ABSTRACT
Brain-derived neurotrophic factor (BDNF) regulates neuronal plasticity by targeting the tyrosine kinase B receptor (TrkB) receptor, but limited researches concentrate on the role of BDNF/TrkB signaling in vestibular compensation. In this study, rats with unilateral vestibular dysfunction were established by unilateral labyrinthectomy (UL) and infusion with siBDNF or 7, 8-Dihydroxyflavone (7,8-DHF, a TrkB receptor agonist). The behavioral scores of rats with vestibular deficits were determined and the rotarod test was performed after UL. BDNF and TrkB levels after UL were determined by western blot and quantitative reverse transcription PCR (qRT-PCR). 5-bromo-2ʹ-deoxyuridine (BrdU)-positive cells (newly generated cells) and GAD67-positive cells (GABAergic neurons) were identified by immunohistochemistry. Glial fibrillary acidic protein (GFAP) (astrocyte marker)-positive cells were identified and GABA type A receptor (GABAAR) expression was detected by immunofluorescence. We found that after UL, BDNF and TrkB levels were up-regulated with a maximum value at 4 h, and then progressively down-regulated during 4 h ~ 7 d. Blocking BDNF/TrkB signaling inhibited the recovery from vestibular deficits, decreased the numbers of newly generated cells and astrocytes in medial vestibular nucleus (MVN), inferior vestibular nerve (IVN), superior vestibular nerve (SVN) and lateral vestibular nucleus (LVN), and disrupted the balances of GABAergic neurons and GABAAR expressions in the left (lesioned) side and right (intact) side of MVN, whereas activation of BDNF/TrkB signaling caused opposite results. The current study indicated that BDNF/TrkB signaling avails vestibular compensation, depending on the number of newly generated cells and astrocytes, the rebalance of GABAergic neurons, and GABAAR expression in bilateral MVN.
Introduction
The vestibule, as one of the main structures in the cerebellum, is closely related to the balance of body posture and can coordinate eyeball movement. Unilateral vestibular dysfunction or vestibulopathy abruptly shuts down the spontaneous resting potential of vestibular nuclei (VN) neurons on the damaged side of the brainstem, leading to an imbalance between the damaged side and intact contralateral VN neurons [Citation1]. This imbalance causes diverse signs and symptoms in different species, such as concussion [Citation2] and spontaneous nystagmus [Citation3], which will be ameliorated in days or weeks through vestibular compensation [Citation4]. Resting activities of bilateral VN neurons are rebalanced through vestibular compensation. However, the mechanism of vestibular compensation is complex and has not been fully elucidated.
VN complex mainly consists of four basic neuron nuclei: superior vestibular nucleus (SVN), inferior vestibular nucleus (IVN), medial vestibular nucleus (MVN) and lateral vestibular nucleus (LVN). Bilateral MVN is a common target in thiamine depletion and is related to acute vestibular failure [Citation5]. One of the causes of vestibular injury may be associated with the degeneration of peripheral vestibular receptor hair cells, or the changes in the number of neurons in the Scarpa ganglion or the brainstem VNC [Citation6]. It has been demonstrated that gamma amino butyric acid (GABA) is a critical inhibitory neurotransmitter in the cerebellovestibular pathway [Citation7] .
Brain-derived neurotrophic factor (BDNF), a dimeric protein, is a kind of neurotrophin (NT) that profoundly affects the development of the nervous system of vertebrates [Citation7]. Sophie Dutheil et al. [Citation1] found that in the early time window after unilateral vestibular neurectomy (UVN), a significant remodeling of BDNF-dependent excitatory markers occurred in the brainstem. This report also demonstrated that BDNF, which can regulate vestibular function recovery and neurogenesis in mammals, and/or KCC2 may represent a new therapeutic strategy at the vestibular scale. BDNF has a pleiotropic effect in the central nervous system. Acute and gradual increases in extracellular BDNF concentration cause the transient and continuous activation of tyrosine kinase B receptor (TrkB), a tyrosine protein kinase and its downstream signals, respectively, leading to differences in molecular and cellular functions [Citation8]. Studies have proved that the dysregulation of the BDNF/TrkB pathway is implicated in neurological diseases, such as depression [Citation9], as well as nerve damage in retinal degeneration [Citation10].
Based on the research cited above, it was hypothesized that BDNF/TrkB signaling could influence the recovery of vestibular compensation. The present study aimed to investigate whether BDNF/TrkB signaling impacts the time course of vestibular compensation, the plasticity mechanisms in VN, the rebalance of GABAergic neurons and the GABA type A receptor (GABAAR) in the left (lesioned) side and right (intact) side of MVN after unilateral vestibular dysfunction. The goal of this work is to shed a new insight into the development of therapeutic options to attenuate the vestibular symptoms and promote recovery of vestibular function.
Materials and methods
Ethics statement
All the animal experiments were performed in accordance with the guidelines of the China Council on Animal Care and Use. The protocol was approved by the Committee of Experimental Animals of Yongchuan Traditional Chinese Medicine Hospital of Chongqing (YTCMHC+20190158). Every attempt was made to minimize both pain and discomfort to the animals. The animal experiments were performed in Yongchuan Traditional Chinese Medicine Hospital of Chongqing.
Animals
Adult male Sprague–Dawley (SD) rats (200 ~ 250 g) were obtained from Shanghai SLAC Laboratory Animal Co., Ltd. The rats were housed in a temperature and humidity-controlled room under a normal 12 h light/dark cycle with food and water available.
Animal grouping and treatment
To construct a unilateral vestibular dysfunction model [Citation11] and determine the expressions of BDNF and TrkB at different indicated times (0 h, 4 h, 1 d, 2 d, 3 d, 5 d, 7 d), rats were divided into Sham (n = 4) and Model groups (n = 28). Rats in the Model group received unilateral labyrinthectomy (UL), while those in the Sham group received a sham operation. Four rats were selected from each group to assess vestibular deficits through behavioral scoring and rotarod test, and the remaining 24 rats in the Model group were used to determine the expressions of BDNF and TrkB by quantitative reverse transcription PCR (qRT-PCR) and western blot at different indicated times (0 h, 4 h, 1 d, 2 d, 3 d, 5 d, 7 d).
To further explore the functions of BDNF and TrkB in the development of vestibular compensation, rats were divided into five groups as follows: Sham (n = 20), Model (n = 20), CSF (n = 20), 7, 8-DHF (n = 20) and siBDNF (n = 20) groups.
SiBDNF group: the rats underwent complete UL and the right lateral ventricles of all rats were implanted with an osmotic minipump (Alzet) connected with a cerebral perfusion tube. SiRNAs targeting BDNF (siBDNF; ON-TARGETplus, Dharmacon; gene, Rat BDNF; GenBank accession number, NM 012513) were used to suppress translation and BDNF protein synthesis. SiBDNF was diluted to the required concentrations using artificial cerebrospinal fluid (CSF) [Citation1]. Subcutaneous minipumps (flow rate: 2.5 μL/h) were filled with artificial CSF containing siBDNF.
7, 8-DHF group: the rats underwent the same operative procedure as the siBDNF group, except that the minipumps (flow rate: 2.5 μL/h) were filled with artificial CSF containing 7, 8-Dihydroxyflavone (7,8-DHF; TrkB receptor agonist, 5.06300, Sigma).
CSF group: the rats underwent the same operative procedure as the siBDNF group, except that the rats were infused with artificial CSF only.
Four rats were selected from each group to assess vestibular deficits through behavioral scoring and rotarod test at different indicated times (4 h, 1 d, 2 d, 3 d, 5 d, 7 d). The remaining 16 rats in each group were selected for the detections of 5-bromo-2ʹ-deoxyuridine (BrdU)-positive cells on MVN by BrdU immunohistochemistry on day 3 (n = 4 each group), GABAAR expression in the left side and right side of MVN by GABAAR immunofluorescence on day 3 (n = 4 each group), glial fibrillary acidic protein (GFAP) in MVN, IVN, SVN and LVN by GAD67 immunohistochemistry on day 7 (n = 4 each group), and GAD67-positive cells in the left side and right side of MVN by GAD67 immunohistochemistry on day 7 (n = 4 each group).
Behavioral scoring for vestibular deficits
Postural asymmetry, head roll tilt, and nystagmus were assessed to score the vestibular deficits of rats with a maximum score of 10. The behavioral scoring for vestibular deficits was performed at 4 h and on day(s) 1, 2, 3, 4, 5 and 7 after surgery. The scores of postural asymmetry, head roll tilt and nystagmus were determined by using a rolling barrel, measuring the angle between the jaw and the horizontal plane, and visually inspecting, respectively. Details of the behavioral scoring were described in the published reports by Chang et al. [Citation11] and Filip Bergquist et al. [Citation12].
Rotarod test
The locomotor balance of rats was determined by rotarod test [Citation11]. In brief, before surgery, rats were trained on an automated four-lane rotarod unit (EGR FINE with 7-cm-diameter drums; Seoul, South Korea) for 10 d. The test was performed following the surgery. Rats were placed on a drum that was smoothly accelerated from 12 rpm/min to 16 rpm/min. The staying time of each rat on the drum was recorded as the latency to fall. After two adaptive learning trials, three more trials (T1–T3) were subsequently conducted. The rats were trained until their fall latency reached a plateau. Then in the test phase after surgery, the same protocol as the training phase was adopted. The rotarod scores were presented as the ratio of the latency to fall in the test phase to the longest latency to fall in the training phase.
Quantitative reverse transcription PCR (qRT-PCR)
Brain tissue from one rat was used as one sample for qRT-PCR and six samples were obtained at each time point from the rats in the surgical group. Total RNA was extracted using TRIzol® Reagent (15,596,018, ThermoFisher, USA). CDNAs were generated using a Takara RNA PCR Kit (RR036B, Takara). QPCR was performed in the 7500 Fast Real-Time PCR System (Applied Biosystems, Foster City, CA, USA) using a SYBR Green PCR Kit (RR820Q, Takara). GAPDH was synthetized by Sangon Biotech and used as an internal control. The mRNA levels in surgical group rats that were sacrificed at 0 h post operation were used as controls. The data were analyzed using the 2−ΔΔCT method [Citation13]. Primer sequences used in this research are listed in .
Table 1. Primer sequences used for quantitative reverse transcription PCR (qRT-PCR)
Western blot
Brain tissue from one rat was used as one sample for western blot and six samples were obtained at each time point from the rats in the surgical group. Total protein was extracted using RIPA buffer (R0010, Solarbio) and the concentration was measured using a BCA Protein Assay Kit (PC0020, Solarbio). The supernatants of the protein were separated by 15% SDS-PAGE and then transferred onto a PVDF membrane (FFP26, Beyotime, China). The membrane was blocked with 5% (w/v) skimmed milk for 1 h at 37°C, and then incubated with specific primary antibodies (listed in ) at 4°C overnight. Subsequently, the membrane was treated with HRP-conjugated secondary antibody (Goat Anti-Rabbit, 1:2000, ab205718, Abcam, USA) for 1 h at 37°C, and was then washed 3 times at 10-min intervals. The specific protein signal was detected with an ECL detection kit (P0018FS, Beyotime) and imaged using Image J software (Image J 1.8.0, National Institute of Health).
Table 2. List of primary antibodies used for western blots
Immunochemistry
BrdU immunohistochemistry
BrdU incorporated a thymidine analogue into newly synthesized DNA, which was used as a neurogenesis marker in this study. BrdU immunohistochemistry was performed as previous reported [Citation11]. On the 2nd day after surgery, rats received intraperitoneal injection of 300 mg/kg BrdU (B9285, Sigma). The next day, after being deeply anesthetized with 1.5% isoflurane, all rats were perfused with 0.9% saline and 4% (v/v) paraformaldehyde (PFA; A44124401, Sinopharm Chemical Reagent Co., Ltd). Brain tissues were removed from the skull, fixed in PFA overnight at 4°C, and then embedded in paraffin for preparing paraffin sections. The locations of MVN, IVN, SVN and LVN were identified according to the rat brain atlas reported by Paxinos et al. [Citation14]. Then the tissues of MVN were cut into sections (5 μm), placed on poly-L-lysine-coated glass slides, and fixed with formaldehyde. After deparaffinization and antigen retrieval using Lab Vision™ PT Module™ Deparaffinization and Heat-Induced Epitope Retrieval Solutions (100X) (TA-250-PM1X, Thermo Scientific) respectively, the slides were blocked with 1% H2O2 in methanol for 12 min, and then incubated with goat anti-BrdU (Biotin) antibody (ab2284, 1:2000, Abcam) at 4°C for 24 h. A streptavidin-HRP complex (A0303, 1:200, Beyotime, China) and 3,3 N-Diaminobenzidine Tertrahydrochloride (DAB, P0202, Beyotime) were used for detection, followed by hematoxylin staining (C0107, Beyotime). BrdU-positive cells were observed under a light microscope (CX31; Olympus, Tokyo, Japan). For quantification, the relative number of BrdU-positive cells was determined based on the total number of cells in each group. Each optical section resulted from three scanning averages.
GAD67 immunohistochemistry
GAD67 was expressed in GABAergic neurons that can be labeled by GAD67 immunomarkers. For the detection of GAD67-positive cells in the left (lesioned) side and right (intact) side of MVN, on the 7th day after surgery, all rats that had been anesthetized deeply with 1.5% isoflurane were perfused with 0.9% saline and 4% (v/v) PFA. The brain tissues were removed from the skull, fixed in PFA at 4°C overnight, and then embedded in paraffin for preparing paraffin sections. The tissues of the left side and right side of MVN were cut into sections (5 μm), placed on slides, and fixed. The slides were then sequentially incubated with the primary Mouse Anti-GAD67 antibody (ab26116, 1:2000 Abcam) and the secondary antibody HRP-conjugated Goat Anti-Mouse IgG H&L (ab205719, Abcam). After incubation in DAB for 8 min at 37°C, the slides were stained by hematoxylin staining at 37°C. GAD67-positive cells were observed under a light microscope (CX31; Olympus, Tokyo, Japan). For quantification, the relative number of GAD67-positive cells was determined based on the total number of cells in each group.
Immunofluorescence
Glial fibrillary acidic protein (GFAP) immunofluorescence
GFAP, a specific type of intermediate filament protein, was used as an astrocyte marker. GFAP immunofluorescence was performed as previously reported [Citation1]. On the 7th day post-surgery, rats were anesthetized deeply with 15% isoflurane, and perfused with 0.9% saline and 4% (v/v) PFA. After decapitation, the brain tissues were removed from the skull, fixed in PFA overnight at 4°C, and then embedded in paraffin for preparing paraffin sections. The tissues were cut into sections (5 μm), placed on slides, and fixed. Slides of MVN, IVN, SVN and LVN were incubated with the primary monoclonal Rabbit Anti-GFAP antibody (ab7260, 1:1000, Abcam) at 4°C overnight, and then treated with Goat Anti-Rabbit IgG H&L (Alexa Fluor® 594) (ab150080, 1:1000, Abcam). For quantification, mean intensity measurements of GFAP labeling was determined using image J software and relative to Sham group.
GABAA Receptor (GABAAR) immunofluorescence
GABAA R immunofluorescence was performed as previously reported [Citation1]. On the 3rd day post-surgery, rats were anesthetized deeply with 15% isoflurane, and perfused with 0.9% saline and 4% (v/v) PFA. After decapitation, the brain tissues were removed from the skull, fixed in PFA overnight at 4°C, and then embedded in paraffin for preparing paraffin sections. The tissues were cut into sections (5 μm), placed on slides, and fixed. Slides of the left (lesioned) side and right (intact) side of MVN were incubated with Mouse Anti-GABAA Receptor alpha 1 antibody (ab94585, Abcam) and HRP-conjugated Goat Anti-Mouse IgG H&L (ab205719, Abcam) in order. Fluorescence images were captured with an immunofluorescence laser confocal microscope (Nikon). Mean intensity measurements of GABAAR labeling was determined using image J software and relative to Sham group.
Statistical analysis
IBM SPSS software version 21.0 (IBM, NY, USA) was used for statistical analyses. Comparisons were performed by one-way analysis of variance (ANOVA). Tukey’s test was used as post-hoc test. The data were shown as mean ± standard deviation (S.D.).
Results
It was postulated that BDNF/TrkB signaling influences the time course of vestibular compensation. In terms of neuronal plasticity, such a signaling helps promote vestibular balance in VN. We addressed that activation of BDNF/TrkB signaling is crucial to make rebalance of homeostasis in bilateral MVN after UL. Newly generated cells, astrocytes and GABA were required for vestibular compensation. This work aimedto provide novel perspectives regarding the development of therapeutic options to alleviate the vestibular symptoms and favor recovery of vestibular function.
The time course of vestibular compensation after UL
The Sham group showed no vestibular deficits after sham surgery (). However, rats in the Model group showed severe vestibular deficits immediately following surgery. In the Model group, postural asymmetry was significantly decreased with time (), p < 0.01 or p < 0.001) and reached a minimum value close to that in the Sham group (), p < 0.05); head roll tilt was gradually improved and reached a maximum value approximately 7 d after surgery ()); and nystagmus was relieved signally 3 d following surgery and disappeared 5 d following surgery ()). Besides, the rotarod scores of rats in the Model group were increased over time following surgery (), p < 0.01 or p < 0.001) and reached a maximum value 5 d after surgery ()), while the rotarod scores of rats in the Sham group remained constant after surgery. Compared to 0 h, the expressions of BDNF and TrkB in rats of the Model group were starkly augmented at 4 h after surgery (, p < 0.001), but successively diminished during 4 h ~ 7 d after surgery and finally reached a stable level over time ().
Figure 1. Time course of vestibular compensation after unilateral labyrinthectomy. Twelve SD rats were divided into two groups: rats in the Model group underwent unilateral labyrinthectomy (n = 6) and those in the Sham group underwent a sham operation (n = 6). (a) Postural asymmetry scores of rats at 4 h and on day(s) 1, 2, 3, 5, 7 in the Sham and Model groups. (b) Head roll tilt scores of rats at 4 h and on day(s) 1, 2, 3, 5, 7 in the Sham and Model groups. (c) Nystagmus scores of rats at 4 h and on day(s) 1, 2, 3, 5, 7 in the Sham and Model groups. (d) Rotarod scores of rats at 4 h and on day(s) 1, 2, 3, 5, 7 in the Sham and Model groups. * p < 0.05 or ** p < 0.01 or *** p < 0.001 vs Sham. The data were shown as mean ± standard deviation (S.D.)
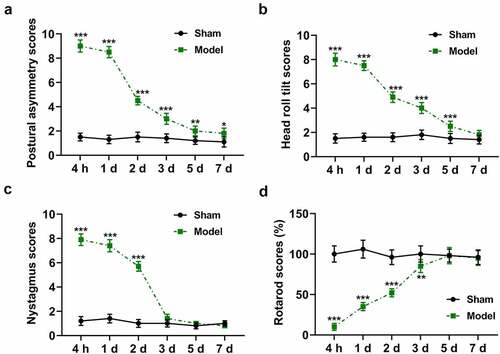
Figure 2. Time course of vestibular compensation after unilateral labyrinthectomy. (a and b) The expression of BDNF protein in the Model group during 0–7 d following surgery was determined by western blot. (a and c) The expression of TrkB protein in the Model group during 0–7 d following surgery was determined by western blot. (d) The expression of BDNF mRNA in the Model group during 0–7 d following surgery was determined by qRT-PCR. (e) The expression of TrkB mRNA in the Model group during 0–7 d following surgery was determined by qRT-PCR. GAPDH was used as an internal control. ** p < 0.01 or *** p < 0.001 vs 0 h. The data were shown as mean ± standard deviation (S.D.)
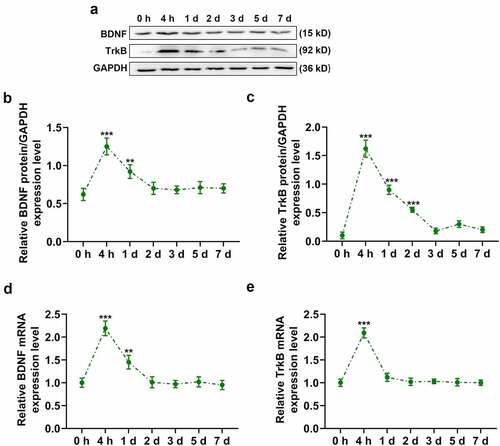
BDNF/TrkB signaling pathway was required for behavioral recovery after UL
To confirm whether the BDNF/TrkB signaling pathway regulates behavioral recovery of animals following UL, we infused artificial CSF, siBDNF, or 7,8-DHF intracerebroventricularly into rats for longitudinal behavioral analyses. The knock-down of BDNF and the effect of 7,8-DHF were detected using qPCR of BDNF and TrkB, respectively. The data revealed that the mRNA level of BDNF was prominently down-regulated, while that of TrkB was dramatically up-regulated on day 7 (Supplementary Fig. 1AB, p < 0.001). Compared to rats in the Model group, the vestibular compensation process in rats of CSF group was virtually unaffected (). In contrast with rats in the CSF group, the recovery of rats from vestibular compensation in the siBDNF group was remarkably inhibited, as evidenced by the repressed recovery from postural asymmetry (), p < 0.05 and p < 0.001), head roll tilt (), p < 0.05 and p < 0.001), nystagmus (), p < 0.001) and locomotor balance (), p < 0.001). However, the recovery of rats from vestibular compensation in 7,8-DHF group was observably promoted (, p < 0.01 or p < 0.001).
Figure 3. The BDNF/TrkB signaling pathway was required for behavioral recovery after unilateral labyrinthectomy. Thirty SD rats were divided into five groups: rats in the Model group underwent unilateral labyrinthectomy (n = 6); rats in the Sham group underwent a sham operation (n = 6); rats in the artificial cerebrospinal fluid (CSF) group underwent unilateral labyrinthectomy and were infused with CSF (n = 6); rats in the siBDNF group underwent unilateral labyrinthectomy and were infused with siBDNF (n = 6); and rats in the 7,8-DHF group underwent unilateral labyrinthectomy and were infused with 7,8-DHF, a TrkB agonist (n = 6). (a) Postural asymmetry scores of rats at 4 h and on day(s) 1, 2, 3, 5, 7 in the Sham, Model, CSF, siBDNF and 7, 8-DHF groups. (b) Head roll tilt scores of rats at 4 h and on day(s) 1, 2, 3, 5, 7 in the Sham, Model, CSF, siBDNF and 7, 8-DHF groups. (c) Nystagmus scores of rats at 4 h and on day(s) 1, 2, 3, 5, 7 d in the Sham, Model, CSF, siBDNF and 7, 8-DHF groups. (d) Rotarod scores of rats at 4 h and on day(s) 1, 2, 3, 5, 7 in the Sham, Model, CSF, siBDNF and 7, 8-DHF groups. * p < 0.05 or ** p < 0.01 or *** p < 0.001 vs. Sham; # p < 0.05 or ## p < 0.01 or ### p < 0.001 vs. CSF. CSF, cerebrospinal fluid; 7,8-DHF, a TrkB Agonist. The data were shown as mean ± standard deviation (S.D.)
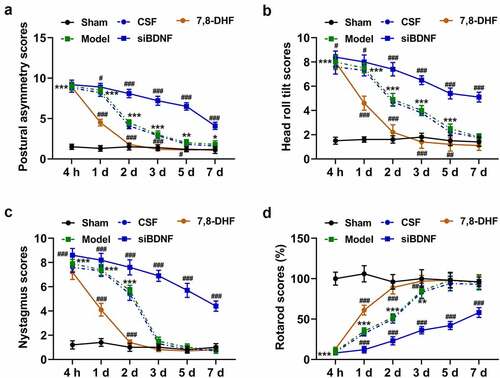
BDNF/TrkB signaling pathway influenced cell proliferation in VN
The results illustrated that compared with rats in the Model group, the number of BrdU-positive cells in VN (MVN, IVN, SVN and LVN) of rats in the CSF group experienced a moderate influence after UL (); by contrast, such number of rats in the siBDNF group after UL was evidently lessened (, p < 0.001), while an opposite result in IVN, SVN and LVN appeared in the 7,8-DHF group (, p < 0.001).
Figure 4. The BDNF/TrkB signaling pathway influenced the proliferation and survival of new cells in vestibular nucleus (MVN, IVN, SVN and LVN). (a) Photomicrographs showing BrdU immunostainings in vestibular nucleus (MVN, IVN, SVN and LVN) 3 d after unilateral labyrinthectomy with continuous drug infusion in the fourth ventricle (artificial CSF, siBDNF, or 7, 8-DHF). (b) The relative BrdU-positive cell in the vestibular nucleus from each group was determined. CSF, cerebrospinal fluid; 7,8-DHF, a TrkB Agonist. *** p < 0.001 vs. counterparts in Sham; ### p < 0.001 vs. counterparts CSF group
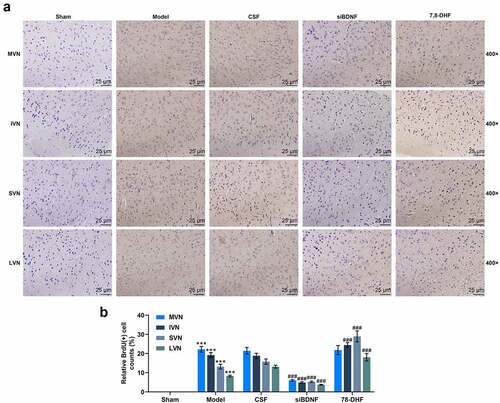
BDNF/TrkB signaling pathway influenced the numbers of astrocytes in VN
We further determined the influence of the BDNF/TrkB signaling pathway on astrocytes and microglia in VN (MVN, IVN, SVN and LVN). Compared to rats treated with CSF, the number of GFAP-positive cells in rats was overtly decreased by short-term infusion with siBDNF (, p < 0.01), but was appreciably elevated by 7, 8-DNF (, p < 0.001).
Figure 5. The BDNF/TrkB signaling pathway influenced astrocytes in vestibular nucleus (MVN, IVN, SVN and LVN). (a) Photomicrographs showing GFAP immunostainings in MVN, IVN, SVN and LVN 7 d after unilateral labyrinthectomy with continuous drug infusion in the fourth ventricle (artificial CSF, siBDNF, or 7, 8-DHF). (b) Histograms showing the GFAP immunostaining in both intact and lesioned lateral vestibular nucleus 7d after unilateral labyrinthectomy
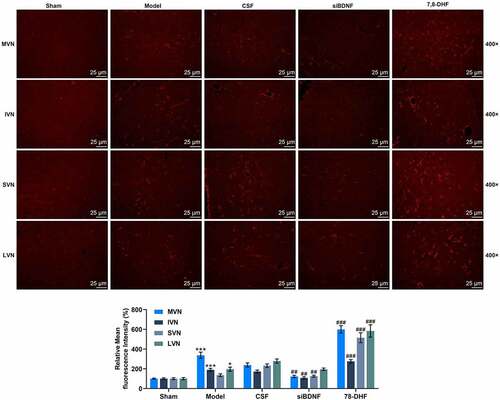
BDNF/TrkB signaling pathway influenced the number of GABAergic neurons in the left (lesioned) side and right (intact) side of MVN
To examine the impact of the BDNF/TrkB signaling pathway on neuromodulator systems in the vestibular compensation process, we applied GAD67 immunomarker to label GABAergic neurons in the left (lesioned) side and right (intact) side of MVN after UL (). The results showed that the number of GAD67-positive cells (GABAergic neurons) in the left side of rats in the CSF group was conspicuously boosted compared to that in the left side of rats in the Sham group (, p < 0.001), indicating a significant asymmetry between the two sides of the MVN complex. There was no significant difference in the left side and right side of MVN of rats between the Model and CSF groups (). When compared with rats in the CSF group, the number of GAD67-positive cells in the left and right sides of MVN in rats of the siBDNF group was observably decreased (, p < 0.01), and while the number of GAD67-positive cells in the right side of MVN in rats of the siBDNF group was significantly lower than that in the left side of MVN in rats of the siBDNF group (, p < 0.001). However, it’s almost the same on both sides of MVN in 7,8-DHF group (. The data implied that the BDNF/TrkB signaling pathway restored the balance of GABAergic neurons between the lesioned side and intact side of MVN after UL.
Figure 6. The BDNF/TrkB signaling pathway influenced the number of GABAergic neurons and GABAAR expression in the left (lesioned) side and right (intact) side of MVN. (a) Photomicrographs showing GAD67 immunostaining in the left (lesioned) side and right (intact) side of MVN 7 d after unilateral labyrinthectomy with continuous drug infusion in the fourth ventricle (artificial CSF, siBDNF, or 7, 8-DHF). (b) The relative GAD67-positive cells for Figure 6a. (c and d) Photomicrographs showing GABAAR immunostaining in the left (lesioned) side and right (intact) side of MVN 3 d after unilateral labyrinthectomy with continuous drug infusion in the fourth ventricle (artificial CSF, siBDNF, or 7, 8-DHF). CSF, cerebrospinal fluid; 7,8-DHF, a TrkB Agonist. *** p < 0.001 vs. counterparts in Sham; ^^ p < 0.01 or ^^^ p < 0.001 vs. left side; # p < 0.01 or ### p < 0.001 vs. counterparts in CSF
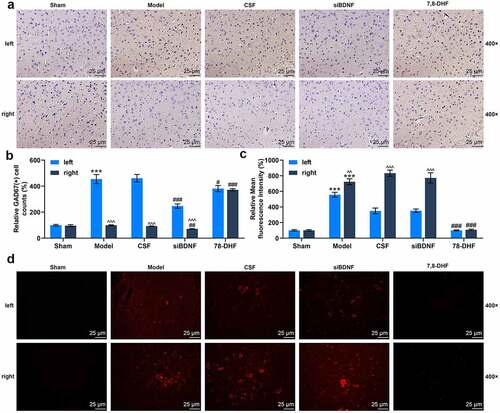
BDNF/TrkB signaling pathway influenced GABAAR expression in the left and right sides of MVN
The results manifested that GABAAR expression was pronouncedly up-regulated in both lesioned and intact sides of MVN in rats of the Model group as compared to rats in the Sham group (, p < 0.001). GABAAR expression in the left side of MVN in rats of the Model and CSF groups was considerably up-regulated compared to that in the left side of MVN in rats of the Sham group (, p < 0.01), indicating a notable asymmetry between the two sides of the MVN complex. Besides, in the siBDNF group, GABAAR expression in the right side was signally higher than that in the left side (, p < 0.01). However, GABAAR expression level was almost the same in both sides of MVN in 7,8-DHF group (). The data denoted that 7, 8-DHF infusion effectively restored the balance between GABAAR expressions in the lesioned side and intact side of MVN ().
Discussion
Vestibular compensation, which is the behavioral recovery from lesions to the peripheral vestibular system, is attributed to plasticity of the central vestibular system. After binding to the TrkB, BDNF can acutely regulate synaptic transmission and plasticity in the central nervous system, thereby participating in the vestibular compensation process [Citation7]. In the present study, we demonstrated that BDNF/TrkB signaling enhances vestibular neurogenesis, thereby accelerating functional recovery following vestibular injury. This novel plasticity mechanism could partly explain vestibular compensation process. Our findings also shed a new insight into the development of therapeutic options to attenuate the vestibular symptoms and promote recovery of vestibular function.
It has been suggested that BDNF signaling plays a key role in vestibular compensation in guinea pigs after UL [Citation15,Citation16]. Dutheil et al. [Citation1] proved that BDNF signaling is required for behavioral recovery after UVN. BDNF can modulate the synaptic transmission and plasticity of inhibitory synapses by binding to TrkB [Citation17]. To confirm the effect of BDNF/TrkB signaling on vestibular recovery, siBDNF or 7, 8-DHF was infused into rats after UL. In this study, siBDNF infusion starkly delayed the restoration of rat behaviors following UL, while 7, 8-DHF infusion markedly accelerated such restoration, which was consistent with the findings in another report that BDNF infusion decreased spontaneous nystagmus in guinea pigs after vestibular damage [Citation15]. Thus, local activation of BDNF/TrkB signaling seems to expedite the time course of vestibular compensation. However, the molecular mechanisms underlying this process remain to be fully elucidated.
Here, we discovered that continuous 7,8-DHF administration into the ventricle after UL pronouncedly enhances the production of newly generated cells in VN while siBDNF produces opposite results, confirming the proneurogenic properties of BDNF in vivo. Dutheil et al. [Citation1] proposed that continuous BDNF administration into the fourth ventricle following UVN facilitates the production of newly generated cells [Citation1]. Similarly, BDNF infusion into the lateral ventricle of the adult rat leads to new neurons in the striatum, septum, thalamus, and hypothalamus [Citation18]. Astrocytes or GABAergic neurons are differentiated from newly generated cells, which provide commissural inhibition to contralesional VN neurons [Citation19,Citation20]. Abnormal plasticity mechanisms are triggered in the local microenvironment of VN: newborn cells, such as GABAergic neurons, microglia, or astrocytes, survive and acquire different phenotypes, whereas the animal eventually recovered completely from the deficits [Citation1]. Astrocytes can protect against and repair brain injury by making changes in their morphology and proliferation [Citation21]. BDNF enhances neuronal survival and differentiation in the central nervous system during disease development and promotes synaptic modulation in adults [Citation22]. BDNF and its receptor TrkB have been proved to be crucial to many forms of neuronal plasticity [Citation23]. TrkB, activated by its ligand BDNF, has been regarded as a potent modulator of plasticity in both development and adulthood from neurite pruning and branching events during the development of peripheral nervous system (PNS) and CNS [Citation24]. Our observations confirmed that siBDNF infusion dramatically inhibited the production of astrocyte reaction, while 7, 8-DHF did the opposite. Our data signified that the activation of BDNF/TrkB signaling promoted the formation of neuronal plasticity after UL, thereby accelerating the time course of vestibular compensation.
GABAergic neurons are the main inhibitory neurons in the CNS, and play critical roles in a variety of pathophysiological processes [Citation25]. Depolarized GABAergic signaling in subicular microcircuit mediates secondary generalized seizure (sGS) in temporal lobe epilepsy [Citation26]. A previous study indicated that GABAergic signaling alters post-UVN-associated plasticity mechanisms occurring in the VN and can directly impact the time course of vestibular compensation [Citation19]. Consistent with previous study [Citation1], we confirmed that UL exclusively promoted proliferation of GABAergic neurons on the lesioned side, which was likely conducive to rebalancing the ratio of excitation to inhibition between the bilateral MVN. Surprisingly, we observed that imbalance between the bilateral MVN was still sustained 7 d after treatment with siBDNF, whereas 7, 8-DHF led to a symmetric decrease of GABAergic neurons in the bilateral MVN. We also verified that the expression of GABAAR was up-regulated in the bilateral MVN of rats infused with siBDNF 3 d after UL, while 7, 8-DHF infusion promoted the rebalance. Similarly, Julia et al. [Citation27] put forward that cortistatin-expressing interneurons require TrkB signaling to suppress neural hyper-excitability. Gu et al. [Citation28] suggested that supplying BDNF to the injured epileptogenic brain may reverse the structural and functional abnormalities in the fast-spiking GABAergic interneurons and provide an antiepileptogenic therapy. BDNF overexpression enhances the preconditioning effect of brief episodes of hypoxia, promoting survival of GABAergic neurons [Citation29]. Additionally, down-regulation of BDNF brings about GABAergic neuroplasticity dysfunction and late-life anxiety disorder [Citation30]. Brady et al. [Citation31] also corroborated that a depolarizing inhibitory GABAAR activity modulates GABAergic synapse plasticity through ERK and BDNF signaling [Citation31]. These data imply that BDNF/ TrkB signaling and GABA both facilitate a return to the homeostasis in the bilateral MVN. Our results also highlight a short therapeutic critical time window between 3 and 7 d after UL when some markers of excitability are locally changed and during which the effects of pharmacological treatments are likely to be more efficient.
In recent years, more and more advanced technologies have been applied in biomedical research and clinical practice, among which RNA sequencing (RNA-seq) experiments have brought new ideas and directions for the research of various diseases. Luigi Donato et al. [Citation32,Citation33] explored the potential role of lncRNAs and genes in the pathogenesis of retinitis pigmentosa using RNA-seq technology and found that some lncRNAs and genes might be ideal candidates for novel molecular markers and therapeutic strategies. Xu et al. [Citation34] also reported that lncRNA XIST promotes proliferation, migration, and invasion of retinoblastoma cells by regulating microRNA-191-5p and BDNF. Glyoxalase-1 (GLO-1) is known as a vital enzyme in removing AGE precursors, and its role in neurotrophic signals may be related to BDNF [Citation35]. GLO-1 was also found to be associated with retinitis pigmentosa lesions [Citation36]. The Ras-like protein RalBP1 is known to regulate cell viability [Citation37], and is found to be involved in pathological processes based on inflammation-related neurodegeneration, such as retinitis pigmentosa [Citation38]. In line with the relationship between BDNF/TrkB and retinal degeneration [Citation10], we suspected that BDNF/TrkB signaling may also have a certain interaction with GLO-1 and RalBP1. In addition, cerebral cavernous malformation (CCM), a cluster of capillaries in the brain, is associated with brain damage [Citation39–41], whereas the roles of BDNF/TrkB signaling and CCM-related genes are still unclear. Also, whether BDNF/TrkB signaling is related to vestibular cavernous hemangioma needs further exploration. Therefore, RNA sequencing (RNA-seq) experiments need to be applied in future research to more comprehensively study the gene changes in vestibular compensation and CCM, as well as the changes in the levels of biomarkers related to BDNF/TrkB signaling pathway. Also, the additional potential mechanisms beyond the activation of TrkB receptor in the recovery process are awaited to be expounded.
However, there is also room for improvement in our research. This study only conducted on male animals, which may lead to sex bias and limit the generalizability of the data in females.
Conclusion
The data indicated that BDNF-TrkB signaling is instrumental in vestibular compensation, and its activation leads to the acceleration of vestibular compensation by promoting the recovery from vestibular deficits, increasing the numbers of newly generated cells, astrocytes and GABAergic neurons, and enhancing GABAAR expression. This novel plasticity mechanism could partly explain how the system returns to homeostasis between the lesioned and intact VN, which was considered to be an important process of vestibular compensation. Our findings also shed novel light into the development of therapeutic options to attenuate the vestibular symptoms and promote recovery of vestibular function.
Authors’ contributions
Substantial contributions to conception and design: Dehong Mao, Zhongmei He
Data acquisition, data analysis and interpretation: Wei Xuan, Jiao Deng, Weichun Li, Xiaoying Fang, Linglong Li, Feng Zhang
Drafting the article or critically revising it for important intellectual content: Dehong Mao, Zhongmei He
Final approval of the version to be published: Dehong Mao, Zhongmei He, Wei Xuan, Jiao Deng, Weichun Li, Xiaoying Fang, Linglong Li, Feng Zhang
Agreement to be accountable for all aspects of the work in ensuring that questions related to the accuracy or integrity of the work are appropriately investigated and resolved: Dehong Mao, Zhongmei He, Wei Xuan, Jiao Deng, Weichun Li, Xiaoying Fang, Linglong Li, Feng Zhang
Supplemental Material
Download Zip (282.5 KB)Availability of data and materials
The analyzed data sets generated during the study are available from the corresponding author on reasonable request
Supplementary material
Supplemental data for this article can be accessed here
Disclosure statement
No potential conflict of interest was reported by the author(s).
Additional information
Funding
References
- Dutheil S, Watabe I, Sadlaoud K, et al. BDNF signaling promotes vestibular compensation by increasing neurogenesis and remodeling the expression of potassium-chloride cotransporter KCC2 and GABAA Receptor in the vestibular nuclei. J Neurosci. 2016;36(23):6199–6212.
- Mucha A, Fedor S, DeMarco D. Vestibular dysfunction and concussion. Handb Clin Neurol. 2018;158:135–144.
- Choi JY, Kim JH, Kim HJ, et al. Central paroxysmal positional nystagmus: characteristics and possible mechanisms. Neurology. 2015;84(22):2238–2246.
- Zwergal A, Gunther L, Brendel M, et al. In vivo imaging of glial activation after unilateral labyrinthectomy in the rat: a [(18)F]GE180-PET study. Front Neurol. 2017;8:665.
- Kattah JC, Guede C, Hassanzadeh B. The medial vestibular nuclei, a vulnerable target in thiamine deficiency. J Neurol. 2018;265(1):213–215.
- Smith PF. Age-related neurochemical changes in the vestibular nuclei. Front Neurol. 2016;7:20.
- Zhou L, Zhou W, Zhang S, et al. BDNF signaling in the rat cerebello-vestibular pathway during vestibular compensation: BDNF signaling in vestibular compensation. FEBS J. 2015;282(18):3579–3591.
- Guo W, Nagappan G, Lu B. Differential effects of transient and sustained activation of BDNF-TrkB signaling. Dev Neurobiol. 2018;78(7):647–659.
- Wei L, Kan LY, Zeng HY, et al. BDNF/TrkB pathway mediates the antidepressant-like role of H(2)S in CUMS-exposed rats by inhibition of hippocampal ER Stress. Neuromolecular Med. 2018;20(2):252–261.
- Kimura A, Namekata K, Guo X, et al. Neuroprotection, growth factors and BDNF-TrkB signalling in retinal degeneration. Int J Mol Sci. 2016;17(9):1584.
- Chang MY, Park S, Choi JJ, et al. MicroRNAs 218a-5p, 219a-5p, and 221-3p regulate vestibular compensation. Sci Rep. 2017;7(1):8701.
- Bergquist F, Ludwig M, Dutia MB. Role of the commissural inhibitory system in vestibular compensation in the rat. J Physiol. 2008;586(18):4441–4452.
- Livak KJ, Schmittgen TD. Analysis of relative gene expression data using real-time quantitative PCR and the 2(-Delta Delta C(T)) method. Methods. 2001;25(4):402–408.
- Paxinos G, Watson CR, Emson PC. AChE-stained horizontal sections of the rat brain in stereotaxic coordinates. J Neurosci Methods. 1980;3(2):129–149.
- Maingay MG, Sansom AJ, Kerr DR, et al. The effects of intra-vestibular nucleus administration of brain-derived neurotrophic factor (BDNF) on recovery from peripheral vestibular damage in Guinea pig. Neuroreport. 2000;11(11):2429–2432.
- Bolger C, Sansom AJ, Smith PF, et al. An antisense oligonucleotide to brain-derived neurotrophic factor delays postural compensation following unilateral labyrinthectomy in Guinea pig. Neuroreport. 1999;10(7):1485–1488.
- Drake-Baumann R. Activity-dependent modulation of inhibition in purkinje cells by TrkB ligands. Cerebellum. 2006;5(3):220–226.
- Pencea V, Bingaman KD, Wiegand SJ, et al. Infusion of brain-derived neurotrophic factor into the lateral ventricle of the adult rat leads to new neurons in the parenchyma of the striatum, septum, thalamus, and hypothalamus. J Neurosci. 2001;21(17):6706–6717.
- Dutheil S, Escoffier G, Gharbi A, et al. GABAA Receptor agonist and antagonist alter vestibular compensation and different steps of reactive neurogenesis in deafferented vestibular nuclei of adult cats. J Neurosci. 2013;33(39):15555–15566.
- Dutheil S, Brezun JM, Leonard J, et al. Neurogenesis and astrogenesis contribution to recovery of vestibular functions in the adult cat following unilateral vestibular neurectomy: cellular and behavioral evidence. Neuroscience. 2009;164(4):1444–1456.
- De Senna PN, Bagatini PB, Galland F, et al. Physical exercise reverses spatial memory deficit and induces hippocampal astrocyte plasticity in diabetic rats. Brain Res. 2017;1655:242–251.
- Guo W, Nagappan G, Lu B. Differential effects of transient and sustained activation of BDNF-TrkB signaling. Dev Neurobiol. 2018;78(7):647–659.
- Harward SC, Hedrick NG, Hall CE, et al. Autocrine BDNF-TrkB signalling within a single dendritic spine. Nature. 2016;538(7623):99–103.
- Johnstone A, Mobley W. Local TrkB signaling: themes in development and neural plasticity. Cell Tissue Res. 2020;382(1):101–111.
- Xu MY, Wong AHC. GABAergic inhibitory neurons as therapeutic targets for cognitive impairment in schizophrenia. Acta Pharmacol Sin. 2018;39(5):733–753.
- Wang Y, Xu C, Xu Z, et al. Depolarized GABAergic signaling in subicular microcircuits mediates generalized seizure in temporal lobe epilepsy. Neuron. 2017;95(92–105.e5). DOI:10.1016/j.neuron.2017.08.013.
- Hill JL, Jimenez DV, Mai Y, et al. Cortistatin-expressing interneurons require TrkB signaling to suppress neural hyper-excitability. Brain Struct Funct. 2019;224(1):471–483.
- Gu F, Parada I, Shen F, et al. Structural alterations in fast-spiking GABAergic interneurons in a model of posttraumatic neocortical epileptogenesis. Neurobiol Dis. 2017;108:100–114.
- Turovskaya MV, Gaidin SG, Vedunova MV, et al. BDNF overexpression enhances the preconditioning effect of brief episodes of hypoxia, promoting survival of GABAergic neurons. Neurosci Bull. 2020;36(7):733–760.
- Zhu G, Sun X, Yang Y, et al. Reduction of BDNF results in GABAergic neuroplasticity dysfunction and contributes to late-life anxiety disorder. Behav Neurosci. 2019;133(2):212–224.
- Brady ML, Pilli J, Lorenz-Guertin JM, et al. Depolarizing, inhibitory GABA type A receptor activity regulates GABAergic synapse plasticity via ERK and BDNF signaling. Neuropharmacology. 2018;128:324–339.
- Donato L, Scimone C, Alibrandi S, et al. Transcriptome analyses of lncRNAs in A2E-stressed retinal epithelial cells unveil advanced links between metabolic impairments related to oxidative stress and retinitis pigmentosa. Antioxidants (Basel). 2020;9(4):318.
- Donato L, D’Angelo R, Alibrandi S, et al. Effects of A2E-induced oxidative stress on retinal epithelial cells: new insights on differential gene response and retinal dystrophies. Antioxidants (Basel). 2020;9(4).
- Xu Y, Fu Z, Gao X, et al. Long non-coding RNA XIST promotes retinoblastoma cell proliferation, migration, and invasion by modulating microRNA-191-5p/brain derived neurotrophic factor. Bioengineered. 2021;12(1):1587–1598.
- Tseng YT, Tsai YH, Fülöp F, et al. 2-iodo-4ʹ-methoxychalcone attenuates methylglyoxal-induced neurotoxicity by activation of GLP-1 receptor and enhancement of neurotrophic signal, antioxidant defense and glyoxalase pathway. Molecules. 2019;24(12):2249.
- Donato L, Scimone C, Alibrandi S, et al. Discovery of GLO1 new related genes and pathways by RNA-seq on A2E-stressed retinal epithelial cells could improve knowledge on retinitis pigmentosa. Antioxidants (Basel). 2020;9(5):416.
- Ieong C, Ma J, Lai W. RALBP1 regulates oral cancer cells via Akt and is a novel target of miR-148a-3p and miR-148b-3p. J Oral Pathol Med. 2019;48(10):919–928.
- Scimone C, Donato L, Esposito T, et al. A novel RLBP1 gene geographical area-related mutation present in a young patient with retinitis punctata albescens. Hum Genomics. 2017;11(1):18.
- Scimone C, Donato L, Katsarou Z, et al. Two novel KRIT1 and CCM2 mutations in patients affected by cerebral cavernous malformations: new information on CCM2 penetrance. Front Neurol. 2018;9:953.
- Rinaldi C, Bramanti P, Scimone C, et al. Relevance of CCM gene polymorphisms for clinical management of sporadic cerebral cavernous malformations. J Neurol Sci. 2017;380:31–37.
- Adachi K, Yoshida K, Akiyama T, et al. Cavernous angioma of the vestibular nerve: case report and literature review. Surg Neurol. 2008;70(1):82–86. discussion 6.