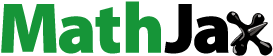
ABSTRACT
This study investigated anti-polyphenol oxidase activity and mechanism of purified total flavonoids (PTF) from young loquat fruits. PTF remarkably inhibited the activity of polyphenol oxidase (PPO) with an IC50 value of 21.03 ± 2.37 μg/mL. Based on enzyme kinetics, PTF was found to be a potent, mixed-type, and reversible inhibitor of PPO. The fluorescence intensity of PPO was quenched by PTF through forming a PTF-PPO complex in a static procedure. Therefore, this study authenticated PTF as an efficient PPO inhibitor, which would contribute to their utilization in food industry.
1. Introduction
Polyphenol oxidase (PPO, EC 1.14.18.1), or tyrosinase, is a rate-limiting enzyme in the catalysis of L-DOPA to o-quinones, and finally melanin biosynthesis [Citation1,Citation2]. In the food industry, PPO has a crucial role in the enzyme-mediated browning of vegetables and fruits, which often leads to significant losses of economic value and nutritional quality [Citation3]. Browning due to enzymatic activities is a major problem of the food industry, particularly for vegetables, seafood products, and fruits [Citation4]. Hence, efficient and secure inhibitors of PPO are crucial for their use in the food industry.
Browning in food results in a multitude of adverse effects on food preservation. Therefore, browning inhibitors are widely utilized in the food industry for the inhibition of browning due to enzymes [Citation5,Citation6]. Various anti-browning agents such as oxalic acid, citric acid, 4-hexylresorcinol, and ascorbic acid are good browning inhibitors, but they are not as effective as sulfite compounds which were confirmed potential health risks to sensitive individuals [Citation7]. Therefore, it is imperative to discover effective and safe options for these chemicals.
Natural flavonoids have been receiving increasing attention due to their various biological activities. The flavonoids have been shown to be effective in the treatment of various morbidities such as cardiovascular risk, Alzheimer’s disease, age-related disorders, hyperglycemia, and cancer [Citation8]. In particular, the flavonoids also have antibacterial, antioxidative, and anti-inflammatory effects [Citation9]. A large number of young loquat fruits are clipped off during the process of planting to achieve better yield and quality of loquat fruits. So there are sufficient raw materials of the young loquat fruits for extraction of useful chemicals. However, there are only a few relevant studies on the flavonoids of young loquat fruits.
In this research, we investigated the inhibition effect and mechanism of PTF on PPO by using UV-visible absorption spectroscopy, full wavelength scanning study, fluorescence quenching assay, and three-dimensional fluorescence spectra. This study aimed to evaluate purified total flavonoids (PTF) from young loquat fruits and its anti-polyphenol oxidase activity and offer a scientific basis for their applications in food industry.
2. Materials and methods
2.1. Reagents and enzymes
L-3,4-dihydroxyphenylalanine (L-DOPA), polyphenol oxidase (EC 1.14.18.1), and Rutin were procured from Sigma-Aldrich (St. Louis, USA). Ethanol for the extraction was obtained from Sinopharm (Shanghai, China). Macroporous adsorption resin AB-8 was obtained from Donghong Chemical Co. Ltd (Jiangsu, China).
2.2. Extraction and purification of TF
The process of extraction and purification was implemented according to our previous report [Citation10]. Young fruits of loquat of the ‘Baiyu’ cultivar were collected at Dongshan Zhen, Suzhou, in Jiangsu Province (China). The young loquat fruits were dried in an oven (DHG-9055A; Yiheng, China). Dry powder of the fruits was obtained by grinding and sieving the dried young fruits. The powders were extracted through ultrasound-assistance in a ratio of 1: 64 (solid: liquid; g/mL), in 49% ethanol at 65°C for 20 min. The crude flavonoids were then separated with an AB-8 column, which was eluted with water and ethanol-water (49%) in this order. Removal of ethanol was done through rotary evaporation and the part that remained was freeze-dried to acquire the purified total flavonoids (PTF). The standard used here was Rutin, and the estimated purity was 93.02%.
2.3. Wavelength scanning study
The study was conducted with reference to our previous experimental method [Citation11]. L-DOPA oxidation was conducted in the presence or absence of PTF. Three milliliter of reaction media contained 0.5 mmol/L L-DOPA, sodium phosphate buffer at pH 6.8 and 20 μg/mL PTF. Finally, the enzyme was mixed into the media and the absorbance was measured on the Beckman DU-8000 spectrophotometer (Beckman Coulter, Pasadena, CA, USA).
2.4. Enzymatic kinetics experiments
The experiments were referred to previous studies method [Citation12]. For the assay of enzyme activity, 3 mL of reaction media contained 0.5 mmol/L L-DOPA, sodium phosphate buffer at pH 6.8, and 0.1 mL PTF at different concentrations. The temperature of the reaction was 30°C. The enzyme activity was measured at 475 nm having 3700 (M−1 cm−1) as the molar absorption coefficient on the Beckman DU-8000 spectrophotometer.
2.5. Fluorescence quenching assay
The enzyme and inhibitor interaction was examined by fluorescence quenching [Citation13], which was recorded by a Varian Cary Eclipse fluorescence spectrophotometer from Agilent (Santa Clara, USA) at 290 nm excitation wavelength [Citation14]. The fluorophore of is Tyr and Trp residues. The fluorescence spectrum in the range of 300 to 450 nm was recorded. The kind of fluorescence quenching mechanism was determined using the Stern–Volmer equation [Citation15].
where F and F0 indicate the intensities of fluorescence with or without an inhibitor. Kq denotes the rate constant for biomolecular quenching. τ0 represents the average fluorophore lifetime without the inhibitor (τ0 = 10−8 s). [I] denotes the inhibitor concentration. KSV denotes the Stern–Volmer’s constant for quenching.
Dynamic and static are the two modes of fluorescence quenching [Citation16]. For the static fluorescence quenching interaction, the calculation of Ka (binding constant) and n (number of binding sites) could be done by the double logarithmic equation [Citation17].
The PPO 3D (three-dimensional) fluorescence spectra with and without PTF were measured by using emission and excitation wavelengths in the range of 200 to 600 nm.
2.6. Statistical data analysis
Analyses of all statistical data were conducted using GraphPad Prism 6 (CA, USA). The error bars are represented as the means ± SEM from experiments performed in at least three triplicates.
3. Results
PPO is an important enzyme that is responsible for browning in fruits and vegetables. PPO inhibitors are quite important in the area of medicine and food industry. Natural products flavonoids have high therapeutic potential against multifactorial and complex diseases. In this study, the inhibitory effect and mechanism of PTF on PPO was studied by multi-spectroscopic methods. The results demonstrated that PTF was an effective inhibitor of PPO, which could provide scientific basis in the development of natural PPO inhibitors.
3.1. Impact of PTF concentration on PPO activity
The inhibition of PPO activity due to PTF from young loquat fruits was examined. As shown in , PTF exhibited a strong inhibition against the PPO activity in a dose-dependent manner but was not suppressed completely. The concentration of PTF causing the loss of 50% relative enzyme activity (IC50) was determined to be 21.03 ± 2.37 μg/mL and was less than that of quercetin (IC50 = 39.2 ± 4.1 μg/mL) and proanthocyanidins from cherimoya pericarp (IC50 = 37.3 ± 3.6 μg/mL) [Citation18,Citation19], manifesting that PTF from young loquat fruits was a more effective PPO inhibitor.
3.2. L-DOPA oxidation in the absence and presence of PTF
The PPO catalysis spectra obtained during L-DOPA oxidation without ()) and with ()) PTF in the reaction system are presented. The peak absorbance at 475 nm was 0.402 at 9 min post-PPO addition. In the presence of PTF, the peak intensity decreased to 0.131, representing a decrease of 67.41%, indicating that PTF from young loquat fruits was a strong inhibitor of PPO.
3.3. PTF type and associated mechanism of PPO inhibition
The enzyme activity against the PPO concentration with different concentrations of PTF in the reaction system was plotted to evaluate the inhibitory mechanism. As shown in , a group of straight lines crossed the origin, and the increase in PTF concentration, caused a decrease in the slope of the line, showing that the presence of PTF did not reduce PPO content, but caused a decline in the activity of PPO. The above results suggested that the inhibition of PTF on PPO was reversible.
Figure 3. Inhibition mechanism of PTF from young loquat fruits on PPO. The concentrations of PTF for lines 1–5 were 0, 5, 10, 15, and 20 μg/mL
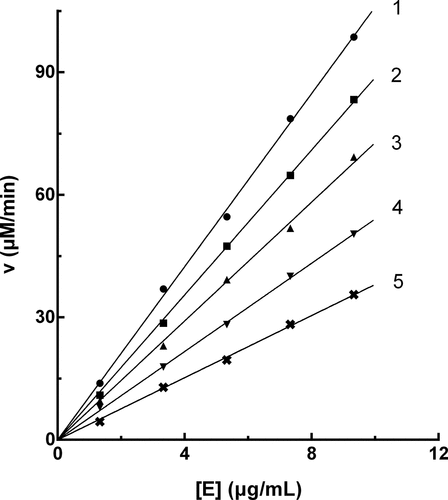
The inhibitory kinetic behavior of PTF on PPO was then studied. The Lineweaver-Burk plot was shown in ). The intersection of all straight lines occurred in the second quadrant, indicating the mixed-type inhibitor characteristic of PTF. The inhibition constants of PTF binding with the free enzyme (KI) and with the enzyme-substrate complex (KIS) were 17.95 ± 0.53 μg/mL and 69.99 ± 4.52 μg/mL, respectively. KI and KIS were calculated from ), respectively, with absolute value of intercept from X-axi. The value of KIS was higher than that of KI, suggesting the affinity of PTF with PPO-L-DOPA complex was weaker than that with PPO.
3.4. PPO Fluorescence quenching in the presence of PTF
To demonstrate the interaction between PPO and PTF, fluorescence quenching was carried out. As presented in ), there was a gradual reduction in fluorescence intensity of PPO with the increase in PTF concentration. The reduction was 56.66% when the PTF concentration was up to 20 μg/mL ()), which demonstrated that PTF was a good fluorescence quencher of PPO.
Figure 5. Effect of PTF from young loquat fruits on the emission spectrum of PPO. (a), Intrinsic fluorescence changes. (b), Florescence intensity changes. (c), Stern–Volmer curves of PTF. (d), Plot of log[(F0 − F)/F] against log[I] for PTF
![Figure 5. Effect of PTF from young loquat fruits on the emission spectrum of PPO. (a), Intrinsic fluorescence changes. (b), Florescence intensity changes. (c), Stern–Volmer curves of PTF. (d), Plot of log[(F0 − F)/F] against log[I] for PTF](/cms/asset/fc5a35ec-bf88-4961-8df5-e72b282ed863/kbie_a_1886387_f0005_oc.jpg)
The Stern-Volmer equation was applied to assess the mechanism of fluorescence quenching. F0/F increased gradually with increasing concentration of PTF ()), which presented a good linear relationship, showing that the PPO fluorescence quenching process by PTF was in a static mode [Citation20]. The values of Kq (biomolecular quenching rate constant) and KSV was determined to be 5.578 × 106 (μg/mL·s)−1 and 5.578 × 10−2 (μg/mL·s)−1, respectively.
For the static fluorescence quenching, n (the number of binding sites) and Ka (the binding constant) could be estimated from the lg[(F0 − F)/F] plot compared with lg[I] ()). The values of n and Ka were 1.124 and 0.043 (μg/mL)−1, respectively. The number of binding sites (n) was nearly equal to 1, demonstrating only a single binding site for PTF on PPO.
3.5. Three-dimensional fluorescence spectroscopy
A powerful fluorescence analysis assay, three-dimensional fluorescence spectroscopy, was used to investigate the conformational change of PPO. According to , the peak a is the Rayleigh scattering peak (λex = λem), while peak b is the second-order-scattering peak (λex = 2λem) [Citation21]. Peak 1 (λem = 340 nm, λex = 280 nm) indicates the feature of Tyr and Trp residues. Peak 2 (λem = 340 nm, λex = 230 nm) mainly reveals the characteristic of polypeptide chain skeletal structure of PPO, which caused by the π → π* transition [Citation22]. With the addition of PTF to PPO solution, the intensity of fluorescence of peaks 1 and 2 reduced from 90.3 to 33.8, and 43.9 to 23.2, respectively. The above result demonstrates the conformational change of PPO during the formation of the PTF-PPO complex.
4. Discussion
The main catalytic function of PPO is conversion of tyrosine into melanin pigments, which leads to browning of vegetables and fruits [Citation23]. Hence, efficient and secure inhibitor of PPO is crucial in improving food quality. Flavonoids are a broad group of low-molecular-weight natural antioxidant compounds [Citation24]. In our present study, we found that natural extract PTF was an efficient inhibitor of PPO with the semi-inhibitory rate (IC50) 21.03 ± 2.37 μg/mL. From Lineweaver-Burk plot, we can calculate that the inhibition constants KI was 17.95 ± 0.53 μg/mL and KIS was 69.99 ± 4.52 μg/mL. The KI value was much lower than the KIS value, indicating that the inhibitor has a stronger affinity to PPO than PTF-PPO complex.
5. Conclusions
In conclusion, this study showed that PTF from young loquat fruits was a reversible, mixed competitive, and efficient inhibitor of PPO. The IC50 for PTF was 21.03 ± 2.37 μg/mL. The fluorescence of PPO was quenched by PTF due to PTF-PPO complex formation in a static process. In addition, PTF was bound to PPO at a single binding site and caused the conformational change of PPO. This study reveals the feasibility of using PTF from young loquat fruits in food industry.
Highlights
PTF is efficient, reversible, and mixed competitive inhibitors of PPO.
PTF has one binding site with PPO.
PTF induced conformational change of PPO.
Supplemental Material
Download ()Disclosure statement
Authors declare no conflict of interest.
Supplementary material
Supplemental data for this article can be accessed here.
Additional information
Funding
References
- Cui Y, Liang G, Hu YH, et al. Alpha-substituted derivatives of cinnamaldehyde as tyrosinase inhibitors: inhibitory mechanism and molecular analysis. J Agric Food Chem. 2015;63:716–722.
- Korner A, Pawelek J. Mammalian tyrosinase catalyzes three reactions in the biosynthesis of melanin. Science. 1982;217:1163–1165.
- Dong T, Cao Y, Jiang CZ, et al. Cysteine protease inhibitors reduce enzymatic browning of potato by lowering the accumulation of free amino acids. J Agric Food Chem. 2020;68:2467–2476.
- Loizzo MR, Tundis R, Menichini F. Natural and synthetic tyrosinase inhibitors as antibrowning agents: an update. Compr Rev Food SCI F. 2012;11:378–398.
- Paudel P, Seong SH, Wagle A, et al. Antioxidant and anti-browning property of 2-arylbenzofuran derivatives from Morus alba Linn root bark. Food Chem. 2020;309:125739.
- Niphadkar SS, Vetal MD, Rathod VK. Purification and characterization of polyphenol oxidase from waste potato peel by aqueous two-phase extraction. Prep Biochem Biotechnol. 2015;45:632–649.
- Mohsenabadi M, Ghasemnezhad M, Hashempour A, et al. Inhibition of polyphenol oxidases and peroxidase activities in green table olives by some antibrowning agents. Agri Consp Sci. 2018;82:375–381.
- Panche AN, Diwan AD, Chandra SR. Flavonoids: an overview. J Nutr Sci. 2016;5:e47.
- Musumeci L, Maugeri A, Cirmi S, et al. Citrus fruits and their flavonoids in inflammatory bowel disease: an overview. Nat Prod Res. 2020;34:122–136.
- Huang GL, Wu MR, Ma JJ, et al. Optimization of extraction process of total flavonoids from young loquat fruit and analysis of its antioxidant activity. J Food Saf Qual. 2020;11:3112–3118.
- Huang GL, Ma JJ, Sui SY, et al. Optimization of extraction of loquat flowers polyphenolics and its antioxidant and anti-polyphenol oxidase properties. Bioengineered. 2020;11:281–290.
- Huang Q, Chai WM, Ma ZY, et al. Antityrosinase mechanism of ellagic acid in vitro and its effect on mouse melanoma cells. J Food Biochem. 2019;43:e12996.
- PK S, Bathula C, KN C, et al. Usage of graphene oxide in fluorescence quenching-linked immunosorbent assay for the detection of Cry2Ab protein present in transgenic plants. J Agric Food Chem. 2020;68:3656–3662.
- Chai WM, Ou-Yang C, Huang Q, et al. Antityrosinase and antioxidant properties of mung bean seed proanthocyanidins: novel insights into the inhibitory mechanism. Food Chem. 2018;260:27–36.
- Jia J, Gao X, Hao M, et al. Comparison of binding interaction between beta-lactoglobulin and three common polyphenols using multi-spectroscopy and modeling methods. Food Chem. 2017;228:143–151.
- Li Y, He W, Liu J, et al. Binding of the bioactive component jatrorrhizine to human serum albumin. Biochim Biophys Acta. 2005;1722:15–21.
- Shahabadi N, Maghsudi M, Rouhani S. Study on the interaction of food colourant quinoline yellow with bovine serum albumin by spectroscopic techniques. Food Chem. 2012;135:1836–1841.
- Chai WM, Lin MZ, Wang YX, et al. Inhibition of tyrosinase by cherimoya pericarp proanthocyanidins: structural characterization, inhibitory activity and mechanism. Food Res Inter. 2017;100:731–739.
- Chen QX, Kubo I. Kinetics of mushroom tyrosinase inhibition by quercetin. J Agric Food Chem. 2002;50:4108–4112.
- Wang Y, Zhang G, Yan J, et al. Inhibitory effect of morin on tyrosinase: insights from spectroscopic and molecular docking studies. Food Chem. 2014;163:226–233.
- Chaturvedi SK, Ahmad E, Khan JM, et al. Elucidating the interaction of limonene with bovine serum albumin: a multi-technique approach. Mol Biosyst. 2015;11:307–316.
- Tian ZY, Song LN, Zhao Y, et al. Spectroscopic study on the interaction between naphthalimide-polyamine conjugates and Bovine Serum Albumin (BSA). Molecules. 2015;20:16491–16523.
- Guo XY, Lv YQ, Ye Y, et al. Polyphenol oxidase dominates the conversions of flavonol glycosides in tea leaves. Food Chem. 2021;339:128088.
- Juca MM, Cysne Filho FMS, de Almeida JC, et al. Flavonoids: biological activities and therapeutic potential. Nat Prod Res. 2020;34:692–705.