ABSTRACT
We investigated the effect of the long noncoding RNA (lncRNA) maternally expressed gene 3 (MEG3) on hepatocellular carcinoma (HCC) tumorigenesis and progression by targeting miR-5195-3p and transcription factor forkhead box O1 (FOXO1) to identify a novel target for HCC treatment. HCC clinical samples were collected, and cell counting kit-8 (CCK-8), and transwell migration and invasion assays were performed. Furthermore, interaction was detected via double luciferase reporter and RNA pull-down assays. MEG3, miR-5195-3p, and FOXO1 expression was determined by quantitative real-time polymerase chain reaction (RT-qPCR) and Western blotting. Xenograft tumor models were established to investigate the effect of MEG3 in vivo. Compared with normal tissues, MEG3 expression was significantly downregulated in HCC tissues. MEG3 overexpression inhibited the viability and migration of HCC cells. Double luciferase reporter and RNA pull-down assays confirmed the binding between MEG3 and miR-5195-3p as well as between miR-5195-3p and FOXO1. RT-qPCR and Western blotting results showed that MEG3 inhibited the expression of miR-5195-3p and promoted that of FOXO1. Additionally, MEG3 overexpression inhibited HCC tumorigenesis and progression in xenograft tumor models while depletion of MEG3 exerted the opposite way. Therefore, the lncRNA MEG3 inhibits HCC tumorigenesis and progression through the miR-5195-3p/FOXO1 signaling axis.
KEYWORDS:
Introduction
Hepatocellular carcinoma (HCC) affects thousands of patients worldwide every year [Citation1]. HCC is highly malignant among common tumors, and its mortality rate ranks third in the world. It has a number of molecular characteristics, including distant metastasis and local invasiveness, especially intrahepatic metastasis, which is very common after surgery [Citation2]. Although HCC therapy has made considerable progress and development in terms of surgical techniques, chemotherapy drugs and targeted drugs in recent years, HCC still has a very high incidence and mortality rate, posing a serious threat to human health [Citation3]. Therefore, the molecular mechanism of HCC, prognostic diagnostic tumor indicators, and drug targets for targeted therapy require further in-depth study [Citation4].
In recent years, long non-coding RNA (lncRNA) has attracted increasing attention and research from many aspects in the biomedical field [Citation5]. In the study of many diseases, some lncRNAs that function as diagnostic and prognostic tools have gradually emerged. Recent findings have also confirmed that lncRNAs have a variety of biological functions, including regulating chromosome recombination, controlling gene transcription, and mRNA processing [Citation6]. LncRNAs involved in HCC also have a wide range of mechanisms, including DNA inactivation, methylation, transcriptional promotion, and activation of other RNA molecules [Citation7]. Moreover, studies have shown that lncRNA can undergo various epigenetic modifications, including methylation and ubiquitination, and act together with microRNAs (miRNAs) to form a network regulatory structure [Citation8].
Maternally expressed gene 3 (MEG3), located on human chromosome 14q32.3, is involved in inhibiting the growth of various malignant tumor cells and is closely related to the occurrence, development, metastasis and other biological processes of tumors [Citation9]. Changing expression level of lncRNA MEG3 can affect the metabolic process of body cells [Citation10]. MEG3 can also reduce the abundance of murine double minute 2 (MDM2), a negative regulator of p53, thus affecting the expression of tumor suppressor p53 [Citation11]. It can selectively activate the downstream target gene of p53, growth differentiation factor-15 (GDF15), and participate in the occurrence and development of malignant tumors including HCC [Citation11,Citation12]. This study aims to further improve the mechanism of MEG3 in HCC on the basis of previous studies.
In this study, we aimed to find the function and mechanism of MEG3 in HCC. Cell viablity, migration, and invasion were combined to evaluate function of MEG3 and target gene in vitro, while tumor growth was for in vivo experiment. These findings may provide novel targets for the treatment of HCC.
Materials and methods
HCC samples
In total, 40 pairs of HCC and adjacent normal tissues were surgically removed from patients undergoing primary HCC surgery in The First Affiliated Hospital of Guangzhou University of Chinese Medicine. Written informed consent was obtained from all patients, and the study was approved by the ethics committee of The First Affiliated Hospital of Guangzhou University of Chinese and was conducted in accordance with the Declaration of Helsinki.
Cell culture
Human normal liver cell line QSG-7701 and human HCC cell lines HepG2, Hep3B, Huh7, and MHCC-97 H were purchased from the American Type Culture Collection (Manassas, VA, USA). The different cell lines were cultured in Dulbecco’s modified Eagle’s medium (Solarbio, China) supplemented with 10% fetal bovine serum (Hyclone, USA) in a 5% CO2 incubator at 37°C [Citation13].
Cell transfection
All the plasmids (vector, MEG3, mimic-NC, miR-5195-3p mimic, si-nc, si-MEG3, sh-nc, and sh-forkhead box O1 (FOXO1)) were purchased from Sangon Biotech. Co., Ltd. (Shanghai, China). The cells were transfected using lipo3000 (Biosharp, China) according to the manufacturer’s instructions [Citation13].
Cell counting kit 8 (CCK-8) assay
The transfected cells were diluted in 96-well plates at a density of 5 × 103 cells per well. CCK-8 regeant (10 μL; Solarbio) was added to each well and incubated for 1 h at 37°C in the dark [Citation14]. The absorbance was recorded at 450 nm with a microplate reader. After the detection values were obtained, the cell viability and proliferation rates were calculated.
Transwell assay
The transfected cells were diluted in 24-well plates at 2 × 105 cells per well. Cell suspension (150 μL) was added to the Transwell upper chamber (Millipore, USA). 500 μL complete mediun was added to the Transwell lower chamber [Citation15]. 24 h later, the cells were fixed in methanol for 15 min and stained with crystal violet for 30 min. Cell migration and invasion was observed under a microscope (Leica, Germany).
RNA extraction and real-time polymerase chain reaction (PCR) analysis
Total cell RNA was extracted using RNA Fast kit (Solarbio), and cDNA synthesis was performed using HiScript®II Reverse Kit (Vazyme, China). Quantitative real-time PCR (RT-qPCR) was performed using SYBR Green PCR Mix (Biosharp) on an IQ5 PCR System (Bio-Rad Laboratories, Hercules, CA, USA). The program used was as follows: 94°C for 1 min, 94°C for 20 s, 58°C for 30 s, 72°C for 30 s, with 40 cycles. The primers used were as follows: MEG3, sense: 5ʹ-GTCAATACGGATCATATCCT-3ʹ, antisense: 5ʹ-ACTGCAACTGACCATTCTAC-3ʹ; miR-5195-3p, sense: 5ʹ-AGCAGTTTATTGACATAAATCAA-3ʹ, antisense: 5ʹ-CGTGAACTCGGTCTATGATTCA-3ʹ; FOXO1, sense: 5ʹ-AACTTTCGCTTAGTGGAACGT-3ʹ, antisense: 5ʹ-ACCCTCATACCTTTGGAACAG-3ʹ; U6, sense: 5ʹ-CTCGCTTCGGCAGCACA-3ʹ, antisense: 5ʹ-ACGCTTCACGAATTTGC-3ʹ; and GAPDH, sense: 5ʹ-AGAAGGCTGGGGCTCATTTG-3ʹ, antisense: 5ʹ-AGGGGCCATCCACAGTCTTC-3ʹ. The relative expression of the target genes was calculated using 2−ΔΔCt [Citation16]. U6 and GAPDH were used as the internal control for miRNA and mRNA, respectively.
RNA pull-down assay
Biotinylated miR-5195-3p (biotin-miR-5195-3p) and nc (biotin-nc) were transfected into HCC cells. After 48 h, the transfected cells were treated with lysis buffer for 10 min and harvested. The cells were then incubated with M-280 Streptavidin magnetic beads (Invitrogen, Waltham, MA, USA) at 4°C for 3 h. Precooled lysis buffer was used to wash the cells, and RT-qPCR was performed to measure the enrichment of MEG3 and FOXO1.
Western blotting
The transfected cells were collected and resuspended in 0.5 mL radioimmunoprecipitation assay buffer (Solarbio,). Cell lysates were placed on ice for 30 min, followed by centrifugation at 10,000 g for 20 min. Protein concentration was determined using the bicinchoninic acid kit (Thermo Fisher Scientific, USA). Protein samples (20 μg) were electrophoresed via sodium dodecyl sulfate-polyacrylamide gel electrophoresis and transferred onto a polyvinylidene Fluoride membrane. The The membrane was blocked with 5% nonfat milk in Tris-buffered saline with Tween 20 at room temperature for 2 h and then probed with FOXO1 and GAPDH primary antibody (1:2000 dilution; Boster, China) at 4°C overnight. The secondary antibody used for detection was a horseradish peroxidase-conjugated anti-rabbit IgG, and the protein expression was detected using an ECL chemiluminescence detection kit (Boster).
Double luciferase reporter assay
Wild-type (wt) MEG3 or mutant (mut) sequence of FOXO1 were synthetized and coloned into a GV272 reporter vector. The wt or mutant MEG3 or FOXO1 vectors were transfected into HepG2 and Huh7 cells which have been transfected with miR-5195-3p mimic or control vectors. After transfection for 24 h, cells were collected and analyzed using a dual-luciferase reporting system.
Xenograft tumor model
Female nude mice (four to five weeks old) were purchased from Beijing Vital River Laboratory Animal Technology Co., Ltd. and randomly divided into si-nc, si-MEG3, vector and MEG3 overexpression groups (n = 3 per group). Stably transfected cells were subcutaneously injected into the mice (1 × 106 cells per mouse), and tumor size was measured weekly [Citation17]. After four weeks, the mice were anesthetized via intraperitoneal injection of 1% pentobarbital sodium (160 mg/kg) and sacrificed via asphyxiation. Liver tumor tissues were isolated and photographed, and their volume and weight were measured. Tumor volume was calculated as follows: V (mm3) = (length×width2)/2.
Statistical analysis
GraphPad Prism 5.0 was used for statistical analysis. The data were expressed as the mean ± standard deviation.The t-test was used for comparison between the two groups and one-way analysis of variance was used among multiple groups. Statistical significance was set at P < 0.05.
Results
lncRNA MEG3 is downregulated in tumor tissues and cell lines of HCC
First, bioinformatics analysis indicated that MEG3 lncRNA expression in HCC samples was significantly downregulated compared to that in normal tissues ()). To further confirm this, we detected MEG3 expression in tumor and normal tissues in patients with HCC. Interestingly, MEG3 expression was significantly downregulated in tumor tissues compared with normal tissues ()). Furthermore, we determined the expression level of MEG3 in liver cell lines, and found that it was significantly lower than that of the normal liver cell line QSG-7701 ()). Among the four liver cancer cell lines, HepG2 and Huh7 expressed the lowest MEG3 abundance. Therefore, HepG2 and Huh7 were used in subsequent experiments.
Figure 1. LncRNA MEG3 is downregulated in HCC tissues and cell lines
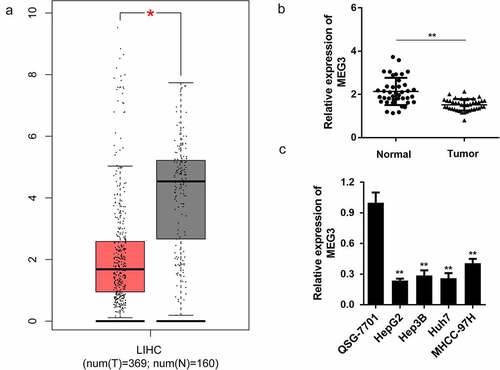
Dysregulated MEG3 affects tumor progression in HepG2 and Huh7 cells
In order to study the function of MEG3 in HCC, MEG3 of HepG2 and Huh7 cells were upregulated and downregulated separately, RT-qPCR results indicated that compared with the vector group, transfection of MEG3-overexpressing vector significantly enhanced MEG3 expression while knockdown of MEG3 exerted the opposite way ()). Cell viability was then determined via the CCK-8 assay. The results indicated that elevated MEG3 suppressed cell viability of both HepG2 and Huh7 cells while cell viability was promoted by suppressing MEG3 () and (c)). Transwell assay indicated that MEG3 overexpression inhibited HCC cell migration and invasion, which were accelerated by downexpression of MEG3 () and (e)). These data reveal that MEG3 inhibits HCC cell proliferation, migration, and invasion.
Figure 2. MEG3 affects the proliferation, migration, and invasion of HCC cell lines HepG2 and Huh7. (a) qPCR was performed to detect MEG3 expression in HepG2 and Huh7 cell lines. (b, c) CCK-8 was used to detect HCC cell proliferation after transfection. (d, e) Transwell assay was used to assess the migration and invasion of HCC cells. *P < 0.05, **P < 0.01
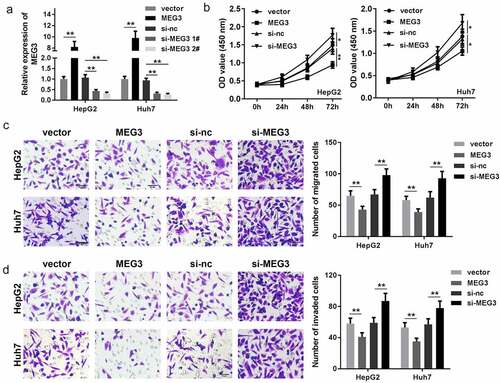
MEG3 targets miR-5195-3p
In order to further study the mechanism of MEG3 in HCC progression, we first predicted the miRNA that MEG3 binds to. Bioinformatics results showed that MEG3 could potentially bind to miR-5195-3p ()). Luciferase reporter assay further confirmed the binding relationship between MEG3 and miR-5195-3p. miR-5195-3p mimic transfection significantly inhibited the luciferase activity of the reporter carrying the wt MEG3 sequence while there was no change in luciferase activity of the mut group ()). Meanwhile, RNA pull-down assay indicated that the biotin labeled miR-5195-3p probe could enrich the expression of MEG3, which indicates their interaction ()). Moreover, the expression level of miR-5195-3p was notably increased upon MEG3 knockdown notably but was reduced upon MEG3 overexpression ()). At the same time, miR-5195-3p expression was significantly upregulated in tumor tissues compared with normal tissues in HCC ()).
Figure 3. MEG3 sponges miR-5195-3p in HCC cells. (a) The binding site between MEG3 and miR-5195-3p was predicted by bioinformatics analysis. (b) Luciferase reporter assay was used to confirm the interaction between MEG3 and miR-5195-3p. (c) RNA pull-down assay was performed to determine whether MEG3 sponges miR-5195-3p. (d) MiR-5195-3p expression level was detected in MEG3 overexpression or knockdown group using qPCR. (e) qPCR was performed to evaluate the expression of miR-5195-3p in HCC tissues. **P < 0.01
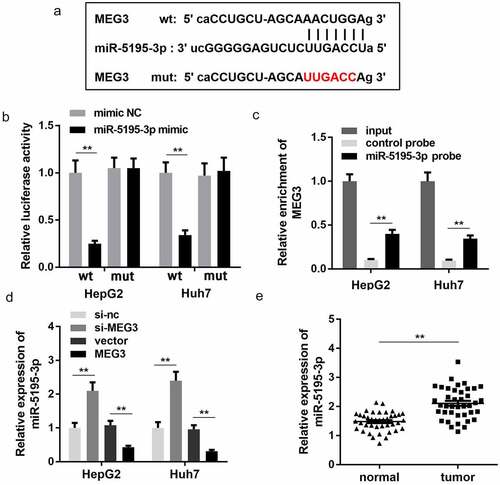
miR-5195-3p targets FOXO1 in HCC cells
In order to further study the mechanism of MEG3 in HCC, we analyzed the downstream target genes of miR-5195-3p. Bioinformatics results showed that FOXO1 could be combined with miR-5195-3p ()). Detection of luciferase reporter gene expression and RNA pull-down assay confirmed the binding between FOXO1 and miR-5195-3p () and (c)) in HepG2 and Huh7 cells. RT-qPCR and Western blotting confirmed that the effect of the miR-5195-3p mimic was consistent with that of the miR-5195-3p inhibitor and could significantly inhibit the expression of FOXO1 ()). Moreover, compared with normal tissues, FOXO1 was significantly downregulated in HCC tissues ()). Based on these results, we further speculated that MEG3 might inhibit HCC development by regulating miR-5195-3p and FOXO1 expression.
Figure 4. FOXO1 is a target gene of miR-5195-3p. (a) The binding site between miR-5195-3p and FOXO1 was predicted by bioinformatics analysis. (b) Luciferase reporter assay was used to confirm the interaction between FOXO1 and miR-5195-3p. (c) RNA pull-down assay was performed to determine whether miR-5195-3p targets FOXO1. (d, e) FOXO1 expression level was detected in miR-5195-3p overexpression or knockdown group using qPCR and Western blotting. (f) qPCR was performed to evaluate the expression of FOXO1 in HCC tissues. **P < 0.01
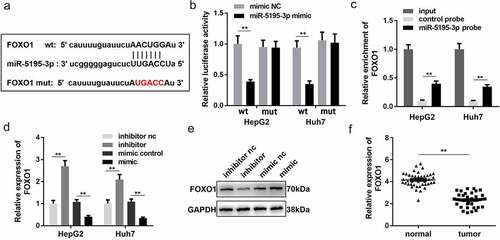
MiR-5195-3p and FOXO1 knockdown both reverse the effect of MEG3 on HCC cell proliferation, migration, and invasion
Rescue experiments were performed to further confirm the interaction of MEG3 with miR-5195-3p and FOXO1. qPCR results indicated that MEG3 overexpression promoted the expression of FOXO1, while miR-5195-3p overexpression and FOXO1 knockdown significantly inhibited FOXO1 expression ()). Subsequently, functional studies, such as CCK-8 and transwell assay, were carried out and indicated that miR-5195-3p or FOXO1 knockdown reversed the effect of MEG3 on the proliferation, migration, and invasion of HCC cells ()).
Figure 5. Co-expression of MEG3 and FOXO1 inhibits HCC progression in liver cancer cell lines. (a) qPCR was performed to detect FOXO1 expression in HepG2 and Huh7 cell lines. (b) CCK-8 was used to detect HCC cell proliferation after transfection. (c, d) Transwell assay was used to assess HCC cell migration and invasion. **P < 0.01
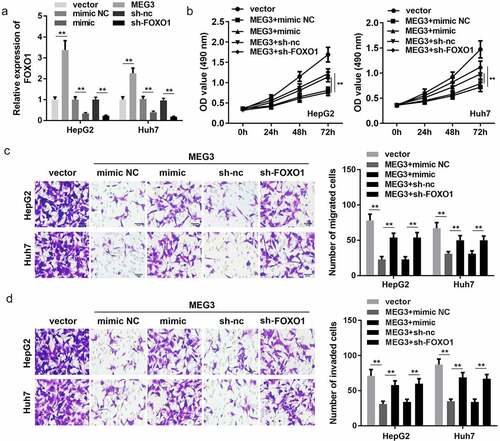
MEG3 affects HCC progression in vivo
To further confirm the function of MEG3, an in vivo experiment was performed. We successfully established the mouse allograft model, and the results showed that the tumor weight and volume of the MEG3 overexpression group decreased significantly when compared with those of the vector control group ()). Meanwhile, in the MEG3 overexpression group, expression of miR-5195-3p was significantly inhibited, while that of MEG3 and FOXO1 was significantly enhanced, leading to an increased HCC inhibition ()). Depletion of MEG3 exerted the opposite way on tumor weight and volume as well as MEG3 and FOXO1 expression ()). The xenograft tumor model demonstrates that overexpression of MEG3 inhibits HCC progression and knockdown of MEG3 suppresses aggressiveness of HCC in vivo.
Figure 6. Xenograft tumor model demonstrates that overexpression of MEG3 inhibites the HCC progression. (a) The images of tumor in two groups. (b) The growth curve was established. (c) Tumors weight in each group was detected. (d-f) MEG3, miR-5195-3p and FOXO1 expression level was detected in the tumor tissues using qPCR. **P < 0.01, ***P < 0.001
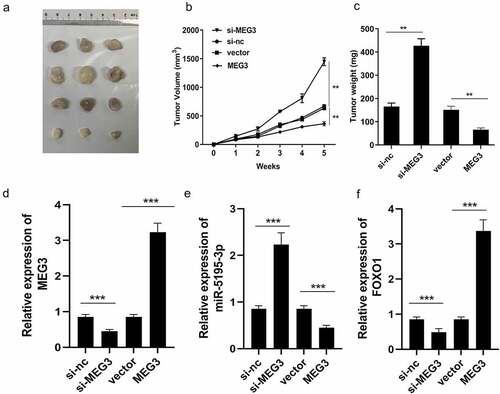
Discussion
In this study, we found that MEG3 expression was significantly downregulated in HCC tissues, and this was closely related to tumor development, invasion, and metastasis. MEG3 overexpression suppressed aggressiveness of HCC in vitro and in vivo while inhibition of MEG3 exerted the opposite function. Furthermore, MEG3 could bind with miR-5195-3p to regulate FOXO1 expression, thereby affect cellular functions of HCC cells.
Accumulating evidence suggested that aberrant expressed lncRNAs may participate in process of HCC development [Citation18]. Since 2009, scholars have found that MEG3 may be related to the occurrence and development of HCC [Citation12,Citation19]. In the past decade, MEG3 has been proved to have inhibitory effects on HCC in vivo and in vitro [Citation12,Citation19,Citation20]. Our data also verified that HCC was suppressed by overexpressed MEG3, and aggravated by inhibition of MEG3 in vitro and in vivo.
In recent years, increasing studies have shown that dysregulation of miRNAs exists in a variety of solid tumors such as HCC and is closely related to the diagnosis, stage, progression, prognosis, and treatment response of tumors, suggesting that miRNAs may be involved in tumor occurrence and development [Citation21]. In addition, with the in-depth research on the mechanism of competitive endogenous RNAs (ceRNA) [Citation22], more and more miRNAs have been found to be able to be regulated by MEG3 to affect the process of HCC [Citation23–25]. Therefore, it is crucial to improve the miRNA network bined with MEG3 and confirm its regulation of HCC. Multiple studies have reported that the expression of miR-5195-3p was downregulated in cervical, triple-negative breast, and colon cancer, which reduces the chemotherapy sensitivity of tumor cells to paclitaxel and inhibits the TGF-β signaling pathway and cell cycle progression, leading to malignant tumor progression [Citation26]. However, the relationship between miR-5195-3p and HCC has not been studied and remains unclear. To elucidate the mechanism underlying MEG3 function, we determined the miRNA sponged by MEG3. Bioinformatics and experimental analysis confirmed that MEG3 can target miR-5195-3p in HCC cells. Furthermore, our data demonstrated that suppression of cell viability, migration, and invasion induced by overexpression of MEG3 were dramatically reversed by miR-5195-3p mimic vectors; demonstrating MEG3 inhibits aggressiveness of HCC by regulating miR-5195-3p.
miRNAs target and inhibit the expression of their target genes [Citation27]. Identifying and validating the target genes of miRNAs are critical for elucidating the specific mechanisms of miRNAs in certain diseases [Citation28]. FOXO1 was identified to be a direct target of miR-5195-3p in HCC cells. FOXO1 belongs to a conserved family of transcription factors, the FOX family [Citation29–31]. FOXO1 transcription factors are important intracellular transcription factors whose activity is involved in cell proliferation, differentiation, stress response, and metabolism [Citation32–34]. In tumor studies, abnormal expression of FOXO1 has been associated with tumorigenesis, tumor-induced angiogenesis and metastasis, tumor cell tolerance to stress, and tumor cell metabolic homeostasis [Citation35,Citation36]. Dysregulated FOXO1 has been confirmed to function as tumor suppressor in HCC [Citation37,Citation38]. In this study, we found that MEG3 took effect through sponging miR-5195-3p to suppress the FOXO1 expression. Our study demonstrated that MEG3 regulated FOXO1 through miR-5195-3p in HCC.
Conclusion
In summary, the expression level of MEG3 is significantly downregulated in HCC cells and tissues, and this reduced expression is closely related to HCC progression. MEG3 inhibits cell proliferation and metastasis by regulating the expression of miR-5195-3p and FOXO1 in HCC cells, and inhibits tumor growth in vivo. Therefore, MEG3 may be a potential clinical drug target for HCC treatment. However, the more precise regulation mechanism in the process of HCC metastasis and recurrence needs to be further studied.
Highlights
lncRNA MEG3 is dowregulated in HCC tissues and cells.
Overexpression of MEG3 suppresses tumor growth in vitro and in vivo.
Upregulation of MEG3 contributes to inhibit aggressiveness of HCC by modulating the miR-5195-3p/FOXO1 axis
Disclosure statement
No potential conflict of interest was reported by the author(s).
Additional information
Funding
References
- Zucman-Rossi J, Jeannot E, Nhieu JT, et al. Genotype-phenotype correlation in hepatocellular adenoma: new classification and relationship with HCC. Hepatology [Journal Article; Multicenter Study; Research Support, Non-U.S. Gov’t]. 2006 March 01;43(3):515–524.
- Burrel M, Llovet JM, Ayuso C, et al. MRI angiography is superior to helical CT for detection of HCC prior to liver transplantation: an explant correlation. Hepatology [Journal Article; Research Support, Non-U.S. Gov’t]. 2003 Oct 01;38(4):1034–1042.
- Han ZG. Functional genomic studies: insights into the pathogenesis of liver cancer. Annu Rev Genomics Hum Genet [Journal Article; Research Support, Non-U.S. Gov’t; Review]. 2012 Jan 20;13:171–205.
- Park JM, Kim TH, Bae JS, et al. Role of resveratrol in FOXO1-mediated gluconeogenic gene expression in the liver. Biochem Biophys Res Commun [Journal Article; Research Support, Non-U.S. Gov’t]. 2010 Dec 17;403(3–4):329–334.
- Braconi C, Kogure T, Valeri N, et al. microRNA-29 can regulate expression of the long non-coding RNA gene MEG3 in hepatocellular cancer. Oncogene. 2011 Jan 01;30(47):4750–4756.
- Fornari F, Milazzo M, Chieco P, et al. MiR-199a-3p regulates mTOR and c-Met to influence the doxorubicin sensitivity of human hepatocarcinoma cells. Cancer Res [Journal Article]. 2010 June 15;70(12):5184–5193.
- Xiong X, Tao R, DePinho RA, et al. Deletion of hepatic FoxO1/3/4 genes in mice significantly impacts on glucose metabolism through downregulation of gluconeogenesis and upregulation of glycolysis. Plos One [Journal Article; Research Support, N.I.H., Extramural]. 2013 Jan 20;8(8):e74340.
- Guo X, Gao L, Wang Y, et al. Advances in long noncoding RNAs: identification, structure prediction and function annotation. Brief Funct Genomics [Journal Article; Research Support, Non-U.S. Gov’t; Review]. 2016 Jan 01;15(1):38–46.
- Hsieh PF, Yu CC, Chu PM, et al. Long non-coding RNA MEG3 in cellular stemness. Int J Mol Sci [Journal Article; Review]. 2021 May 19;22:10.
- Al-Rugeebah A, Alanazi M, Parine NR. MEG3: an oncogenic long non-coding RNA in different cancers. Pathol Oncol Res [Journal Article; Review]. 2019 July 01;25(3):859–874.
- Wei GH, Wang X. lncRNA MEG3 inhibit proliferation and metastasis of gastric cancer via p53 signaling pathway. Eur Rev Med Pharmacol Sci [Journal Article]. 2017 Oct 01;21(17):3850–3856.
- Zhang Y, Liu J, Lv Y, et al. LncRNA meg3 suppresses hepatocellular carcinoma in vitro and vivo studies. Am J Transl Res [Journal Article]. 2019 Jan 20;11(7):4089–4099.
- Zhang X, Chen J, Jiang S, et al. N-Acetyltransferase 10 enhances doxorubicin resistance in human hepatocellular carcinoma cell lines by promoting the epithelial-to-mesenchymal transition. Oxid Med Cell Longev [Journal Article]. 2019 Jan 20;2019:7561879.
- Zhou Y, Ren H, Dai B, et al. Hepatocellular carcinoma-derived exosomal miRNA-21 contributes to tumor progression by converting hepatocyte stellate cells to cancer-associated fibroblasts. J Exp Clin Cancer Res [Journal Article]. 2018 Dec 27;37(1):324.
- Wang J, He H, Jiang Q, et al. CBX6 promotes HCC metastasis via transcription factors Snail/Zeb1-mediated EMT mechanism. Onco Targets Ther [Journal Article]. 2020 Jan 20;13:12489–12500.
- Zhang Q, Cheng S, Cao L, et al. LINC00978 promotes hepatocellular carcinoma carcinogenesis partly via activating the MAPK/ERK pathway. Biosci Rep [Journal Article]. 2020 March 27;40:3.
- Guo D, Zhang D, Ren M, et al. THBS4 promotes HCC progression by regulating ITGB1 via FAK/PI3K/AKT pathway. FASEB J [Journal Article; Research Support, Non-U.S. Gov’t]. 2020 Aug 01;34(8):10668–10681.
- Huang Z, Zhou JK, Peng Y, et al. The role of long noncoding RNAs in hepatocellular carcinoma. Mol Cancer [Journal Article; Research Support, Non-U.S. Gov’t; Review]. 2020 April 15;19(1):77.
- Shi X, Sun M, Liu H, et al. Long non-coding RNAs: a new frontier in the study of human diseases. Cancer Lett [Journal Article; Research Support, Non-U.S. Gov’t; Review]. 2013 Oct 10;339(2):159–166.
- Zhuo H, Tang J, Lin Z, et al. The aberrant expression of MEG3 regulated by UHRF1 predicts the prognosis of hepatocellular carcinoma. Mol Carcinog [Journal Article; Research Support, Non-U.S. Gov’t]. 2016 Feb 01;55(2):209–219.
- Xue R, Dong L, Zhang S, et al. Investigation of volatile biomarkers in liver cancer blood using solid-phase microextraction and gas chromatography/mass spectrometry. Rapid Commun Mass SP. 2008 Jan 01;22(8):1181–1186.
- Qi X, Zhang DH, Wu N, et al. ceRNA in cancer: possible functions and clinical implications. J Med Genet [Journal Article; Review]. 2015 Oct 01;52(10):710–718.
- Liu Z, Chen JY, Zhong Y, et al. lncRNA MEG3 inhibits the growth of hepatocellular carcinoma cells by sponging miR-9-5p to upregulate SOX11. Braz J Med Biol Res [Journal Article]. 2019 Jan 20;52(10):e8631.
- Li X, Cheng T, He Y, et al. High glucose regulates ERp29 in hepatocellular carcinoma by LncRNA MEG3-miRNA 483-3p pathway. Life Sci [Journal Article]. 2019 Sep 01;232:116602.
- He JH, Han ZP, Liu JM, et al. Overexpression of long non-coding RNA MEG3 inhibits proliferation of hepatocellular carcinoma Huh7 cells via negative modulation of miRNA-664. J Cell Biochem [Journal Article; Research Support, Non-U.S. Gov’t]. 2017 Nov 01;118(11):3713–3721.
- Song Z, Guo Q, Wang H, et al. miR-5193, regulated by FUT1, suppresses proliferation and migration of ovarian cancer cells by targeting TRIM11. Pathol Res Pract [Journal Article]. 2020 Nov 01;216(11):153148.
- Yang J, Li T, Gao C, et al. FOXO1 3ʹUTR functions as a ceRNA in repressing the metastases of breast cancer cells via regulating miRNA activity. Febs Lett [Journal Article; Research Support, Non-U.S. Gov’t]. 2014 Aug 25;588(17):3218–3224.
- Yu W, Qiao Y, Tang X, et al. Tumor suppressor long non-coding RNA, MT1DP is negatively regulated by YAP and Runx2 to inhibit FoxA1 in liver cancer cells. Cell Signal [Journal Article; Research Support, Non-U.S. Gov’t]. 2014 Dec 01;26(12):2961–2968.
- Zhao Y, Yang J, Liao W, et al. Cytosolic FoxO1 is essential for the induction of autophagy and tumour suppressor activity. Nat Cell Biol [Journal Article; Research Support, Non-U.S. Gov’t]. 2010 July 01;12(7):665–675.
- Wu S, Zhang G, Li P, et al. miR-198 targets SHMT1 to inhibit cell proliferation and enhance cell apoptosis in lung adenocarcinoma. Tumour Biol [Journal Article]. 2016 April 01;37(4):5193–5202.
- He XX, Chang Y, Meng FY, et al. MicroRNA-375 targets AEG-1 in hepatocellular carcinoma and suppresses liver cancer cell growth in vitro and in vivo. Oncogene [Journal Article; Research Support, Non-U.S. Gov’t]. 2012 July 12;31(28):3357–3369.
- Wang P, Ren Z, Sun P. Overexpression of the long non-coding RNA MEG3 impairs in vitro glioma cell proliferation. J Cell Biochem. 2012 Jan 01;113(6):1868–1874.
- Chen J, Huang Q, Wang F. Inhibition of FoxO1 nuclear exclusion prevents metastasis of glioblastoma. Tumour Biol [Journal Article; Retracted Publication]. 2014 July 01;35(7):7195–7200.
- Walters ZS, Villarejo-Balcells B, Olmos D, et al. JARID2 is a direct target of the PAX3-FOXO1 fusion protein and inhibits myogenic differentiation of rhabdomyosarcoma cells. Oncogene [Journal Article; Research Support, Non-U.S. Gov’t]. 2014 Feb 27;33(9):1148–1157.
- Huang J, Jiao J, Xu W, et al. MiR-155 is upregulated in patients with active tuberculosis and inhibits apoptosis of monocytes by targeting FOXO3. Mol Med Rep [Journal Article]. 2015 Nov 01;12(5):7102–7108.
- Tsuchida A, Yamauchi T, Ito Y, et al. Insulin/Foxo1 pathway regulates expression levels of adiponectin receptors and adiponectin sensitivity. J Biol Chem [Journal Article]. 2004 July 16;279(29):30817–30822.
- Jiang J, Huang Z, Chen X, et al. Trifluoperazine activates FOXO1-related signals to inhibit tumor growth in hepatocellular carcinoma. DNA Cell Biol [Journal Article]. 2017 Oct 01;36(10):813–821.
- Wang Q, Yang X, Zhou X, et al. MiR-3174 promotes proliferation and inhibits apoptosis by targeting FOXO1 in hepatocellular carcinoma. Biochem Biophys Res Commun [Journal Article; Research Support, Non-U.S. Gov’t]. 2020 June 11;526(4):889–897.