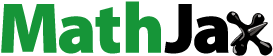
ABSTRACT
The fur is hard to decompose during the fermentation process of diseased swine carcasses. In order to enhance the enzymolysis of pigskin, the ultrasonic was proposed to use during the process of the enzymatic hydrolysis. The response surface optimization experiments were carried out with the DH (degree of hydrolysis) as the response value and the optimum conditions for enzymatic hydrolysis were determined. Based the optimum conditions, orthogonal experiments were carried out with ultrasonic frequency, power and time as variables, and optimal ultrasonic parameters were obtained. Without the assistance of ultrasonic, the descending order of influence factors on DH was, temperature>SC(Substrate concentration)>RES(The ratio of enzyme to substrate)>pH. Moreover, the DH value is of 10.42% under the following optimal conditions: RES is of 16,006 U/g, the temperature is of 48.92°C, the SC is of 59.76 g/L and pH is of 10.43. Frequency has the greatest effect on DH, followed by power, and finally time. The optimum hydrolysis time is of 5 h, and the DH is of 22.94% were obtained under the following optimum ultrasonic pretreatment conditions: frequency combination is of (20,40,40), power is of 600 W and time is of 25 min. Comparing with the group without ultrasonic pretreatment, the DH for the ultrasonic assistance increased by 4%, the hydrolysis time was shorten by 3 h, and the total amino acids increased by 15.98%.
1 Introduction
In recent years, the quantity of livestock and poultry are increasing with the large-scale development of animal husbandry in China. The number of slaughtering of pigs in 2016 was 685 million, but the annual death rate caused by various diseases was 8% to 12% [Citation1]. These diseased swine carcasses are a huge source of environmental pollution, while it is also a pool of resource after a proper disposal [Citation2]. The harmless treatment of diseased swine carcasses had attracted increasing attention [Citation3,Citation4]. Incineration and burial are the current practices for diseased swine carcasses disposal. However, these methods are not environmentally friendly [Citation5]. Currently, biological treatment is preferred. Anaerobic digestion is a promising technology for the treatment of sterilized animal carcasses [Citation6]. But the fur is hard to decompose in this process and incineration or landfill is still needed in the end, which will cause energy waste and environmental pollution [Citation7,Citation8]. Enzyme hydrolysis treatment can be an effective method for pigskin pretreatment and the process requires only temperature control at low and can promote the decomposition of organic matter in the solid phase [Citation9].
At present, studies on enzymatic hydrolysis of pigskin mainly focus on the field of medicine and food. Zhang [Citation10] used three proteases to hydrolyze fresh pigskin respectively and adopted the orthogonal design to determine the optimum condition of hydrolysis by assessing the degree of hydrolysis. Wu [Citation11] studied the hydrolysis of pigskin with Protamex complex protease and the optimum conditions for enzymatic hydrolysis of pigskin were obtained by response surface methodology, when pH is 6.3, temperature is 53.2°C, RES(The ratio of enzyme to substrate) is 2.65%, SC(Substrate concentration) is 15.0% and hydrolysis time 6 h, the DH(Degree of hydrolysis) is 16.13%. Based on the gel properties and selecting hydroxyproline content as an index, Su [Citation12] selected the fresh pigskin as raw material to study the factors which affects the enzymatic hydrolysis of pepsin. The enzymatic hydrolysis conditions were optimized by Box-Behnken design, when the enzyme dosage is 0.25%, the ratio of material to liquid is 1:2 g/mL, and hydrolyzate for 1.65 h at 41.15°C, under this condition the content of hydroxyproline is 4.8495%.
Ultrasonic has the dual properties of energy and fluctuation, and its mechanical and cavitation effects can make cell rupture to improve the reaction efficiency and the reaction time will be shorten [Citation13,Citation14]. Zhang [Citation15] got the production of antioxidant peptides from wheat bran by enzymatic hydrolysis with ultrasonic assistance, then they studied the effects of papain dosage, ultrasonic power, reaction temperature, reaction time, and pH on the antioxidant activity of wheat bran polypeptides, and the optimal ultrasonic assisted enzymatic hydrolysis conditions were obtained. Maria et al. [Citation16] evaluated the effect of ultrasonic treatment on the commercial cellulases and the enzymatic hydrolysis of sugarcane bagasse. There was an 89.37% higher concentration of reducing sugars in comparison with the control (unsonicated sample) under the optimal conditions. Jiao et al. [Citation17] proposed ultrasonic-assisted ammonium bicarbonate pretreatment of corn stalk. The results showed that the highest saccharification rate of corn stalk was 82.61%, which was remarkably increased by 355% compared to the control group. Aylin et al. [Citation18] compared the effect of ultrasonication and microwave sludge disintegration on the anaerobic co-digestion of olive and grape pomaces. Ultrasonication pretreatment was found much more effective than microwave irradiation. The specific energy applied in microwave pretreatment was almost 9 times higher than that of used in ultrasonication, resulting only 10–15% increases in biogas yield. Kumari and Singh [Citation19] studied the production of methane and ethanol by ultrasonic assisted petha waste water pretreatment of rice straw. Their results indicated that the methane yield was about three times more than that of reactors without ultrasonication and the ethanol yield was about two times more than that of reactors without ultrasonication.
Ultrasonic can increase enzyme activity at low intensity and appropriate frequency, and the application of ultrasonic in enzymatic hydrolysis of pigskin can improve the enzymatic activity under suitable substrate concentration and promote the hydrolysis process. Based on the reviews, few studies applied ultrasonic to decomposition of pigskin. The ultrasonic was applied to the enzymatic hydrolysis process of pigskin to explore the optimum conditions in this paper. Mathematical model was established by using Box-Behnken central composite design, a response surface optimization experiment with four factors and three levels was carried out with the DH as the response value. And the optimum conditions for enzymatic hydrolysis were determined. On this basis, three-dimensional ultrasonic pretreatment with different frequency, power, and time was added, the ultrasonic conditions which were most suitable for enzymatic hydrolysis were analyzed through orthogonal experiment.
2 Materials and methods
2.1 Materials and instruments
The pigskin of Non violent illness death pig as raw materials was derived from Muyuan Group, Henan Province, and the compositions were determined by chemical method. The compositions of pigskin are shown in . The data represents the mass fraction. The Alkaline protease used in experiment were purchased from Shanghai Yuanye Biotechnology Co., Ltd, and other reagents are analytical pure.
Table 1. The compositions of pigskin
Added the shaved pigskin, fat and water to the raw material according to the set ratio, adjusted to the appropriate pH, and put into the ultrasonic reactor for pretreatment (the control group without pretreatment). After this, the enzyme was added in proportion and moved to a constant temperature water bath (HH-2, Shanghai Corchen Experimental Instrument, China) for enzymatic hydrolysis. Then inactivated for 10 min at 85°C in resistance furnace(TCXC1200- II, Shanghai Tongke Electric furnace equipment, China) and centrifugation(TDL-5-A, Shanghai Anting Science Instrument) 30 min under 5000 r/min condition. The supernatant was taken and the DH was measured.
The multifrequency ultrasonic reactor in this experiment is a self-made device by Key Laboratory of New Materials and Facilities for Rural Renewable Energy of China’s Ministry of Agriculture. The structure of the device is shown in the .
2.2 Experiment scheme
The effects of temperature, SC, RES and pH on enzymatic hydrolysis were investigated. Taken the DH as the research objective. The optimum single-factor experiment conditions were selected from the following, temperature (40, 45, 50, 55, 60°C), SC (20 g/L, 40 g/L, 60 g/L, 80 g/L, 100 g/L), RES (11,000 U/g, 13,000 U/g, 15,000 U/g, 17,000 U/g, 19,000 U/g), and pH (7, 8, 9, 10, 11). In the single-factor experiments, the other three factors were controlled separately as temperature was 50°C, SC was 60 g/L, RES was 15,000 U/g, and pH was 10.
According to the principle of Box-Behnken test design and the result of single-factor experiment. The appropriate range of each factor in the response surface design was determined, that was temperature (45, 50, 55 °C), SC (40 g/L, 60 g/L, 80 g/L), RES (13,000 u/g, 15,000 u/g, 17,000 u/g), and pH (8, 9, 10). And the optimum process parameters were obtained by analyzing the regression equation.
According to the principle of orthogonal experiment design, the effects of different frequencies (20 kHz, 40 kHz, 60 kHz), powers (400 W, 600 W, 800 W), and times (20 min, 25 min, 30 min) on the DH were studied based on the experiment of response surface optimization. And then the DH and hydrolysis time were compared between the optimum ultrasonic pretreatment group and control group.
2.3 Test method
Determination of water content was measured according to GB 5009.3–2010. Total nitrogen content was determined according to ISO 937–1978 (E). Protein content was determined according to micro Kjeldahl method GB/T 6432–1994. The fat content was determined according to Soxhlet extraction GB/T 5009.6–2003. Ash content was determined according to burning weighing method GB/T 5009.4–2003. Determination of amino acids was measured according to GB/T 5009.124–2003. Enzyme activity was determined according to protease activity SB/T 10317–1999. The pH value was determined by a pH meter (PHS-3 C, Nanjing Coro Analysis Instrument, China). Total nitrogen were measured by Automatic Kjeldahl (LJELTEC2300, Foss). The SEM were observed by Environmental Scanning Electron Microscope (QUANTA-650, FEI, USA).
Degree of hydrolysis was measured according to formaldehyde titration. Transferred 5.0 mL of supernatant after centrifugation to a 100mL volumetric flask and diluted with water to volume. Taken V = 20 mL from the constant volume bottle and added 60 mL deionized water, then mixed uniformity. Adjusted pH to 8.2 by titration with 0.05 mol/L sodium hydroxide standard solution and added 10.00 mL formaldehyde solution (the content ranges from 36% to 40%), adjusted pH to 9.2 with 0.05 mol/L sodium hydroxide standard solution after stirred uniformly. Recorded the consumption of sodium hydroxide as V1. Taken 5 mL deionized water instead of enzymatic hydrolyzate, then repeat the above operation, recorded the consumption of sodium hydroxide as V0. X is the amino nitrogen content in enzymatic hydrolyzate (mg/mL)
The free amino acids were determined by Liquid chromatograph (2695, Voight science and Technology Limited public). On chromatographic conditions, selected Phenomenex Luna C18(250 mm×4.6 mm, 5 m) as chromatographic column, 0.05 mol/L sodium acetate buffer solution(containing 1%N-N DMF) as mobile phase A and the ratio of acetonitrile to water in mobile phase B is 1:1. Setting the flow rate as 1 mL per minute, column temperature as 40°C, detection wavelength as 360 nm, and injection volume as 10 L.
3 Results and analysis
3.1 Results of single-factor experiment
The results of single factor experiment are shown in .
The temperature has a great influence on the DH of alkaline protease. At 40 ~ 50°C, the reaction rate of enzyme hydrolysis is faster and faster with the increase of temperature. And the DH reaches the maximum value of 10.35% at 50°C.With the increase of substrate concentration, the DH increase first and then decreases. The reaction rate of hydrolysis will be reduced when the substrate concentration is too low or too high. When the substrate concentration is of 60 g/L, the DH is of 10.38%. The DH increases rapidly with the increase of RES, when RES is at low range. And when RES reaches the value of 15,000 U/g, the DH tendes to be gentle. Taking both hydrolysis speed and cost into consideration, the most appropriate choice of RES is around 15,000 U/g. With the gradual increase of pH, DH increases first and then decreases, and when the pH value is of 10, alkaline protease has the strongest hydrolysis ability to pigskin with the DH of 10.21%.
3.2 Results of response surface experiment
The Box-Behnken experimental design was to find the best process parameters by analyzing the regression equation. The results are shown in the .
Table 2. Design and analysis for response surface
Through data analysis, the follow equation is obtained.
It can be seen from the that, the linear terms X1, X3, X4, the interaction terms X1X3, X2X3, X3X4 and the quadratic terms X12, X22, X32, X42 are very significant. Accordingly, the influence of test factors on response value is not a linear relation. The lack of fit item P = 0.0623 > 0.05 is not obvious thus the equation is better fitted to the experiment. And the model reaches a very significant level (P < 0.01), and the correlation coefficient R2 = 0.9666. It shows that 96.66% of the variation of DH is derived from the selected variables. Therefore, it is feasible to use the equation model to simulate the relationship between the factor and response value.
Table 3. Variance analysis of regression model
The response surface stereogram and contour plot are as shown in .
According to the results of Box-Behnken experiment and analysis of variance, the optimal combination of these four factors is obtained by Design Expert 8, when RES is of 16,006 U/g, the temperature is of 48.92°C, the SC is of 59.76 g/L, and pH is of 10.43. Under these conditions, the DH is of 10.42%. And in order to verify the reliability of the equation, three groups of repeated experiment were carried out under the optimal combination conditions. The DH values of experiment results are 10.37%, 10.43%, and 10.39%, respectively. The relative error between actual value and predicted value is 0.22%. So this equation model can predict the actual hydrolysis condition accurately.
3.3 Results of orthogonal experiment of ultrasonic pretreatment
It can be seen from that the DH of pigskin first increases and then decreases with the ultrasonic power. When the power is in a certain range, the structural damage of pigskin is becoming more and more serious with the increase of ultrasonic power. This is beneficial to the contact between enzyme and substrate, exposed more suitable enzymatic reaction points, and promoted the enzymatic reaction. And then DH increases. When the power is 600 W, the DH reaches the maximum value of 16.3%. As the power continues to increase, the structure of enzyme is destroyed, the activity of enzyme is reduced and the DH is gradually reduced.
Before 25 min, the DH of pigskin increases with the ultrasonic pretreatment time. But the DH decreases gradually after 25 min. When the pretreatment time is 25 min, the DH reaches its maximum value of 16.8%. It is indicated that the structure of pigskin and enzyme activity are related to the ultrasonic pretreatment time. With the increase of pretreatment time, the cavitation and mechanical effects of ultrasonic destroyed the structure of pigskin and promoted enzymatic reaction. When the time is more than 25 min, the structure and activity of enzyme are destroyed gradually, affecting the hydrolysis process and reducing DH.
As can be seen from , in single-frequency group, the DH increases first and then decreases with the increase of ultrasonic frequency. And when the frequency is 40 kHz, alkaline protease has the best hydrolysis effect on pigskin. The reason is that the structure of pigskin is destroyed with the increase of frequency, which increases the probability of enzyme contacting substrate and promotes the enzymatic reaction. But when the frequency is too high, the structure of enzyme molecular is destroyed, its activity is reduced, the enzymatic reaction is slowed down and then the DH is reduced.
Dual frequency group is better than single frequency group, but not all of them. The highest DH is (20, 40) test group. The dual frequency bidirectional ultrasonic used in this experiment is the two waves propagate vertically at different frequency, its uniformity and penetration are strong. More complex waveforms were generated through the superposition of ultrasonic waves, it can improve the enzyme activity effectively and destroy the substrate structure. And the enzymatic reaction is carried out smoothly so the DH increases.
Similarly, the ultrasonic in three-dimensional space has a stronger penetration, the superposition of three-dimensional ultrasonic produced more complex waveforms and the increase of enzyme activity is more obvious, which is more conducive to hydrolysis. It can be seen by comparison that the highest DH in triple frequency group is superior to the other two groups. And the (20, 40), (20, 40, 40), (40, 40, 40) group promotes the enzymatic better.
The results of orthogonal experiment of ultrasonic pretreatment and analysis of variance are as shown in and respectively.
Table 4. Results of orthogonal experiment of ultrasonic pretreatment
Table 5. The analysis of variance
From the R value of the orthogonal experiment results, it can be seen that the magnitude and order of influence factors on DH is, frequency>power>time. The optimal combination of hydrolysis condition is A1B2C2, which corresponding to frequency combination is (20, 40, 40), power is 600 W and time is 25 min.
It can be seen from that from the beginning of reaction to 1 h, the DH increases rapidly, the control group reaches 10.38% and the ultrasonic pretreatment group reaches 17.88%. Ultrasonic pretreatment has a significant promoting effect on hydrolysis. Then the DH continues to increase, but its increase rate significantly decreases. After 8 h, the DH of control group tends to be gentle. It can be concluded that the best hydrolysis time is 8 h and the DH is 18.73% at this moment. After 5 h, the curve of ultrasonic pretreatment group tends to be gentle. The best hydrolysis time is 5 h for ultrasonic pretreatment group and the DH is 22.94% at that time. This indicates that ultrasonic pretreatment under suitable conditions can shorten the hydrolysis time 3 h and increase the DH by 4%.
The SEM of pigskin untreated and after pretreatment are shown in . It can be seen that the surface of untreated pigskin is clear and uniform. And the direct enzymatic hydrolysis of pigskin is limited because of the three-dimensional structure of pigskin fibrils. After ultrasonic pretreatment, the structure of pigskin is destroyed, the protein is dissolved and the tissue is softened, which can make the enzyme contacted with the protein directly and conducive to enzymatic reaction.
Setting the control group conditions as enzyme dosage is of 16,006 U/g, temperature is of 48.92°C, substrate concentration is of 59.76 g/L and pH is of 10.43, and ultrasonic group conditions as ultrasonic frequency is (20, 40, 40), power is of 600 W, and pretreatment time is of 25 min, the two groups are enzymolysis for 1 h normally.
The types and quantities of free amino acids in hydrolyzates are shown in the . The total amount of free amino acids in hydrolyzate after ultrasonic treatment reaches 667.31 mg/100 mL, which is 15.89% higher than that in control group. It indicates that ultrasonic pretreatment can promote enzymatic reaction.
4 Conclusion
Ultrasonic pretreatment obviously enhanced hydrolysis of pigskin. Under optimum conditions (the ratio of enzyme to substrate is of 16,006 U/g, temperature is of 48.92°C, substrate concentration is of 59.76 g/L, pH is of 10.43), hydrolysis time is 8 h and hydrolysis degree is 18.73%. Under optimum ultrasonic conditions (ultrasound frequency is of (20, 40, 40), power is of 600 W, pretreatment time is of 25 min), hydrolysis time was shorten by 3 h and hydrolysis degree increased by 4%. Moreover, isothermal enzymatic hydrolysis for 1 h after ultrasonic pretreatment, amino acids in enzymatic hydrolyzates increased by 15.98%.
Highlights
Ultrasonic pretreatment was proposed for enzymolysis of pigskin.
Degree of hydrolysis with the ultrasonic assistance increased by 4%.
The hydrolysis time with the ultrasonic assistance was shorten by 3 h.
Amino acids in hydrolysates increased by 15.98% with the ultrasonic assistance.
Acknowledgments
This work was supported by the [National Natural Science Foundation of China] under Grant [number 51706063]; [Young Key Teachers Training Program of Henan Higher Education Institutions] under Grant [number 2018GGJS028], and [Henan Youth Talent Project] under Grant [2019HYTP026].
Disclosure Statement
No potential conflict of interest was reported by the authors.
Additional information
Funding
References
- National Bureau of Statistics of the People’s Republic of China. National statistical yearbook of China in 2016. Beijing: China Statistics Press; 2016.
- Zhang HY. Experimental study on the biological and chemical combined treatment on corpse of livestock and poultry. Ph.D. Graduation thesis of Henan Agricultural University. China; 2015.
- Harmless treatment supervision team of Chinese Ministry of Agriculture. Investigation report of harmless treatment of Shandong, Jiangxi and Hubei. China Anim Quarantine. 2013;30:6–8.
- Wang SM. Suggestions on biological safety treatment and resource utilization of dead animals. China Anim Quarantine. 2013;30:15–17.
- Xu J, Mustafa AM, Lin HJ. Effect of hydrochar on anaerobic digestion of dead pig carcass after hydrothermal pretreatment. Waste Manage. 2018;78:849–856.
- Gwyther CL, Williams A, Golyshin PN. The environmental and biosecurity characteristics of livestock carcass disposal methods. Waste Manage. 2011;31:767–778.
- EL-Refal HA, Abdel MA, Gaballa A. Improvement of the newly isolated Bacillus pumilus FH9 keratinolytic activity. Process Biochem. 2005;40:2325–2332.
- Park GT, Son HJ. Keratinolytic activity of Bacillus megaterium F 7-1, a feather-degrading mesophilic bacterium. Microbiol Res. 2009;164(4):478–485.
- Pavlovic I, Knez Z, Skerget M. Hydrothermal reactions of agricultural and food processing wastes in sub and supercritical water. J Agric Food Chem. 2013;61:8003–8025.
- Zhang ZS, Li GJ, Shu Y. Research on preparation process of collagen polypeptide from pig skin by two-step-hydrolysis. FASEB J. 2014;28:LB397.
- Wu L. Optimization of enzymatic hydrolysis conditions of pigskin. Modern Food Sci Technol. 2009;25:1058–1060.
- Su W, Yang XH, Wang Y, et al. Optimization of enzymatic hydrolysis of porcine skin based on collagen characteristics and antioxidant activity of resulting hydrolysate. Food Sci Technol. 2015;36:70–76.
- Prado M, Borea L, Cesaro A, et al. Removal of emerging contaminant and fouling control in membrane bioreactors by combined ozonation and sonolysis. Int Biodeterior Biodegrad. 2016;119:577–586.
- Wang ZY, Hao WF. Study on the Jew’s ear protein extraction by ultrasonic method. Biomass Chemical Engineering. 2007;41:25–27.
- Zhang H. Preparation technology of antioxidant peptides from wheat bran by ultrasonic-assisted enzymatic hydrolysis. Food Sci Technol. 2016;41:219–224.
- de Carvalho Silvello MA, Martínez J, Goldbeck R. Increase of reducing sugars release by enzymatic hydrolysis of sugarcane bagasse intensified by ultrasonic treatment. Biomass Bioenergy. 2019;122:481–489.
- Jiao YZ, Guo CP, Wang SP, et al. Enhancement of converting corn stalk into reducing sugar by ultrasonic-assisted ammonium bicarbonate pretreatment. Bioresour Technol. 2020;302:122878.
- Aylin Alagöz B, Yenigün O, Erdinçler A. Ultrasound assisted biogas production from co-digestion of wastewater sludges and agricultural wastes: comparison with microwave pre-treatment. Ultrason Sonochem. 2018;40:193–200.
- Kumari D, Singh R. Ultrasonic assisted petha waste water pretreatment of rice straw for optimum production of methane and ethanol using mixed microbial culture. Renewable Energy. 2020;145:682–690.