ABSTRACT
Magnolia sieboldii K. Koch is endemic to China and has high medicinal and ornamental values. However, its seed exhibits morphophysiological dormancy, and the molecular mechanisms of which are not clearly understood. To reveal the regulation mechanism of the ABA signal in seed dormancy, the M. sieboldii ABA receptor Pyrabactin Resistance 1 (PYR1) gene was cloned and analyzed. Analysis of the MsPYR1 sequence analysis showed that the full-length cDNA contained a complete open reading frame of 987 bp and encoded a predicted protein of 204 amino acid residues. The protein had a relative molecular weight of 22.661 kDa and theoretical isoelectric point of 5.01. The transcript levels of MsPYR1 were immediately upregulated at 16 DAI and then decreased at 40 DAI. The highest transcript level of MsPYR1 was found in the dry seeds, indicating that the MsPYR1 gene may play an important role in the regulation of dormancy. The MsPYR1 gene cDNA was successfully expressed in E. coli Rosetta (DE3), and the protein bands were consistent with the prediction. The Anti-MsPYR1antibody could detect the expression of MsPYR1 in M. sieboldii. The results provided a foundation for further study of the function of the MsPYR1 gene.
ABBREVIATIONS
ABA: Abscisic acid; MPD: morphophysiological; PYR1: Pyrabactin Resistance1; PYL: Pyr1-Like; RCAR: Regulatory Components of Aba Receptors; PP2C: protein phosphatases 2C; SnRK2: sucrose non-fermenting1-related protein kinase2; DAI: day after imbibition; NCBI: National Center for Biotechnology Information; BCA: Bicinchoninic acid; CDD: Conserved Domains.
Introduction
Magnolia sieboldii K. Koch is widely distributed in China, Korea, and Japan. M. sieboldii has high ornamental and medicinal values [Citation1]. The plant had been designated as the City Flower of Benxi in P.R. China and the National Flower of North Korea. Given the difficulty of natural regeneration, the population of M. sieboldii has been decreasing. M. sieboldii has been classified in the IUCN Red List because of ‘least concern’ [Citation2]. The seed dormancy type belongs to the levels of morphophysiological (MPD) [Citation3]. Abscisic acid (ABA) can affect seed germination by modulating ABA-related functional genes. However, the molecular mechanism of the MPD of the M. sieboldii seed remains unknown.
The phytohormone ABA regulates plant growth and development processes, such as seed dormancy, fruit ripening, leaf senescence, and stress resistance [Citation4]. ABA signaling is required for vegetative and reproductive growth, stomatal aperture, and transcriptional response to the hormone [Citation5]. The ABA signal transduction cascade is one of the best characterized input transmission pathways of plants at the molecular level. The ABA signaling pathway has identified a large number of proteins [Citation6]. Pyrabactin Resistance1 (PYR1)/Pyr1-Like (PYL)/ Regulatory Components of Aba Receptors (RCAR) ABA receptors, clade A type protein phosphatases 2 C (PP2C) and group III sucrose non-fermenting1-related protein kinase2 (SnRK2) subfamily are essential core components of the upstream signal transduction network that regulates ABA-responsive processes, including dormancy and germination [Citation7–10].
The PYR/PYL/RCAR ABA receptor specifically perceives ABA. The PYR/PYL/RCAR ABA receptors have identified 15 members in the tomato [Citation11] and 14 members in Arabidopsis [Citation5]. PYR1 is critical for seed germination and seedling establishment [Citation10], positive regulation of fruit ripening [Citation12], reducing vegetative growth and seed production [Citation5], inducing stomatal closing movement [Citation13], and response to dehydration stress [Citation14]. The expression of PYR1 in the seeds is higher than that in the other tissues [Citation15].
PYR1 is necessary for ABA signaling in vivo. A study has shown that ABA binds to PYR1 and inhibits PP2Cs [Citation15]. The primary objective of the present study was to clone the PYR1 gene from M. sieboldii, study its expression at different germination stages. An attempt was made to express MsPYR1 protein. The cloning, transcription, and translation analysis of the PYR1 gene provide a foundation for further study on the molecular mechanism of the ABA signal.
Materials and methods
Plant material and germination assays
M. sieboldii trees were grown at the Shenyang Botanical Garden (41° 850′ N, 123° 727′ E, 28 masl) in Shenyang, Liaoning, China. Fresh matured seeds were collected from 20 trees with average height of 7 m on 20 October 2018. The coat was removed, and the seeds were surface sterilized using potassium permanganate (0.05% [w/w]) for 15 min and rinsed thrice with sterilized distilled water. The seeds were allowed to imbibe different treatments, i.e., 10 mM ABA and water, in Petri dishes at 20°C for 48 h. Each treatment had three biological replicates of 100 seeds per Petri dish. After imbibition, the seeds were cold stratified in the dark at 4°C. Seed samples were collected at 0 h imbibition (dry seed, GZ), 0 day after imbibition (DAI) and every 8th DAI then stored at −80°C for RNA extraction.
RNA extraction and cDNA synthesis
The five key time points for seed germination were as follows: dry seed, 0 DAI, 16 DAI, 40 DAI, and 56 DAI. The seeds were collected at these time points and used for total RNA extraction. The RNA was extracted using the RNA Simple Total RNA Kit (Tiangen, Beijing, China) [Citation16]. The concentration and purity of the RNA obtained were detected using Nanodrop2000 UV electrophotometer (Thermo Scientific). The cDNA was synthesized using the GoScriptTM Reverse Transcription System (Promega).
PYR1 gene cloning and sequencing
The full length of the primers of PYR1 () was designed based on the transcriptome data by using the Primer 5 software (unpublished). The PYR1 clone was amplified using PrimeSTAR® HS DNA Polymerase (TaKaRa). RT-PCR reaction system was used in a 50 μL of reaction mixture including PrimeSTAR HS Taq (25 μL), primers (2 μL), cDNA template (1 μL), and ddH2O. The RT-PCR reaction conditions were as follows: 30 s at 98°C; 30 cycles of 10 s at 98°C, 15 s at 58°C, 1 min at 72°C; and a 10 min extension at 72°C. The PCR fragments were connected into the pMD18-T vector (TaKaRa) and sequenced by TaKaRa Co., Ltd.
Table 1. Primers used in this research
Quantitative real-time PCR (qRT-PCR)
The qRT-PCR procedure was established using the qTOWER 2.0/2.2 Real Time PCR Systems (USA). All primers are listed in . The qRT-PCR reaction system was used in a 20 μL reaction mixture including PrimeSTAR HS Taq (10 μL), primers (1.6 μL), cDNA template (2 μL), and ddH2O (6.4 μL). The qRT-PCR reaction conditions were as follows: 30 s at 95°C; and 40 cycles of 5 s at 95°C, 20 s at 60°C. The data were analyzed using the 2−ΔΔCt method described by Zhang et al [Citation17].
Bioinformatics analysis
The open reading frame (ORF) and sequence alignment were analyzed using DNAMAN version 6.0. The transmembrane regions were predicted using TMHMM 2.0 (http://www.cbs.dtu.dk/services/TMHMM/). The structural regions were predicted using the National Center for Biotechnology Information (NCBI) (https://www.ncbi.nlm.nih.gov/Structure/cdd/wrpsb.cgi). The protein motif was conducted by using the Simple Modular Architecture Research Tool (SMART) (http://smart.embl-heidelberg.de/). The phylogenetic tree was constructed using Clustalx2.1 and MEGA7.0 [Citation18].
Prokaryotic expression of the PYR1 gene
The prokaryotic expression vector pET28a-PYR1 was constructed by PCR with pMD18-T-PYR1 as template. The pET28a-PYR1 construct was transformed into E. coli Rosetta (DE3). The positive clones were screened by PCR with pET28a-F and pET28a-R as primers (). The monoclones were selected and inoculated in fresh LB liquid medium (containing 50 mg/L kanamycin) for approximately 4 h at 37°C, and the OD600 was approximately 0.6. IPTG with a final concentration of 0.5 mM was added to induce the expression of PYR1 [Citation19].
Purification of the recombinant protein
The bacterial culture solution was induced at 15°C for 15 h, and then the lysed cell was ultrasonicated. The cell lysates were loaded to the chromatographic columns with Ni IDA resin. The column was washed with 200 mL of the wash buffer containing 2 M NaCl and 15 mL of the wash buffer containing 50 mM NaCl. The proteins were eluted with 20 mL of an elute buffer containing 20 mM iminazole, then the proteins were eluted with 20 mL of another elute buffer containing 250 mM iminazole [Citation20]. Approximately 1 mg/mL of pure recombinant PYR1 was obtained.
Antibody preparation and western blot analyses
After the concentration of the Bicinchoninic acid (BCA) protein was determined, two New Zealand rabbits (2–2.5 kg) were injected subcutaneously with 400 µg, immunized once at 1.5 weeks and the process was performed four times. Blood samples were collected for detection, and the titer of the antiserum against PYR1 was determined by indirect ELISA. The titer was greater than 1:500,000, and the final blood samples were collected to prepare the antiserum. Western blot analysis was performed as described [Citation21]. After 12% SDS-PAGE electrophoresis, the gels were transferred onto a Trans-BlotTurboTM (BioRad) transfer system. Anti-PYR1 antibody (1:500) was used as the primary antibody and anti-rabbit IgG (1:5000) as the secondary antibody.
Results and discussion
Cloning and sequence analysis of MsPYR1
The full-length PYR1 cDNA was obtained from M. sieboldii seed by RT-PCR. The length of the PCR product contained a complete ORF of 615 bp and encoded a predicted protein of 204 amino acid residues (). The cDNA sequence was submitted to GenBank under the accession number MW505908.
Multiple amino-acid sequence alignment revealed homology between the predicted amino-acid sequence of MsPYR1 and other PYR1 proteins (). MsPYR1 showed an amino-acid sequence similarity of 65.22% with PtPYR1 from Populus trichocarpa, 64.65% with VvPYR1 from Vitis vinifera, 64.45% with PpPYR1 from Prunus persica, 61.95% with JcPYR1 from Jatropha curcas, 61.86% with RcPYR1 from Ricinus communis, 61.47% with QsPYR1 from Quercus suber, 61.04% with SlPYR1 from Solanum lycopersicum and 57.62% with AtPYR1 from Arabidopsis thaliana.
To evaluate the molecular evolutionary relationships among MsPYR1 and other PYR1s, the phylogenic tree was constructed using the amino acid sequence through the MEGA7.0 software. MsPYR1 was more closely related to Arabidopsis thaliana, Vitis vinifera, and especially Cinnamomum micranthum ().
Bioinformatic analysis of MsPYR1
The relative molecular weight of the MsPYR1 protein was 22.661 kDa, and the theoretical isoelectric point of the protein was 5.01. The amino acid with the highest content in the protein was Glu (9.8%), and the lowest content was Trp (0.5%) ().
Table 2. The amino acid composition of MsPYR1 gene encodes the protein
The conserved domain of the MsPYR1 protein was predicted by Conserved Domains (CDD) in the NCBI. The MsPYR1 protein had a structural domain of the PYR/PYL/RACR protein family, which in turn belonged to the START/RHO_alpha_C/PITP/Bet_v1/CoxG/CalC superfamily and contained putative hydrophobic ligand binding sites, protein interfaces, and gate structures (). The SMART analysis showed a Polyketide_cyc2 domain Leu53th to Glu194th (). The three-dimensional structure of the MsPYR1 protein was predicted using Normal model and the crystal structure of FePYR1 was selected as a template, the sequence identity between MsPYR1 protein and FePYR1 protein was 88% ().
Transcription analysis of MsPYR1 in the different stratification stages
qRT-PCR was used to analyze the transcription of MsPYR1 at different stratification stages (). The highest MsPYR1 transcript was detected in the dry seeds. During stratification, the PYR1 transcript was immediately upregulated at 16 DAI and then decreased. The lowest expression was observed at 40 DAI, during which the seed coat began to crack. Therefore, MsPYR1 may play a vital role in the imbibition and seed germination. In Arabidopsis, PYR/PYLs were also expressed at high levels in the dry seeds, which were more sensitive to ABA [Citation15]. PYR/PYLs play a crucial role in the regulation of seed dormancy and stomatal switch. Compared with the wild type, the pyr/pyls mutants were less sensitive to ABA [Citation22,Citation23]. This characteristic could explain the beginning of the cracking of the seed coat and the low expression level of MsPYR1 at 40 DAI. So, ABA is a major plant hormone involved seed germination and early seedling development [Citation24,Citation25].
Prokaryotic expression of MsPYR1 and immunoblot analysis
The recombinant plasmid PET28A-MSPYR1 was transformed into the E. coli Rosetta (DE3) cell. After induction with 0.5 mM IPTG at 37°C for 2 h, 12% SDS-PAGE electrophoresis was performed (). The results showed that after IPTG induction, a protein band appeared near 26 kDa, which was slightly larger than the theoretical molecular weight of 22.661 kDa. This characteristic may be affected by the his-tag. After expansion, the MsPYR1 protein was purified by using 6× His protein purification kit. E. coli Rosetta (DE3) is frequently used for the high expression of eukaryotic or prokaryotic proteins [Citation26,Citation27].
Figure 6. Analysis of the expression of recombinant pPID1 by SDS-PAGE Lanes 1: 0 mM IPTG induction. Lanes 2,3: 0.5 mM IPTG induction. Lan M: protein molecular weight marker
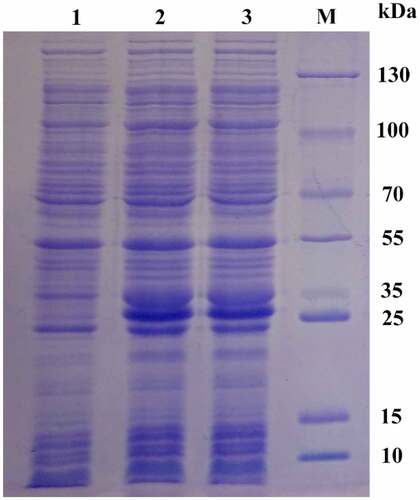
The protein was loaded into a Ni-IDA affinity column to bind the polyhistidine-tagged recombinant MsPYR1. The purity of MsPYR1 eluted from the first Ni-IDA affinity column with 20 mM imidazole was approximately 20%. After purification of the second Ni-IDA affinity column with 250 mM imidazole, MsPYR1 was approximately 80%, which was exhibited as a single band on the SDS–PAGE with the molecular weight of 26 kDa (). The expression product easily formed inclusion bodies, which increased the difficulty for protein purification [Citation28]. To avoid the formation of inclusions and enhance the soluble yield of the target protein, the expression was performed at a low temperature (15°C) [Citation29,Citation30]. In this study, the E. coli Rosetta (DE3) strain had a low expression level of the recombinant proteins as inclusion bodies ().
Figure 7. SDS–PAGE of purified recombinant protein MsPYR1 Lane 1: the precipitate of the crude cell lysate. Lan 2: the supernatant of the crude cell lysate. Lan 3: effluent fractions of loading sample. Lan 4: the fraction of the first elution with 20 mM imidazole eluate fractions. Lan 5: the fraction of the first elution with 250 mM imidazole eluate fractions. Lan 6: residual fraction. Lan M: protein molecular weight marker
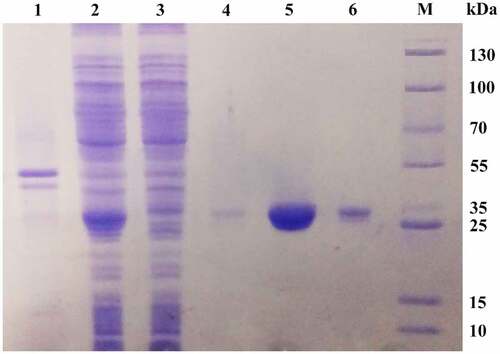
Anti-MsPYR1 antibodies are used as a convenient detection tool to bind/recognize the target proteins with higher stability and affinity. The presence of a cross-reacting protein appeared at the approximate size of 26 kDa in the purification of recombinant proteins and seed protein during the immunoblot analysis (). When the primary antibody was diluted 500 times, the recombinant protein (2 ng) and seed protein 100 (µg) could be detected by the assay.
Conclusions
In the present study, an ABA receptor gene, MsPYR1 was cloned and successfully expressed in E. coli. The expression pattern of MsPYR1 at different stratification stages was analyzed using qPCR. The highest transcript level of MsPYR1 was found in the dry seeds. The pure recombinant MsPYR1 protein was successfully obtained. The Anti-MsPYR1 antibody could detect the expression of MsPYR1 in M. sieboldii. The results provided a foundation for further study of the function of the MsPYR1 gene. The results of present study provide valuable information for further functional studies of MsPYR1 gene.
Acknowledgements
We thank the anonymous reviewers for valuable suggestions. We thank Dalian Takara Biomedical Technology Co. Ltd. for the technology support.
Disclosure statement
No potential conflict of interest was reported by the author(s).
Additional information
Funding
References
- Nan L, Nam -H-H, Park J-C, et al. Ethanol extract of Magnolia sieboldii buds ameliorated esophageal tissue injury induced by gastric acid reflux in rats via regulating the nuclear factor-κB signaling pathway. Pharmacogn Mag. 2020;16:161.
- KhelaS. Magnolia sieboldii. The IUCN red list of threatened species. 2014; e.T194003A2293976.(www.iucnredlist.org)
- Lu X-J, Zhang X-L, Mei M, et al. Proteomic analysis of Magnolia sieboldii K. Koch seed germination. J Proteom. 2016;133:76–85.
- Gupta MK, Lenka SK, Gupta S, et al. Agonist, antagonist and signaling modulators of ABA receptor for agronomic and post-harvest management. Plant Physiology and biochemistry. 2020;148:10–25.
- Gonzalez-Guzman M, Pizzio GA, Antoni R, et al. Arabidopsis PYR/PYL/RCAR receptors play a major role in quantitative regulation of stomatal aperture and transcriptional response to abscisic acid. The Plant Cell. 2012;24:2483–2496.
- Chen K, Li GJ, Bressan RA, et al. Abscisic acid dynamics, signaling, and functions in plants. Journal of Integrative Plant Biology. 2020;62:25–54.
- Cutler SR, Rodriguez PL, Finkelstein RR, et al. Abscisic acid: emergence of a core signaling network. Annu Rev Plant Biol. 2010;61:651–679.
- Fujii H, Chinnusamy V, Rodrigues A, et al. In vitro reconstitution of an abscisic acid signalling pathway. Nature. 2009;462:660–U138.
- Umezawa T, Nakashima K, Miyakawa T, et al. Molecular basis of the core regulatory network in ABA responses: sensing, signaling and transport. Plant Cell Physiol. 2010;51:1821–1839.
- Umezawa T, Sugiyama N, Mizoguchi M, et al. Type 2C protein phosphatases directly regulate abscisic acid-activated protein kinases in Arabidopsis. Proc Natl Acad Sci U S A. 2009;106:17588–17593.
- González-Guzmán M, Rodríguez L, Lorenzo-Orts L, et al. Tomato PYR/PYL/RCAR abscisic acid receptors show high expression in root, differential sensitivity to the abscisic acid agonist quinabactin, and the capability to enhance plant drought resistance. Journal of Experimental Botany. 2014;65:4451–4464.
- Chai Y-M, Jia H-F, Li C-L, et al. FaPYR1 is involved in strawberry fruit ripening. J Exp Bot. 2011;62:5079–5089.
- Park E, Kim T-H. Production of ABA responses requires both the nuclear and cytoplasmic functional involvement of PYR1. Biochem Biophys Res Commun. 2017;484:34–39.
- Fan W, Zhao M, Li S, et al. Contrasting transcriptional responses of PYR1/PYL/RCAR ABA receptors to ABA or dehydration stress between maize seedling leaves and roots (vol 16, 99, 2016). BMC Plant Biol. 2018;18. DOI:10.1186/s12870-018-1283-8.
- Park S-Y, Fung P, Nishimura N, et al. Abscisic acid inhibits type 2C protein phosphatases via the PYR/PYL family of START proteins. Science. 2009;324:1068–1071.
- Hou Z, Lu X-J, Zhang X-L, et al. Selection and optimization of total RNA extraction methods from Magnolia sieboldii different tissues. Journal of Central South Univerdity of Forestry & Technology. 2016;36:109–115.
- Zhang B, Zhang H, Chen Q, et al. Molecular cloning and analysis of a receptor-like promoter of Gbvdr3 gene in sea island cotton. Genet Mol Res. 2016;15:gmr. 15028636.
- Sun J, Wang S, Wang X, et al. Cloning and expression analyses of a cellobiohydrolase gene from Auricularia heimuer. Biotechnol Biotechnol Equip.2019;33:1327–1334.
- Liu Z, Sun T, Wang S, et al. Cloning, molecular properties and differential expression analysis of the isopentenyl diphosphate isomerase gene in Sanghuangporus baumii. Biotechnol Biotechnol Equip. 2020;34:623–630.
- Chen X, Huang Z, Zhou B, et al. Expression and purification of porcine Akirin2 in Escherichia coli. Turk J Biol. 2014;38:339–345.
- Zhang X-L, Qi M-F, Xu T, et al. Proteomics profiling of ethylene-induced tomato flower pedicel abscission. J Proteomics. 2015;121:67–87.
- Gonzalez-Guzman M, Pizzio GA, Antoni R, et al. Arabidopsis PYR/PYL/RCAR receptors play a major role in quantitative regulation of stomatal aperture and transcriptional response to abscisic acid. Plant Cell. 2012;24:2483–2496.
- Bueso E, Rodriguez L, Lorenzo-Orts L, et al. The single-subunit RING-type E3 ubiquitin ligase RSL1 targets PYL4 and PYR1 ABA receptors in plasma membrane to modulate abscisic acid signaling. Plant J. 2014;80:1057–1071.
- Gao Y, Zeng Q, Guo J, et al. Genetic characterization reveals no role for the reported ABA receptor, GCR2, in ABA control of seed germination and early seedling development in Arabidopsis. Plant J. 2007;52:1001–1013.
- Kim H, Hwang H, Hong J-W, et al. A rice orthologue of the ABA receptor, OsPYL/RCAR5, is a positive regulator of the ABA signal transduction pathway in seed germination and early seedling growth. J Exp Bot. 2012;63:1013–1024.
- Huang M, Hu Y, Liu X, et al. Arabidopsis LEAFY COTYLEDON1 mediates postembryonic development via interacting with PHYTOCHROME-INTERACTING FACTOR4. The Plant Cell. 2015;27:3099–3111.
- Wang H, Chen X, Huang Z, et al. Expression and purification of porcine PID1 gene in Escherichia coli. Turk J Biol. 2014;38:523–527.
- Mukhija R, Rupa P, Pillai D, et al. High-level production and one-step purification of biologically active human growth hormone in Escherichia coli. Gene. 1995;165:303–306.
- Fathi-Roudsari M, Akhavian-Tehrani A, Maghsoudi N. Comparison of three Escherichia coli strains in recombinant production of reteplase. Avicenna J Med Biotechnol. 2016;8:16–22.
- Wang LN, Yu B, Han GQ, et al. Design, expression and characterization of recombinant hybrid peptide attacin-thanatin in Escherichia coli. Mol Biol Rep. 2010;37:3495–3501.