ABSTRACT
Lactate blunts the anticancer immune response in breast cancer (BC). However, little is known about the exact effect of lactate transporters such as monocarboxylate transporter 4 (MCT4) on immunotherapy. In this study, we investigated the expression status and prognostic value of MCT4 in BC through large-scale transcriptome data. Our results showed that MCT4 was overexpressed in BC, particularly in the basal-like molecular subtype. Overexpression of MCT4 was significantly correlated with high BC lesion grade and poor prognosis. Enrichment analysis indicated that the MCT4-related genes were involved in immune- and metabolism-related bioprocesses, such as myeloid leukocyte activation, the adaptive immune system, and catabolic process. We also found that the expression of MCT4 in BC lesions was associated with immune cell infiltration and glycolytic rate-limiting enzymes like pyruvate kinase M2 (PKM2) and hexokinases-3 (HK3). Our observations indicate that MCT4 may play a pivotal role in the maintenance of the tumor immune microenvironment (TIME) through metabolic reprogramming. The enzymes of the glycolysis pathway (MCT4, PKM2, and HK3) may thus serve as new targets to modulate the TIME and enhance immunotherapy efficiency.
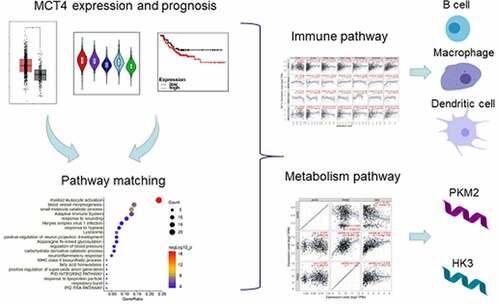
1. Introduction
Breast cancer (BC) is the most common malignant disease worldwide and estimated to account for approximately 30% of new cancer cases and 15% of cancer deaths[Citation1]. In recent years, breakthroughs in the field of immunotherapy have provided new approaches for BC treatment [Citation2,Citation3]. Programmed death ligand 1 (PD-L1) [Citation4] interacting with Programmed cell death 1 (PD1) to induce an immunosuppressive effect [Citation5] has been the focus of immunotherapy. The Food and Drug Administration (FDA) has granted approval of PD-L1 inhibitor atezolizumab when combined with nanoparticle albumin-bound (nab)-paclitaxel for unresectable locally advanced or metastatic triple negative breast cancer (TNBC) [Citation6], based on the results of the IMpassion130 trial [Citation7,Citation8]. However, some BC patients, even some TNBC patients with positive PD-L1 expression, are refractory to current immunotherapy [Citation9]. These results indicate that the suppressive tumor immune microenvironment (TIME) in BC may not be completely reversed from the combination of PD-L1 inhibitor and chemotherapy. Thus, it is important to investigate the regulation mechanism of TIME in BC patients and to find new targets that will enhance the efficiency of immunotherapy.
Most BC cells exhibit distinct metabolic characteristics that promote their proliferation and progression, which is called metabolic reprogramming [Citation10]. An altered metabolism has profound effects on the tumor microenvironment [Citation11]. For example, high levels of glycolysis, regardless of oxygen concentration, result in increased lactate production and accumulation [Citation12,Citation13]. The tumor-produced lactate is internalized by cytotoxic T cells (CTLs) and inhibits their proliferation and anticancer function through the suppression of p38 and JNK/c-Jun activation, which is required for IFN-γ production [Citation14]. The lactate can also polarize macrophages toward a tumor-promoting M2-phenotype and can exert the immunosuppressive function by activating G-protein-coupled receptor 132. Moreover, the anticancer immune response of dendritic cells (DCs) can be inhibited by lactate by inhibiting the differentiation of monocytes into dendritic cells [Citation14]. Therefore, the elevated level of lactate is not only a by-product of BC glycolysis but also blunts the anticancer immune response in a concentration-dependent manner and plays a critical role in TIME modulation.
To maintain a high lactate level, monocarboxylate transporters (MCTs), particularly MCT1 and MCT4, make significant contributions [Citation15]. The MCTs are encoded by the SLC16A gene family and function as a gate for the transport of lactate and other monocarboxylates [Citation16,Citation17]. However, only four proteins encoded by gene members of the SLC16A family are well studied and have known functions, namely, MCT1 (SLC16A1), MCT4 (SLC16A3), MCT2 (SLC16A7), and MCT3 (SLC16A8) [Citation18,Citation19]. Among MCTs, MCT1 and MCT4 are overexpressed in cancer and are related to poor prognosis [Citation20–22]. However, the relationship among MCTs, TIME, and prognosis of BC patients is still unclear. Furthermore, some enzymes in the glycolysis pathway, such as pyruvate kinase and hexokinase, also regulate the production of lactate and might also exert an immune modulation effect.
To provide new insight into the potential function of MCTs and related enzymes in the TIME modulation of BC patients, the expression status and prognostic value of MCTs in BC were systematically investigated through large-scale transcriptome data involving more than 10,000 samples. In addition, the enrichment analysis to determine the MCT4-related bioprocesses was conducted. The association among MCT4, immune infiltration, and other glycolysis enzymes was also analyzed. This is the first comprehensive study to characterize MCT4 expression in BC patients from molecular, immune, and clinical aspects.
2. Materials and methods
2.1 Oncomine 4.5 database
The Oncomine 4.5 database (https://www.oncomine.org), with 715 datasets and 86,733 samples, is a comprehensive analysis platform for diverse cancers research from gene level, among which breast cancer accounts for the largest proportion containing 132 datasets with 14,277 samples in total [Citation23]. Here, it served to discover the expression statues of MCT1 and MCT4 and to compare their expressive differences between normal tissues and cancers. The data of mRNA expression and DNA copy number of MCT1 and MCT4 in all types of cancers were investigated. ‘SLC16A1’ (MCT1) and ‘SLC16A3’ (MCT4) were input as key search words respectively with thresholds set as following: p = 1-E4, fold change = 2, gene rank = top 10%.
2.2 TIMER 2.0 database
The TIMER 2.0 database (https://cistrome.shinyapps.io/timer/) supplies multiple analysis methods from the immune aspect [Citation24]. In this article, it was employed to assess the correlation of MCT4 expression with immune infiltration in different subtypes of BC. The immune cells included B cells, CD8 + T cells, CD4 + T cells, macrophages, neutrophils, and dendritic cells. It was also applied to question the relationship of MCT4, HK3 and PKM2.
2.3 Bc-genExMiner v4.5 database
bc-GenExMiner v4.5 database (http://bcgenex.centregauducheau.fr) is a systematic analysis tool for breast cancer transcriptomic data, including 10,716 DNA microarrays and 4712 RNA-seq [Citation25]. In this study, the ‘expression’ module was applied to investigate gene expression profiles in different subtypes of BC using DNA microarray datasets (n = 10,001) and RNA-Seq datasets (n = 4712) respectively. Moreover, DNA microarrays data were explored in the ‘prognostic’ module to statistically identify the prognostic value of MCT4 for BC.
2.4 GOBO 1.0.3 database
The GOBO database (http://co.bmc.lu.se/gobo) is another analysis tool for breast cancer with 1881-samples [Citation26]. It is designed in three different modules, data set module, web interface module and analysis module. Through the ‘Tumors’ selection in the analysis module, the expression levels of (SLC16A3) MCT4, hexokinase-3 (HK3) and pyruvate kinase M2 (PKM2), in all subtypes of BC were studied when they were input as gene symbols respectively. In this analysis, no multivariate parameters were (ER-status, Node status, Grade stratified, Age stratified, Size stratified) selected.
2.5 Kaplan-meier plotter database
The Kaplan-Meier plotter (http://kmplot.com) allows the acquirement of the effect of 70,632 genes on survival in 21 caners, especially for breast cancer (n = 6,234) [Citation27]. Here, Kaplan-Meier survival curves supplied the access to investigate MCT4’s prognosis value for BC. In accordance with the lower quartile expression value, BC patients were split into high-MCT4 expression group and low-MCT4 expression group. Then the relationship of MCT4 expression and the relapse-free survival (RFS) (n = 3955) was obtained with the calculation of hazard ratio with 95% confidence intervals, and log-rank P value.
2.6 The metascape database
The Metascape Database (https://metascape.org) is a resource for gene annotation and analysis with its data updating monthly [Citation28]. This tool was employed to study the biological process of MCT4 using the 100 most relevant genes of MCT4 in BC from the LinkedOmics database according to the Pearson correlation test. The genes were served for enrichment analysis in three modules (merged analysis, GO analysis, and KEGG analysis).
3. Results
As the overexpressed lactate exporter in BC, MCT4 is a potential target for modulating the TIME and improving immune therapy in BC patients. In this study, a comprehensive analysis was performed to investigate MCT4 expression signatures based on the largest transcriptome dataset available. The MCT4 level was associated with immune cell infiltration and prognosis of BC. In addition, the correlation between MCT4 and other main enzymes in the glycolysis pathway (PKM2, HK3) in BC were also characterized.
3.1 Expression signatures of MCT1 and MCT4
First, the expression profiles of MCT1 and MCT4 in tumor and adjacent normal tissues were assessed using the Oncomine database (83 DNA datasets and 572 mRNA datasets, ). MCT1 and MCT4 expressions were higher in most cancers. Remarkably, 16 analyses exhibited MCT4 overexpression in BC, while no analysis demonstrated upregulation of MCT1. Next, this study analyzed the difference in gene expression between BC and normal tissues based on The Cancer Genome Atlas (TCGA) and GTEx datasets. MCT4 exhibited significantly higher expression in BC than in normal tissues, while no marked difference was observed in MCT1 expression (). These results indicate that MCT4, not MCT1, has the potential to predict BC prognosis.
3.2 MCT4 expression profile in BC
As investigated above, MCT4 was overexpressed in BC patients. Accordingly, the expression signature of MCT4 in BC was further explored using GOBO and bc-GenExMiner v4.5. First, GOBO (n = 1881) was applied to determine the expression levels of MCT4 in various clinical subtypes and stages (). The overexpression of MCT4 was observed in basal-like, human epidermal growth factor receptor 2 (HER2)-enriched, and luminal B subtypes. Higher grade BC tended to have higher MCT4 expression. No association was observed between MCT4 expression and ER status. Then, bc-GenExMiner v4.5 () was used to further show the phenotype of MCT4. In the merged microarray datasets (n = 10,001), MCT4 expression was also higher in basal-like and luminal B subtypes. In addition, MCT4 expression was higher in progesterone receptor (PR)- and HER2+ groups than in PR+ and HER2- groups. Moreover, upregulation of MCT4 was associated with a higher Nottingham Prognostic Index (NPI) and Scarff-Bloom-Richardson (SBR) grade. However, the ER- BC group expressed higher levels of MCT4 than the ER+ BC group, which was inconsistent with the GOBO database analysis. The inconsistency perhaps resulted from the smaller amounts of samples in the GOBO database. Those are consistent with early reports suggesting that triple-negative breast cancer and high-grade disease were correlated with MCT4 expression [Citation29,Citation30]. Finally, the merged RNA-Seq datasets (n = 4712) were investigated to make the expression profile of MCT4 more credible (). As expected, the results were in line with that of merged microarray datasets. Collectively, these results show that MCT4 was overexpressed in HER2-enriched and basal-like BC patients.
Figure 2. The expression pattern of MCT4 in different subtypes of BC using the GOBO dataset (n = 1881)
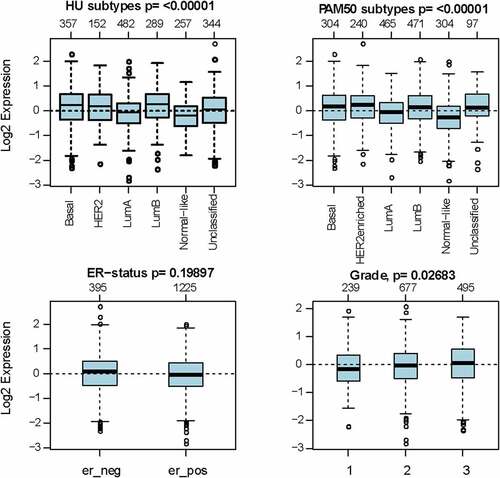
Figure 3. The expression profile of MCT4 in different subtypes of BC using the merged microarray datasets (n = 10,001)
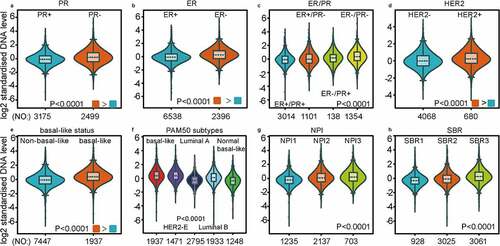
Figure 4. MCT4 expression signature from RNA-seq level exploiting merged RNA-seq datasets (n = 4712)
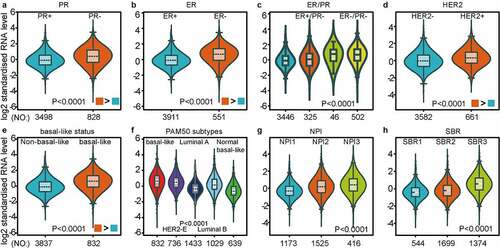
3.3 Relationship between MCT4 Expression and BC patient prognosis
Encouraged by the results above, Kaplan–Meier analyses were conducted to explore the influence of MCT4 on patient prognosis. Among the immunophenotypes, higher MCT4 expression predicted worse relapse-free survival (RFS) (n = 3955) in PR+, PR-, ER+, ER-, and HER2- BC but not in HER2+ BC. Among intrinsic subtypes, MCT4 overexpression was associated with poor RFS in luminal A and B subtypes but not in basal-like subtypes (). To validate the prognostic value of MCT4 in BC, this study further performed the prognostic analysis of MCT4 in the largest BC transcriptomic dataset, the bc-GenExMiner v4.5 database, using data from its DNA microarrays. Higher MCT4 expression was significantly accompanied by worse disease-free survival (DFS) (n = 8849) in BC, especially for PR+, PR-, ER+, and ER- BC. In contrast, among intrinsic subtypes, MCT4 overexpression predicted poor DFS in both HER2-enriched and basal-like subtypes (). These results suggest that MCT4 is strongly related to the prognosis of HER2-enriched and basal-like subtypes in BC patients.
Figure 5. The kaplan-meier survival curves of high and low MCT4 expression in BC through the kaplan-meier plotter database. the RFS in (a) PR+ BC, (b) PR- BC, (c) ER+ BC, (d) ER- BC, (e) HER2+ BC, (f) HER2- BC, (g) basal-like subtype, (h) luminal A subtype, (i) luminal B subtype. RFS: relapse-free survival; HR: hazard ratio
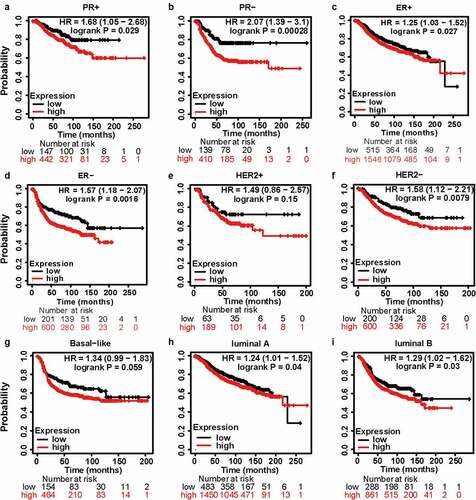
Figure 6. The kaplan-meier survival curves of high and low MCT4 expression in BC through the bc-GenExMiner v4.5
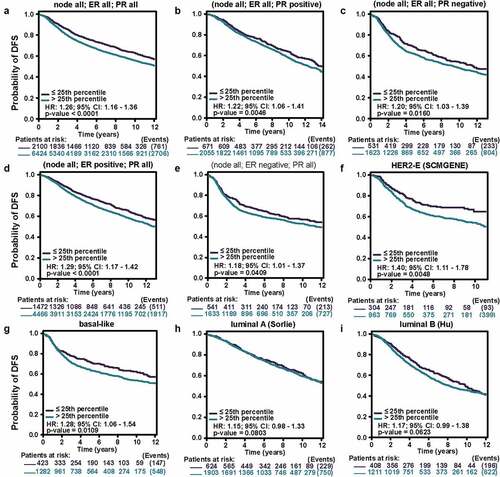
3.4 Related biological processes of MCT4
To further investigate the function of MCT4 in BC, the biological activities that involved MCT4 were explored. Through the LinkedOmics (http://www.linkedomics.org) database, the 100 most relevant genes of MCT4 in BC were screened from the TCGA database by Pearson’s correlation analysis (Pearson R > 0.45, Table S1). Then, enrichment analyses (), Gene Ontology (GO), and Kyoto Encyclopedia of Genes and Genomes (KEGG) analysis (Figure S1) were conducted. The results indicate the MCT4-related genes participate in immune responses including myeloid leukocyte activation, adaptive immune system, and major histocompatibility complex (MHC) class II biosynthetic process, as well as metabolism-related bioprocess such as small molecule catabolic process, the carbohydrate derivative catabolic process, and fatty acid homeostasis. These results demonstrate that MCT4 affected not only the cancer metabolism but also the TIME.
3.5 Correlation between MCT4 expression and immune infiltrates in BC
The TIMER database was used to probe the correlation between immune infiltration and MCT4 expression in BC (). The expression of MCT4 was positively correlated with dendritic cell infiltration in all BC patients (correlation = 0.351), especially in the basal-like subtype (correlation = 0.316) and luminal subtype (correlation = 0.317). Moreover, macrophage infiltration exhibited a positive correlation (the correlation coefficient was 0.328) with MCT4 expression in the HER2+ subtype while B-cell infiltration showed a negative correlation (the correlation coefficient was −0.385). These results imply that MCT4 expression in HER2-enriched and basal-like BC patients is related to immune infiltration, which is critical to the success of immune therapy.
3.6 Correlation between MCT4 Expression and carbon metabolism-related enzymes
Given that MCT4 engaged in metabolic reprogramming, the PathCards database was searched, and 70 genes in the central carbon metabolism pathway in cancer were obtained (Table S2). Interestingly, two enzymes, HK3 and PKM2, existed in both MCT4-associated genes and central carbon metabolism-related genes (). Afterward, the study explored the relationship between MCT4, HK3 and PKM2 with tumor purity in the TIMER database. As exhibited in , MCT4 was related to HK3 (partial.cor = 0.337) and PKM2 (partial.cor = 0.615), while HK3 and PKM2 did not show a significant correlation. The GOBO database was then applied to determine the expression statuses of HK3 and PKM2 (). As expected, HK3 and PKM2 were both overexpressed in basal-like and HER2-enriched molecular groups, and ER- patients had higher HK3 and PKM2 expression than ER+ patients. Higher HK3 or PKM2 expression was also accompanied by a higher tumor grade.
4. Discussion
This study investigated the expression profile of MCT4 in BC, involving more than 10,000 samples and multiple verifications. These results showed that MCT4 was overexpressed in BC patients and was related to poor prognosis, particularly for HER2-enriched and basal-like subtypes. Moreover, MCT4 expression was related to immune infiltration in these two subtypes. Recent studies [Citation31] have reported that higher levels of [Citation13]C label exchange via MCT in more aggressive BC such as TNBC, which agrees with the observed MCT4 overexpression in basal-like subtype. Studies have also reported the potential of MCT4 as a new anticancer therapeutic target and prognostic indicator in other cancers, such as colorectal cancer [Citation32] and prostate cancer [Citation33]. Thus, it is anticipated that MCT4 will be a promising therapeutic target for BC, especially for TNBC. In fact, MCT4 has been demonstrated to be an important regulator of BC cell survival [Citation29]. MCT4 inhibition caused intracellular lactate accumulation, increased reactive oxygen species levels, and induced cancer cell apoptosis [Citation34,Citation35].
The enrichment analysis found that MCT4-related genes were involved not only in metabolic but also in immune-related biological processes and pathways, such as myeloid leukocyte activation, the adaptive immune system, and catabolic process. MCT4 expression was significantly related to immune infiltration in BC. The TIMER database showed that MCT4 expression was related to dendritic cell infiltration in all BC patients (correlation = 0.351), especially in basal-like subtypes (correlation = 0.316). Macrophages and B-cell infiltration were correlated with MCT4 expression in HER2+ subtypes (the correlation coefficients were 0.328 and −0.385, respectively). These findings show moderate correlations among MCT4 expression, macrophages, B-cells, and dendritic cells. A recent study has reported that downregulation of MCT4 promotes the cytotoxicity of NK cells in BC [Citation36], suggesting that MCT4 is involved in suppressive TIME. Another study has demonstrated that MCT4 is responsible for the immunosuppression effect attributed either to abrogating macrophage maturity or to disturbing T cell metabolism [Citation37]. These results indicate that MCT4 modulates the TIME and has the potential to enhance the efficiency of immunotherapy in BC.
A significant correlation between MCT4 and rate-limiting enzymes in glycolysis (PKM2 and HK3, partial.cor = 0.337 and 0.615, respectively) was also observed. More importantly, these enzymes were also similar in expression and prognosis patterns as explored in the GOBO database. The results show that both HK3 and PKM2 are overexpressed in basal-like and HER2-enriched molecular groups, and ER- patients have higher HK3 and PKM2 expression than ER+ patients. Furthermore, higher HK3 or PKM2 expression was accompanied by a higher tumor grade.
PKM2 catalyzes the rate-limiting step of glycolysis and is explicitly expressed in various tumor cells [Citation15]. The exact mechanism of PKM2 in regulating TIME is still under investigation [Citation38]. A recent study has reported that TEPP-46, a well-characterized allosteric activator of PKM2, inhibits the pathogenicity of macrophages, DCs, T cells, and tumor cells [Citation39,Citation40], suggesting that inhibition of PKM2 has the potential to influence the TIME. As the first rate-limiting enzymes of glycolysis, HKs act in various cells (such as tumor and immune cells) [Citation41]. Large pooled analyses were performed in HK2 [Citation42], but few studies shed light on the HK3 mechanism. A previous study has showed that HK3 has a stronger relationship with tumor [Citation17]F-FDG uptake than HK2, indicating its role in the activation of aerobic glycolysis [Citation43]. Recently, researchers have also found that HK3 expression in tumor tissues may pertain to immune status [Citation44], suggesting its capability to regulate the TIME via metabolic reprogramming, similar to MCT4 and PKM2.
There are several limitations in the study. First, all the data were retrieved from online databases, and the derived conclusion needs to be verified by external in vitro/in vivo experiments. Second, the prognosis value of MCT4 relative to overall survival of BC patients was not evaluated due to insufficient data. Finally, although the study showed MCT4, PKM2, and HK3 were associated with immune infiltration of BC patients, the specific mechanisms of how these enzymes modulate the TIME need further investigation.
5. Conclusion
In summary, MCT4 expression was observed to be associated with aggressive BC and poor prognosis. Our data also suggested that MCT4-related genes were involved in metabolic- and immune-related biological processes. MCT4 expression was consistent with immune cell infiltration in BC; therefore, MCT4 might be a promising target for anticancer application. Additionally, we propose that the main enzymes in the glycolysis pathway (MCT4, PKM2, and HK3) may function together by modulating TIME and enhancing the efficiency of anticancer immunotherapy in invasive BC, especially in HER2-enriched BC and TNBC.
6. HIGHLIGHTS
MCT4 was overexpressed in breast cancer, especially in the basal-like subtype.
MCT4-related genes were involved in immune and metabolism related bioprocesses.
MCT4 expression was associated with the immune cells and other glycolysis enzymes.
Supplemental Material
Download ()Disclosure statement
The authors declare that the research was conducted in the absence of any commercial or financial relationships that could be construed as a potential conflict of interest.
Supplementary material
Supplemental data for this article can be accessed here
Additional information
Funding
References
- Siegel RL, Miller KD, Fuchs HE, et al. Cancer Statistics, 2021. CA A Cancer J Clin. 2021;71(1):7–33.
- How CW, Ong YS, Low SS, et al. How far have we explored fungi to fight cancer? Semin Cancer Biol. 2021;S1044579X21000596. DOI:10.1016/j.semcancer.2021.03.009
- Shin Low S, Pan Y, Ji D, et al. Smartphone-based portable electrochemical biosensing system for detection of circulating microRNA-21 in saliva as a proof-of-concept. Sens Actuators B Chem. 2020;308:127718.
- Kwa MJ, Adams S. Checkpoint inhibitors in triple-negative breast cancer (TNBC): where to go from here: checkpoint Inhibitors in TNBC. Cancer. 2018;124(10):2086–2103.
- Zheng Y, Yang Y, Wu S, et al. MPDL3280A with adoptive cell immunotherapy exerts better antitumor effects against cervical cancer. Bioengineered. 2017;8(4):367–373.
- Schmid P, Rugo HS, Adams S, et al. Atezolizumab plus nab-paclitaxel as first-line treatment for unresectable, locally advanced or metastatic triple-negative breast cancer (IMpassion130): updated efficacy results from a randomised, double-blind, placebo-controlled, phase 3 trial. Lancet Oncol. 2020;21(1):44–59.
- Reddy SM, Carroll E, Nanda R. Atezolizumab for the treatment of breast cancer. Expert Rev Anticancer Ther. 2020;20(3):151–158.
- Cortés J, André F, Gonçalves A, et al. IMpassion132 Phase III trial: atezolizumab and chemotherapy in early relapsing metastatic triple-negative breast cancer. Future Oncol. 2019;15(17):1951–1961.
- Quintela-Fandino M, Holgado E, Manso L, et al. Immuno-priming durvalumab with bevacizumab in HER2-negative advanced breast cancer: a pilot clinical trial. Breast Cancer Res. 2020;22(1):124.
- Wan M, Zhuang B, Dai X, et al. A new metabolic signature contributes to disease progression and predicts worse survival in melanoma. Bioengineered. 2020;11(1):1099–1111.
- Pavlova NN, Thompson CB. The emerging hallmarks of cancer metabolism. Cell Metab. 2016;23(1):27–47.
- Vander Heiden MG, Cantley LC, Thompson CB. Understanding the Warburg Effect: the metabolic requirements of cell proliferation. Science. 2009;324(5930):1029–1033.
- Baltazar F, Afonso J, Costa M, et al. Lactate beyond a waste metabolite: metabolic affairs and signaling in malignancy. Front Oncol. 2020;10:231.
- Certo M, Tsai C-H, Pucino V, et al. Lactate modulation of immune responses in inflammatory versus tumour microenvironments. Nat Rev Immunol. 2021;21(3):151–161.
- Poole RC, Halestrap AP. Transport of lactate and other monocarboxylates across mammalian plasma membranes. Am J Physiol Cell Physiol. 1993;264(4):C761–82.
- Halestrap AP, The MD. SLC16 gene family from monocarboxylate transporters (MCTs) to aromatic amino acid transporters and beyond. Pflugers Archiv Eur J Physiol. 2004;447(5):619–628.
- Sonveaux P, Végran F, Schroeder T, et al. Targeting lactate-fueled respiration selectively kills hypoxic tumor cells in mice. J Clin Invest. 2008;118:3930–3942. doi:10.1172/JCI36843.
- Felmlee MA, Jones RS, Rodriguez-Cruz V, et al. Monocarboxylate Transporters (SLC16): function, regulation, and role in health and disease. Pharmacol Rev. 2020;72(2):466–485.
- Yu S, Wu Y, Li C, et al. Comprehensive analysis of the SLC16A gene family in pancreatic cancer via integrated bioinformatics. Sci Rep. 2020;10(1):7315.
- Li Z, Wu Q, Sun S, et al. Monocarboxylate transporters in breast cancer and adipose tissue are novel biomarkers and potential therapeutic targets. Biochem Biophys Res Commun. 2018;501(4):962–967.
- de Oliveira ATT, Pinheiro C, Longatto-Filho A, et al. Co-expression of monocarboxylate transporter 1 (MCT1) and its chaperone (CD147) is associated with low survival in patients with gastrointestinal stromal tumors (GISTs). J Bioenerg Biomembr. 2012;44(1):171–178.
- Contreras-Baeza Y, Sandoval PY, Alarcón R, et al. Monocarboxylate transporter 4 (MCT4) is a high affinity transporter capable of exporting lactate in high-lactate microenvironments. J Biol Chem. 2019;294(52):20135–20147.
- Rhodes DR, Yu J, Shanker K, et al. ONCOMINE: a cancer microarray database and integrated data-mining platform. Neoplasia. 2004;6(1):1–6.
- Li T, Fan J, Wang B, et al. TIMER: a web server for comprehensive analysis of tumor-infiltrating immune cells. Cancer Res. 2017;77(21):e108–10.
- Jézéquel P, Gouraud W, Ben Azzouz F, et al. bc-GenExMiner 4.5: new mining module computes breast cancer differential gene expression analyses. Database. 2021;2021:007.
- Ringnér M, Fredlund E, Häkkinen J, et al. GOBO: gene expression-based outcome for breast cancer online. PLoS ONE. 2011;6(3):e17911.
- Á N, Munkácsy G, Győrffy B. Pancancer survival analysis of cancer hallmark genes. Sci Rep. 2021;11(1):6047.
- Zhou Y, Zhou B, Pache L, et al. Metascape provides a biologist-oriented resource for the analysis of systems-level datasets. Nat Commun. 2019;10(1):1523.
- Baenke F, Dubuis S, Brault C, et al. Functional screening identifies MCT4 as a key regulator of breast cancer cell metabolism and survival: MCT4 supports breast cancer cell survival. J Pathol. 2015;237(2):152–165.
- Xiao S, Zhu H, Shi Y, et al. Prognostic and predictive value of monocarboxylate transporter 4 in patients with breast cancer. Oncol Lett. 2020;20(3):2143–2152.
- Gallagher FA, Woitek R, McLean MA, et al. Imaging breast cancer using hyperpolarized carbon-13 MRI. Proc Natl Acad Sci USA. 2020;117(4):2092–2098.
- Brown TP, Ganapathy V. Lactate/GPR81 signaling and proton motive force in cancer: role in angiogenesis, immune escape, nutrition, and warburg phenomenon. Pharmacol Ther. 2020;206:107451.
- Choi SYC, Xue H, Wu R, et al. The MCT4 Gene: a novel, potential target for therapy of advanced prostate cancer. Clin Cancer Res. 2016;22(11):2721–2733.
- Todenhöfer T, Seiler R, Stewart C, et al. Selective inhibition of the lactate transporter MCT4 reduces growth of invasive bladder cancer. Mol Cancer Ther. 2018;17(12):2746–2755.
- Puri S, Juvale K. Monocarboxylate transporter 1 and 4 inhibitors as potential therapeutics for treating solid tumours: a review with structure-activity relationship insights. Eur J Med Chem. 2020;199:112393.
- Long Y, Gao Z, Hu X, et al. Downregulation of MCT4 for lactate exchange promotes the cytotoxicity of NK cells in breast carcinoma. Cancer Med. 2018;7(9):4690–4700.
- Sun X, Wang M, Wang M, et al. Role of proton-coupled monocarboxylate transporters in cancer: from metabolic crosstalk to therapeutic potential. Front Cell Dev Biol. 2020;8:651.
- Li Y, Li X, Liu J, et al. PKM2, a potential target for regulating cancer. Gene. 2018;668:48–53.
- Palsson-McDermott EM, Dyck L, Zasłona Z, et al. Pyruvate Kinase M2 Is Required for the Expression of the Immune Checkpoint PD-L1 in Immune Cells and Tumors. Front Immunol. 2017;8:1300.
- Angiari S, Runtsch MC, Sutton CE, et al. Pharmacological activation of pyruvate kinase M2 Inhibits CD4+ T cell pathogenicity and suppresses autoimmunity. Cell Metab. 2020;31(2):e8.
- Kudryavtseva AV, Fedorova MS, Zhavoronkov A, et al. Effect of lentivirus-mediated shRNA inactivation of HK1, HK2, and HK3 genes in colorectal cancer and melanoma cells. BMC Genet. 2016;17(S3):156.
- Shi T, Ma Y, Cao L, et al. B7-H3 promotes aerobic glycolysis and chemoresistance in colorectal cancer cells by regulating HK2. Cell Death Dis. 2019;10(4):308.
- van Berkel A, Rao JU, Kusters B, et al. Correlation between in Vivo18 F-FDG PET and immunohistochemical markers of glucose uptake and metabolism in pheochromocytoma and paraganglioma. J Nucl Med. 2014;55(8):1253–1259.
- Tuo Z, Zheng X, Zong Y, et al. HK3 is correlated with immune infiltrates and predicts response to immunotherapy in non‐small cell lung cancer. Clin Transl Med. 2020;10(1):319–330.