ABSTRACT
The gut microbiota system plays a vital role in liver diseases. This study aimed to address the diversity of gut microbiota and its correlations with clinical parameters in healthy individuals, chronic liver disease (CLD), and hepatocellular carcinoma (HCC) patients. Fecal specimens of nine healthy individuals, 11 CLD, and 21 HCC were collected. The diversity of gut microbiota was examined by PCR and Illumina MiSeq sequencing and analyzed using 16S rRNA gene sequencing database. The correlations between gut microbiota and the clinical parameters of participants were also addressed. Compared to healthy individuals, Firmicutes at a phylum level decreased in CLD and HCC patients and Proteobacteria increased (p < 0.05). The composition of Blautia on a genus level in CLD and HCC patients significantly decreased compared to healthy controls (p < 0.05). Firmicutes composition was negatively associated with age and number of males (p < 0.05) and was positively associated with monocytes, high-density lipoprotein cholesterol (HDL-C), and estimated glomerular filtration rate (eGFR) levels (p < 0.05). At a genus level, Blautia composition was negatively associated with cirrhosis, age, and number of males (p < 0.01), while it was positively associated with red blood cells (RBCs), triglycerides, HDL-C, and lymphocyte levels (p < 0.05). Conclusively, there was a significant compositional difference in gut microbiota in CLD and HCC patients compared with healthy subjects. Firmicutes and Blautia in gut microbiota system lessened in CLD and HCC patients. Clinical biochemical parameters have an impact on the diversity of gut microbiota in liver diseases.
1. Introduction
The gut microbiome is present in the intestinal system and produces metabolites that exert various supporting effects on host biological functions [Citation1]. Dysbiotic microbiota can affect the host’s immune system and mucosal integrity through multiple mechanisms involving modulation of inflammasome signalings and regulation of immune cell functions and responses [Citation2]. Currently, it is unclear whether the dysbiotic microbiota is a cause of diseases or a consequence of disease-related changes. However, microbiota transplantation is a promising therapeutic approach for some pathological phenotypes, suggesting a causative relationship between gut microbiota and disease pathogenesis [Citation3–6].
In view of the close links between the gut system and the liver, many pathological processes have been addressed in light of a microbe-centered hypothesis of liver damage [Citation7]. Changes in the composition and function of gut microbiota are correlated with the development and progression of multiple kinds of liver diseases [Citation8,Citation9]. Many studies have focused on the links of noninfectious variables and dysbiotic microbiota in liver diseases. In a report by Lu et al., the authors found that the densities of Bifidobacteria and Lactobacillus were significantly decreased and those of the Enterococcus and Enterobacteriaceae were significantly increased in chronic hepatitis B (CHB) patients compared to healthy controls [Citation10]. In addition, the intestinal bacterial products and gut microbiota imbalance can promote progression to cirrhosis and hepatocellular carcinoma (HCC) [Citation11–13]. Unfortunately, most literature identified the pathological changes of gut microbiota in the progression of nonviral HCC patients [Citation1,Citation9].
Since chronic liver diseases, particularly chronic hepatitis B virus (HBV) infection, have been demonstrated to have an impact on cirrhosis and tumorigenesis, this study aims to investigate the diversity of gut microbiota in healthy individuals, chronic liver diseases (mainly CHB subjects), and HCC patients. This study wants to compare the gut microbiota composition in different liver diseases, in the hope of gaining a deep understanding of the disease progression and cancer development.
2. Materials and methods
2.1. Ethic statement
All participants provided the written informed consent. The protocol and informed consent documents were reviewed and approved by the Ethics Committee, Shanghai Public Health Clinical Center, Fudan University (No. 2020-S117-01).
2.2. Participants
Nine healthy individuals, 11 chronic liver diseases (CLD), and 21 HCC in Shanghai Public Health Clinical Center, Fudan University, were included in this pilot study. The healthy individuals met the following criteria: 1) normal blood /urine/stool routine tests and liver-kidney functions, 2) no history of liver disease, and 3) no intestinal probiotics and antibiotics prescriptions within 2 weeks before sample collection. CLD patients included chronic hepatitis and cirrhosis patients, regardless of the disease status and liver functions. HCC was either diagnosed pathologically or radiologically according to guidelines for diagnosis and treatment of primary liver cancer in China – two of the four imaging studies, including computed tomography (CT), magnetic resonance imaging (MRI), gadolinium-ethoxybenzyl-diethylenetriamine pentaacetic acid MRI, or contrast-enhanced ultrasonography, showing an arterial enhanced mass less than 2 cm, or one of the four imaging studies above showing an arterial enhanced mass greater than 2 cm [Citation14]. Subjects with acute or chronic gastrointestinal diseases, renal failure, liver failure, sepsis, autoimmune disorders, and other uncontrolled life-threatening diseases were excluded from the study. Fecal samples of all the participants were collected with germ-free collection boxes. The fecal samples were stored in −80°C refrigerator immediately.
2.3. Laboratory examinations
Blood routine tests and liver-kidney functions of participants were conducted by the Medical Laboratory on the basis of the Standard Operation Procedure for medical examinations in Shanghai Public Health Clinical Center, Fudan University.
2.4. DNA extraction and PCR amplification
The genomic DNA of the microbial community was extracted from all these samples using a TransStart® FastPfu DNA Polymerase system (TransGen AP221-02, TransGen Biotech, Beijing, China) following the manufacturer’s instructions. The DNA extract was detected on 1% agarose gel, and DNA concentration and purity were determined using a NanoDrop 2000 UV-vis spectrophotometer (Thermo Scientific, Wilmington, USA). The hypervariable regions V3-V4 of the bacterial 16S rRNA gene were amplified using an ABI GeneAmp® 9700 PCR thermocycler (ABI, CA, USA) with primer pairs 338 F (5ʹ- ACTCCTACGGGAGGCAGCAG −3ʹ) and 806 R (5ʹ- GGACTACHVGGGTWTCTAAT −3ʹ). The PCR amplification of the 16S rRNA gene was conducted according to the procedures [Citation15,Citation16]. PCR reactions were performed in triplicate. According to the manufacturer’s instructions, the PCR product was extracted from 2% agarose gel and purified by the AxyPrep DNA Gel Extraction Kit (Axygen Biosciences, Union City, CA, USA) and quantified using Quantus™ Fluorometer (Promega, USA).
2.5. Illumina MiSeq sequencing
The purified amplified products were combined on the Illumina MiSeq PE300 platform/NovaSeq PE250 platform (Illumina, San Diego, USA) by isomolar and paired-terminal sequencing according to the standard protocols by Shanghai Majorbio Bio-Pharm Technology Co. Ltd. (Shanghai, China).
2.6. Processing of sequencing data
The original 16S rRNA gene sequencing readings were demultiplexed, quality-filtered using fastp version 0.20.0 [Citation17], and merged using FLASH version 1.2.7 [Citation18] according to the criteria reported by Wang et al. [Citation19].
Operational taxonomic units (OTUs) with 97% similarity cutoff [Citation20,Citation21] were clustered using UPARSE version 7.1 [Citation20], and chimeric sequences were identified and deleted. The classification of each OTU representative sequence was computed using RDP Classifier version 2.2 [Citation22] with a confidence threshold of 0.7 for the 16S rRNA database.
2.7. Statistical analysis
The normally distributed continuous data were described by mean ± standard deviation (SD) and the enumeration data was described by the frequency with percentage. Student’s t-test and chi-square test based on variable types are used to analyze the differences of variables between groups. The relative abundance was used to compute the density of gut microbiota. Principal coordinate analysis (PCoA) was used to compare the composition of gut microbiota between groups. The distance in gut microbiota composition between samples was calculated using the Bray-Curtis dissimilarity matrix. Spearman correlation analysis between clinical data and gut microbiota was also performed. Stata v16.1 (Stata, Texas, USA) was used. A two-side p < 0.05 were considered significance.
3. Results
Using 16S rRNA sequencing analysis, a significant compositional difference in gut microbiota was observed in CLD and HCC patients compared with healthy subjects. Compared to healthy individuals, Firmicutes at a phylum level decreased in CLD and HCC patients and Proteobacteria increased. The composition of Blautia on a genus level in CLD and HCC patients significantly decreased compared to healthy controls. Firmicutes and Blautia compositions were affected by multiple clinicopathological factors, for instance, age, gender, and HDL-C. The current study preliminarily indicated that alterations of gut microbiota might be involved in the progression of liver diseases.
3.1. Participant characteristics
As summarized in , one male was in the healthy control group, seven in the CLD group, and 21 in the HCC group (p < 0.05). The average age of healthy individuals, CLD and HCC patients were 29.2 years, 44.5 years, and 62.4 years, respectively (p < 0.05). Most HCC patients suffered from cirrhosis compared to healthy controls and CLD patients (p < 0.05). The levels of red blood cells (RBC), hemoglobin, platelets, and lymphocytes in blood routine tests were significantly low in HCC patients (p < 0.05). By concerning liver functions, the levels of alanine aminotransferase (ALT), aspartate aminotransferase (AST), alkaline phosphatase (ALP), and gamma-glutamyl transferase (GGT) were significantly higher in CLD and HCC patients compared to healthy individuals (p < 0.05). On the contrary, the albumin levels of CLD and HCC patients were significantly decreased compared to healthy controls (p < 0.05). The serum levels of triglycerides and total cholesterol were also significantly different in these three groups (p < 0.05), and the levels of HDL-C and LDL-C gradually decreased in the healthy controls, CLD and HCC patients (p < 0.05). In addition, the fasting plasma glucose (FPG) levels gradually increased from healthy individuals, CLD subjects to HCC patients (p < 0.05). No significances of the urea, creatinine and eGFR were observed in these three groups (p > 0.05).
Table 1. Baseline characteristics of participants included in this study
3.2. Diversity of gut microbiota on phylum and genus levels
As shown in , Firmicutes were more abundant in healthy individuals and decreased in CLD and HCC patients at phylum level, while the percentage of Proteobacteria increased in CLD and HCC patients compared to healthy controls (). In addition, Bacteroidota and Synergistota were more abundant in HCC patients compared to healthy controls and CLD patients (). At genus level, Blautia was more abundant in healthy individuals, while it decreased in CLD patients and much lower in HCC patients (). The Circos diagrams of gut microbiota composition in healthy individuals, CLD, and HCC patients at phylum and genus levels are given in Figure S1 and Figure S2, respectively.
3.3. Comparison of gut microbiota composition
As shown in , the compositions of gut microbiota in healthy individuals, CLD, and HCC patients were significantly different (Adonis, p = 0.001, ). The composition of the microbiome in HCC patients was significantly different from that in healthy individuals and CLD patients (Adonis, p = 0.001 and p = 0.005, respectively, and d). No significance of gut microbiota composition was found between healthy individuals and CLD patients (Adonis, p = 0.067, ).
Figure 2. Principal co-ordinates analysis (PCoA) of gut microbiota among healthy individuals, CLD and HCC patients
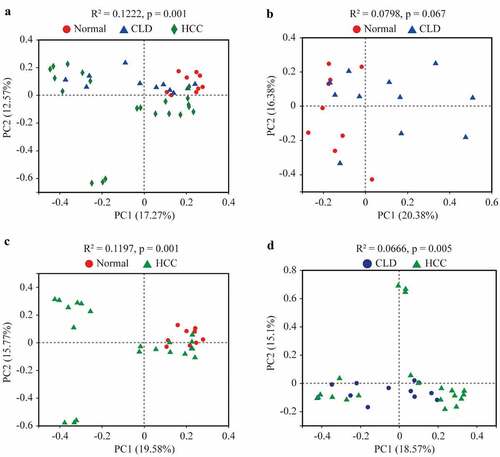
At the phylum level, Proteobacteria had a significantly higher proportion in both CLD and HCC patients compared to healthy individuals (both p < 0.05, and b). Additionally, the community composition of Firmicutes was significantly decreased in HCC patients compared to healthy individuals (p < 0.001, ) and CLD patients (p < 0.05, ). With a relatively lower proportion, Patescibacteria was differentially distributed between HCC patients and healthy controls/CLD patients (both p < 0.05, and c); Verrucomicrobiota was also significantly different between HCC patients and healthy controls (p < 0.05, ).
Figure 3. Proportion comparisons of gut microbiota between healthy individuals and CLD patients (a), healthy individuals and HCC patients (b), and CLD and HCC patients (c) on phylum level
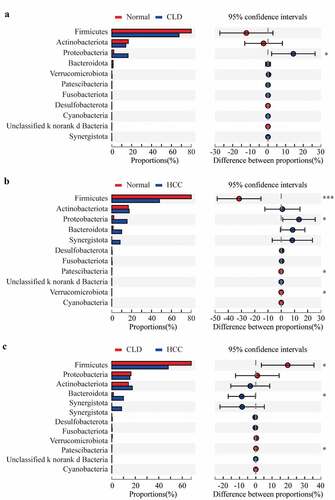
Compared to healthy individuals, the composition of Blautia at genus level in CLD and HCC patients significantly decreased (p < 0.05 and p < 0.001, respectively, and b); Escherichia-Shigella proportion significantly increased in CLD patients (p < 0.05, ); Eubacterium hallii group and Fusicatenibacter were significantly lower in HCC patients (p < 0.001 and p < 0.05, respectively, ). Compared to CLD patients, proportions of Blautia, Eubacterium hallii group, and Ruminococcus gnavus group were significantly lower in HCC patients (all p < 0.05, ). Conversely, the proportion of Streptococcus was significantly higher in HCC patients compared to CLD cases (p < 0.05, ).
3.4. Correlations between gut microbiota and clinical biochemical parameters
Multicollinearity of the clinical biochemical parameters was checked by calculating the Variance Inflation Factor (VIF). All variables with a VIF value of less than eight were included in the correlation analysis. After VIF analysis, variables, namely, male, age, HBV infection, cirrhosis, ascites, intestinal microecological agents, RBC, neutrophils, lymphocytes, monocytes, AST, GGT, direct bilirubin (DBiL), triglycerides, HDL-C, urea, creatinine, FPG, and eGFR, were taken for the Spearman correlation model (Supplementary Table S1).
As detailed in , Firmicutes composition was negatively associated with age and male numbers (p < 0.01, ) and was positively associated with monocytes, HDL-C, and eGFR levels (p < 0.05, ). Proteobacteria were negatively correlated with RBC, HDL-C, intestinal microecological agents, and eGFR levels (p < 0.05, ) and were positively correlated with age, male numbers, and creatine (p < 0.05, ). Bacteroidota was negatively associated with creatine and lymphocytes and was positively associated with cirrhosis, HBV infection, and eGFR (p < 0.05, ). Fusobacteriota was negatively correlated with lymphocytes and positively correlated with HBV infection and eGFR (p < 0.05, ). Patescibacteria was negatively associated with age and positively associated with RBC, triglycerides, and eGFR (p < 0.05, ). Verrucomicrobiota was conversely correlated with cirrhosis and male numbers and positively correlated with triglycerides, HDL-C, and intestinal microecological agents (p < 0.05, ).
Figure 5. Spearman correlations between gut microbiota and clinical biochemical parameters on phylum level
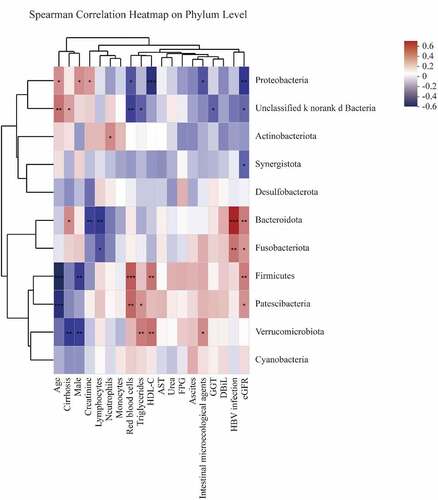
The Spearman correlations of the gut microbiome at genus level and variables are described in . Blautia composition was negatively associated with cirrhosis, age, and male (p < 0.01, ), while it was positively associated with RBC, triglycerides, HDL-C, and lymphocyte levels (p < 0.05, ). Additionally, HBV infection positively contributed to increasing proportions of Megasphaera, Streptococcus, Veillonella, Prevotella, Eubacterium ventriosum group, Agathobacter, Monoglobus, Parabacteroides, Alistipes, Lachnoclostridium, Bacteroides, and Lachnospiraceae NK4A136 group (p < 0.05, ).
4. Discussion
A balanced gut microbiota provides essential nutrients, maintains intestinal mucosal integrity, protects the body from pathogens, produces antimicrobial peptides, and plays an active role in the innate and adaptive immune system [Citation23,Citation24]. The imbalance of the gut microbiota leads to an increased risk of endotoxemia, insulin resistance, systemic inflammation, obesity and metabolic disorders, nonalcoholic fatty liver diseases, and cancer [Citation23–26]. Currently, probiotics and prebiotics can restore the normal flora of the gut microbiome and show favorable efficacy in the treatment of some pathological conditions including liver diseases [Citation9,Citation27,Citation28]. The present study partly supplements the understanding of gut microbiota in the development of CLD and HCC.
Firmicutes and Bacteroidetes are the most common phylum in the gut microbiota [Citation23], and Firmicutes are more abundant than Bacteroidetes [Citation29]. Organisms in Firmicutes phyla are an important part of normal flora. Many Firmicutes are able to produce endospores, which are strongly dehydrated, and highly resistant to environmental stresses, and can survive extreme conditions. In addition, Firmicutes can generate more abundant energy than Bacteroidetes [Citation30]. The Firmicutes abundance was reported to be correlated with body mass index in healthy individuals [Citation31,Citation32]. Pathologically, Firmicutes could induce obesity and hepatic steatosis and promoted the elevation of TNF-α mRNA levels, suggesting that Firmicutes might be involved in the pathogenesis of the nonalcoholic fatty liver disease (NAFLD) [Citation33–35]. Many studies have shown a significant increase in Firmicutes in patients with gallstone disease [Citation36]. Moreover, increased Firmicutes has been reported in multiple sclerosis patients [Citation37,Citation38]. In contrast to elevated proportions of Firmicutes in these pathological phenotypes, the present study indicated that CLD and HCC patients had a remarkably low proportion of Firmicutes, which might lead to more sensitivity to environmental stresses and lack of energy. The current profile of Firmicutes could be a reference for deep investigations aimed at illustrating the contribution of this phylum to the progression of liver diseases and HCC.
As for lower taxa of the Firmicutes, Blautia has been shown to be significantly and negatively related to visceral fat accumulation in healthy Japanese people of men and women [Citation39,Citation40]. These findings partly explained that Blautia was positively associated with triglycerides and HDL-C in this study. Blautia is widely distributed in mammalian feces and intestines and has indicated a series of potential probiotic properties [Citation41]. It plays a favorable role in alleviating inflammatory diseases and metabolic disorder, with antibacterial activities against specific microorganisms [Citation41–43]. Emerging literature demonstrated that Blautia is of great importance in maintaining host health and correlating with physiological dysfunctions including cancer and various inflammatory diseases [Citation41,Citation44]. For instance, Blautia decreased significantly in diabetes both in children and adults compared to healthy controls [Citation45,Citation46], and increased abundance of Blautia was correlated with the improvements in glucose and lipid homeostasis [Citation47]. Increased amounts of Blautia were associated with a reduced lethality and favorable survival in acute graft-versus-host disease after allogeneic blood/marrow transplantation in blood malignancies [Citation48]. In the present report, Blautia was the highest in healthy individuals, low in CLD patients, and the lowest in HCC patients and inversely correlated with cirrhosis. Kakiyama et al. revealed that cirrhosis patients had a lower abundance of Blautia compared to healthy controls, which is inconsistent with the results of this study [Citation49]. That is, Blautia should be a potential agent in the comprehensive treatment for liver diseases.
In the current report, Firmicutes and Blautia compositions were correlated with clinical biochemical parameters, such as age, male, triglycerides, HDL-C, RBC, monocytes, and lymphocyte levels. Part of these factors were supported by previous publications [Citation33,Citation36,Citation41,Citation50]. Notably, age and gender were differentially distributed in the healthy individuals and CLD and HCC patients in the current study. As reported previously, the gut microbiota community varied as age changed and was influenced by gender [Citation51,Citation52]. Considering the different distributions of variables among healthy individuals and CLD and HCC patients, the correlations between gut microbiota composition and clinical biochemical parameters should be reevaluated in the same populations using variable-matching methods in the future.
Some limitations exist in this study. First, this study focused on the phylum and genus levels and did not conduct in-depth studies at the species or even strain levels. Second, the sample size is relatively small and no tumor-free cirrhosis patients are included. Third, variables within each group and among the groups were differently distributed, like age and gender, and biases of factors correlating with gut microbiome existed. Although, the results preliminarily revealed the changes of gut microbiota during the development of liver diseases, reduction of Firmicutes and Blautia might worsen the severity of liver pathological conditions.
5. Conclusion
There was a significant compositional difference in gut microbiota in CLD and HCC patients compared with healthy subjects. Notably, Firmicutes at a phylum level and Blautia at a genus level significantly decreased in CLD and HCC patients compared with healthy individuals. Firmicutes and Blautia compositions were affected by multiple clinicopathological factors, for instance, age, gender, and HDL-C. The current study preliminarily indicated that changes in gut microbiota composition were involved in the progression of liver diseases. Reversing the imbalanced gut microbiota system might be a potential therapy in this population.
Highlights
The gut microbiota changed as the liver disease developed.
There was a significant compositional difference in gut microbiota in CLD and HCC patients compared with healthy subjects.
Firmicutes and Blautia lessened in CLD and HCC patients.
The composition of gut microbiota is influenced by multiple clinicopathological factors.
Authors’ contribution
Tianyou Chen and Rongrong Ding have contributed equally to this work.
Supplemental Material
Download ()Disclosure statement
The authors declared no conflicts of interest in this work.
Data availability
All the datasets were available from the corresponding author (Z. Yang) with reasonable request.
Supplementary material
Supplemental data for this article can be accessed here.
Additional information
Funding
References
- Albhaisi SAM, Bajaj JS, Sanyal AJ. Role of gut microbiota in liver disease. Am J Physiol Gastrointest Liver Physiol. 2020 Jan 1;318(1):G84–G98.
- Levy M, Kolodziejczyk AA, Thaiss CA, et al. Dysbiosis and the immune system. Nat Rev Immunol. 2017 Apr;17(4):219–232.
- Berer K, Gerdes LA, Cekanaviciute E, et al. Gut microbiota from multiple sclerosis patients enables spontaneous autoimmune encephalomyelitis in mice. Proc Natl Acad Sci USA. 2017 Oct 3;114(40):10719–10724.
- Ellekilde M, Selfjord E, Larsen CS, et al. Transfer of gut microbiota from lean and obese mice to antibiotic-treated mice. Sci Rep. 2014 Aug 1;4:5922.
- Kostic AD, Gevers D, Siljander H, et al. The dynamics of the human infant gut microbiome in development and in progression toward type 1 diabetes. Cell Host Microbe. 2015 Feb 11;17(2):260–273.
- Scheperjans F, Aho V, Pereira PA, et al. Gut microbiota are related to Parkinson’s disease and clinical phenotype. Mov Disord. 2015 Mar;30(3):350–358.
- Giuffre M, Campigotto M, Campisciano G, et al. A story of liver and gut microbes: how does the intestinal flora affect liver disease? A review of the literature. Am J Physiol Gastrointest Liver Physiol. 2020 May 1;318(5):G889–G906.
- Betrapally NS, Gillevet PM, Bajaj JS. Gut microbiome and liver disease. Transl Res. 2017 Jan;179:49–59.
- Altamirano-Barrera A, Uribe M, Chavez-Tapia NC, et al. The role of the gut microbiota in the pathology and prevention of liver disease. J Nutr Biochem. 2018 Oct;60:1–8.
- Lu H, Wu Z, Xu W, et al. Intestinal microbiota was assessed in cirrhotic patients with hepatitis B virus infection. Intestinal microbiota of HBV cirrhotic patients. Microb Ecol. 2011 Apr;61(3):693–703.
- Jun DW, Kim KT, Lee OY, et al. Association between small intestinal bacterial overgrowth and peripheral bacterial DNA in cirrhotic patients. Dig Dis Sci. 2010 May;55(5):1465–1471.
- Ponziani FR, Bhoori S, Castelli C, et al. Hepatocellular carcinoma is associated with gut microbiota profile and inflammation in nonalcoholic fatty liver disease. Hepatology. 2019 Jan;69(1):107–120.
- Yoshimoto S, Loo TM, Atarashi K, et al. Obesity-induced gut microbial metabolite promotes liver cancer through senescence secretome. Nature. 2013 Jul 4;499(7456):97–101.
- Department of Medical Administration NH, Health Commission of the People’s Republic of C. Guidelines for diagnosis and treatment of primary liver cancer in China (2019 edition) . Zhonghua Gan Zang Bing Za Zhi. 2020 Feb 20;28(2):112–128.
- Huang JN, Wen B, Zhu JG, et al. Exposure to microplastics impairs digestive performance, stimulates immune response and induces microbiota dysbiosis in the gut of juvenile guppy (Poecilia reticulata). Sci Total Environ. 2020 Sep 1;733:138929.
- Jiang W, Wang T, Liu C, et al. A 16S rRNA gene sequencing based study of oral microbiota in migraine patients in China. Bioengineered. 2021 Dec;12(1):2523–2533.
- Chen S, Zhou Y, Chen Y, et al. fastp: an ultra-fast all-in-one FASTQ preprocessor. Bioinformatics. 2018 Sep 1;34(17):i884–i890.
- Magoc T, Salzberg SL. FLASH: fast length adjustment of short reads to improve genome assemblies. Bioinformatics. 2011 Nov 1;27(21):2957–2963.
- Wang G, Li X, Zhou Y, et al. Effects of heat stress on gut-microbial metabolites, gastrointestinal peptides, glycolipid metabolism, and performance of broilers. Animals (Basel). 2021 Apr 30;11(5):1286.
- Edgar RC. UPARSE: highly accurate OTU sequences from microbial amplicon reads. Nat Methods. 2013 Oct;10(10):996–998.
- Peace TA, Brock KV, Stills HF Jr. Comparative analysis of the 16S rRNA gene sequence of the putative agent of proliferative ileitis of hamsters. Int J Syst Bacteriol. 1994 Oct;44(4):832–835.
- Wang Q, Garrity GM, Tiedje JM, et al. Naive Bayesian classifier for rapid assignment of rRNA sequences into the new bacterial taxonomy. Appl Environ Microbiol. 2007 Aug;73(16):5261–5267.
- Pushpanathan P, Mathew GS, Selvarajan S, et al. Gut microbiota and its mysteries. Indian J Med Microbiol. 2019 Apr-Jun;37(2):268–277.
- Marchesi JR, Adams DH, Fava F, et al. The gut microbiota and host health: a new clinical frontier. Gut. 2016 Feb;65(2):330–339.
- Chadha J, Nandi D, Atri Y, et al. Significance of human microbiome in breast cancer: tale of an invisible and an invincible. Semin Cancer Biol. 2021 May;70:112–127.
- Fang Y, Yan C, Zhao Q, et al. The roles of microbial products in the development of colorectal cancer: a review. Bioengineered. 2021 Dec;12(1):720–735.
- Dailey FE, Turse EP, Rossow B, et al. Probiotics for gastrointestinal and liver diseases: an updated review of the published literature. Endocr Metab Immune Disord Drug Targets. 2019;19(5):549–570.
- Ponziani FR, Nicoletti A, Gasbarrini A, et al. Diagnostic and therapeutic potential of the gut microbiota in patients with early hepatocellular carcinoma. Ther Adv Med Oncol. 2019;11:1758835919848184.
- Jasirwan COM, Muradi A, Hasan I, et al. Correlation of gut Firmicutes/Bacteroidetes ratio with fibrosis and steatosis stratified by body mass index in patients with non-alcoholic fatty liver disease. Biosci Microbiota Food Health. 2021;40(1):50–58.
- Turnbaugh PJ, Ley RE, Mahowald MA, et al. An obesity-associated gut microbiome with increased capacity for energy harvest. Nature. 2006 Dec 21;444(7122):1027–1031.
- Dominianni C, Sinha R, Goedert JJ, et al. Sex, body mass index, and dietary fiber intake influence the human gut microbiome. PLoS One. 2015;10(4):e0124599.
- Galle F, Valeriani F, Cattaruzza MS, et al. Mediterranean diet, physical activity and gut microbiome composition: a cross-sectional study among Healthy Young Italian adults. Nutrients. 2020 Jul 21;12(7). DOI:10.3390/nu12072164.
- Chen YH, Chiu CC, Hung SW, et al. Gnotobiotic mice inoculated with Firmicutes, but not bacteroidetes, deteriorate nonalcoholic fatty liver disease severity by modulating hepatic lipid metabolism. Nutr Res. 2019 Sep;69:20–29.
- Mouzaki M, Comelli EM, Arendt BM, et al. Intestinal microbiota in patients with nonalcoholic fatty liver disease. Hepatology. 2013 Jul;58(1):120–127.
- Ley RE. Obesity and the human microbiome. Curr Opin Gastroenterol. 2010 Jan;26(1):5–11.
- Grigor’eva IN. Gallstone Disease, Obesity and the Firmicutes/Bacteroidetes ratio as a possible biomarker of gut dysbiosis. J Pers Med. 2020 Dec 25;11:1.
- Cosorich I, Dalla-Costa G, Sorini C, et al. High frequency of intestinal TH17 cells correlates with microbiota alterations and disease activity in multiple sclerosis. Sci Adv. 2017 Jul;3(7):e1700492.
- Kozhieva M, Naumova N, Alikina T, et al. The core of gut life: firmicutes profile in patients with relapsing-remitting multiple sclerosis. Life (Basel). 2021 Jan 14;11(1):55.
- Fujio-Vejar S, Vasquez Y, Morales P, et al. The gut microbiota of healthy Chilean subjects reveals a high abundance of the phylum verrucomicrobia. Front Microbiol. 2017;8:1221.
- Ozato N, Saito S, Yamaguchi T, et al. Blautia genus associated with visceral fat accumulation in adults 20-76 years of age. NPJ Biofilms Microbiomes. 2019;5(1):28.
- Liu X, Mao B, Gu J, et al. Blautia-a new functional genus with potential probiotic properties? Gut Microbes. 2021 Jan-Dec;13(1):1–21.
- Kalyana Chakravarthy S, Jayasudha R, Sai Prashanthi G, et al. Dysbiosis in the gut bacterial microbiome of patients with uveitis, an inflammatory disease of the eye. Indian J Microbiol. 2018 Dec;58(4):457–469.
- Benitez-Paez A, Gomez Del Pugar EM, Lopez-Almela I, et al. Depletion of Blautia species in the microbiota of obese children relates to intestinal inflammation and metabolic phenotype worsening. mSystems. 2020 Mar 24;5:2.
- Luu TH, Michel C, Bard JM, et al. Proportion of Blautia sp. is associated with clinical stage and histoprognostic grade in patients with early-stage breast cancer. Nutr Cancer. 2017 Feb–Mar;69(2):267–275.
- Inoue R, Ohue-Kitano R, Tsukahara T, et al. Prediction of functional profiles of gut microbiota from 16S rRNA metagenomic data provides a more robust evaluation of gut dysbiosis occurring in Japanese type 2 diabetic patients. J Clin Biochem Nutr. 2017 Nov;61(3):217–221.
- Murri M, Leiva I, Gomez-Zumaquero JM, et al. Gut microbiota in children with type 1 diabetes differs from that in healthy children: a case-control study. BMC Med. 2013 Feb 21;11:46.
- Tong X, Xu J, Lian F, et al. Structural alteration of gut microbiota during the amelioration of human type 2 diabetes with hyperlipidemia by metformin and a traditional Chinese herbal formula: a multicenter, randomized, open label clinical trial. mBio. 2018 May 22;9(3). DOI:10.1128/mBio.02392-17.
- Jenq RR, Taur Y, Devlin SM, et al. Intestinal blautia is associated with reduced death from graft-versus-host disease. Biol Blood Marrow Transplant. 2015 Aug;21(8):1373–1383.
- Kakiyama G, Pandak WM, Gillevet PM, et al. Modulation of the fecal bile acid profile by gut microbiota in cirrhosis. J Hepatol. 2013 May;58(5):949–955.
- Indiani C, Rizzardi KF, Castelo PM, et al. Firmicutes/bacteroidetes ratio in the gut microbiota: a systematic review. Child Obes. 2018 Nov/Dec;14(8):501–509.
- Haro C, Rangel-Zuniga OA, Alcala-Diaz JF, et al. Intestinal microbiota is influenced by gender and body mass index. PLoS One. 2016;11(5):e0154090.
- Zhang YK, Zhang Q, Wang YL, et al. A comparison study of age and colorectal cancer-related gut bacteria. Front Cell Infect Microbiol. 2021;11:606490.