in 100 mL of 25 mg/L concentration of MO dye solution at the pH of 7.0. ) explored that the percentage removal of MO dye was decreased as the temperature increased from 30 to 60°C. The reason for this behavior, weak adsorptive force between the MO dye and the adsorption sites on the surface of the SIPR-700 and number of available active sites were less at higher temperature. Therefore, fewer amounts of MO dye molecules were interacting with the adsorption sites at SIPR-700. This factor could significantly affect the adsorption process by decreasing the adsorption capacity of SIPR-700. Finally, the results suggest that adsorption of MO dye on SIPR-700 was favored at lower temperature (30°C) and present adsorption process is exothermic in nature.
Conclusion
In this investigation, the present research work concentrating on the isolation and recognizable proof of copper resistant bacteria which has potential points of interest in degrading copper present in industrial wastewater. The isolated bacteria were identified as Pantoea dispersa by sequencing study. The physicochemical properties of Sphaeranthus indicus biomass flourish with Cu(II) ions after phytoremediation and its pyrolytic subsidiaries (biochar) were explored. Plant residues acquired after phytoremediation contain a lot of Cu(II) ion. Plant residues pyrolysis at 700°C gave best conditions to the further treatment and the reutilization of plant deposits acquired after phytoremediation. This examination exhibited that pyrolysis is a successful innovation for changing over the metal(loid)-rich biomass into important items. In expansion, the pyrolysis items (SIPR-700) produced from plant residues got after phytoremediation showed incredible execution in MO dye adsorption. Consequently, pyrolysis is an attainable methodology for the transfer of plant residues got after phytoremediation also; their pyrolysis products can fill in as potential elective sorbents for the cleaning of toxic contaminants from wastewater.
Highlights
Rhizoremediation of Cu(II) ion contaminated soils using Pantoea dispersa strain with Sphaeranthus indicus plant has studied.
The bioaccumulation and translocation of Cu(II) ions in Sphaeranthus indicus plant was estimated.
Plant residues biochar was prepared by pyrolysis process and surface morphology of biochar was characterized.
Biosorption of methylene orange dye onto biochar has been investigated.
Supplemental Material
Download ()Disclosure statement
No potential conflict of interest was reported by the authors.
Supplementary material
Supplemental data for this article can be accessed here.
Additional information
Notes on contributors
P. R. Yaashikaa
P. R. Yaashikaa, Senior Research Fellow, Department of Chemical Engineering, SSN College of Engineering, has 3 years of research experience in the area of Environmental Biotechnology. She has graduated from Vivekananda College of Engineering for Women, Namakkal with First Rank Gold Medalist, Anna University, in Biotechnology during the year 2010–2014. She has obtained her master’s degree in biotechnology with first rank from Bannari Amman Institute of Technology, Coimbatore during the year 2014–2016 and doing her PhD degree (Thesis Submitted) from SSN College of Engineering, Anna University, and Chennai under the guidance of Dr P Senthil Kumar. She has 12 publications in International and National Journals and 15+ book chapters published in Elsevier, Springer, CRC Press, etc. She has received best paper awards in various international and national conferences. She is also a member of International Association of Engineers (IAENG) and European Federation of Biotechnology (EFB).
P. Senthil Kumar
Dr. P. Senthil Kumar, Associate Professor in the Department of Chemical Engineering, was born on 8 May 1982 in Madurai, Tamilnadu, India. He has 14 years of teaching and research experience and 1 year of industrial experience. He is a recognized Anna University Research Supervisor to guide Ph.D./M.S. candidates. Under his guidance, eight PhD scholars have completed and nine PhD scholars are currently doing their active research in the area of Waste Water Treatment and Alternative Fuels. He published more than 185 research papers in highly reputed International Journals and 80 research papers in National/International Conferences. He also published more than 80 book chapters, publishers: Elsevier, Springer, CRC Press, etc. He organized/attended more than 100 externally/internally funded conferences/workshops/seminars at national and international level and also he had given more than 50 invited research talks in various academic/research institutions in India. An author’s h index of his publication is 33, i10 index of 90 along with google citations of 4686.
Sunita Varjani
Dr. Sunita Varjani is Scientific Officer at Gujarat Pollution Control Board, Gandhinagar, Gujarat, India. Her major areas of research are Industrial and Environmental Microbiology/Biotechnology. She has worked as visiting scientist at EPFL, Lausanne, Switzerland. Dr. Varjani has authored more than 165 publications, including research and review papers, books, book chapters and conference communications. She has won several awards, including Young Scientist Awards from Biotech Research Society, India (2018), Microbiologist’s Society India (2018-19), Association of Microbiologists of India (2018), International Society for Energy, Environment and Sustainability (2018) and AFRO-ASIAN Congress on Microbes for Human and Environmental Health, New Delhi (2014); Highly Cited and Highly downloaded papers, Bioresource Technology, Elsevier; Top Reviewer Award - 2018, Bioresource Technology, Elsevier; Top Reviewer Award - 2017, Bioresource Technology, Elsevier and Best Paper Awards in national and international conferences in 2008, 2012, 2013, 2018 and 2019. She is member of editorial board of Journal of Energy and Environmental Sustainability and has served as guest editor of special issues of Bioresource Technology, Environmental Science and Pollution Research, ASCE- Journal of Environmental Engineering, Bioengineered, Industrial Crops and Products Journal, Energy and Environment, Environmental Science and Pollution Research and others. She is Management Council Member of the BRSI (www.brsi.in).
A. Saravanan
Dr. A. Saravanan, Assistant Professor in the Department of Biotechnology, Rajalakshmi Engineering College, Tamilnadu, India. He has completed his Ph.D. with the specialization of Environmental Biotechnology (Surface modified agro waste biomass with enhanced adsorption properties for heavy metal removal: An eco-friendly approach) at SSN College of Engineering, under Faculty of Technology, Anna University, and Chennai. His Bachelor degree – B.Tech (Biotechnology) in Sri Nandhanam College of Engineering and Technology, Vellore and Master degree – M.Tech (Industrial Biotechnology) in Annamalai University, Chidambaram. He has experience in Wastewater treatment technology, Adsorption, Biopesticide removal, antimicrobial studies, metallic nanoparticle synthesis, anaerobic wastewater treatment, and Phytoremediation. He has 5 years of teaching experience and 03 year of research experience. He has published 48 research papers in highly reputed International Journals. He also published more than 25+ book chapters, publishers: Elsevier, Springer, CRC Press, etc. He has attended more than 20 conferences/workshops/seminars at national and international level. He has received IEI – Young Engineer Award in August 2019 from Institute of Engineers, India, Best paper awards in various conferences and Best Faculty Award – 2014 for the outstanding performance for the academic years 2013 - 2014. He is also a member of International Association of Engineers (IAENG) and European Federation of Biotechnology (EFB).
References
- Babu AG, Kim J-D, Oh B-T. Enhancement of heavy metal phytoremediation by Alnus firma with endophytic Bacillus thuringiensis GDB-1. J Hazard Mater. 2013;250–251:477–483.
- Liu C, Wang Q, Jia F, et al. Adsorption of heavy metals on molybdenum disulfide in water: a critical review. J Mol Liq. 2019;292:111390.
- Ullah A, Heng S, Munis MFH, et al. Phytoremediation of heavy metals assisted by plant growth promoting (PGP) bacteria: a review. Environ Exp Bot. 2015;117:28–40.
- Ali H, Khan E, Sajad MA. Phytoremediation of heavy metals—Concepts and applications. Chemosphere. 2013;91:869–881.
- Mahar A, Wang P, Ali A, et al. Challenges and opportunities in the phytoremediation of heavy metals contaminated soils: a review. Ecotox Environ Safe. 2016;126:111–121.
- Pandey VC. Phytoremediation of heavy metals from fly ash pond by Azolla caroliniana. Ecotox Environ Safe. 2012;82:8–12.
- Rungwa S, Arpa G, Sakulas H, et al. Phytoremediation – an eco-friendly and sustainable method of heavy metal removal from closed mine environments in Papua new Guinea. Procedia Earth Planet Sci. 2013;6:269–277.
- Nejadshafiee V, Islami MR. Adsorption capacity of heavy metal ions using sultone-modified magnetic activated carbon as a bio-adsorbent. Mater Sci Eng C. 2019;101:42–52.
- Almomani F, Bhosale R, Khraisheh M, et al. Heavy metal ions removal from industrial wastewater using magnetic nanoparticles (MNP). Appl Surf Sci. 2020;506:144924.
- Abdullah N, Yusof N, Lau WJ, et al. Recent trends of heavy metal removal from water/wastewater by membrane technologies. J Ind Eng Chem. 2019;76:17–38.
- Joseph L, Jun B-M, Flora JRV, et al. Removal of heavy metals from water sources in the developing world using low-cost materials: a review. Chemosphere. 2019;229:142–159.
- Saravanan A, Kumar PS, Yashwanthraj M. Sequestration of toxic Cr(VI) ions from industrial wastewater using waste biomass: a review. Desal Water Treat. 2017;68:245–266.
- Carolin CF, Kumar PS, Saravanan A, et al. Efficient techniques for the removal of toxic heavy metals from aquatic environment: a review. J Environ Chem Eng. 2017;5:2782–2799.
- Jeevanantham S, Saravanan A, Hemavathy MV, et al. Removal of toxic pollutants from water environment by phytoremediation: a survey on application and future prospects. Environ Technol Innov. 2019;13:264–276.
- Liu X, Germaine KJ, Ryan D, et al. Genetically modified Pseudomonas biosensing biodegraders to detect PCB and chlorobenzoate bioavailability and biodegradation in contaminated soils. Bioeng Bugs. 2019;10:33–42.
- Sivasubramaniam D, Franks AE. Bioengineering microbial communities: their potential to help, hinder and disgust. Bioengineered. 2016;7:137–144.
- Hosseini SM, Alibakhshi H, Jashni E, et al. A novel layer-by-layer heterogeneous cation exchange membrane for heavy metal ions removal from water. J Hazard Mater. 2020;381:120884.
- Anis SF, Hashaikeh R, Hilal N. Reverse osmosis pretreatment technologies and future trends: a comprehensive review. Desalination. 2019;452:159–195.
- Tran T-K, Chiu K-F, Lin C-Y, et al. Electrochemical treatment of wastewater: selectivity of the heavy metals removal process. Int J Hydrogen Energ. 2017;42:27741–27748.
- Chen Q, Yao Y, Li X, et al. Comparison of heavy metal removals from aqueous solutions by chemical precipitation and characteristics of precipitates. J Water Process Eng. 2018;26:289–300.
- Kim -J-J, Kim Y-S, Kumar V. Heavy metal toxicity: an update of chelating therapeutic strategies. J Trace Elem Med Biol. 2019;54:226–231.
- Smara A, Delimi R, Chainet E, et al. Removal of heavy metals from diluted mixtures by a hybrid ion-exchange/electrodialysis process. Sep Purif Technol. 2007;57:103–110.
- Huang J, Yuan F, Zeng G, et al. Influence of pH on heavy metal speciation and removal from wastewater using micellar-enhanced ultrafiltration. Chemosphere. 2017;173:199–206.
- Awad AM, Shaikh SMR, Jalab R, et al. Adsorption of organic pollutants by natural and modified clays: a comprehensive review. Sep Purif Technol. 2019;228:115719.
- Rostami S, Azhdarpoor A. The application of plant growth regulators to improve phytoremediation of contaminated soils: a review. Chemosphere. 2019;220:818–827.
- Cristaldi A, Conti GO, Jho EH, et al. Phytoremediation of contaminated soils by heavy metals and PAHs. A brief review. Environ Technol Innov. 2017;8:309–326.
- Ashraf S, Ali Q, Zahir ZA, et al. Phytoremediation: environmentally sustainable way for reclamation of heavy metal polluted soils. Ecotox Environ Safe. 2019;174:714–727.
- Egamberdieva D, Wirth S, Li L, et al. Microbial cooperation in the rhizosphere improves liquorice growth under salt stress. Bioengineered. 2017;8:433–498.
- Xu Z, Lei Y, Patel J. Bioremediation of soluble heavy metals with recombinant Caulobacter crescentus. Bioeng Bugs. 2010;1:207–212.
- Pulford ID, Watson C. Phytoremediation of heavy metal-contaminated land by trees—a review. Environ Int. 2003;29:529–540.
- Souza EC, Vessoni-Penna TC, de Souza Oliveira RP. Biosurfactant-enhanced hydrocarbon bioremediation: an overview. Int Biodeterior Biodegrad. 2014;89:88–94.
- Rajkumar M, Sandhya S, Prasad MNV, et al. Perspectives of plant-associated microbes in heavy metal phytoremediation. Biotechnol Adv. 2012;30:1562–1574.
- Arwidsson Z, Johansson E, von Kronhelm T, et al. Remediation of metal contaminated soil by organic metabolites from fungi—production of organic acids. Water Air Soil Pollut. 2010;205:215–226.
- Raskin I, Smith RD, Salt DE. Phytoremediation of metals: using plants to remove pollutants from the environment. Curr Opin Biotechnol. 1997;8:221–226.
- Sarma H, Nava AR, Prasad MNV. Mechanistic understanding and future prospect of microbe-enhanced phytoremediation of polycyclic aromatic hydrocarbons in soil. Environ Technol Innov. 2019;13:318–330.
- Dimkpa CO, Merten D, Svatos A, et al. Metal-induced oxidative stress impacting plant growth in contaminated soil is alleviated by microbial siderophores. Soil Biol Biochem. 2009;41:154–162.
- Khan AG. Role of soil microbes in the rhizospheres of plants growing on trace metal contaminated soils in phytoremediation. J Trace Elem Med Biol. 2005;18:355–364.
- Zhang X, Su C, Liu X, et al. Effect of plant-growth-promoting rhizobacteria on phytoremediation efficiency of Scirpus triqueter in pyrene-Ni co-contaminated soils. Chemosphere. 2020;241:125027.
- Hynninen A, Tonismann K, Virta M. Improving the sensitivity of bacterial bioreporters for heavy metals. Bioeng Bugs. 2010;1:132–138.