ABSTRACT
Cutaneous squamous cell carcinoma (cSCC) is a highly prevalent skin malignancy, and the effective therapy still remains a challenge. Crocin can be used for cSCC therapy. This study explored the effects of cSCC cells treatment with crocin in vitro and in vivo. The study used A431 and SCL-1 cells lines, cSCC human samples and BALB/C nude mice for investigations. Apoptosis was determined by MTT assays, while miR-320a and ATG2B expressions were validated through RT-qPCR. Interaction of miR-320a with ATG2B was examined via dual luciferase reporter assay. The autophagy and apoptosis proteins expressions were further confirmed through western blot and immunofluorescence staining assays. The results indicated a significantly upregulated miR-320a, but a down-regulated ATG2B expression in the cSCC clinical samples. Crocin significantly repressed cSCC cells growth, and induced apoptosis through autophagy. Furthermore, miR-320a expression was inhibited and ATG2B expression was increased. Dual luciferase reporter assay revealed that miR-320a regulated ATG2B expression directly. Additionally, the upregulation of ATG2B expression in cSCC cells inhibited cell proliferation and led to cell apoptosis. Crocin also reduced tumor growth and stimulated the apoptosis in vivo. In conclusion, miR-320a is upregulated and ATG2B is down-regulated in cSCC, Crocin suppresses the proliferation and induces apoptosis of cSCC cells. Further, Crocin increases autophagy while miR-320a hinders autophagy and the apoptotic effects of crocin on cSCC cells. MiR-320a binds ATG2B directly, and ATG2B expression is upregulated by crocin. Finally, Crocin triggers cSCC cells apoptosis in vivo. Crocin can target ATG2B/miR-320a and may be an effective alternative for cSCC treatment.
1. Introduction
Cutaneous SCC has a high global prevalence, and its risk factors include ultraviolet radiation (UVR), smoking, chemical exposure and immunosuppression [Citation1]. The prognosis of cSCC mainly depends on tumor size, depth, differential status, anatomical location and pathological types [Citation2]. Current management options of cSCC include surgical removal, radiotherapy, cryotherapy, curettage and electrodesiccation, chemotherapy, electrochemotherapy, targeted therapy and immunotherapy [Citation3]. Despite these advances, the failure rates of these treatments are high, and cSCC can easily recur and metastasize. Thus, exploring new approaches to treating cSCC is necessary.
Autophagy is among the essential protective cellular pathways that modulate stress-induced adaptation and control of damage. The process sequesters organelles and long-lived proteins in vesicles coated with membrane, thus referred to as autophagosomes. This mechanism of survival, initiated by different stress types, may block extrinsic and intrinsic apoptotic pathways [Citation4], and tumor cells utilizes autophagy to evade starvation and hypoxia. Interestingly, anticancer treatments such as DNA-inactivating drugs or antagonists of hormones initiates autophagy as pro-survival response [Citation5]. Consequently, the ability to modulate autophagy is key in cancer research and treatment. The role of autophagy-related 2 (ATG2) is not clearly characterized in cutaneous SCC and only a few studies have demonstrated that homologous ATG2A and ATG2B genes are involved in the formation of autophagosome in HeLa cells [Citation6]
MiRNAs are approximately 22–25 nucleotide short single-stranded non-coding RNAs that bind directly to the target messenger RNAs’ 3ʹ-untranslated regions (3ʹ-UTRs), leading to degradation of mRNA or translational suppression. Specifically, miR-320a has been identified as a metastatic suppressor in various cancers such as colorectal cancer (CRC) whereby high expression of miR-320a in CRC stage II tumors was linked with better disease-free survival in the patients with CRC [Citation7] Mechanistic investigations demonstrated that miR-320a exerts its inhibitory role by targeting various genes, eg neuropilin 1 (in CRC cells) [Citation8], guanine nucleotide-binding protein G subunit alpha-1 (in hepatocellular carcinoma) [Citation9] and aquaporin 1 and 4 (in cerebral ischemia) [Citation10]. miR-320 has also been shown to suppress Wnt/beta-catenin signaling pathway through targeting the β-catenin mRNA’s 3′-UTR [Citation11]. The miR-320a characteristics, which controls the expression of various targets, demonstrates its significance in the regulatory network for the development of diseases. Thus, identifying and characterizing its possible novel targets may provide new insights into understanding the biological and pathological roles of miR-320a in cSCC.
Crocin is one of the constituents of saffron and has diverse pharmacological effects, such as anti-oxidant, anti-inflammatory, and anti-depressant effects [Citation12]. Further, Crocin can inhibit the growth of cancer cells, and enhance tumor’s sensitivity to chemotherapy or radiation. Previous study also reported the effectiveness of crocin in reducing migration, invasion, and epithelial–mesenchymal transition in gastric cancers through miR-320/KLF/HIF1α axis, [Citation13]. Similarly, crocin has also been reported to trigger apoptosis in breast carcinoma cells [Citation14].
In relation to autophagy, crocin has been reported to exert the apoptotic effects on various malignancies, such as hepatocellular carcinoma [Citation15], and cervical cancer, via promoting autophagic apoptosis [Citation16]. However, studies reporting the use of crocin against cSCC are entirely nonexistent. The study hypothesized that Crocin plays anti-proliferative and apoptotic roles on cutaneous squamous cell carcinoma via miR-320a/ATG2B. This study aimed at assessing the expression of MiR-320a and ATG2B in human cutaneous squamous cell carcinoma, understanding the effects of Crocin in proliferation and apoptosis of cSCC cells. Further, the study aimed at determining the effects of Crocin and MiR-320a in autophagy in cSCC cells. Finally, the role of Crocin in cSCC cells apoptosis in vivo was investigated.
2. Materials and methods
2.1 Clinical samples
The clinical work was approved by the Ethical Committee of Zibo Central Hospital. Thirty-two confirmed cSCC patients were enrolled in the study from December 2018 to December 2019. The patients did not receive any treatment before inclusion into the study. After a written informed consent, carcinoma and adjacent normal tissues were obtained from the patients through biopsy for comparison and analysis. The diagnosis was confirmed by two experienced pathologists. The obtained samples were stored at −80°C for further analysis. Clinical and pathological features of patients were shown in .
Table 1. Clinicopathological features of cSCC patients enrolled in our study
2.2 Chemicals and Reagents
cSCC cell lines-A431 and SCL-1 cells were purchased from the American Type Culture Collection, USA. Crocin was purchased from MedChemExpress, USA, and dissolved in dimethyl sulfoxide (DMSO, Sigma–Aldrich, USA). Dulbecco’s modified Eagle’s medium (DMEM) was acquired from Sigma-Aldrich, USA. Penicillin-streptomycin, trypsin-EDTA and fetal bovine serum (FBS) were obtained from Gibco (Grand Island, NY, USA). Si-ATG2B, si-negative control (NC), mimic negative control (NC), miR-320a mimic, inhibitor negative control (NC), and miR-320a inhibitor were all purchased from Genomeditch, Shanghai, China. MTT kits were purchased from Beyotechnology, Shanghai, China.
2.3 cell culture and transfection
Two cSCC cell lines-A431 and SCL-1 cells--were cultured in Dulbecco’s modified Eagle’s medium supplemented with 10% fetal bovine serum and 1% penicillin–streptomycin (50 mg/L penicillin; 100 µg/ml streptomycin in a 6-well platesThe plates were incubated at 37°C in a humid atmosphere of 5% CO2. Approximately 3 × 105 cells per well were seeded in 6-well plates and incubated overnight. When the cells were at approximately 80% confluence, si-ATG2B, si-negative control (NC), mimic negative control (NC), miR-320a mimic, inhibitor negative control (NC), and miR-320a inhibitor were transiently transfected into the tumor cells using Lipofectamine 2000, following the manufacturer’s guidelines. After a 48 h incubation in 37°C and 5% CO2, the transfected tumor cells were used for further assays [Citation17].
2.4 Quantitative real-time PCR (qRT-PCR)
Total RNA extraction was done using Trizol reagent (Sigma–Aldrich, USA), while total miRNA was obtained by using Molpure Cell/Tissue miRNA Kit (Yeasen, Shanghai, China). In addition, mRNA was transcribed using Hifair III One Step RT-qPCR Probe Kit (Yeasen, Shanghai, China), while TaqMan MicroRNA Reverse Transcription Kit (Invitrogen, USA) was used to transcribe miRNA. Real-time PCR was done using ABI 7900 Fast PCR system (applied Biosystems) using SYBR green (Roche, Switzerland). U6 was used as endogenous control for miR-320a. The endogenous control for other primers was GAPDH. The 2−∆∆Ct method was utilized for determination of expressions of relative mRNA or miRNA [Citation18]. All the assays were plated in triplicate and repeated thrice. The primers in our experiments were listed in .
Table 2. Primers in experiments
2.5 Cell viability assay
Assessment of Cell viability was done by MTT assay. The SCL-1 and A431 cells in logarithmic phase were harvested for counting. The density of cells was adjusted to a density of 4 × 104 cells per ml by DMEM, then plated into 96-well plates and cultured at 37°C in a 5% CO2 incubator. After 12 h, the supernatant was discarded and various concentrations of crocin (concentration 0, 1, 2 and 4 mM) were added to the corresponding treatments and incubated 24 h or 48 h. Approximately 20 µl MTT solution was added to every well for 4 h and centrifuged at 4°C for 10 min at 1,750 x. The supernatant was discarded and 150 µl of DMSO was added per-well. The sample was then shaken in a shaker for 10 min and OD value was measured at 570 nm wavelength in spectrophotometer (Hitachi, Ltd., Tokyo, Japan). Each treatment was done in triplicates. The rate of Inhibition was calculated as follows: Inhibition rate (%) = (control group A – experimental group A)/control group A x100%[Citation19].
2.6 Dual luciferase reporter activity assay
The predicted ATG2B 3′-UTR sequences encompassing the binding sites of miR-320a were amplified through PCR and consequently sub-cloned into a dual-luciferase reporter to construct pMIR-GLO (Promega) forming the pMIR-GLO- ATG2B -WT reporter vector. The mutant reporter vector pMIR-GLO- ATG2B -MUT was obtained by a site-directed mutagenesis kit (New England Biolabs). A431 and SCL-1 cells were co-transfected with miR-320a mimics or scramble control and with pMIR-GLO- ATG2B-WT or MUT reporter vector using Lipofectamine 2000 (Thermo Fisher Scientific) and incubated for 48 h. luciferase activities were then assessed with the Dual-Luciferase Reporter Assay System (Promega) [Citation17]. All experiments were plated in triplicate and repeated thrice.
2.7 Immunofluorescent staining assay
A431 cells (1 × 105 cells/well) and SCL-1 cells (1 × 105 cells/well) were grown on glass slides in culture well plates and then treated with crocin (concentration 0, 1, 2 and 4 mM) and cultured for 24 h. Cells in the culture plate were washed in PBS for three times, fixed with 4% paraformaldehyde for 15 min, and re-washed thrice in PBS. Later, cells were permeabilized using Triton X-100, washed three times in PBS, blocked in 1% BSA, and incubated with rabbit anti-mouse LC3B antibody (1:100) overnight. Finally, the samples were incubated with DAPI in the dark for 5 min, and washed in PBST. The slides were dried with an absorbent paper, sealed with a liquid containing anti-fluorescence quenching agent, and finally observed under a fluorescence microscope and the images captured, and analyzed using ImageJ software [Citation20].
2.8 Western blotting
Cell proteins extraction was done using RIPA lysis buffer (Biotechnology, Shanghai, China) conjugated with protease inhibitor cocktail (Beyotechnology, Shanghai, China). Proteins were loaded into 12% SDS-PAGE gel. After electrophoresis, proteins were transferred onto polyvinylidene fluoride membrane (Thermo Fischer Scientific, USA). These membranes were blocked with 4% bovine serum albumin for 1 h at room temperature, after which they were incubated with primary antibodies at 4 o C for about 24 h. Next, these membranes were incubated with the appropriate secondary antibodies at room temperature for around 1 h. The primary antibodies against cleaved caspase-3 (1:1000, Abcam, UK; ab32042), cleaved caspase-9 (1:1000, Abcam, UK; ab2324), ATG2B (1:1000, Abcam, UK; ab189934), BECN1 (1:1000, Abcam, UK; ab210498), LC3B (1:1000, Abcam, UK; ab192890), cyclin D1 (1:1000, Abcam, UK; ab16663) were used in this research. GAPDH (1:1000, Beyotechnology, China; AF1186) was used as the internal control. The membranes were incubated for 1 h with HRP-conjugated secondary antibodies (Santa Cruz). Finally, protein bands were visualized by ECL kits (Millipore, USA), and analyzed using ImageJ software.
2.9 Animal experiments
Healthy six BALB/c nude mice (4–6 weeks old, male, 18–20 g) were purchased from the institutional animal center. Housing, care, and anesthesia to the experimental animals were provided following the international and Institutional Animal Welfare and Ethics Committee guidelines. The animals were housed in the animal rooms (10-h light/14-h dark cycle, 22–27°C, relative humidity: 40–60%) under sterile environment. These animals were randomly divided into two groups: (1) the control group (n = 3) and (2) the crocin treatment group (n = 3). A pellet of 2.5 × 107 A431 cells, suspended in cold PBS (100 ul) was injected into the right flank of each BALB/c nude mice subcutaneously. Crocin (50 mg/kg) was administered into each mice in the crocin treatment group every day for 3 weeks by intraperitoneal injection, and an equal amount of natural saline was given to rats in the control group in the same way. Mice were sacrificed by cervical dislocation. Also, tumor xenografts were also collected. The tumor size and body weight were periodically determined.
Tumor measurements were determined by a caliper ruler. The tumor volume was determined by the equation: tumor volume (mm3) = (length × width [Citation2])/2. The length and the width were presented in millimeters [Citation21].
2.10 Statistical methods
Data were analyzed using on GraphPad Prism 8.0 and presented as mean ± standard deviation (SD). Two-tailed P < 0.05 was considered significant. All our experiments were independently performed three times. Student’s t-test and one-way analysis of variance (ANOVA, Bonferroni post hoc test) were adopted in our analysis, depending on the experiment. Pearson correlation test was also done where appropriate.
3. Results
3.1 MiR-320a is upregulated and ATG2B is down-regulated in human cutaneous squamous cell carcinoma
Cutaneous squamous cell carcinoma is a highly prevalent tumor, and the treatment remains a challenge. Crocin is a saffron with a wide medical use. The current study hypothesized that crocin induce anti-proliferative and apoptotic effects on cutaneous squamous cell carcinoma through miR-320a/ATG2B. The study aimed at assessing whether crocin induces apoptosis of cSCC and the involvement of miR-320a/ATG2B axis. To study the expression of MiR-320a and ATG2B in human cutaneous squamous cell carcinoma, 32 cSCC clinical samples and the adjacent normal skin tissues were obtained and analyzed through RT-qPCR. According to the results, MiR-320a expression was upregulated in cSCC samples as compared to the control samples (). However, ATG2B expression was significantly downregulated in the cSCC positive clinical samples than in the control samples (). Moreover, miR-320a expression was negatively associated with the expression of ATG2B (). Together, these data indicated that MiR-320a is upregulated and ATG2B is down-regulated in human cSCC.
Figure 1. Clinical sample analysis. (a) MiR-320a expression in cSCC tissues and normal adjacent tissues. (b) ATG2B expression in cSCC tissues and normal adjacent tissues. (c) The link between miR-320a and ATG2B expression (Pearson correlation analysis). **P < 0.01, ***P < 0.001 versus the control group
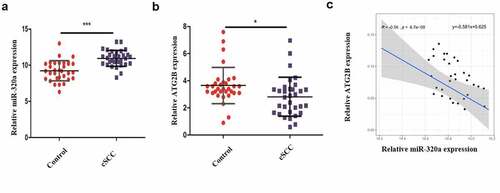
3.2 Crocin suppresses the proliferation and induces apoptosis of cSCC cells
To determine the effects of crocin on tumor cells proliferation, A431 and SCL-1 cells were first treated with 0, 1, 2, or 4 mM of crocin for 24 h. According to the results, cell viability was reduced in a dose-dependent manner (). Cell inhibitory rate was calculated to determine the IC50 of crocin in A431 and SCL-1 cell line. The results showed that the highest cell viability inhibition rate was observed at 4 mM concentration of crocin with 3.2 ± 24.78 IC50 (). Next, 4 mM crocin was chosen as the concentration for the subsequent experiments. A431 and SCL-1 cells were subjected to crocin treatment for 24 or 48 h, and then cell proliferation was evaluated. cSCC cells proliferation was significantly reduced by crocin (). Further, Western blot experiments to analyze the apoptosis of cSCC cells indicated a significantly down-regulated cyclin D1 expression following the tumor cells treatment with 4 mM crocin for 48 h (). These observations demonstrated that Crocin suppresses the proliferation and induces apoptosis of cSCC cells.
Figure 2. Crocin suppresses the proliferation of cSCC cells and induces their apoptosis. (a) MTT assay to evaluate cell viability. (b) Inhibition rate curve used for the determination of IC50 of crocin on the proliferation of A431 and SCL-1 cells treated with 1, 2 and 4 mM crocin for 48 h. (c) MTT assay to examine cell proliferation. (d) Western blots for cyclin D1 and cleaved caspase-3 expression. NS, non-significant, *P < 0.05, **P < 0.01, ***P < 0.001 versus the control group
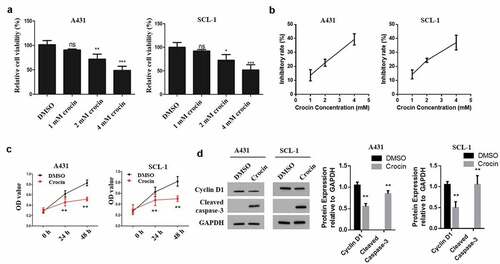
3.3 Crocin increases autophagy in cSCC cells
To investigate the effect of crocin on autophagy of tumor cells, the cells were treated with 4 mM of crocin and incubated for 48 h. The expression of autophagy protein, LC3 puncta was then examined through immunofluorescent staining. According to the results, LC3 puncta expression was significantly increased following the treatments of A431 and SCL-1 cell lines with crocin (). Additionally, the expressions of BECN1 and LC3B autophagy key protein markers were assessed through western blot experiments. The observations indicated a significantly increased BECN1 and LC3B proteins expression in the crocin-treated tumor cells compared to the DMSO-treated controls (). These observations confirmed that crocin might trigger the apoptosis in cSCC cells via autophagy.
2.4 MiR-320a hinders autophagy and the apoptotic effects of crocin on cSCC cells
To explore how crocin influences cSCC cells, A431 and SCL-1 cells were treated with crocin, and miR-320a mRNA expression was analyzed through qRT-PCR. The results demonstrated a significantly reduced miR-320a expression following the treatment with crocin (). Next, both cell lines were transfected with miR-320a mimics and its negative control, miR-320a inhibitor and inhibitor negative control (NC), and ATG2B inhibitor and its negative control. The miR-320a mRNA expression was analyzed through qRT-PCR. According to the observation, miR-320a mRNA expression was significantly reduced compared to the control (). Later, western blot experiment was undertaken to analyze, BENC1, LC3B and cleaved caspace 3 expression in tumor cells transfected with miR-320a inhibitor and the controls. The observations demonstrated a significantly increased BECN1, LC3B and cleaved caspace 3 proteins expressions in cells transfected with miR-320a inhibitors compared to the control groups as shown in . Taken together, the observations demonstrate that miR-320a might play a negative role in inducing autophagy and apoptosis following crocin treatment in cSCC cells.
Figure 4. MiR-320a mediates autophagy and the apoptotic effects of crocin on cSCC cells. (a) MiR-320a expression in both cell lines following crocin treatment. (b) MiR-320a expression following transfection. (c) Western blots for BECN1, LC3B and cleaved caspase-3. **P < 0.01, ***P < 0.001 versus the control group
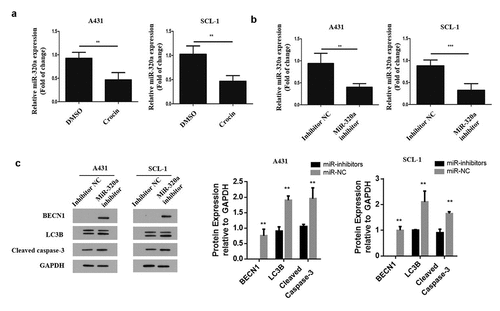
3.5 MiR-320a binds ATG2B directly
To identify the MiR-320a target and understand the mechansisms underlying its role in cSCC cancer, ENCOR1 was used. The resulting prediction indicated that miR-320a could target ATG2B directly and the complementary sequence between these two genes is illustrated in . Next, dual luciferase reporter assay was performed to determine whether miR-320a binds directly to 3ʹUTR of ATG2B. First, two types of luciferase constructs of ATG2B were made: pGL3-ATG2B-WT and pGL3-ATG2B-MUT. A431 cells were co-transfected with pGL3-ATG2B-WT and mimic NC, miR-320a mimic, inhibitor negative control (NC) or miR-320a inhibitor, respectively. The results show that after transfecting A431 cells with pGL3-ATG2B-WT, the co-transfection with miR-320a mimic significantly reduced the luciferase activity, whereas the co-transfection with miR-320a inhibitor significantly increased luciferase activity (). Further, A431 cells were co-transfected with pGL3-ATG2B-MUT and mimic negative control (NC), miR-320a mimic, inhibitor negative control (NC) or miR-320a inhibitor respectively. The results indicated that transfection with miR-320a mimic or pGL3-ATG2B-MUT + miR-320a did not have any effect on the luciferase activity (). To confirm the relationship between miR-320a and ATG2B, A431 cells were transfected with miR-320a mimic and mimic NC, respectively, and the expression of ATG2B was analyzed by western blot. According to the results, ATG2B expression was significantly elevated in MiR-320a inhibitor transfection compared to the miR-320a mimic transfection group (). Therefore, these observations confirm that ATG2B is one of the direct binding sites of miR-320a.
Figure 5. ATG2B expression is upregulated by crocin. (a) The illustration of the complementary sequence between miR-320a and ATG2B. (b) The luciferase activity in wild-type luciferase constructs. (c) The luciferase activity in mutant-type luciferase constructs. (d) ATG2B expression following miR-320a inhibitor transfection. NS, non-significant, *P < 0.05, **P < 0.01, ***P < 0.001 versus the control group
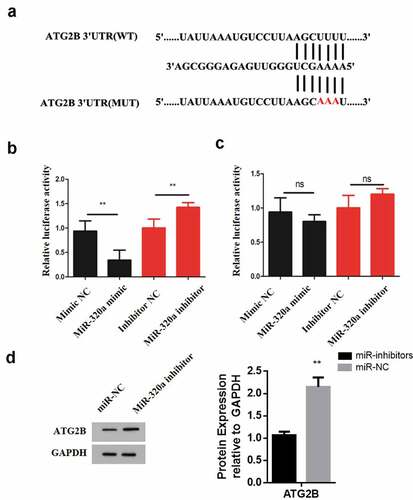
3.6 ATG2B expression is upregulated by crocin
To explore how miR-320a influences autophagy in cSCC cells, the expression of the genes related to autophagy in these cells was assessed through qRT-PCR. It was found that ATG2B mRNA expression was remarkably upregulated following the treatments of both tumor cells with crocin (). Western blotting to determine ATG2B expression showed a significantly elevated ATG2B protein expression following treatment with crocin as compared to the DMSO treated group (). To determine the role of ATG2B in autophagy, lentivirus was devised to transfect cSCC cells and knock down ATG2B expression in cSCC cells, and qRT-PCR was used to confirm the transfection efficiency. The results confirmed a significantly down-regulated relative ATG2B expression in the si-ATG2B group as compared to the si-NC group (). After ATG2B expression was silenced in these two cell lines, the expression of cleaved caspase-3 was examined through western blot. The cleaved caspase-3 expression was significantly increased following ATG2B knockdown in both the cell lines as compared to the si-NC group (). Taken together, these results confirm that crocin induces the apoptosis in cSCC cells via ATG2B.
3.7 Crocin triggers the apoptosis in cSCC in vivo
To confirm the apoptosis role of crocin in vitro, A431 cells were subcutaneously injected into nude mice to induce tumors the experimental mice were then treated with 4 mM of crocin and tumor growth monitored. The animals were eventually sacrificed and the tumor sizes and weight were determined. The results indicated a significantly inhibited tumor size in the treatment group compared to the control. (). Similarly, the tumor weight in the crocin treatment group was significantly lower than in the control group (). Determination of also, cyclin D1 expression through western blot confirmed a significantly reduced cyclin D1 expression in the crocin treatment group compared to the negative treatment group. Cleaved caspase-3, ATG2B, LC3B and BECN1 expression were also increased by crocin treatment compared to those in the control group (). Summarized together, these data generally confirm that crocin suppresses cSCC growth and induces apoptosis in vivo.
4. Discussion
Cutaneous SCC has a high recurrence rate and a high chance of metastasis. The conventional treatment methods for cSCC include chemotherapy and surgery, which have had long time challenges of remission. Besides, most drugs pose a harmful side effects. Consequently, developing more effective novel cSCC treatments methods is necessary. The current research assessed the effects of crocin in the treatment of cSCC. The findings demonstrated that crocin could promote the apoptosis of SCC and repress the proliferation in SCC both in vitro and in vivo.
The role of crocin in inhibiting growth has been reported in various cancers, for instance, such as breast cancer [Citation14]. The mechanism of inhibition of proliferation in breast carcinoma cells by crocin has been reported to involve binding tubulin dimers and oligomers, resulting in the distorted formation of microtubular network in breast cancer cells [Citation22]. Similarly, reports have demonstrated that crocin can bind tubulin at the vinblastine location and lead to a pause in cellular mitosis by increasing the activity of checkpoint proteins [Citation23]. In this study, crocin was directly linked to autophagy pathway, and ATG2B was demonstrated to be upregulated by crocin. Even though Autophagy has been reported to play dual functions in cancer down-regulation or promotion [Citation24], crocin in this particular study was shown to promote autophagy, (autophagy-related gene 2; ATG2b) consequently suppressing cSCC. Beclin 1 function as a repressor of tumor, hence it was highly increased in crocin treatment in our study, which is in agreement with several other related studies [Citation25]. Furthermore, LC3B, a marker for the autophagosomal membrane was also highly expressed following treatment with crocin. The same observation had been reported by Jong et al, following treatment of Glioblastoma cells with curcumin [Citation26].
MiR-320a has previously been reported to play a tumor suppressor role in Acts in Non-small Cell Lung Cancer [Citation17]. Similarly, past works demonstrated that the proliferation and metastasis of lung adenocarcinoma cells could be suppressed by miR-320a [Citation27]. Another related study indicated that that miR-320a exhibits anti-proliferative and apoptotic effects on multiple myeloma cells via regulating pre-B-cell leukemia transcription factor 3 [Citation28]. However, in this report, miR-320a expression was increased in cSCC and it was suppressed following treatment with crocin, depicting that crocin may regulate the proliferation of SCC cells epigenetically.
Additionally, the current work shows that crocin induced autophagic apoptosis in SCC cells. Similar observations have been made in hepatocellular carcinoma treatment using crocin [Citation15]. This work specifically identified that crocin could induce cell apoptosis in SCC through miR-320/ATG2B axis. Through dual luciferase reporter assay, it was shown that miR-320a could target ATG2B in a direct manner, which has not been reported before. MiR-320a has been demonstrated to regulate autophagy in Parkinson’s disease and cervical cancer [Citation29] . The link between apoptosis, ATG2B, and autophagy has been widely studied. ATG2B and DICER1 targeting has been shown to impair the autophagy in chronic lymphocytic leukemia cells and trigger their apoptosis [Citation30]. Similarly, in osteoarthritis, targeting ATG2B can induce cell apoptosis [Citation31]. Consequently, from the study observations, targeting ATG2B in SCC could lead to its apoptosis.
Conclusion
MiR-320a is upregulated and ATG2B is down-regulated in human cutaneous squamous cell carcinoma, Crocin suppresses the proliferation and induces apoptosis of cSCC cells. Further, Crocin increases autophagy in cSCC cells, while MiR-320a hinders autophagy and the apoptotic effects of crocin on cSCC cells. MiR-320a binds ATG2B directly, and ATG2B expression is upregulated by crocin. Finally, Crocin triggers cSCC cells apoptosis in vivo. The use of crocin to target ATG2B/miR-320a could be a novel and effective alternative for the treatment of cutaneous squamous cell carcinoma.
Research highlights
MiR-320a is upregulated and ATG2B is down-regulated in human cutaneous squamous cell carcinoma.
Crocin suppresses the proliferation, and induces apoptosis and autophagy of cSCC cells.
Crocin regulates the expression of ATG2B, which is a direct target of miR-320a.
Crocin triggers cSCC cells apoptosis in vivo.
Disclosure statement
No potential conflict of interest was reported by the author(s).
References
- Green AC, Olsen CM. Cutaneous squamous cell carcinoma: an epidemiological review. Br J Dermatol. 2017;177(373–381). DOI:10.1111/bjd.15324
- Motaparthi K, Kapil JP, Velazquez EF. Cutaneous Squamous Cell Carcinoma: review of the Eighth Edition of the American Joint Committee on Cancer Staging Guidelines, Prognostic Factors, and Histopathologic Variants. Adv Anat Pathol. 2017;24(4):171–194.
- Shelton ME, Adamson AS. Review and Update on Evidence-Based Surgical Treatment Recommendations for Nonmelanoma Skin Cancer. Dermatol Clin. 2019;37(9):425–433.
- Kroemer G, Mario G, Levine B . Autophagy and the integrated stress response.[J]. Molecular Cell, 2010, 40(2):280–293
- Kovaleva V, Mora R, Park Y J, et al. miRNA-130a Targets ATG2B and DICER1 to Inhibit Autophagy and Trigger Killing of Chronic Lymphocytic Leukemia Cells[J]. Cancer Research, 2012; 72(7):1763–1772.
- Wei, Ma, Li, et al. miR-143 inhibits cell proliferation by targeting autophagy-related 2B in non-small cell lung cancer H1299 cells.[J]. Molecular Medicine Reports, 2015, 11(1):571–576.
- Zhao H, Dong T, Zhou H, et al. miR-320a suppresses colorectal cancer progression by targeting Rac1[J]. Carcinogenesis(4):886.
- He, Xiangjun, Dong, et al. microRNA-320a inhibits tumor invasion by targeting neuropilin 1 and is associated with liver metastasis in colorectal cancer (vol 27, pg 685, 2012)[J]. Oncology Reports, 2015.
- Jian Yao, Lin-hui Liang, et al. GNAI1 Suppresses Tumor Cell Migration and Invasion and is Post-Transcriptionally Regulated by Mir-320a/c/d in Hepatocellular Carcinoma[J]. Cancer Biology & Medicine, 2012, 9(4):234–241.
- Sepramaniam S, Sugunavathi, Armugam, et al. MicroRNA 320a Functions as a Novel Endogenous Modulator of Aquaporins 1 and 4 as Well as a Potential Therapeutic Target in Cerebral Ischemia*. 2010;285:29223–29230.
- I-Shan H, Chang K C, Yao-Tsung T, et al. MicroRNA-320 suppresses the stem cell-like characteristics of prostate cancer cells by downregulating the Wnt/beta-catenin signaling pathway.[J]. Carcinogenesis, 2013(3):530–538.
- Hoshyar R, Mollaei H. A comprehensive review on anticancer mechanisms of the main carotenoid of saffron, crocin. J Pharm Pharmacol. 2017;69(11):1419–1427.
- Zhou Y, Xu Q, Shang J, et al. Crocin inhibits the migration, invasion, and epithelial-mesenchymal transition of gastric cancer cells via miR-320/KLF5/HIF-1α signaling. J Cell Physiol. 2019;234(10):17876–17885.
- Lu P, Lin H, Gu Y, et al. Antitumor effects of crocin on human breast cancer cells[J]. International Journal of Clinical & Experimental Medicine, 2015;8(11):20316.
- Yao C, Liu -B-B, Qian X-D, et al. Crocin induces autophagic apoptosis in hepatocellular carcinoma by inhibiting Akt/mTOR activity[J]. Onco Targets Ther, 2018;11:2017–2028.
- Zhao L, Zhao X, et al. The α-tocopherol derivative ESeroS-GS induces cell death and inhibits cell motility of breast cancer cells through the regulation of energy metabolism[J]. European Journal of Pharmacology, 2014;745:98–107.
- Khandelwal A, Sharma U, Barwal T S, et al. Circulating miR-320a Acts as a Tumor Suppressor and Prognostic Factor in Non-small Cell Lung Cancer[J]. Frontiers in Oncology, 2021; 11.
- Wei, Ma, Li, et al. miR-143 inhibits cell proliferation by targeting autophagy-related 2B in non-small cell lung cancer H1299 cells.[J]. Molecular Medicine Reports, 2015, 11(1):571–576.
- Chen S, Shuang Z, Wang X, et al. Crocin inhibits cell proliferation and enhances cisplatin and pemetrexed chemosensitivity in lung cancer cells[J]. Translational Lung Cancer Research, 2015, 4(6):775.
- Hu A, Huang J J, et al. Curcumin suppresses invasiveness and vasculogenic mimicry of squamous cell carcinoma of the larynx through the inhibition of JAK-2/STAT-3 signaling pathway[J]. American Journal of Cancer Research, 2014, 5(1):278.
- Fulzele SV, Chatterjee A, Shaik MS, et al. Inhalation delivery and anti-tumor activity of celecoxib in human orthotopic non-small cell lung cancer xenograft model. Pharm Res. 2006;23(9):2094–2106.
- Hire RR, Srivastava S, Davis MB, et al. Antiproliferative Activity of Crocin Involves Targeting of Microtubules in Breast Cancer Cells. Sci Rep. 2017;7(1). DOI:10.1038/srep44984
- Sawant AV, Srivastava S, Prassanawar SS, et al. Crocin, a carotenoid, suppresses spindle microtubule dynamics and activates the mitotic checkpoint by binding to tubulin. Biochem Pharmacol. 2019;163(32):32–45.
- Salminen A, Kaarniranta K, Kauppinen A . Beclin 1 interactome controls the crosstalk between apoptosis, autophagy and inflammasome activation: Impact on the aging process[J]. Ageing Research Reviews, 2013, 12(2):520–534.
- Yun CW, Lee SH. The Roles of Autophagy in Cancer. Int J Mol Sci. 2018;19(11):3466.
- Lee J-E, Yoon SS, Moon E-Y. Curcumin-Induced Autophagy Augments Its Antitumor Effect against A172 Human Glioblastoma Cells. Biomol Ther (Seoul). 2019;27(5):484–491.
- Lv Q, Hu J-X, Li Y-J, et al. MiR-320a effectively suppresses lung adenocarcinoma cell proliferation and metastasis by regulating STAT3 signals. Cancer Biol Ther. 2017;18(3):142–151.
- Lu Y, Wu D, Wang J, et al. miR-320a regulates cell proliferation and apoptosis in multiple myeloma by targeting pre-B-cell leukemia transcription factor 3. Biochem Biophys Res Commun. 2016;473(4):1315–1320.
- Li G, Yang H, Zhu D, et al. Targeted suppression of chaperone-mediated autophagy by miR-320a promotes α-synuclein aggregation. Int J Mol Sci. 2014;15(9):15845–15857.
- Kovaleva V, Mora R, Park YJ, et al. miRNA-130a Targets ATG2B and DICER1 to Inhibit Autophagy and Trigger Killing of Chronic Lymphocytic Leukemia Cells. Cancer Res. 2012;72(7):1763–1772.
- Li H, Li Z, Pi Y, et al. MicroRNA-375 exacerbates knee osteoarthritis through repressing chondrocyte autophagy by targeting ATG2B[J]. Aging (Albany NY), 2020;12(8).