ABSTRACT
Cardiomyocyte apoptosis and autophagy play important roles in acute myocardial infarction (AMI), but the effect of leucine-rich alpha-2-glycoprotein 1 (LRG1) on the apoptosis and autophagy of H9c2 has not yet been reported. It was found through differential gene analysis and LASSO analysis that LRG1 was the key gene in AMI. In this study, western blot was applied to detect the protein expression of Bax, Bcl2, LC3, p62, LRG1 and hypoxia-inducible factor-1α (HIF-1α); CCK-8 assay was employed to detect cell viability; Annexin V-FITC/PI staining was adopted to evaluate apoptosis, and immunofluorescence assay was applied to detect autophagy. Under hypoxia conditions in H9c2 cells, LRG1 protein levels were increased, the cell activity was decreased, and apoptosis and autophagy were promoted; the downregulated LRG1 significantly enhanced cell viability but inhibited apoptosis and autophagy. When knocking down HIF-1α in the overexpressed LRG1 cells, the effects of LRG1 were reversed under hypoxia condition. In conclusion, LRG1/HIF-1α promoted H9c2 cell apoptosis and autophagy in hypoxia, potentially providing new ideas for the determination and treatment of AMI.
Abbreviation: LRG1: Leucine-rich alpha-2-glycoprotein 1; LRR: leucine-rich repeat; HIF-1α: Hypoxia-inducible factor-1α; AMI: acute myocardial infarction
Introduction
Acute myocardial infarction (AMI) is characterized by acute onset, high mortality and disability rates. It accounts for 50% of the world’s cardiovascular deaths and is the leading killer of human beings [Citation1]. AMI is a kind of ischemic heart disease, which mainly refers to myocardial necrosis caused by acute and persistent ischemia and hypoxia of the coronary artery [Citation2]. Cardiomyocyte injury is mainly resulted from irreversible apoptosis of some myocardial cells [Citation2]. In the early stages of AMI, cardiomyocyte injury is predominantly apoptotic [Citation3]. Besides, apoptosis is considered as the main source of myocardial cell loss after myocardial infarction (MI) and an important factor in ventricular remodeling and the development of heart failure [Citation4,Citation5]. Autophagy can be activated by cell ischemia, hypoxia and nutritional deficiency. Studies have shown that cardiomyocyte autophagy can be rapidly activated in the early stages of MI and can rescue myocardial cells in the infarct margin area and limit the expansion of MI area [Citation6,Citation7]. On the contrary, excessive autophagy will lead to the development of cardiovascular diseases; for example, prolonged hypoxia can induce the autophagic death of cardiomyocytes [Citation8]. Therefore, cardiomyocyte apoptosis and autophagy play crucial parts in AMI.
In this study, three AMI datasets were downloaded from the Gene Expression Omnibus database (GEO, http://www.ncbi.nlm.nih.gov/geo/) and analyzed by bioinformatics. It was found that leucine-rich alpha-2-glycoprotein 1 (LRG1) is a key gene related to AMI. LRG1, as a member of the leucine-rich repeat (LRR) family, was first isolated from human serum in 1977 [Citation9]. It is a secretory glycoprotein, which contains eight repeated common sequences, and each of its sequences consists of 24 amino acid residues [Citation10]. LRG1 was involved in cell proliferation, immune response, apoptosis, cell migration and angiogenesis [Citation11–14]. Moreover, LRG1 is overexpressed in cancers, including pancreatic, bladder, ovarian and biliary tract cancers [Citation15–18]. However, the role of LRG1 in AMI has not been reported yet.
Hypoxia-inducible factor-1α (HIF-1α) was first discovered while studying the gene function of hepatoma cells [Citation19]. HIF-1α was found regulating the transcription of many hypoxia-related genes, such as basic metabolism, survival, differentiation and apoptosis of cells [Citation20]. HIF-1α is also involved in the downstream signal transduction process of hypoxic response [Citation21]. HIF-1α/BNIP3 pathway can induce the autophagy and apoptosis of H9c2 during myocardial ischemia–reperfusion injury [Citation22]. In this study, the effect of LRG1/HIF-1α on the apoptosis and autophagy of hypoxia-induced H9c2 cells was explored.
This study aimed to research the role of LRG1 in AMI, and it was verified by in vitro cell experiments that LRG1/HIF-1α can regulate cell apoptosis and autophagy.
Materials and methods
Bioinformatics analysis (differential gene analysis + LASSO analysis)
The datasets of AMI were downloaded from the GEO database: GSE66360 (49 AMI patients + 50 normal subjects) [Citation23], GSE123342 (65 AMI patients + 64 AMI patients 30 days after AMI + 37 AMI patients 1 year after AMI + 22 stable coronary artery diseases (CADs) + 4 technical repeats) [Citation24] and GSE62646 (28 AMI patients (sampled at admission, discharge, and 6 months after discharge) + 14 stable CADs) [Citation25]. Limma was used for differential gene analysis [Citation26]. For GSE66360, the differences between AMI patients and normal subjects were analyzed; for GSE123342, the differences between 65 AMI patients and 22 CAD patients were analyzed; and for GSE62646, the differences between 28 AMI patients (on admission) and 14 CAD patients were analyzed. The results of difference analyses of these three datasets were taken as the intersection to identify the differentially expressed genes (DEGs, different from normal people or CAD) in AMI patients. These DEGs were analyzed by gene ontology (GO) terms and Kyoto encyclopedia of Genes and Genomes (KEGG) pathway enrichment analysis [Citation27]. The protein–protein interaction (PPI) network analysis was performed on these genes using STRING online software [Citation28]. The DEGs were analyzed by LASSO in three datasets to determine the final key genes [Citation29].
Cell culture
H9c2 was grown in DMEM-F12 medium (Gibco, USA) with 10% fetal bovine serum and cultured in a closed incubator with 5% CO2 and 95% relative humidity at 37°C. The logarithmic-phase cells were treated with hypoxia (1% O2, 5% CO2, and 94% N2) to establish a hypoxia cell model [Citation30].
CCK-8 assay
H9c2 cells were treated as per different experimental protocols. Then, according to the instructions of CCK-8 Kit (DOJINDO, Japan), CCK-8 reagent was added into each well. After 4 h of incubation, the absorbance of each group was detected by an enzyme-labeled instrument at 450 nm, and the cell viability was calculated.
Annexin V-FITC/PI staining
After 12 h of hypoxia treatment, the apoptosis rate of cardiomyocytes H9c2 was measured. The hypoxia-treated H9c2 was collected. After centrifugation at 1500 rpm for 5 min, the medium was discarded, and the cells were collected. The cells were resuspended with a binding buffer (Sigma Company, USA), and the cell concentration was 1 × 106 cells/ml. Then, 5 µl Annexin V-FITC was added, mixed well, and incubated in the dark for 10 min at 25°C, while 10 µl PI (final concentration of 50 µl/ml) was added and incubated in the dark for 5 min at 4°C. FACS420 flow cytometry was used to detect and calculate the cell mortality. This experiment was conducted three times repeatedly, and the average value was obtained.
Immunofluorescence
GFP-LC3 plasmid was transfected into H9c2. After the relevant treatment according to different experimental protocols, H9c2 was cultured overnight in a constant temperature incubator. Cells were fixed with 4% paraformaldehyde at room temperature for 15 min and stained with DAPI at room temperature in the dark for 10 min. Then, the cells were sealed with mounting medium (DAKO, Denmark) and dried and finally observed under a fluorescence microscope [Citation31].
Western blot
The expression of Bax, Bcl2, LC3, p62, LRG1 and HIF-1α proteins in H9c2 cardiomyocyte was detected by western blot. General procedure: collecting cardiomyocytes H9c2 treated by certain experiments, extracting the total cell protein, and measuring the protein concentration by BCA method (Cell Signaling Technology (CST), USA). After SDS-PAGE electrophoresis, the protein samples were then transferred to PVDF membrane and blocked with skimmed milk powder, followed by the addition of primary antibody (Bax (#14796, CST, 1:1000), Bcl2 (#3869, CST, 1:1000), LC3 (#3868, CST, 1:1000), p62 (#23214, CST, 1:1000), LRG1 (ab181882, Abcam, 1:1000), and HIF-1α (#14179, CST, 1:1000)), and incubated overnight at 4°C. On the following day, peroxidase-labeled goat anti-rabbit IgG (1:5000) was added and incubated for 2 h on a horizontal decolorization shaker. An ECL kit (Beyotime Biotechnology, Shanghai, China) was applied to detect the bands of the western blot. β-actin (#8457, CST, 1:1000) was applied as internal reference.
Transfection
Rat cardiomyocytes H9c2 were seeded in six-well plates with 5 × 105 cells per well. When the cells were fused to about 80%, the serum-free medium was replaced, and siLRG1, siHIF-1α, LRG1 overexpression plasmids or their corresponding negative controls (siNC or OE-NC) were transfected according to the instructions of Lipofectamine (Thermo Fisher, USA). Serum-free Opti-MEM medium was taken and incubated for 5 min at room temperature by adding 6 µl Lipofectamine 3000. After that, siNC, siLRG1, siHIF-1α, OE-NC or LRG1 overexpression plasmids were added to mix them well. After incubation at 25°C for 20 min, the mixture was added to the cells and cultured with the cells for 6 h. The culture medium was removed and replaced with DEME-F12 medium containing 10% fetal bovine serum. The cells were further cultured for 24 h.
Statistical analysis
Statistical software SPSS 27.0 was employed to analyze the data. Data were expressed as mean ± standard deviation (x ± s), and t-test was used to compare data between the two groups. One-way ANOVA was applied to compare data between multiple groups. When P < 0.05, the difference was regarded statistically significant.
Results
In this study, we aimed to research the role of LRG1 in AMI, and it was verified that LRG1 could promote hypoxia-induced cardiomyocyte apoptosis and autophagy by regulating HIF-1α. At first, it was found through differential gene analysis and LASSO analysis that LRG1 was the key gene in AMI. Then, rat cardiomyocytes H9c2 were treated with hypoxia to establish a hypoxia cell model. Apoptosis and autophagy were evaluated by Annexin V-FITC/PI staining and immunofluorescence assay. Finally, siHIF-1α was applied to verify that LRG1 produced a marked effect by regulating HIF-1α.
Bioinformatics analysis results
As seen from the results of the difference analysis, there were 377 DEGs in GSE66360 (301 were upregulated and 76 were downregulated); 671 DEGs in GSE123342 (606 were upregulated and 65 were downregulated); and 396 DEGs in GSE62646 (175 were upregulated and 221 were downregulated) ()). Using the Venn diagram, 13 common upregulated DEGs and 1 common downregulated DEG were found among the three datasets ()). Through GO enrichment analysis, these 14 shared DEGs were shown mainly enriched in GO terms such as tertiary granule, and neutrophil activation involved in immune response ()). KEGG enrichment analysis displayed that these genes were significantly enriched in the B-cell receptor signaling pathway, osteoclast differentiation, and other pathways ()). PPI network analysis of these genes revealed protein interactions in 10 of them ()). By LASSO analysis, 7 of 14 genes were retained in GSE62646, 11 in GSE6636 and 10 in GSE123342 (). The four genes simultaneously retained in the three datasets were LILRB2, ZFP36, LRG1 and CYP1B1, and they were all elevated at the onset of AMI and then returned to normal levels as observed in GSE123342 ()). Studies have reported that LRG1 can be used as a judgment index for the early occurrence and prognosis evaluation of AMI [Citation22], but the specific mechanism of action has not been investigated. In this study, through in vitro cell experiments, the role of LRG1 in cardiomyocyte injury was studied.
Table 1. LASSO analysis results of 14 common DEGs in 3 datasets
Figure 1. Bioinformatics analysis results. (a) The differential genes heatmap of GSE62646, GSE66360 and GSE123342; (b) Venn diagram of DEGs; (c) GO enrichment analysis of 14 common DEGs; (d) KEGG enrichment analysis of 14 common DEGs; (e) PPI analysis of common differential genes; (f) The expression of four key genes at different stages in GSE123342
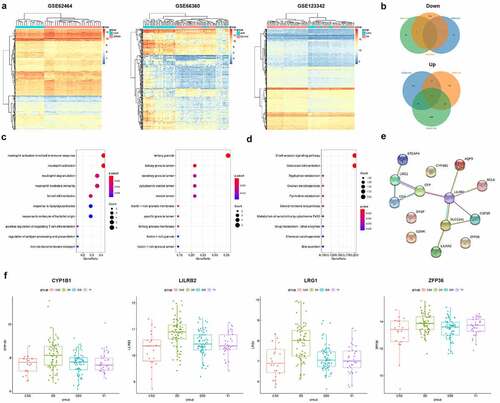
Hypoxia inhibits H9c2 cell viability, promotes apoptosis and autophagy, and promotes LRG1 expression
Hypoxia-induced cardiomyocyte H9c2 mimicked an AMI cell model in vitro. A significant decrease in cell viability was shown by CCK-8 assay under prolonged hypoxia treatment, but no significant change was displayed in cell viability beyond 12 h ()). According to Annexin V-FITC/PI staining results, the apoptotic rate increased after hypoxia treatment ()), and western blot results showed that the expression of pro-apoptotic protein Bax increased, while the expression of the anti-apoptotic protein Bcl2 decreased ()). To detect the autophagy of H9c2, immunofluorescence assay was used to detect the formation of LC3 punctations. After 12 h of hypoxia treatment, plenty of green punctations were formed in the cells, indicating that there were more autophagosomes ()); ) shows that the expression of autophagic protein LC3-II increased, while the expression of p62 decreased. The above results suggested that hypoxia inhibited the cell viability of H9c2 and promoted its apoptosis and autophagy. Besides, it was shown by western blot analysis that LRG1 expression was upregulated in hypoxia-treated H9c2 cardiomyocyte ()).
Figure 2. Effect of hypoxia on H9c2. (a) Cell viability was detected by CCK-8 after hypoxia treatment (0, 4, 8, 12, 18, 24 h); (b) Apoptosis was detected by Annexin V-FITC/PI staining; (c) Autophagy was detected by immunofluorescence assay (the scale bars = 50 μm); (d) Western blot was used to detect the expression of Bax, Bcl2, LC3, p62 and LRG1 protein. *P < 0.05, **P < 0.01, ***P < 0.001
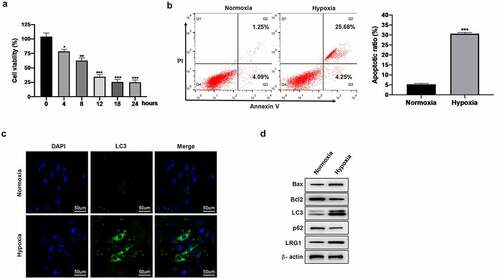
Downregulated LRG1 enhances the cell viability and inhibits the apoptosis of cardiomyocyte H9c2
To analyze the effect of LRG1 on H9c2 under hypoxia conditions, siNC or siLRG1 was transfected into H9c2 and then cultured under hypoxia conditions for 12 h. ) displays the good interference effect of siLRG1. CCK-8 results showed that the knockdown of LRG1 restored the reduced cell viability induced by hypoxic conditions ()). Additionally, the results of Annexin V-FITC/PI staining illustrated that the downregulation of LRG1 decreased the apoptotic rate of H9c2 in hypoxic conditions ()). We further explored the expression levels of apoptosis-related genes. Western blot results showed that the knockdown of LRG1 under hypoxic conditions reduced the expression of Bax and promoted the expression of Bcl-2 ()). The above results indicated that the downregulation of LRG1 enhanced the viability of cardiomyocyte H9c2 and inhibited their apoptosis under hypoxia conditions.
Figure 3. Effect of LRG1 on cell viability and cell apoptosis in H9c2. (a) The interference effect of siLRG1 was detected by western blot; (b) Cell viability was detected by CCK-8; (c) Apoptosis was detected by Annexin V-FITC/PI staining; (d) Western blot was used to detect the expression of Bax and Bcl2 protein. When normoxia as control, ***P < 0.001. When hypoxia + siNC as control, ##P < 0.01, ###P < 0.001
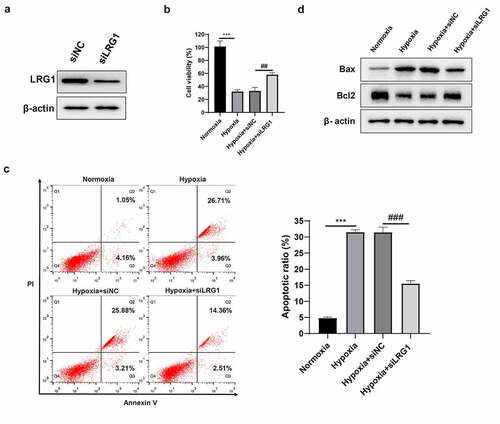
Downregulated LRG1 inhibits autophagy
H9c2 was transfected with siNC or siLRG1 and then incubated under hypoxia conditions for 12 h. Immunofluorescence experiments displayed that the downregulation of LRG1 resulted in fewer green bright punctations in cells, indicating that there were fewer autophagosomes and hypoxia-induced autophagy was inhibited ()). Western blot results showed that the downregulated LRG1 decreased the expression of autophagy protein LC3-II and increased the expression of p62 under hypoxic conditions ()). The above results indicated that downregulated LRG1 inhibited hypoxia-induced autophagy.
LRG1 regulates the expression of HIF-1α in H9c2
HIF-1α functions as a regulator of the adaptive response to hypoxia and plays an important role in AMI. It was found that LRG1 could affect hypoxia-induced apoptosis and autophagy, and we would further explore whether LRG1 could regulate the expression of HIF-1α. It was revealed by western blot results that LRG1 overexpression plasmid led to the increase of LRG1 and HIF-1α, while siLRG1 reduced the expression of LRG1 and HIF-1α (). The above results implied that LRG1 regulated the expression of HIF-1α in H9c2.
Downregulated HIF-1α reverses the effect of LRG1 on H9c2 viability and apoptosis
To analyze whether the effect of LRG1 on H9c2 cardiomyocyte is related to HIF-1α, H9c2 was transfected with OE-NC, LRG1, siNC or siHIF-1α and then cultured under hypoxia conditions for 12 h. A good interference effect of siHIF-1α is shown in ). The results of CCK-8 manifested that the overexpressed LRG1 decreased cell viability compared with the hypoxia+OE-NC group, and the knockdown of HIF-1α completely restored the effect of LRG1 on cell viability under hypoxic conditions ()). Annexin V-FITC/PI staining results revealed that compared with the hypoxia+OE-NC group, the overexpressed LRG1 significantly increased the apoptotic rate, and the depletion of HIF-1α decreased the effect of LRG1 in promoting apoptosis under hypoxic conditions ()). In addition, the overexpressed LRG1 promoted the expression of Bax but inhibited the expression of Bcl2, and the knockdown of HIF-1α reversed the regulation of LRG1 on Bax and Bcl2 ()). The above results showed that downregulated HIF-1α reversed the effect of LRG1 overexpression plasmid on cardiomyocyte H9c2 viability and apoptosis.
Figure 6. siHIF-1α reversed the effect of LRG1 on H9c2 viability and cell apoptosis. (a) The interference effect of siHIF-1α was detected by western blot; (b) Cell viability was detected by CCK-8; (c) Apoptosis was detected by Annexin V-FITC/PI staining; (d) Western blot was used to detect the expression of Bax and Bcl2 protein. When normoxia as control, ***P < 0.001. When hypoxia+OE-NC as control, ###P < 0.001. When hypoxia+LRG1+ siNC as control, &&P < 0.01
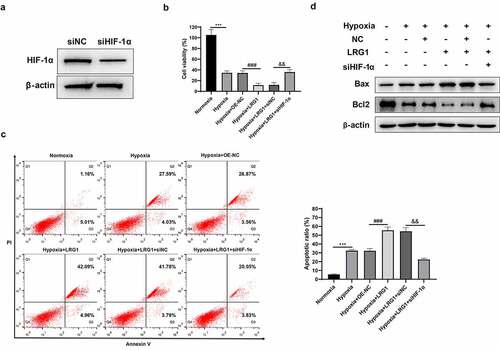
Downregulated HIF-1α reverses the effect of LRG1 on H9c2 autophagy
H9c2 was transfected with OE-NC, LRG1, siNC or siHIF-1α and then incubated under hypoxia conditions for 12 h. Immunofluorescence experiments showed that compared with the hypoxia+OE-NC group, the overexpressed LRG1 caused a substantial number of green bright punctations in the cells, indicating that autophagosomes were increased and autophagy was promoted ()). The results of western blot displayed that the expression of the autophagia protein LC3-II was significantly elevated, whereas p62 was decreased ()). Compared with the hypoxia + LRG1+ siNC group, autophagosomes were reduced, and autophagy was inhibited in the hypoxia + LRG1+ siHIF-1α group when the expression of HIF-1α was downregulated ()); correspondingly, the LC3-II expression was significantly reduced, and p62 expression was increased ()). The above results showed that downregulated HIF-1α reversed the effect of LRG1 overexpression plasmid on cardiomyocyte H9c2 autophagy.
Discussion
With the increasing stress in people’s lives, the incidence of AMI is on the rise and tends to be younger [Citation32]. Moreover, the mortality rate of cardiovascular diseases is higher than that of cancer, and more than half of all cardiovascular deaths are caused by AMI [Citation33]. In recent years, the search for marker molecules with high sensitivity and specificity for the timely diagnosis, prevention and control of the occurrence and development of AMI has become a research hotspot for the treatment and prognosis evaluation of AMI [Citation34,Citation35].
In this study, three AMI datasets were downloaded from GEO, and after the differential gene analysis was performed, and the intersection was taken, the common DEGs of the three datasets were obtained. These DEGs were then subjected to GO and KEGG enrichment analysis and PPI network analysis. To further narrow down the target genes studied, LASSO analysis was performed on the three datasets, and the results were intersected. Finally, four key genes related to AMI including LILRB2, ZFP36, LRG1, CYP1B1 were obtained. Besides, some studies have reported that LRG1 can be used as a predictor for the early occurrence and prognosis evaluation of AMI [Citation22], but the specific mechanism of LRG1 has not been reported yet.
In this study, an in vitro cell model was established by hypoxia treatment of cardiomyocyte H9c2. It was found that hypoxia inhibited the viability and promoted the apoptosis of H9c2 cells, and autophagy was also enhanced. It was also found that hypoxia treatment promoted the expression of LRG1 in cardiomyocyte H9c2. The results suggested that LRG1 might be associated with the apoptosis and autophagy of cells. We depleted the endogenous expression of LRG1 in H9c2, and an enhanced cell viability and a decrease in cell apoptosis and autophagy were observed under the hypoxic condition. Consistent with this phenomenon, we examined that the knockdown of LRG1 promoted the expression of Bcl2 but decreased the expression of Bax. Bax and Bcl2 are positively and negatively regulated apoptotic proteins. When the expression of Bcl2 is higher than that of Bax, the cell area survives; otherwise, the cell area is apoptotic [Citation36]. Meanwhile, the LRG1 protein expression was downregulated by siLRG1 in H9c2 treated with hypoxia, and it was found that the autophagosome became less, the expression of autophagic protein LC3-II decreased, and the expression of p62 increased. The results demonstrated that changes in LRG1 protein expression affected the apoptosis and autophagy of H9c2 cardiomyocyte.
HIF-1α, as an important regulator of MI, has been reported in numerous studies; for example, it was reported that HIF-1α/miR-10b-5p/PTEN affected hypoxia-induced cardiomyocyte apoptosis [Citation37]; and the enhanced expression of cytokines HIF-1α and vascular endothelial growth factor can promote angiogenesis after AMI [Citation38]. This study found that LRG1 upregulation significantly increased HIF-1α expression, while siLRG1 reduced the expression of HIF-1α. Furthermore, a study has reported that in colorectal cancer, HIF-1α is induced by LRG1 in a concentration- and time-dependent manner, and LRG1/HIF-1α can regulate epithelial–mesenchymal transition and angiogenesis [Citation39]. Meanwhile, LRG1 modulates HIF-1α stability and promotes keratinocyte migration and wound repair by elevating ELK3 levels [Citation40]. In this study, it was found that the downregulation of HIF-1α reversed the effects of LRG1 in H9c2.
Conclusion
All the above results indicated that LRG1/HIF-1α was associated with apoptosis and autophagy in hypoxia-induced H9c2 cardiomyocyte damage. Hypoxia reduces cell activity and promotes apoptosis and autophagy by upregulating the expression of LRG1 and HIF-1α. This study provides theoretical support for the role of LRG1 in AMI.
Highlights
It was found that LRG1 is the key gene in AMI by differential gene analysis and LASSO analysis.
Hypoxia inhibits H9C2 cell viability, promotes apoptosis and autophagy, and promotes LRG1 expression.
LRG1 promotes the apoptosis and autophagy of hypoxia-induced H9c2 cells by regulating HIF-1α.
Author contributions
Study design: Jiajie Feng
Data collection: Jiachen Zhan
Data analysis: Jiachen Zhan
Interpretation of data: Shuangshuang Ma
Draft manuscript: Jiajie Feng
Review manuscript: Shuangshuang Ma
Disclosure statement
No potential conflict of interest was reported by the author(s).
Data availability statement
The datasets used and/or analyzed during the current study are available from the corresponding author on reasonable request.
Additional information
Funding
References
- Schussler-Lenz M, Beuneu C, Menezes-Ferreira M, et al. Cell-based therapies for cardiac repair: a meeting report on scientific observations and European regulatory viewpoints. Eur J Heart Fail. 2016;18:133–141.
- White HD, Thygesen K, Alpert JS, et al. Clinical implications of the third universal definition of myocardial infarction. Heart. 2014;100:424–432.
- Hojo Y, Saito T, Kondo H. Role of apoptosis in left ventricular remodeling after acute myocardial infarction. J Cardiol. 2012;60:91–92.
- Minicucci MF, Azevedo PS, Polegato BF, et al. Heart failure after myocardial infarction: clinical implications and treatment. Clin Cardiol. 2011;34:410–414.
- Whelan RS, Kaplinskiy V, Kitsis RN. Cell death in the pathogenesis of heart disease: mechanisms and significance. Annu Rev Physiol. 2010;72:19–44.
- Chiong M, Wang ZV, Pedrozo Z, et al. Cardiomyocyte death: mechanisms and translational implications. Cell Death Dis. 2011;2:e244.
- Kanamori H, Takemura G, Goto K, et al. The role of autophagy emerging in postinfarction cardiac remodelling. Cardiovasc Res. 2011;91:330–339.
- Liu X, Deng Y, Xu Y, et al. MicroRNA-223 protects neonatal rat cardiomyocytes and H9c2 cells from hypoxia-induced apoptosis and excessive autophagy via the Akt/mTOR pathway by targeting PARP-1. J Mol Cell Cardiol. 2018;118:133–146.
- Haupt H, Baudner S. [Isolation and characterization of an unknown, leucine-rich 3.1-S-alpha2-glycoprotein from human serum (author’s transl)]. Hoppe Seylers Z Physiol Chem. 1977;358:639–646.
- Takahashi N, Takahashi Y, Putnam FW. Periodicity of leucine and tandem repetition of a 24-amino acid segment in the primary structure of leucine-rich alpha 2-glycoprotein of human serum. Proc Natl Acad Sci U S A. 1985;82:1906–1910.
- Serada S, Fujimoto M, Terabe F, et al. Serum leucine-rich alpha-2 glycoprotein is a disease activity biomarker in ulcerative colitis. Inflamm Bowel Dis. 2012;18:2169–2179.
- Lynch J, Fay J, Meehan M, et al. MiRNA-335 suppresses neuroblastoma cell invasiveness by direct targeting of multiple genes from the non-canonical TGF-beta signalling pathway. Carcinogenesis. 2012;33:976–985.
- Zhong D, Zhao S, He G, et al. Stable knockdown of LRG1 by RNA interference inhibits growth and promotes apoptosis of glioblastoma cells in vitro and in vivo. Tumour Biol. 2015;36:4271–4278.
- Wang X, Abraham S, Jag M, et al. LRG1 promotes angiogenesis by modulating endothelial TGF-beta signalling. Nature. 2013;499:306–311.
- Furukawa K, Kawamoto K, Eguchi H, et al. Clinicopathological significance of leucine-rich alpha2-glycoprotein-1 in sera of patients with pancreatic cancer. Pancreas. 2015;44:93–98.
- Linden M, Lind SB, Mayrhofer C, et al. Proteomic analysis of urinary biomarker candidates for nonmuscle invasive bladder cancer. Proteomics. 2012;12:135–144.
- Andersen JD, Boylan KL, Jemmerson R, et al. Leucine-rich alpha-2-glycoprotein-1 is upregulated in sera and tumors of ovarian cancer patients. J Ovarian Res. 2010;3:21.
- Sandanayake NS, Sinclair J, Andreola F, et al. A combination of serum leucine-rich alpha-2-glycoprotein 1, CA19-9 and interleukin-6 differentiate biliary tract cancer from benign biliary strictures. Br J Cancer. 2011;105:1370–1378.
- Dong W, Gao D, Lin H, et al. New insights into mechanism for the effect of resveratrol preconditioning against cerebral ischemic stroke: possible role of matrix metalloprotease-9. Med Hypotheses. 2008;70:52–55.
- Ni Chroinin D, Asplund K, Asberg S, et al. Statin therapy and outcome after ischemic stroke: systematic review and meta-analysis of observational studies and randomized trials. Stroke. 2013;44:448–456.
- Chen L, Luo S, Yan L, et al. A systematic review of closure versus medical therapy for preventing recurrent stroke in patients with patent foramen ovale and cryptogenic stroke or transient ischemic attack. J Neurol Sci. 2014;337:3–7.
- Zhang Y, Liu D, Hu H, et al. HIF-1alpha/BNIP3 signaling pathway-induced-autophagy plays protective role during myocardial ischemia-reperfusion injury. Biomed Pharmacother 2019; 120:109464.
- Muse ED, Kramer ER, Wang H, et al. A whole blood molecular signature for acute myocardial infarction. Sci Rep. 2017;7:12268.
- Vanhaverbeke M, Vausort M, Veltman D, et al. Peripheral blood RNA levels of QSOX1 and PLBD1 are new independent predictors of left ventricular dysfunction after acute myocardial infarction. Circ Genom Precis Med. 2019;12:e002656.
- Kiliszek M, Burzynska B, Michalak M, et al. Altered gene expression pattern in peripheral blood mononuclear cells in patients with acute myocardial infarction. PLoS One. 2012;7:e50054.
- Ritchie ME, Phipson B, Wu D, et al. Limma powers differential expression analyses for RNA-sequencing and microarray studies. Nucleic Acids Res. 2015;43:e47.
- Song X, Du R, Gui H, et al. Identification of potential hub genes related to the progression and prognosis of hepatocellular carcinoma through integrated bioinformatics analysis. Oncol Rep. 2020;43:133–146.
- Li L, Lei Q, Zhang S, et al. Screening and identification of key biomarkers in hepatocellular carcinoma: evidence from bioinformatic analysis. Oncol Rep. 2017;38:2607–2618.
- Tibshirani R. The LASSO method for variable selection in the Cox model. Stat Med. 1997;16:385–395.
- Liu J, Jiang M, Deng S, et al. miR-93-5p-containing exosomes treatment attenuates acute myocardial infarction-induced myocardial damage. Mol Ther Nucleic Acids. 2018;11:103–115.
- Sciarretta S, Yee D, Nagarajan N, et al. Trehalose-induced activation of autophagy improves cardiac remodeling after myocardial infarction. J Am Coll Cardiol. 2018;71:1999–2010.
- Gulati R, Behfar A, Narula J, et al. Acute myocardial infarction in young individuals. Mayo Clin Proc. 2020;95:136–156.
- Pollard TJ. The acute myocardial infarction. Prim Care. 2000;27:631–49;vi.
- Goretti E, Wagner DR, Devaux Y. miRNAs as biomarkers of myocardial infarction: a step forward towards personalized medicine? Trends Mol Med. 2014;20:716–725.
- Fathil MF, Md Arshad MK, Gopinath SC, et al. Diagnostics on acute myocardial infarction: cardiac troponin biomarkers. Biosens Bioelectron. 2015;70:209–220.
- Reed JC. Proapoptotic multidomain Bcl-2/Bax-family proteins: mechanisms, physiological roles, and therapeutic opportunities. Cell Death Differ. 2006;13:1378–1386.
- Wu L, Chen Y, Chen Y, et al. Effect of HIF-1alpha/miR-10b-5p/PTEN on hypoxia-induced cardiomyocyte apoptosis. J Am Heart Assoc. 2019;8:e011948.
- Chen Y, Fu L, Han Y, et al. Testosterone replacement therapy promotes angiogenesis after acute myocardial infarction by enhancing expression of cytokines HIF-1a, SDF-1a and VEGF. Eur J Pharmacol. 2012;684:116–124.
- Zhang J, Zhu L, Fang J, et al. LRG1 modulates epithelial-mesenchymal transition and angiogenesis in colorectal cancer via HIF-1alpha activation. J Exp Clin Cancer Res. 2016;35:29.
- Gao Y, Xie Z, Ho C, et al. LRG1 promotes keratinocyte migration and wound repair through regulation of HIF-1alpha stability. J Invest Dermatol. 2020;140:455–64 e8.