ABSTRACT
Accumulating evidence has shown many similarities and differences of gene profiles and pathways between pediatric and adult ulcerative colitis (UC) patients. In this study, we aimed to investigate the shared genes and pathways in intestinal tissues of pediatric and adult UC. Differentially expressed genes (DEGs) between pediatric and adult UC were identified via bioinformatic analysis of Gene Expression Omnibus datasets GSE87473 and GSE126124. Gene Ontology and pathway enrichment were used to analyze overlapped and distinguished DEGs. Gene Set Variation Analysis (GSVA) was utilized for contrast consistency. Mice colitis models were induced by dextran sulfate sodium (DSS) and Citrobacter rodentium. 2616 DEGs were screened out in intestinal tissues of adult UC compared with those of adult healthy controls, and 1195 DEGs in pediatrics. Same pathways between pediatric and adult UC were enriched using overlapped DEGs, mainly related to immune responses and metabolic processes, including butyrate metabolism, which was also identified by GSVA analysis. Of note, butyrate metabolism was the exclusive down-regulated pathway enriched by these two analyses, indicating that butyrate metabolism is one of the key pathways associated with both pediatric and adult UC. In addition, butyrate suppressed DSS-induced and Citrobacter rodentium-induced intestinal inflammation in mice. Therefore, the study revealed that butyrate metabolism was critical in both pediatric and adult UC. And butyrate suppressed colitis in mice, which provided a theoretical basis for the potential treatment of butyrate for UC patients.
Abbreviations: UC, Ulcerative colitis; IBD, Inflammatory bowel disease; DEGs, Differentially expressed genes; GEO, Gene Expression Omnibus; SVA, Spatial variant apodization; LIMMA, Linear models for the microarray data; FC, Fold change; GO, Gene Ontology; KEGG, Kyoto Encyclopedia of Genes and Genomes; GSVA, Gene Set Variation Analysis; MSigDB, Molecular Signatures Database; WT, Wild-type; DSS, Dextran sulfate sodium; HC, Healthy control; SD, Standard deviation; SNHG5, Small nucleolar RNA host gene 5; GLP-2, Glucagon-like peptide 2; GSE, Gene set enrichment; ECM, Extracellular matrix; TCA, Tricarboxylic acid cycle; NA, Not available.
1. Introduction
Ulcerative colitis (UC), which belongs to inflammatory bowel diseases (IBD), is a chronic inflammatory disease of the large intestine. Aberrant expression of various genes are associated with UC[Citation1]. For example, abnormal gene expressions related to immune function are among the critical factors in the pathogenesis of UC [Citation2]. Large quantities of cytokines are also involved in the occurrence and development of UC [Citation3]. Moreover, apoptosis-related genes and cancer-related genes are closely related to intestinal lesions and carcinogenesis of UC [Citation4]. There is no consensus that whether UC is caused by the infectious agents or genetic mutations. Indeed, UC does occur among family members, suggesting that genetic inheritance may be one of the causes [Citation5]. No clinical or demographic parameters are typically associated with therapeutic evaluation, indicating that additional information is still required to improve the definition of patient diagnosis. Currently, the use of microbial markers or gene expression to support specific subsets of UC diagnosis and adjustment of treatment methods are limited.
Diet involvement plays an important role in the pathogenesis and management of UC both in pediatric and adult patients [Citation6,Citation7]. The typical Western diet (high sugar intake with low intake of vegetables) may contribute to the development of UC [Citation8], because intestinal inflammation can be influenced in several pathways, including affecting the intestinal permeability, altering the gut microbiome, and dietary constituents acting as food antigens [Citation9]. As for the role of diet in the management of UC, enteral nutrition, which is supplemented with colostrum, probiotics, and transforming growth factor-β, produces limited treatment responses in UC patients [Citation10]. However, short-chain fatty acids, which are primary products of dietary fiber, consisting of acetate, propionate, and butyrate, could maintain intestinal homeostasis by increasing IL-22 in CD4 + T cells and innate lymphoid cells [Citation11], so that it could improve clinical outcomes potentially.
Accumulating evidence demonstrated that there are similarities and differences between pediatric and adult UC in pathologic changes, symptoms, extraintestinal manifestations, and disease severity. For example, UC tends to be more extensive and aggressive in pediatric UC patients than adult counterparts, and monogenic etiologies are more highly represented in pediatric-onset patients compared with those diagnosed at an older age [Citation12]. Various transcriptomic studies were performed to uncover the gene profiles of UC, including several efforts to discriminate between disease phases [Citation13–15], sample sources (blood versus tissue) [Citation16], microbiota association [Citation17], genders [Citation18], etc. However, the similarity and difference of gene profiles between pediatric and adult UC are still not completely characterized.
In this study, we aimed to identify the overlapped differentially expressed genes (DEGs) between pediatric and adult UC, and then the DEGs were enriched in several essential pathways and functions. We hypothesized that butyrate metabolism would be critical in both pediatric and adult UC patients, which would provide a theoretical basis for the potential treatment of butyrate for UC patients.
2. Materials and methods
2.1. Datasets and processing
GSE8747319 and GSE12612420 were retrieved from Gene Expression Omnibus (GEO) database (http://www.ncbi.nlm.nih.gov/geo/) [Citation19], and the expression profiling arrays were generated using GPL1315819 and GPL624420, respectively. All samples in those microarrays were divided into four groups based on age and disease status: pediatric control, pediatric UC, adult control, and adult UC (Supplementry Material 1). To remove the batch effects and normalization, the algorithm of spatial variant apodization (SVA) was performed [Citation20]. After that, the k-nearest neighbors [Citation21] algorithm was used to process the missing value. Averages were considered gene expression values if the multiple probes were mapped into the same gene symbol.
2.2. DEGs in intestine tissues
To analyze the DEGs in adult control (n = 21) and UC (n = 87), pediatric control (n = 20) and UC (n = 39), linear models for the microarray data (LIMMA) package were used to analyze gene profiles of tissue samples in R Studio (Version 3.6.3). Benjamini-Hochberg method was used to control the false discovery rate [Citation22]. Gene expression values with log2 fold change (FC) > 0.5 and adjusted p-value < 0.05 were defined as the cutoff criteria. Hierarchical clustering analysis was used to build a hierarchy of clusters of the DEGs [Citation23]. After getting the DEGs of tissues in pediatrics and adults, we took the intersection of those groups using VennDiagram (http://www.ehbio.com/Cloud_Platform/front). Overlapped and distinguished DEGs in pediatrics and adults were selected.
2.3. Function and pathway enrichment
These two analyses were based on the Metascape online database (http://metascape.org/) [Citation24]. After inputting the corresponding shared genes from 2.2, genes were collected and grouped into clusters based on the membership similarities. And the parameters were set as follows: (1) p-values < 0.01, (2) minimum counts of 3, and (3) enrichment factors > 1.5. The functional molecular clusters of Gene Ontology (GO) and pathway clusters Kyoto Encyclopedia of Genes and Genomes (KEGG) were selected as functional pathways. More specifically, p-values were calculated based on the accumulative hypergeometric [Citation25], and q-values using the algorithm of Benjamini-Hochberg were used to account for those comparisons [Citation26]. Moreover, to perform hierarchical clustering on the enriched terms, Kappa scores [Citation27] were also used as similarity metrics.
2.4. Gene Set Variation Analysis (GSVA)
GSVA is a nonparametric and unsupervised method for estimating changes in gene set enrichment through samples of expression data sets [Citation28]. The pathway datasets of GO and KEGG were downloaded from Molecular Signatures Database (MSigDB) v7.2 (https://www.gsea-msigdb.org/gsea/msigdb/index.jsp) [Citation29], and tissue probe matrix was used to perform the pathway matrix both in pediatrics and adults. In the study, GSVA was applied to build pathway-centric models of biology and to explore the potential regulatory pathways. The results of 2.3 and 2.4 were combined to identify the significant pathways in pediatrics and adults.
2.5. Mice
Specific pathogen-free C57BL/6 wild-type (WT) mice were purchased from Guangdong Medical Laboratory Animal Center. Mice were housed under standard conditions and cared for according to the institutional guidelines for animal care. The protocols for animal experiments followed the international guidelines and were approved by the Animal Care Committee of the Southern Medical University of China.
2.6. Citrobacter rodentium infection model
WT mice were orally infected with Citrobacter rodentium (5 × 108/ mouse), and mice were administered with or without 200 mM butyrate in drinking water. Mice were sacrificed on day 10. Mouse weights were monitored daily.
2.7. Dextran sulfate sodium (DSS)-induced colitis model
WT mice were administered with 2% DSS in the presence or absence of 200 mM butyrate in drinking water for 7 days, followed by another 3 days of water with or without 200 mM butyrate. Mice were sacrificed on day 10. Mouse weights were monitored daily.
2.8. The inflamed colons of adult UC patients
All patients with UC were recruited from the Department of Gastroenterology, the Third Affiliated Hospital of Guangzhou Medical University. The diagnosis of UC was based on clinical symptoms, endoscopic examination and histological findings. The Institutional Review Board approved this study for Clinical Research of the Third Affiliated Hospital of Guangzhou Medical University (2,021,063). And the characteristics of healthy controls (HC) and UC patients were described in Supplementary Material 2.
2.9. Quantitative RT-PCR
The total RNA was extracted from the colon with TRIzol and followed by cDNA synthesis. Quantitative PCRs were performed by using SYBR Green Gene Expression Assays for EHHADH, HMGCS2, ACSM3, and BDH2 on a Bio-Rad iCycler (Bio-Rad Laboratories, Hercules, CA), and all data were normalized to GAPDH mRNA expression. All the primers were ordered from Integrated DNA Technologies, and listed in Supplementary Material 3.
2.10. Histopathological assessment
At necropsy, mice colons were separated, and Swiss rolls of each were prepared. Tissues were fixed in 10% buffered formalin. Following paraffin embedding, 5-μm sections were prepared and stained with H&E. The severity of colitis was evaluated by a pathological scoring system [Citation30]. The score of 0–3, denoting increasingly severe abnormality, was assigned for each of these parameters and added together to calculate the overall histological score (no signs of inflammation, 0; increased mucosal mononuclear cells were present, 1; increased mucosal and submucosal or transmural mononuclear cells were present, 2; mucosal regeneration with crypt distortion as well as increased crypt proliferation or erosions or ulcers, 3).
2.11. Statistical analysis
Student’s t-test was used for comparison when data were normally distributed, and Mann–Whitney U test was used to measure the difference when data were not normally distributed. All the tests were two-sided. All the data were analyzed using Prism 9.0 (GraphPad Software, San Diego, CA), and presented as mean ± standard deviation (SD). All the data were presented as mean ± SD. *p < 0.05, **p < 0.01, ***p < 0.001.
2.12. Ethical considerations
The research was carried out according to the Helsinki Declaration and approved by the Ethics Committee of Nan Fang Hospital, Southern Medical University, Guangzhou, Guangdong Province, China (No. L2017010).
3. Results
In this bioinformatics study, we found that gene expressions in UC differed from healthy controls both in pediatric and adult populations. The overlapped DEGs between pediatric and adult UC were identified and then they were enriched in several essential pathways and functions. Notably, butyrate metabolism was critical in both pediatric and adult UC patients. And butyrate suppressed colitis in mice, potentially supporting the treatment of butyrate for UC patients.
3.1. Study population and design
In GSE87473 dataset [Citation31], 19 colon samples of pediatric UC patients, 87 colon samples of adult UC patients, and 21 colon samples of adult controls with specific age and disease extent were reported. Meanwhile, in GSE126124 dataset [Citation32], 21 colon tissues of pediatric controls and 20 colon tissues of pediatric UC patients with specific age and gender were reported. After a combination of GSE87473 and GSE126124 datasets (Supplementary Material 1), four groups (pediatric UC, pediatric control, adult UC, and adult control) with intrinsic details of age, including the pediatric group (6–18 years old) and the adult group (>18 years old), were included in the further analysis (). Gene expressions of colon biopsies from adult UC patients and healthy controls, as well as pediatric UC patients and healthy controls, were analyzed to identify molecular signatures of UC in pediatrics and adults. These analyses elucidated key genetic functions and pathways involved in disease pathogenesis, which can be used to identify the shared DEGs, functions, and pathways in pediatric and adult UC patients ().
3.2. Functional enrichment analyses of overlapped DEGs in intestinal tissues of pediatric and adult UC
After screening DEGs based on the cutoff criteria of p-value < 0.05 and [log2FC] > 0.5 (Supplementary Material 4), 3811 DEGs in intestinal tissues of pediatric and adult UC were shown in volcano plots (). A total of 2616 DEGs, including 1535 up-regulated genes and 1081 down-regulated genes, were screened out in intestinal tissues of adult UC compared with those of healthy adult controls (), while 1195 DEGs, including 550 up-regulated genes and 645 down-regulated genes, were found in intestinal tissues of pediatric UC compared with those of healthy pediatric controls ().
Figure 2. DEGs between UC and healthy control. (a) DEGs in adults in Volcano plots. (b) DEGs in pediatrics in Volcano plots. Each symbol represents a gene, and gold or blue color indicates up-regulated or down-regulated genes, respectively. DEGs, Differentially expressed genes; UC, Ulcerative colitis
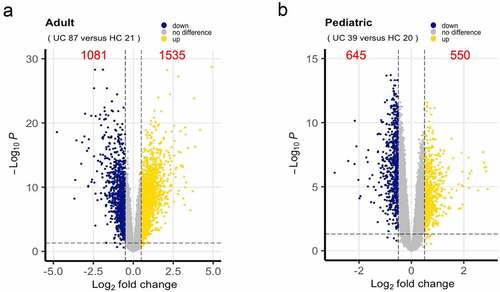
To further identify similar and distinguished DEGs in intestinal tissues between pediatric and adult UC, we compared these two sets of UC patients’ data. 425 up-regulated genes and 352 down-regulated genes were overlapped in pediatric and adult UC (). As shown in , overlapped up-regulated DEGs were mainly enriched in pathways, such as cytokine-cytokine receptor interaction, rheumatoid arthritis, cell adhesion molecules, pertussis, and staphylococcus aureus infection, while function analysis revealed that GO sets such as extracellular matrix structural constituent, glycosaminoglycan binding, integrin binding, cytokine activity, carbohydrate binding and so on were significantly associated with the overlapped up-regulated genes between pediatric and adult UC, of which top 20 GO sets were shown in Supplementary Material 5A. Meanwhile, the top 20 enriched pathways and GO sets of overlapped down-regulated genes were shown in and Supplementary Material 5B. Interestingly, metabolism was the most relevant biological process when analyzing similar down-regulated DEGs in pediatric and adult UC. Seven different metabolic processes were enriched, including drug metabolism-cytochrome P450, tyrosine metabolism, sulfur metabolism, butyrate metabolism, nitrogen metabolism, glycerophospholipid metabolism, and starch and sucrose metabolism.
Figure 3. Enrichment analyses of overlapped DEGs in pediatric and adult UC. (a) Overlapped DEGs in pediatric and adult UC were shown in Venn diagram. (b) Top 20 KEGG pathways were enriched using overlapped up-regulated DEGs. (c) Top 20 KEGG pathways were enriched using overlappped down-regulated DEGs. The enriched analyses were ranked by p-value. DEGs, Differentially expressed genes; UC, Ulcerative colitis; KEGG, Kyoto Encyclopedia of Genes and Genomes
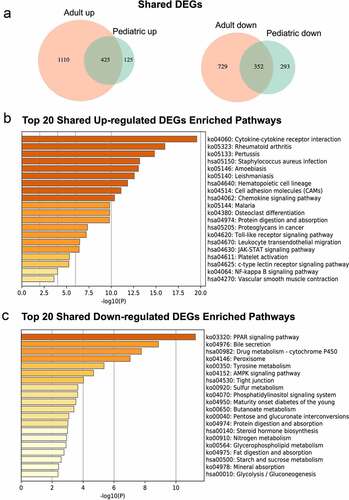
3.3. GSVA analysis of tissue microarray data
A non-parametric unsupervised method GSVA was accessed for gene set enrichment (GSE) in colonic tissue microarray data. It analyzed the changes in the coordinate system and converted the data from a sample matrix gene to a sample matrix set gene, then the enrichment of each sample could be evaluated [Citation33]. This transformation is completed without using a phenotype, thus facilitating compelling and open analysis based on the current path. As shown in , 590 and 148 enrichment analyses were associated with up-regulated genes and down-regulated genes were found in adult UC compared with healthy adult controls, respectively, while 365 and 125 enrichment analyses associated with up-regulated genes and down-regulated genes were found in pediatrics. We also found that there were 221 and 23 enrichment analyses associated with up-regulated pathways and down-regulated pathways in both pediatric and adult UC (). And KEGG associated pathways were shown in .
Table 1. Shared KEGG pathways enrichment analysis of GSVA
Figure 4. Shared gene sets between pediatric and adult UC using GSVA. (a) The differences in pathway enrichment between UC and healthy control in pediatric and adult were visualized by Volcano plot using GSVA. (b) Venn diagram of shared enrichment pathways in pediatric and adult UC. UC, Ulcerative colitis; GSVA, Gene Set Variation Analysis
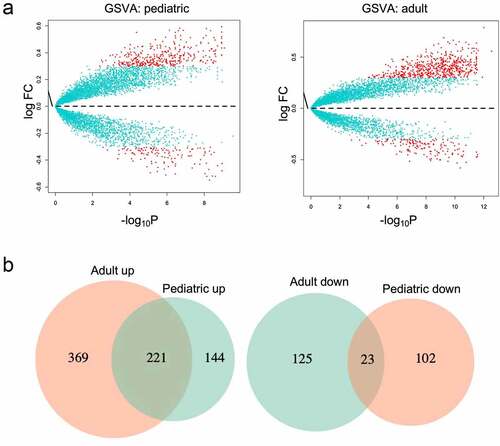
3.4. Identify butyrate metabolism as a key pathway associated with UC in both pediatric and adult populations
Next, we compared the results which were obtained from the above two analyses, and we found that 8 pathways (7 up-regulated and 1 down-regulated) were presented in these two analyses (). Of note, butanoate metabolism, which was also called butyrate metabolism, was the only one down-regulated pathway, indicating butyrate metabolism may be a key pathway associated with UC in both pediatric and adult populations.
Table 2. Comparison of GSVA and metascape analysis
3.5. The validation of gene expression levels in adult UC patients
Based on our previous analysis, we found four genes showed downregulation associated with butyrate metabolism, and we validated colonic HMGCS2, EHHADH, ACSM3, and BDH2 expression in the inflamed mucosa of UC patients. As shown in , HMGCS2, EHHADH, and ACSM3 were significantly decreased in the inflamed mucosa of UC patients compared with healthy controls. However, the expression of BDH2 was almost at the same level both in UC patients and healthy controls ().
3.6. The protective role of butyrate in regulation of intestinal inflammation in mice
Given that butyrate is closely associated with UC, we next investigated the role of butyrate in regulation of colitis. We induced colitis using Citrobacter rodentium infection models. Mice were infected with Citrobacter rodentium and administered with or without butyrate in drinking water. We found that feeding butyrate prevented mice against weight loss and suppressed intestinal inflammation in mice infected with Citrobacter rodentium (). Mice fecal C. rodentium CFU alos decreased after administrated with butyrate (). To further confirm the protective role of butyrate in suppressing colitis, we induced colitis using DSS in mice, and mice were given with or without butyrate in drinking water. Similar to Citrobacter rodentium infection model, butyrate suppressed intestinal inflammation, which was evidenced by decreased weight loss and lower pathology scores (). Moreover, we identified the genes expression regulations after butyrate use in mice models with intestinal inflammation using RT-PCR. The expression of HMGCS2 and ACSM3 were significantly higher in the group that combined butyrate with DSS-induced than those only treated with DSS, while the expression of EHHADH and BDH2 were significantly decreased in butyrate-treated groups (). Taken together, these results demonstrated that butyrate protected the intestines from inflammation induced by both intestinal acute injury and enteric infection.
Figure 6. Butyrate inhibits intestinal inflammation in mice. (a) Mice were orally treated with C.rodentium (5 × 108 CFU/mice) and treated with or without butyrate (200 mM) in drinking water for 10 days. Monitored mice weight every day. (b) Colonic histopathology was analyzed when mice were sacrificed on day 10. (c) Collected the feces and measured the CFU on day 4. (d) Mice were administrated with 2% DSS in drinking water for 7 days and switched to untreated water for another 3 days. Treated with or without butyrate (200 mM) in drinking water for 10 days, and mouse weights were monitored daily. (e) Histopathological change and histological score in of colon in mice colitis models. **p < 0.01. CFU, Colony forming unit; DSS, Dextran sulfate sodium
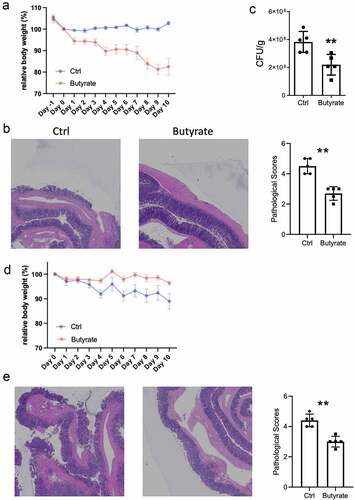
4. Discussion
Currently, the diagnosis of UC mainly depends on epidemiological data, clinical performance, and systematic examination, including laboratory examination, digestive endoscopy, imaging, and pathological examination. However, the diagnosis of UC, including pediatric and adult UC, still lacks gold standards. Therefore, it is necessary to understand the mechanisms underlying the pathogenesis of UC better, and it is crucial to identify the similarities and differences of gene expressions and pathways between pediatric and adult UC. In this bioinformatics study, based on GSE8747319 and GSE12612420 datasets, four groups (pediatric UC, pediatric control, adult UC, and adult control) with intrinsic details of age were included in the analysis, and we found that gene expressions in UC differed from healthy controls both in pediatric and adult populations. In addition, overlapped DEGs between pediatric and adult UC were enriched in several essential pathways and functions, in which butyrate metabolism was critical in both pediatric and adult UC populations.
Interestingly, based on GSVA, we found that butanoate metabolism was the only one down-regulated pathway after two analyses, indicating a critical role for butanoate metabolism in regulating the pathogenesis of UC in both pediatrics and adults. Moreover, butyrate is a cooperative product of many symbiotic bacteria in the intestine. Many studies have confirmed that there was a variety of symbiotic bacteria in the intestine that can produce butyrate directly [Citation34–36]. A small number of bacteria promote the growth of specific bacteria to increase the production of butyrate. Synthetic short-chain fatty acid precursors indirectly lead to an increase in butyrate content [Citation37]. Therefore, supplementation of butyrate can correct the intestinal bacterial dystrophy caused by UC and adjust the excessive release of pro-inflammatory cytokines by activating multiple metabolism-related pathways. Moreover, butyrate activates the regulatory of T cells, further reducing the production of TNF-α and IL-6 [Citation38,Citation39], meanwhile, it could increase the expression of Hif1α and Ahr in CD4 T cells to promote the production of cytokine IL-22, leading to inhibiting intestinal inflammation [Citation11]. In addition, butyrate was also shown to have the ability to inhibit a variety of pro-inflammatory signaling pathways through histone deacetylase inhibitor-dependent and independent mechanisms [Citation40].
It is contradictory that several studies suggested that butyrate supplementation could be beneficial for UC patients [Citation41,Citation42], while other studies showed no effect or even negative effect on intestinal inflammation [Citation43,Citation44]. Specifically, butyrate caused more significant down-regulation on gene expression of inflammatory pathways in colon tissues without inflammation of UC patients that those with inflammation [Citation45]. Meanwhile, Vancamelbeke et al [Citation46]. concluded that butyrate supplementation could not protect against inflammation-induced negative effects on epithelial barrier function. Instead, it strongly up-regulated the expression of inflammatory mRNA and protein. The above-mentioned could partly explain why anti-inflammatory effects of butyrate in UC patients are not always acquired, and the mechanisms of butyrate on UC should be investigated in future studies. However, due to the small sample sizes of both two studies, the results should be further validated. Despite of the indecisive results, butyrate is considered as a therapeutic alternative for UC patients based on its potential anti-inflammatory properties.
It is critical to compare our study with the previous studies on the investigation of butyrate metabolism. For example, the study performed by Preter et al [Citation47]. concluded that the deficiency of butyrate metabolism in UC patients was caused by the decreased expression level of SLC16A1 and enzymes in the butyrate oxidation pathway. And there were differences between our study and the above-mentioned. First, both pediatric and adult UC patients and controls were included in our study instead of merely including adult populations, which may improve the generality of our results. Second, our study newly added EHHADH, HMGCS2, BDH2, and RT-PCR further validated the decreased expression of those three genes in UC patients. Third, due to the deficiency of details of treatment in GSE87473 and GSE126124 datasets, the treatment response of gene expression was not investigated in our study. Meanwhile, the results of our study were not further validated in pediatric UC patients owing to the lack of pediatrics in our hospital. And a further multi-center study with complete details of both pediatric and adult UC patients would be carried out to investigate the gene expression after treatment and make a further validation of the results.
Several characteristics of the patients, including gender, country, and treatment, may affect the butyrate metabolism pathway. There are limited studies being performed to investigate whether gender, and country affect the butyrate metabolism pathway. Indeed, gender and country factors are associated with the risk of UC development (accompanied by impaired butyrate metabolism) [Citation48]. On the one hand, as for gender factor, a higher prevalence of smoking in males might have caused the higher mortality rate of UC than females, because smoking is considered one of the most significant environmental factors of UC [Citation49]. And the influence of sex hormones on UC prognosis based on the brain-gut–microbiota axis is still not completely studied. On the other hand, as for country factor, the country development degree and the related genetic factors contribute to the UC development. The incidence of UC is significantly lower in developing countries than in developed ones [Citation48]. And the genetic risk factors are different between Asian and Western regions in UC [Citation50]. Furthermore, the treatment factor may affect the butyrate metabolism pathway. Preter et al [Citation47]. found that the originally decreased gene expression of ACSM3 in butyrate metabolism pathway could return to control values after infliximab therapy.
There were eight pathways found in the study, including seven shared up-regulated pathways and one shared down-regulated pathway (butyrate metabolism). As for the butyrate metabolism, the connection between these four genes, including (ACSM3, EHHADH, HMGCS2, and BDH2) and butyrate metabolism pathways should be illustrated (Supplementary Material 6). First, ACSM3 participates in the synthesis of butyryl-CoA. Next, EHHADH is associated with the synthesis of Acetoacetyl-CoA which then creates 3-Hydroxymethyl-glutaryl-CoA participated with HMGCS2. And BDH2 joins the acetoacetate to create 3-Hydroxybutanoate. Currently, biologic treatment for UC mostly target symbols such as TNF and IL in up-regulated pathways, leading to more researches focusing on inhibiting symbols in up-regulated pathways. For example, the inhibition of IL1B and IL6 could ameliorate colitis based on the anti-inflammatory and anti-oxidant activity [Citation51], both of which were involved in the shared up-regulated pathways, including cytokine activity, cytokine-cytokine receptor interaction, graft versus host disease, and type I diabetes mellitus. Meanwhile, CXCL10 was associated with UC progression[Citation1], and it was up-regulated in cytokine activity, complement and coagulation cascades, cytokine-cytokine receptor interaction, and systemic lupus erythematosus, which shed light on the drug target for UC patients. After a comparison of pediatric and adult UC patients, the shared pathways would be identified to improve the generality of the study. Considering the overlaps of symbols in the shared up-regulated pathways, researchers could focus on targeting the overlapped symbols to inhibit the up-regulated pathways to suppress colitis.
Besides the butyrate supplementation as a potential treatment for UC patients, several emerging alternatives could be effective in the treatment of UC. In the aspect of gene, the knockdown of the lncRNA (small nucleolar RNA host gene 5) SNHG5 could counteract TNF-α reduced dysfunction of intestinal epithelial cells in UC patients, which may be a promising therapy for UC [Citation52]. Meanwhile, in the aspect of protein, increased serum glucagon-like peptide 2 (GLP-2) level, a hormone secreted by L-cells of intestinal mucosa, inducing reduced microbiota diversity and abundance, may be considered a treatment for UC patients [Citation53].
However, there are still some limitations to be addressed. First, the sample size was small without gender, country, and treatment factors. And an improved bioinformatic analysis with a large sample size would be conducted to improve the accuracy of association between genes and UC both in pediatric and adult patients. Additionally, the four genes enriched in the butyrate metabolism were not measured and validated in pediatric UC patients due to the lack of pediatric patients in our hospital. And a further multi-center study with both pediatric and adult UC patients would be carried out to validate the hypothesis. Finally, the molecular network and genes involved in UC would be investigated to better dissect how to counteract UC onset and progression, such as deeply studying samples from UC patients.
5. Conclusion
The study revealed that butyrate metabolism was critical in association with both pediatric and adult UC. And butyrate suppressed colitis in mice, which provided a theoretical basis for the potential treatment of butyrate for UC patients.
Research highlights
Shared genes and pathways in intestinal tissues of pediatric and adult UC were explored.
Butyrate metabolism was critical in association with both pediatric and adult UC.
Butyrate suppressed colitis in mice models.
The study provided a theoretical basis for the potential treatment of butyrate for UC patients.
Authors’ contributions
Zheng Zhou and Mingsong Li designed and conducted the analysis. Zheng Zhou, Jiasheng Cao, and Xiaoming Liu wrote the manuscript. Mingsong Li revised the munascript. All authors read and approved the final manuscript.
Supplemental Material
Download ()Acknowledgements
We acknowledge the generous support of the Guangdong Provincial Key Laboratory of Gastroenterology, Department of Gastroenterology, Nanfang Hospital, Southern Medical University. This work was supported by the National Natural Science Foundation of China (Grant/Award number: 82070565, 81870389); Guangdong Provincial Bio-engineering Research Center for Gastroenterology Diseases; the Science and Technology Planning Project of Guangdong Province (Grant/Award number: 2017B020209003). We thank Sarun Juengpanich and Win Topatana, who are English native speakers in Department of General Surgery, Sir Run-Run Shaw Hospital, Zhejiang University School of Medicine, Hangzhou 310016, Zhejiang Province, China, for revising and polishing our manuscript.
Disclosure statement
No potential conflict of interest was reported by the author(s).
Supplementary material
Supplemental data for this article can be accessed here.
Availability of data and material
The datasets used and/or analyzed during this study are available from the corresponding author upon reasonable request.
Additional information
Funding
References
- Shi W, Zou R, Yang M, et al. Analysis of genes involved in ulcerative colitis activity and tumorigenesis through systematic mining of gene Co-expression networks. Front Physiol. 2019;10:662. 10.3389/fphys.2019.00662.
- Sarlos P, Kovesdi E, Magyari L, et al. Genetic update on inflammatory factors in ulcerative colitis: review of the current literature. World J Gastrointest Pathophysiol. 2014;5(3):304–321.
- Senhaji N, Serrano A, Badre W, et al. Association of inflammatory cytokine gene polymorphisms with inflammatory bowel disease in a Moroccan cohort. Genes Immun. 2016;17(1):60–65.
- Chakrabarty S, Varghese VK, Sahu P, et al. Targeted sequencing-based analyses of candidate gene variants in ulcerative colitis-associated colorectal neoplasia. Br J Cancer. 2017;117(1):136–143.
- Childers RE, Eluri S, Vazquez C, et al. Family history of inflammatory bowel disease among patients with ulcerative colitis: a systematic review and meta-analysis. J Crohns Colitis. 2014;8(11):1480–1497.
- Magro F, Gionchetti P, Eliakim R, et al. Third European Evidence-based Consensus on Diagnosis and management of ulcerative colitis. part 1: definitions, diagnosis, extra-intestinal manifestations, pregnancy, cancer surveillance, surgery, and Ileo-anal pouch disorders. J Crohns Colitis. 2017;11:649–670.
- Harbord M, Eliakim R, Bettenworth D, et al. Third European evidence-based consensus on diagnosis and management of ulcerative colitis. part 2: current management. J Crohns Colitis. 2017;11:769–784.
- Abegunde AT, Muhammad BH, Bhatti O, et al. Environmental risk factors for inflammatory bowel diseases: evidence based literature review. World J Gastroenterol. 2016;22(27):6296–6317.
- Chapman-Kiddell CA, Davies PS, Gillen L, et al. Role of diet in the development of inflammatory bowel disease. Inflamm Bowel Dis. 2010;16(1):137–151.
- Triantafillidis JK, Tzouvala M, Triantafyllidi E. Enteral nutrition supplemented with transforming growth factor-β, colostrum, probiotics, and other nutritional compounds in the treatment of patients with inflammatory bowel disease. Nutrients. 2020;12(4):1048.
- Yang W, Yu T, Huang X, et al. Intestinal microbiota-derived short-chain fatty acids regulation of immune cell IL-22 production and gut immunity. Nat Commun. 2020;11(1):4457.
- Jerger L, Hyams JS. Special considerations for biologic medications in pediatric ulcerative colitis. Expert Opin Biol Ther. 2020;20(4):429–435.
- Czarnewski P, Parigi SM, Sorini C, et al. Conserved transcriptomic profile between mouse and human colitis allows unsupervised patient stratification. Nat Commun. 2019;10(1):2892.
- Telesco SE, Brodmerkel C, Zhang H, et al. Gene expression signature for prediction of golimumab response in a phase 2a open-label trial of patients with ulcerative colitis. Gastroenterology. 2018;155(4):1008–1011.e8.
- Román J, Planell N, Lozano JJ, et al. Evaluation of responsive gene expression as a sensitive and specific biomarker in patients with ulcerative colitis. Inflamm Bowel Dis. 2013;19(2):221–229.
- Mesko B, Poliskal S, Szegedi A, et al. Peripheral blood gene expression patterns discriminate among chronic inflammatory diseases and healthy controls and identify novel targets. BMC Med Genomics. 2010;3(1):15.
- Moen AEF, Lindstrøm JC, Tannæs TM, et al. The prevalence and transcriptional activity of the mucosal microbiota of ulcerative colitis patients. Sci Rep. 2018;8(1):17278.
- Shah SC, Khalili H, Gower-Rousseau C, et al. Sex-Based differences in incidence of inflammatory bowel diseases—Pooled analysis of population-based studies from western countries. Gastroenterology. 2018;155:1079–1089.e3.
- Barrett T, Wilhite SE, Ledoux P, et al. NCBI GEO: archive for functional genomics data sets–update. Nucleic Acids Res. 2013;41(D1):D991–D995.
- Leek JT, Johnson WE, Parker HS, et al. The sva package for removing batch effects and other unwanted variation in high-throughput experiments. Bioinformatics. 2012;28(6):882–883.
- Irizarry RA, Hobbs B, Collin F, et al. Exploration, normalization, and summaries of high density oligonucleotide array probe level data. Biostatistics. 2003;4(2):249–264.
- Joo JWJ, Hormozdiari F, Han B, et al. Multiple testing correction in linear mixed models. Genome Biol. 2016;17(1):62.
- Serban N, Jiang H. Multilevel functional clustering analysis. Biometrics. 2012;68(3):805–814.
- Zhou Y, Zhou B, Pache L, et al. Metascape provides a biologist-oriented resource for the analysis of systems-level datasets. Nat Commun. 2019;10(1):1523.
- Zar JH. Biostatistical analysis. Englewood Cliffs (N.J): Prentice-Hall; 1984.
- Hochberg Y, Benjamini Y. More powerful procedures for multiple significance testing. Stat Med. 1990;9(7):811–818.
- Cohen J. A coefficient of agreement for nominal scales. Educ Psychol Meas. 1960;20(1):37–46.
- Hänzelmann S, Castelo R, Guinney J. GSVA: gene set variation analysis for microarray and RNA-Seq data. BMC Bioinformatics. 2013;14(1):7.
- Subramanian A, Tamayo P, Mootha VK, et al. Gene set enrichment analysis: a knowledge-based approach for interpreting genome-wide expression profiles. Proc Natl Acad Sci U S A. 2005;102(43):15545–15550.
- Wu W, Sun M, Zhang HP, et al. Prolactin mediates psychological stress-induced dysfunction of regulatory T cells to facilitate intestinal inflammation. Gut. 2014;63(12):1883–1892.
- Li K, Strauss R, Ouahed J, et al. Molecular comparison of adult and pediatric ulcerative colitis indicates broad similarity of molecular pathways in disease tissue. J Pediatr Gastroenterol Nutr. 2018;67(1):45–52.
- Palmer NP, Silvester JA, Lee JJ, et al. Concordance between gene expression in peripheral whole blood and colonic tissue in children with inflammatory bowel disease. PLoS One. 2019;14(10):e0222952.
- Foroutan M, Bhuva DD, Lyu R, et al. Single sample scoring of molecular phenotypes. BMC Bioinformatics. 2018;19(1):404.
- Gyuraszova M, Kovalcikova A, Gardlik R. Association between oxidative status and the composition of intestinal microbiota along the gastrointestinal tract. Med Hypotheses. 2017;103:81–85.
- Qi YF, Sun JN, Ren LF, et al. Intestinal microbiota is altered in patients with gastric cancer from Shanxi Province, China. Dig Dis Sci. 2019;64(5):1193–1203.
- Sorbara MT, Littmann ER, Fontana E, et al. Functional and genomic variation between human-derived isolates of lachnospiraceae reveals inter- and intra-species diversity. Cell Host Microbe. 2020;28(1):134–146.e4.
- Sun M, Wu W, Liu Z, et al. Microbiota metabolite short chain fatty acids, GPCR, and inflammatory bowel diseases. J Gastroenterol. 2017;52(1):1–8.
- Mandaliya DK, Patel S, Seshadri S. The Combinatorial effect of acetate and propionate on high-fat diet induced diabetic inflammation or metaflammation and T cell polarization. Inflammation. 2021;44(1):68–79.
- Yang W, Cong Y. Gut microbiota-derived metabolites in the regulation of host immune responses and immune-related inflammatory diseases. Cell Mol Immunol. 2021;18(4):866–877.
- Ferrer-Picón E, Dotti I, Corraliza AM, et al. Intestinal inflammation modulates the epithelial response to butyrate in patients with inflammatory bowel disease. Inflamm Bowel Dis. 2020;26(1):43–55.
- Assisi RF. Combined butyric acid/mesalazine treatment in ulcerative colitis with mild-moderate activity. Results of a multicentre pilot study. Minerva Gastroenterol Dietol. 2008;54:231–238.
- Vernia P, Annese V, Bresci G, et al. Topical butyrate improves efficacy of 5-ASA in refractory distal ulcerative colitis: results of a multicentre trial. Eur J Clin Invest. 2003;33(3):244–248.
- Steinhart AH, Hiruki T, Brzezinski A, et al. Treatment of left-sided ulcerative colitis with butyrate enemas: a controlled trial. Aliment Pharmacol Ther. 1996;10(5):729–736.
- Vernia P, Monteleone G, Grandinetti G, et al. Combined oral sodium butyrate and mesalazine treatment compared to oral mesalazine alone in ulcerative colitis: randomized, double-blind, placebo-controlled pilot study. Dig Dis Sci. 2000;45(5):976–981.
- Magnusson MK, Isaksson S, Öhman L. The anti-inflammatory immune regulation induced by butyrate is impaired in inflamed intestinal mucosa from patients with ulcerative colitis. Inflammation. 2020;43(2):507–517.
- Vancamelbeke M, Laeremans T, Vanhove W, et al. Butyrate does not protect against inflammation-induced loss of epithelial barrier function and cytokine production in primary cell monolayers from patients with ulcerative colitis. J Crohns Colitis. 2019;13(10):1351–1361.
- De Preter V, Arijs I, Windey K, et al. Impaired butyrate oxidation in ulcerative colitis is due to decreased butyrate uptake and a defect in the oxidation pathway. Inflamm Bowel Dis. 2012;18(6):1127–1136.
- Alatab S, Sepanlou SG, Ikuta K. The global, regional, and national burden of inflammatory bowel disease in 195 countries and territories, 1990-2017: a systematic analysis for the Global Burden of Disease Study 2017. Lancet Gastroenterol Hepatol. 2020;5(1):17–30.
- Law ST, Li KK. Gender-related differences in clinical course of crohn?s disease in an Asian population: a retrospective cohort review. Arq Gastroenterol. 2014;51(2):90–96.
- Yoshitake S, Kimura A, Okada M, et al. HLA class II alleles in Japanese patients with inflammatory bowel disease. Tissue Antigens. 1999;53(4):350–358.
- N PS, Kalyankumarraju M, Puppala ER, Ahmed S, et al. Zanthoxylum alatum Roxb. seed extract ameliorates stress aggravated DSS-induced ulcerative colitis in mice: plausible role on NF-κB signaling axis. J Ethnopharmacol. 2021;279:114385.
- Li H, Xuan J, Zhang W, et al. Long non-coding RNA SNHG5 regulates ulcerative colitis via microRNA-375/Janus kinase-2 axis. Bioengineered. 2021;12(1):4150–4158.
- Li D, Yang Y, Yin X, et al. Glucagon-like peptide (GLP) −2 improved colonizing bacteria and reduced severity of ulcerative colitis by enhancing the diversity and abundance of intestinal mucosa. Bioengineered. 2021;12(1):5195–5209.