ABSTRACT
Circular RNAs (circRNAs) have been reported to be involved in the progression of papillary thyroid carcinoma (PTC). However, the role of circular RNA Pvt1 oncogene (circPVT1) in PTC has rarely been reported. In this study, we aimed to investigate the function and mechanism of circPVT1 in PTC. The expression level of circPVT1, miR-195 and VEGFA were determined by reverse transcription‑quantitative PCR (RT‑qPCR). Fisher’s exact test was used to analyze the correlation between circPVT1 expression and PTC clinical features. Cell Counting Kit-8 (CCK-8) and 5-Ethynyl-2ʹ-deoxyuridine (EdU) staining assay and transwell assay were conducted to evaluate the cell proliferation, migration and invasion ability. Dual-luciferase reporter and Western blot assay were conducted for evaluating the correlation between miR-195 and circPVT1 or VEGFA. The results of RT-PCR showed that the expression level of circPVT1 was significantly upregulated in PTC tissues and cell lines. After downregulating circPVT1 expression in PTC cells, the abilities of cell proliferation, migration, and invasion were obviously suppressed, and the Wnt/β-catenin signaling pathway was also repressed. Besides, miR-195 could both bind to PVT1 and VEGFA, while PVT1 could promote the expression of VEGFA by binding to miR-195. Downregulation of VEGFA expression in PTC cells revealed weakened cell proliferation, migration, and invasion capacities, and restrained Wnt/β-catenin signaling pathway. Therefore, we demonstrated that circPVT1 could promote VEGFA expression by sponging miR-195. CircPVT1 could serve as a molecule sponge for miR-195 and mediate the ceRNA network to promote the expression of VEGFA, thus contributed to the malignant progression of PTC.
Introduction
Thyroid cancer (TC) is one of most prevalent endocrine malignancy worldwide, and its incidence has doubled globally in the last 30 years [Citation1–3], TC is one of the reasons for the high incidence of malignant tumors in women, as it is the fifth most diagnosed malignant tumor in this group [Citation4,Citation5]. Papillary thyroid cancer (PTC) is the most common histotype of TC, accounting for approximately 85% of all TC [Citation6]. Although the prognosis of PTC is generally good, a high quality of life still cannot be guaranteed. This is because some PTC patients show early epidural infiltration, lymphatic metastasis, and even distant metastasis or other high-risk complications [Citation7]. More than 30% of PTC patients develop into lymph node metastasis [Citation8], while 2.6% to 3.7% of patients suffer from distant metastasis [Citation5,Citation9]. The occurrence of distant metastasis and invasion of PTC indicates a poor prognosis, and often the disease cannot be effectively controlled [Citation10]. Consequently, investigating the mechanism of the malignant progression of PTC has vital theoretical significance and potential clinical value.
Circular RNAs (circRNAs) were discovered approximately 40 years ago [Citation11], but have not attracted widespread attention until recently. As circRNAs are ncRNAs, they were initially thought to be byproducts of pre-mRNA splicing errors [Citation12]. Compared with other types of noncoding RNAs (ncRNAs), circRNAs have neither 5ʹ to 3ʹ polarity nor a polyA tail, because they form covalently closed loops. These circRNAs are more conserved and stable than linear ncRNAs [Citation13,Citation14]. In addition, some studies have found that there are four basic types of circRNAs [Citation15]: exon circRNAs, intronic circRNAs, exon-intron circRNAs, and intergenic circRNAs. With the rapid development of next-generation sequencing technology, many circRNAs have been identified in mammalian transcriptomes and various cancers [Citation16–18]. Because circRNA is not easily degraded by exonuclease RNase R, most of them have a long half-life and are widely present in tissues and peripheral blood, and they are highly conserved and have spatial tissue and disease specificity [Citation19,Citation20]. These characteristics endow it with a potential function, such as acting as a miRNA molecular sponge, regulating gene expression and participating in protein translation, and may also be a potential target for disease intervention [Citation21,Citation22]. In addition, circRNA in peripheral blood has stable biological properties and is expected to become a molecular marker for disease diagnosis and prognosis [Citation16,Citation23]. In human cancers, substantial researches have demonstrated that circRNAs may play an essential role in cancer growth, metastasis, and malignant progression. For example, Li et al. find that circRNA hsa_circ_0014130 can act as a miR-132-3p sponge for playing oncogenic roles in bladder cancer by upregulating potassium inwardly rectifying channel subfamily J member 12 (KCNJ12) expression [Citation24]. Zhang et al demonstrate that hsa-circ_0058106 can induce epithelial-mesenchymal transition (EMT) and metastasis in laryngeal cancer via sponging miR-153 and inducing twist family bHLH transcription factor 1 (TWIST1) nuclear translocation [Citation25]. Cai et al show that circ-NOL10 regulated by metadherin (MTDH)/CASC3 exon junction complex subunit (CASC3) can suppress breast cancer progression and metastasis via bind to multiple miRNAs and programmed cell death 4 (PDCD4) [Citation26]. These studies identify that aberrant circRNAs expression may be encouraging diagnosis biomarkers and therapeutic target for PTC.
The circRNA PVT1 (circPVT1) is a recently identified circRNA situated in the known cancer susceptibility region chr8: 128902834–128903244 [Citation27,Citation28]. It originates from the third exon of the PVT1 and has been identified as an oncogene [Citation27]. Previous studies have shown that circPVT1 may play a vital role in the ininiation and progression of several tumors, including laryngeal cancer [Citation29], non-small cell lung cancer [Citation30], gastric cancer [Citation31], clear cell renal cell carcinoma [Citation32], ovarian cancer [Citation33], cervical cancer [Citation34] and breast cancer [Citation35]. However, the expression pattern and biological function of circPVT1 in PTC remain unknown. In the current study, we hypothesized that circPVT1 might assume an essential role in the progression of PTC. The aim of this study was to reveal the biological function and potential molecular mechanism of circPVT1 regulating the progression of PTC. We firstly measured circPVT1 expression level in the PTC tissues and cell lines. Meanwhile, we detected the functions of circPVT1 on the cell growth, migration, and invasiveness of PTC. Finally, we revealed the underlying mechanism that circVRK1 sponged miR-195 and accelerated the VEGFA expression, so as to regulate the Wnt/β-catenin signaling pathway.
Materials and methods sample Collection
Fifty pairs of PTC tumor samples and matching contralateral normal samples were collected from patients with PTC in our hospital between October 2015 and March 2017. The samples were frozen in liquid nitrogen and stored at −80°C until use. We verified the cancer status of all the tissue samples by pathological examination. The Ethics Committee of the Tinglin Hospital of Jinshan District approved the study (2015–023) and informed consent was obtained from patients before surgical operation.
Cell culture
We purchased PTC cell lines (K1, IHH-4, BCPAP, and TPC-1) and human thyroid follicular epithelial cells (Nthy-ori 3–1) from the Hematology Institute (Beijing, China) of the Chinese Academy of Chinese Medical Sciences. The K1, IHH-4 and Nthy-ori 3–1 cells were cultured in Roswell Park Memorial Institute (RPMI)-1640 medium (Invitrogen, Grand Island, NY, USA), while TPC-1 and BCPAP cells were cultured in Dulbecco’s Modified Eagle Medium (DMEM). Additional MEM Non-Essential Amino Acids Solution (NEAA 100X) (Invitrogen, 11140050) was added to the BCPAP cell line. All medium was containing 10% heat-inactivated fetal bovine serum (FBS; Invitrogen), penicillin (100 U/mL)/streptomycin (100 μg/mL). The cells were incubated in a humidified incubator at 37°C and 5% CO2.
Cell transfection
We purchased the PVT1 small interfering RNA (siRNA), VEGFA siRNA, miR-195 inhibitor, and their corresponding negative controls from GenePharma (Shanghai, China). Next, we mixed the cells in a 6-well plate (5 × 105 cells per well). Following the manufacturer’s instructions, after the cell density reached 80%, the transfection reagent was mixed with Lipofectamine 2000 (Invitrogen) and added to the cells [Citation36]. The mixture was incubated at room temperature for 30 min and subsequently transferred to a Petri dish. After transfection for 48 h using Lipofectamine 2000, the transfected cells were collected to assess the transfection efficiency by qRT-PCR. The sequences of primers for transfection were listed below: circPVT1 siRNA: 5ʹ-GCAAAUGAAAGCUACCAAUTT-3ʹ; scramble siRNA: 5ʹ-UUCUCCGAACGUGUCACGUTT-3ʹ; VEGFA siRNA: 5ʹ-CUGGAAUUUGAUAUUCAUUGA-3ʹ; scramble siRNA: 5ʹ-UUCUCCGAACGUGUCAC GUTT-3ʹ; miR‑195 mimics sense, 5ʹ‑UAGCAGCA CAGAAAUAUUGGC‑3ʹ; miR‑195 mimics antisense, 5ʹ‑CAAUAUUUCUGUGCUGCUAUU‑3ʹ; mimics negative control (miR‑NC) sense, 5ʹ‑UUC UCCGAACGUGUCACGUTT‑3ʹ; miR‑NC antisense, 5ʹ‑ACGUGACACGUUCGGAGAATT‑3ʹ.
RNA extraction and reverse transcription‑quantitative PCR (RT‑qPCR)
After transfection for 24–48 h, purified total RNA was extracted from tissues and cells using TRIzol (Invitrogen). cDNA was obtained by reverse transcription in a 20 μL reaction system using the PrimeScript RT reagent Kit (TAKARA, Code No. RR037A) according to the manufacturer’s instructions. Real-time quantitative reverse transcription polymerase-chain reaction (RT-PCR) analysis was performed to determine transfection efficiency using the following conditions: 92°C for 10 min, followed by 40 cycles of 92°C for 10 s and then 60°C for 1 min [Citation37]. GAPDH and U6 were used as the internal reference, respectively. The primer sequences used were as follows: PVT1 (F: 5ʹ-GGTTCCACCAGCGTTATTC-3ʹ; R: 5ʹ-CAACTT CCTTTGGGTCTCC-3ʹ); miR-195 (F: 5ʹ-ACGA TGCCCACGACCAAGCC-3ʹ; R: 5ʹ-AGCACCATC GTCCGCAGGCA-3ʹ); VEGFA (F: 5ʹ-AACTTT CTGCTGTCTTGGGT-3ʹ; R: 5ʹ-TCTCGATTGGA TGGCAGTA-3ʹ); Wnt3a (F: 5ʹ-TCTACGACGT GCACACCTG-3ʹ; R: 5ʹ-CCCTGCCTTCAGGTA GGAGT-3ʹ); β-catenin (F: 5ʹ-CAGCAGCAATT TGTGGAGGG-3ʹ; R: 5ʹ-GCAGCTGCACAAACA ATGGA-3ʹ); GAPDH (F: 5ʹ-GGAATCCACTGG CGTCTTCA-3ʹ; R: 5ʹ-GGTTCACGCCCATCAC AAAC-3ʹ); U6 (F: 5ʹ-CTCGCTTCGGCAGCACA-3ʹ; R: 5ʹ-AACGCTTCACGAATTTGCGT-3ʹ).
Cell proliferation activity assay
We used Cell Counting Kit-8 (CCK-8, Dojindo, Kumamoto, Japan) to detect cell growth ability and the method referred to the previous study [Citation38]. Cells in the medium were digested with 0.25% trypsin and the cell suspensions were subsequently harvested and seeded into 96-well plates, with 6 wells per group. Each well contained at least 2 × 103 cells and 200 μL of the medium. The cells were incubated overnight for adherent growth and the cell supernatant was washed with phosphate-buffered saline. A mixture containing 90 μL of pure 1640 medium and 10 μL of CCK-8 solution (Beyotime Biotechnology, Shanghai, China) was added to each well. After 2 h of incubation, the absorbance of each well was measured at 450 nm using a microplate reader.
Transwell experiment
The cell invasiveness was examined with the 8-mm transwell (Millipore, Billerica, MA, USA)
with (invasion assays) or without (migration assays) the coating of Matrigel. According to previous study [Citation39], the cells were seeded into serum-free medium and added to the upper chamber (1 × 104 cells per chamber) coated with 200 mg/mL of Matrigel. A medium containing 10% FBS was added to the lower chamber as a chemical attractant. After 24 h of incubation, the cells in the upper chamber were removed by wiping with a cotton swab. We then fixed the cells invading the lower surface of the filter in 70% ethanol for 30 min, and stained them for an additional 10 min with 0.2% crystal violet. The invading cells were photographed and counted across five random fields of view in each cell under an optical microscope at 200× magnification.
5-ethynyl-2ʹ-deoxyuridine (EdU) assay
The EdU assay was conducted according to previous study [Citation40]. The cells were pre-inoculated in a 24-well plate at a density of 5 × 103 cells per well. The plated cells were then incubated in 4% methanol for 30 min, followed by permeabilization in 0.5% TritonX-100 for 10 min, and a 30-min reaction in 400 μL of 1× ApollorR. Afterward, the cells were stained with 4ʹ,6-diamidino-2-phenylindole for another 30 min. The EdU-stained cells were counted under a fluorescence microscope (CKX41-F32FL, Olympus, Tokyo, Japan) to calculate the percentage of EdU-positive cells.
Western blot analysis
The experimental method of Western blot referred to the previous literature [Citation41]. Total protein was extracted from the transfected cells and the equal quantity proteins (30 μg) were separated using a 10% polyacrylamide gel and transferred to a 0.22 μm polyvinylidene fluoride membrane. The membranes were then incubated in blocking buffer (5% skim milk) for 2 h, and then incubated with primary antibodies at 4°C overnight. After incubation with the corresponding secondary antibodies for 2 h at room temperature, the protein bars were visualized by chemiluminescence using the ECL reagent (Pierce, Rockford, IL, USA). The antibodies used were as follows: Wnt3a (ab219412, 1/1000, abcam), β-catenin (ab32572, 1/5000, abcam), β-actin (ab8226, 1/1000, abcam), VEGFA (ab52917, 1/10000, abcam) and the anti-rabbit and anti-mouse peroxidase-conjugated secondary antibodies (Santa Cruz Biotechnology).
Dual luciferase reporter experiment
We used bioinformatics websites (starbase, https://starbase.sysu.edu.cn/) to predict the potential targets of miR-195, PVT1, and VEGFA [Citation42]. A PVT1 3ʹ-UTR or a mutant PVT1 3ʹ-UTR with a predicted target site was inserted into a pGL3-promoter vector. Cells in the logarithmic growth phase were seeded into a 96-well plate at a density of 1.5 × 104 cells per well and cultured in an incubator for 24 h. After co-transfecting the cells for 48 h with a final concentration of 50 nmol/L of miRNA mimics or a non-target control, and 100 ng of the dual-reporter gene vector with a target gene 3ʹ UTR or a mutant vector 3ʹ UTR, the medium was aspirated and 35 μL fresh medium added per well. Next, 35 μL of luciferase (Promega, Madison, WI, USA) substrate was added to each well. After shaking for 10 min, the fluorescence intensity was measured. The experiment was repeated 3 times.
Statistical analysis
Data analysis was performed with the SPSS software (SPSS ver. 17.0, SPSS Inc., Chicago, IL, USA), and all the figures were processed with the GraphPad Prism software (Version X; La Jolla, CA, USA). Comparisons between two groups were analyzed using Student’s t-test. One-way analysis of variance (ANOVA) followed by Tukey’s test was utilized to analyze the comparisons among more than two groups. Fisher’s exact test was used to analyze the correlation between circPVT1 expression and PTC clinical features. The Kaplan–Meier method was applied to calculate the survival rate of patients. The results were displayed as mean ± standard deviation (SD). Statistical significance was set at p < 0.05.
Results
This study aimed to reveal the expression pattern, biological function and potential molecular mechanism of circPVT1 in PTC. We hypothesized that circPVT1 might act as a significant role in the progression of PTC. In basis of our results of in vitro experiments, we demonstrated that circPVT1 was obviously up-regulated in osteosarcoma tissues and cell lines, and circPVT1 could sponge miR-195 to up-regulate VEGFA expression to promote PTC progression.
CircPVT1 was highly expressed in cells from PTC tissue
Through RT-PCR, we found that the level of PVT1 expression in PTC tissue was significantly higher than that in non-cancerous tissue . Meanwhile, according to the median of circPVT1 level, we divided 50 cases into two groups, namely, Circ PVT1 High group (n = 25) and circPVT1 Low group (n = 25). The Kaplan–Meier curve indicated that the expression level of circPVT1 had no significant correlation with the overall survival rate of PTC patients (cut-off = 0.225, p> 0.05) . We also analyzed the correlation between the expression levels of PVT1 and special clinical pathological parameters. As shown in , the expression level of PVT1 was significantly related to tumor size, TNM stage, and lymph node metastasis in patients with PTC. Compared to Nthy-ori 3–1 cells, the PVT1 expression level in PTC cell lines (K1, IHH-4, BCPAP, and TCP1) increased significantly . We selected TPC-1 and BCPAP cells for further experiments. First of all, we transfected si-PVT1 into BCPAP cells and assessed the transfection efficiency. We observed a significant decrease in the PVT1 expression levels in the BCPAP cells due to this transfection .
Table 1. Relationship between circPVT1 expression and the clinical pathological characteristics of PTC patients (n = 50)
Figure 1. CircPVT1 had high expression in PTC tissue. a. Compared to cancer-side tissue, the level of PVT1 expression in thyroid papillary carcinoma tissue was significantly increased; b. Statistically analyzed the expression level of PVT1 had no significant correlation with PTC patients; c. Compared to human thyroid follicle epithelial cells (Nthy-ori 3–1), pvT1 was significantly high in thyroid papillary cell lines (K1, IHH-4, BCPAP, TCP1); d. PvT1 expression was significantly reduced after TPC-1 and BCPAP cells transfected si-PVT1. (** P < 0.01, the expression level of PVT1 was normalized to U6)
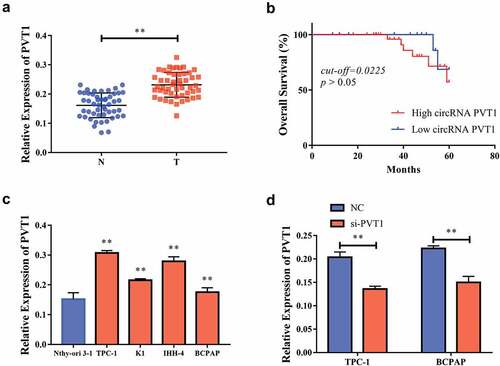
Downregulation of PVT1 could inhibit the proliferation, migration, and invasion of PTC cells
The effect of the PVT1 expression level on the PTC cells was detected using a CCK-8 test. After the reduction of PVT1 expression in TPC-1 and BCPAP cells, the cell proliferation capacity was significantly inhibited . Similar results were observed in the EdU experiment . The transwell assay showed that after the downregulation of PVT1 expression, cell migration , and invasiveness were also significantly reduced . Based on the above observations, we concluded that reducing PVT1 expression can significantly inhibit the proliferation, migration, and invasion of PTC cells.
Figure 2. Down-regulation of circPVT1 could restrain the multiplication, migration as well as aggression of thyroid papillary carcinoma cells. a. CCK-8 test showed that when reducing PVT1 expression in TPC-1 cells, cell proliferation was reduced; b. CCK-8 experiments showed that when PVT1 expression was reduced in BCPAP cells, cell multiplication was reduced; c, d. Flat-panel cloning experiments showed that in TPC-1 as well as BCPAP cells, when reducing PVT1 expression, cell multiplication ability decreased; e, f. Transwell experiments showed that the reduction of PVT1 expression in TPC-1 and BCPAP cells, and the ability to migrate cells decreased; g, h. Transwell experiments showed that the cell attack ability decreased when reducing the expression of PVT1 in TPC-1 as well as BCPAP cells. (** P < 0.01)
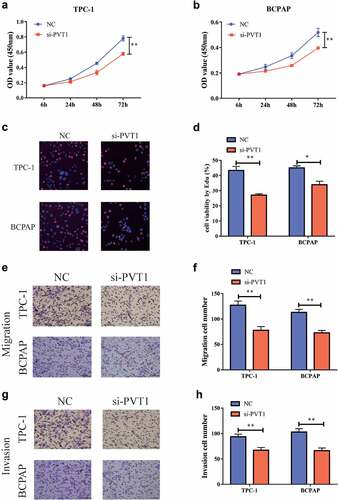
Downregulation of PVT1 could inhibit the Wnt/β-catenin signaling pathway
To further explore the specific role of PVT1 in PTC development, we lowered the expression of PVT1 in the PTC cells and detected the expression of genes related to the Wnt/β-catenin signaling pathway using RT-PCR. The results showed that following the inhibition of PVT1 expression, the mRNA expression of these genes was significantly decreased . Furthermore, the level of proteins related to Wnt/β-catenin signaling pathway-related genes was also significantly reduced . Thus, we found that following the inhibition of PVT1 expression, the expression of the Wnt/β-catenin signaling pathway was also significantly inhibited. This indicated that PVT1 could play a role in activating the Wnt/β-catenin signaling pathway.
Figure 3. circPVT1 could activate the Wnt/β-catenin signal pathway. a. Down-regulation of PVT1 expression in TPC-1 cells inhibits mRNA expression of Wnt/β-catenin signal pathway-related genes; b. Lower PVT1 expression in BCPAP cells inhibits the mRNA expression of Wnt/β-catenin signal pathway-related genes; c. Western blot showed that the level of gene proteins related to the Wnt/β-catenin signal pathway decreased when PVT1 expression was lowered in TPC-1 as well as BCPAP cells. (** P < 0.01)
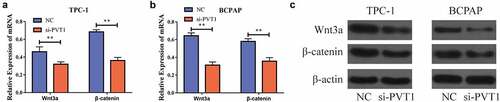
PVT1 could target miR-195 and regulate its expression
We used bioinformatics to predict potential binding targets for circPVT1 and miR-195 , and then constructed wild-type and mutant sequences of PVT1 (PVT1-WT and PVT1-MUT, respectively). Using dual-luciferase reporter gene experiments, we discovered that after the transfection of miR-195 mimics into the TPC-1 and BCPAP cells, the luciferase level in the PVT1-WT 3ʹUTR group was reduced, while there was no significant difference in the luciferase level in the PVT1-MUT 3ʹUTR group . This result implied that PVT1 could target miR-195. To further study the regulatory relationship between the two, we transfected the cells with an miR-195 inhibitor. Using RT-PCR, we found that inhibition resulted in significantly decreased miR-195 expression levels after transfection , and significantly increased PVT1 expression levels . In addition, we found that miR-195 expression was significantly increased after PVT1 expression was downregulated . Thus, we found that PVT1 could target miR-195 and inhibit its expression.
Figure4 circPVT1 could target miR-195. a. Bioinformatics predicts potential binding sites of PVT1 and miR-195; b. Dual luciferase reporting genetic experiment of TPC-1 cells shows that PVT1 can target miR-195; c. Dual luciferase reporting genetic experiment in BCPAP cells shows that PVT1 can target miR-195; d. After transfecting the miR-195 inhibitor into the TPC-1 cell, the expression level of miR-195 decreased; e. BCPAP cells have reduced the level of miR-195 expression after transiting miR-195 pontoon; f. PVT1 expression levels rises after down-regulation of miR-195 expression in TPC-1 as well as BCPAP cells; g. MiR-195 expression levels rises after the expression of PVT1 was reduced in TPC-1 and BCPAP cells. (** P < 0.01, the expression level of PVT1 was normalized to U6)
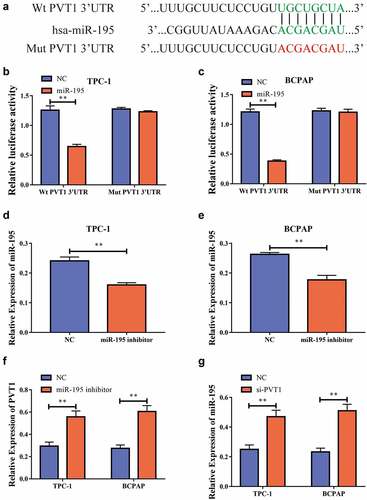
PVT1 could regulate VEGFA expression through miR-195
We also used bioinformatics to predict potential binding targets for miR-195 and VEGFA and then constructed VEGFA wild-type and mutant sequences (VEGFA-WT and VEGFA-MUT, respectively). Using a dual luciferase reporter gene experiment we found that, after transfection of miR-195 mimics into TPC-1 and BCPAP cells, the luciferase levels in the VEGFA-WT 3ʹUTR group decreased, while those in the VEGFA-MUT 3ʹUTR showed no significant difference . This suggested that miR-195 can target VEGFA. To further study the regulatory relationship between the two, we transfected cells with si-VEGFA to inhibit its expression. RT-PCR showed that following transfection, the VEGFA expression level in the cells was significantly reduced , while the miR-195 expression level significantly increased , and the PVT1 expression level decreased significantly . To further explore this regulatory behavior, we used RT-PCR to show that VEGFA expression levels increased after downregulation of miR-195 expression , and also that VEGFA expression was significantly suppressed after PVT1 expression was suppressed . Western blot analysis also showed the same results at the protein level . Based on the above results, we concluded that PVT1 could regulate the expression of VEGFA through targeted binding with miR-195 in the PTC cells.
Figure5 miR-195 could target VEGFA. a. Bioinformatics predicts the potential binding sites between miR-195 and VEGFA; b. Dual luciferase reporting gene experiment in TPC-1 cells shows that miR-195 can target VEGFA; c. Dual luciferase reporting gene experiment in BCPAP cells shows that miR-195 can target VEGFA; d. After transfecting si-VEGFA into TPC-1 as well as BCPAP cells, VEGFA expression was reduced; e. MiR-195 expression levels rises after down-regulating expression of VEGFA in TPC-1 and BCPAP cells; f. PVT1 expression level declines after VEGFA expression is lowered in TPC-1 and BCPAP cells; g. VEGFA expression levels rose after downward expression in TPC-1 and BCPAP cells; h. The expression of VEGFA is reduced after PVT1 expression is reduced in TPC-1 and BCPAP cells; i. Western blot shows that when lowering PVT1 expression in TPC-1 as well as BCPAP cells, VEGFA protein levels decrease; when miR-195 is downgraded, VEGFA protein levels increase. (** P < 0.01, the expression level of VEGFA was normalized to GAPDH)
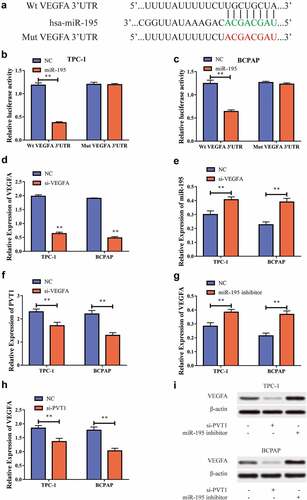
Lowering VEGFA restrained the growth, migration, and invasion of PTC cells
In order to further explore the specific role of VEGFA in the development of PTC, we tested its influence on cell proliferation capacity via a CCK-8 test. The results suggested that the cell proliferation capacity was significantly inhibited after the reduction of VEGFA expression in the TPC-1 and BCPAP cells. The EdU experiment revealed the same outcome . We used the transwell assay to detect cell migration and invasion and found that, after reducing VEGFA expression, the cell migration capacity was significantly reduced and the invasion capacity was also significantly inhibited . Thus, we concluded that reduced VEGFA expression can significantly inhibit the proliferation, migration, and invasion of PTC cells.
Figure 6. Down-regulation of VEGFA could restrain the multiplication, migration as well as aggression of thyroid papillary carcinoma cells. a. CCK-8 test shows that cell proliferation is reduced when reducing VEGFA expression in TPC-1 cells,; b. CCK-8 test shows that VEGFA expression is reduced in BCPAP cells, cell multiplication is reduced; c, d. Flat-panel cloning experiment shows that when VEGFA expression is reduced, cell proliferation is reduced in TPC-1 as well as BCPAP cells; e, f. Transwell experiments shows that the cell migration capacity is reduced when VEGFA expression is reduced in BCPAP and TPC-1 cells; g, h. Transwell experiments shows that cell aggression is reduced when VEGFA expression is reduced in BCPAP and TPC-1 cells. (** P < 0.01)
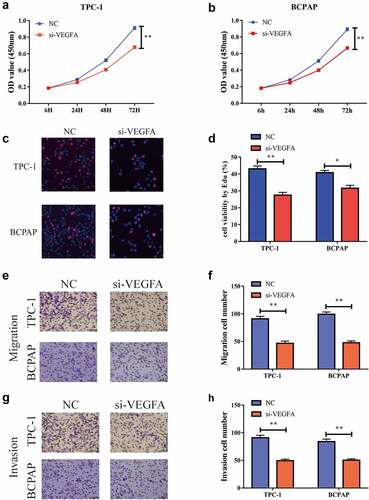
Downregulation of VEGFA inhibited the Wnt/β-catenin signaling pathway
In order to further elucidate the mechanism by which VEGFA is involved in the development of PTC, we tested the expression of Wnt/β-catenin signaling pathway-related genes through RT-PCR after lowering VEGFA expression in the cells. Upon lowering the VEGFA expression, the level of mRNA expression for the Wnt/β-catenin signaling pathway-related genes decreased significantly , and the Western blot also showed significantly lower Wnt/β-catenin signaling pathway-related protein levels . Taken together, we found that the Wnt/β-catenin signaling pathway-related gene expression also significantly inhibited VEGFA expression, and that PVT1 activated the Wnt/β-catenin signaling pathway by targeting miR-195 to upregulate VEGFA expression.
Figure 7. VEGFA could activate the Wnt/β-catenin signal pathway. a. Down-regulation of VEGFA expression in TPC-1 cells inhibits the mRNA expression of Wnt/β-catenin signal pathway-related genes; b. In BCPAP cells, when VEGFA expression is downregulated, the mRNA expression of Wnt/β-catenin signal pathway-related genes is inhibited; c. Western blot shows that when reducing VEGFA expression in BCPAP as well as TPC-1 cells, the protein levels of Wnt/β-catenin signal pathway-related genes decrease. (** P < 0.01)
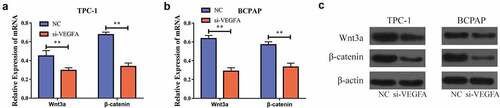
Discussion
In recent decades, the incidence of TC has been increasing, and it is the most common incretory tumor [Citation43,Citation44]. PTC is the main type of TC, accounting for approximately 90% of cases and with an overall 5-year survival rate of approximately 97% [Citation45,Citation46]. However, the clinical prognosis of late PTC is poor, partly because the molecular mechanism of PTC occurrence and development is not adequately understood. Therefore, finding new biomarkers and therapeutic targets to improve the diagnosis and therapeutic outcomes of PTC is a clinical problem.
Many ncRNAs have been shown to regulate the expression of genes in cancer cells and are expected to become molecular biomarkers of cancer [Citation12,Citation47]. The structure of circRNAs has been shown to be highly stable and resistant to RNA degradation pathways. This suggests that circRNAs may be more technically suitable as molecular biomarkers for human cancer. In recent years, many studies have shown that circRNAs are involved in the inniniation and development of PTC. Luo et al. identify that hsa_circ_0001018 can promote PTC by facilitating cell survival invasion, G/S cell cycle progression, and repress cell apoptosis via crosstalk with miR-338-3p and SRY-box transcription factor 4 (SOX4) [Citation48]. Chu et al show that circular RNA circRUNX1 can promote PTC progression and metastasis by sponging miR-296-3p and regulate DHD domain containing 2 (DDHD2) expression [Citation49]. Ye and colleagues have demonstrated that circular RNA circFOXM1 may play a vital role in PTC by sponging miR-1179 and regulate high mobility group box 1(HMGB1) expression [Citation50].
In this study, we found that circPVT1 had significantly increased expression in PTC tissue. Specifically, PVT1 expression level had a significant correlation with tumor size, TNM stage, and lymphatic metastasis in patients with PTC. In addition, we also found that the circPVT1expression level was significantly increased in the PTC cell lines compared to the Nthy-ori 3–1 cells. However, due to the limited number of specimens we collected and the short follow-up time, we have not yet observed the correlation between the expression level of CircPVT1 and the prognosis of PTC patients. At present, we have collected more specimens, and follow-up information will be improved. We will further verify the potential of circPVT1 as a diagnostic marker for PTC.
Then, we dectected the effect of circPVT1 on cell proliferation, migration and invasion. After reducing the expression level of circPVT1 in PTC cells, the ability of cell proliferation, migration, and invasion were significantly inhibited, and the Wnt/β-catenin signaling pathway was also inhibited. The Wnt/β-catenin signaling pathway, also known as the classic Wnt signaling pathway, has been studied in-depth [Citation51,Citation52]. Activation of the classic Wnt signaling pathway can contribute to extracellular Wnt combining with the transmembrane receptors LRP5/6 and Fzd to form a complex. These receptors then become activated, which in turn activates the intracellular Disheveled protein, causing the inhibition of glycogen synthase enzyme-3 activity and subsequent detachment from the axin protein. This prevents formation of the β-catenin degradation complex, which in turn, prevents phosphorylation and degradation of β-catenin, thereby increasing the intracellular β-catenin expression. When β-catenin reaches a certain level, free β-catenin undergoes nuclear transfer and binds to the transcription factor TCF/LEF to form a transcription-activated complex. This complex eventually enhances or weakens the expression of certain genes. The Wnt/β-catenin signaling pathway has also been proved to be involved in the progression of PTC [Citation53–55].
Previous studies documented that circRNAs were involved in regulating diverse biological processes via functioning as competing endogenous RNAs for miRNAs [Citation37,Citation38]. CircPVT1 has been proved to locate mainly in cytoplasm, which meant that it might act as microRNA sponges to regulate downstream gene expression. MiR-195 is dysregulated in several tumors, such as colon cancer [Citation56], non-small lung cancer [Citation57], laryngeal cancer [Citation58,Citation59], gastric cancer [Citation60], cervical cancer [Citation61], colorectal cancer [Citation62], breast cancer [Citation63], lung adenocarcinoma [Citation64] and osteosarcoma [Citation65]. Moreover, miR-195 has been demonstrated that play a significant role in PTC [Citation66–69]. VEGFA is a powerful and specific endothelial cell fissure agent that, in combination with receptors, can participate in promoting the division and proliferation of vascular endothelial cells and increase microvascular permeability [Citation70,Citation71]. VEGFA has been shown to play an important role in tumor development. VEGFA is not only important to endothelial cell survival but also helps circulating endothelial cells reach new blood vessels. This in turn can promote tumor growth and angiogenesis, and thus VEGFA expression may become a new tumor biomarker [Citation72,Citation73]. VEGFA also participates in the development of PTC [Citation74]. In this work, by using the dual luciferase reporter gene and Western blotting, we found that circPVT1 expression can be increased by targeting miR-195 to increase VEGFA expression. We have also shown in numerous experiments that the Wnt/β-catenin signaling pathway was inhibited and the cell proliferation, migration, and invasion abilities decreased after the reduction of VEGFA expression in cells. In summary, we found that circPVT1 could activate the Wnt/β-catenin signaling pathway to promote the development of PTC.
Of course, this article still has many shortcomings. First of all, we have not conducted in vivo experiments to further verify the biological effects and molecular mechanisms of circPVT1. Secondly, there are multiple binding sites for miRNAs on circPVT1. It remains to be explored whether circPVT1 binds to which miRNA plays the most important role. Thirdly, many studies have reported that ncRNAs have the potential to be developed as reagents for clinical treatment [Citation75,Citation76],whether circPVT1 can be used as a therapeutic target for corresponding research and development still needs a lot of experimental proof. Moreover, we also need to collect more clinical specimens to explore the clinical application potential of circPVT1.
Conclusion
Overall, our study demonstrated that circPVT1 expression was frequently increased in PTC and high expression level of circPVT1 was related to tumor growth and metastasis and poor prognosis. From the perspective of mechanism, circPVT1 could function as a molecule sponge for miR-195 and mediated the ceRNA network to promote the expression of VEGFA, finally promoted the malignant progression of PTC. Therefore, the circPVT1/ miR-195/VEGFA axis might be considered as a novel biomarker for prognosis and a promising therapeutic target for PTC treatment. Nonetheless, the current study only presents a theoretical basis for the mechanism of circPVT1 involvement in PTC. Further research is required to explore the specific mechanisms involving various other molecular pathways involved in PTC carcinogenesis.
Disclosure statement
No potential conflict of interest was reported by the author(s).
Data Availability Statement
The data used to support the findings of this study are available from the corresponding author upon reasonable request.
Additional information
Funding
References
- Wang TS, Sosa JA. Thyroid surgery for differentiated thyroid cancer - recent advances and future directions. Nat Rev Endocrinol. 2018;14(11):670–683.
- Leboulleux S, Tuttle RM, Pacini F, et al. Papillary thyroid microcarcinoma: time to shift from surgery to active surveillance? Lancet Diabetes Endocrinol. 2016;4(11):933–942.
- Lim AM, Solomon BJ. Immunotherapy for anaplastic thyroid carcinoma. J Clin Oncol. 2020;38(23):2603–2604.
- Kitahara CM, Sosa JA. The changing incidence of thyroid cancer. N at Rev Endocrinol. 2016;12(11):646–653.
- Tiedje V, Fagin JA. Therapeutic breakthroughs for metastatic thyroid cancer. Nat Rev Endocrinol. 2020;16(2):77–78.
- Fagin JA, Wells SA Jr. Biologic and clinical perspectives on thyroid cancer. N Engl J Med. 2016;375(11):1054–1067.
- Lin RX, Yang SL, Jia Y, et al. Epigenetic regulation of papillary thyroid carcinoma by long non-coding RNAs. Semin Cancer Biol. 2021. DOI:10.1016/j.semcancer.2021.03.027
- Vaccarella S, Lortet-Tieulent J, Colombet M, et al. Global patterns and trends in incidence and mortality of thyroid cancer in children and adolescents: a population-based study. Lancet Diabetes Endocrinol. 2021;9(3):144–152.
- Fallahi P, Ferrari SM, Elia G, et al. Primary cell cultures for the personalized therapy in aggressive thyroid cancer of follicular origin. Semin Cancer Biol. 2020; DOI:10.1016/j.semcancer.2020.06.013.
- How CW, Ong YS, Low SS, et al. How far have we explored fungi to fight cancer? Semin Cancer Biol. 2021. DOI:10.1016/j.semcancer.2021.03.009
- He L, Man C, Xiang S, et al. Circular RNAs’ cap-independent translation protein and its roles in carcinomas. Mol Cancer. 2021;20(1):119.
- Shen H, Liu B, Xu J, et al. Circular RNAs: characteristics, biogenesis, mechanisms and functions in liver cancer. J Hematol Oncol. 2021;14(1):134.
- Yang Y, Lei W, Jiang S, et al. CircRNAs: decrypting the novel targets of fibrosis and aging. Ageing Res Rev. 2021;70:101390.
- van Zonneveld AJ, Kolling M, Bijkerk R, et al. Circular RNAs in kidney disease and cancer. Nat Rev Nephrol. 2021;17(12):814–826.
- Zhou WY, Cai ZR, Liu J, et al. Circular RNA: metabolism, functions and interactions with proteins. Mol Cancer. 2020;19(1):172.
- Wang S, Zhang K, Tan S, et al. Circular RNAs in body fluids as cancer biomarkers: the new frontier of liquid biopsies. Mol Cancer. 2021;20(1):13.
- Tan KL, Chia WC, How CW, et al. Benchtop isolation and characterisation of small extracellular vesicles from human mesenchymal stem cells. Mol Biotechnol. 2021;63(9):780–791.
- Long F, Lin Z, Li L, et al. Comprehensive landscape and future perspectives of circular RNAs in colorectal cancer. Mol Cancer. 2021;20(1):26.
- Goodall GJ, Wickramasinghe VO. RNA in cancer. Nat Rev Cancer. 2020;21(1):22–36.
- Gokool A, Loy CT, Halliday GM, et al. Circular RNAs: the brain transcriptome comes full circle. Trends Neurosci. 2020;43(10):752–766.
- Ma S, Kong S, Wang F, et al. CircRNAs: biogenesis, functions, and role in drug-resistant Tumours. Mol Cancer. 2020;19(1):119.
- Chen LL. The expanding regulatory mechanisms and cellular functions of circular RNAs. Nat Rev Mol Cell Biol. 2020;21(8):475–490.
- Wen G, Zhou T, Gu W. The potential of using blood circular RNA as liquid biopsy biomarker for human diseases. Protein Cell. 2020. DOI:10.1007/s13238-020-00799-3
- Li G, Guo BY, Wang HD, et al. CircRNA hsa_circ_0014130 function as a miR-132-3p sponge for playing oncogenic roles in bladder cancer via upregulating KCNJ12 expression. Cell Biol Toxicol. 2021. DOI:10.1007/s10565-021-09668-z
- Zhang X, Wu N, Wang J. Hsa-circ_0058106 induces EMT and metastasis in laryngeal cancer via sponging miR-153 and inducing Twist1 nuclear translocation. Cell Oncol (Dordr). 2021. DOI:10.1007/s13402-021-00644-7
- Cai Y, Zhao X, Chen D, et al. circ-NOL10 regulated by MTDH/CASC3 inhibits breast cancer progression and metastasis via multiple miRNAs and PDCD4. Mol Ther Nucleic Acids. 2021;26:773–786.
- Ghetti M, Vannini I, Storlazzi CT, et al. Linear and circular PVT1 in hematological malignancies and immune response: two faces of the same coin. Mol Cancer. 2020;19(1):69.
- Traversa D, Simonetti G, Tolomeo D, et al. Unravelling similarities and differences in the role of circular and linear PVT1 in cancer and human disease. Br J Cancer. 2021. DOI:10.1038/s41416-021-01584-7
- Yu F, Lin Y, Ai MM, et al. Knockdown of circular RNA hsa_circ_PVT1 inhibited laryngeal cancer progression via preventing wnt4/beta-Catenin signaling pathway activation. Front Cell Dev Biol. 2021;9:658115.
- Li X, Zhang Z, Jiang H, et al. Circular RNA circPVT1 promotes proliferation and invasion through sponging miR-125b and activating E2F2 signaling in non-small cell lung cancer. Cell Physiol Biochem. 2018;51(5):2324–2340.
- Chen J, Li Y, Zheng Q, et al. Circular RNA profile identifies circPVT1 as a proliferative factor and prognostic marker in gastric cancer. Cancer Lett. 2017;388:208–219.
- Zheng Z, Chen Z, Zhong Q, et al. CircPVT1 promotes progression in clear cell renal cell carcinoma by sponging miR-145-5p and regulating TBX15 expression. Cancer Sci. 2021;112(4):1443–1456.
- Li M, Chi C, Zhou L, et al. Circular PVT1 regulates cell proliferation and invasion via miR-149-5p/FOXM1 axis in ovarian cancer. J Cancer. 2021;12(2):611–621.
- Wang H, Wei M, Kang Y, et al. Circular RNA circ_PVT1 induces epithelial-mesenchymal transition to promote metastasis of cervical cancer. Aging (Albany NY). 2020;12(20):20139–20151.
- Bian Q. Circular RNA PVT1 promotes the invasion and epithelial-mesenchymal transition of breast cancer cells through serving as a competing endogenous RNA for miR-204-5p. Onco Targets Ther. 2019;12:11817–11826.
- Ishola AA, Chien CS, Yang YP, et al. Oncogenic circRNA hsa_circ_0000190 modulates EGFR/ERK pathway in promoting NSCLC. Cancer Res. 2021; DOI:10.1158/0008-5472.CAN-21-1473.
- Chen SW, Zhu SQ, Pei X, et al. Cancer cell-derived exosomal circUSP7 induces CD8(+) T cell dysfunction and anti-PD1 resistance by regulating the miR-934/SHP2 axis in NSCLC. Mol Cancer. 2021;20(1):144.
- Li J, Gao X, Zhang Z, et al. CircCD44 plays oncogenic roles in triple-negative breast cancer by modulating the miR-502-5p/KRAS and IGF2BP2/Myc axes. Mol Cancer. 2021;20(1):138.
- Mao W, Wang K, Xu B, et al. ciRS-7 is a prognostic biomarker and potential gene therapy target for renal cell carcinoma. Mol Cancer. 2021;20(1):142.
- Rong D, Wu F, Lu C, et al. m6A modification of circHPS5 and hepatocellular carcinoma progression through HMGA2 expression. Mol Ther Nucleic Acids. 2021;26:637–648.
- Yin H, Chen L, Piao S, et al. M6A RNA methylation-mediated RMRP stability renders proliferation and progression of non-small cell lung cancer through regulating TGFBR1/SMAD2/SMAD3 pathway. Cell Death Differ. 2021. DOI:10.1038/s41418-021-00888-8
- Li JH, Liu S, Zhou H, et al. starBase v2.0: decoding miRNA-ceRNA, miRNA-ncRNA and protein-RNA interaction networks from large-scale CLIP-Seq data. Nucleic Acids Res. 2014;42(D1):D92–7.
- Salvatore D, Santoro M, Schlumberger M. The importance of the RET gene in thyroid cancer and therapeutic implications. Nat Rev Endocrinol. 2021;17(5):296–306.
- Fallahi P, Ferrari SM, Galdiero MR, et al. Molecular targets of tyrosine kinase inhibitors in thyroid cancer. Semin Cancer Biol. 2020. DOI:10.1016/j.semcancer.2020.11.013
- Dsa M, Zhang L, Durante C, et al. Contemporary debates in adult papillary thyroid cancer management. Endocr Rev. 2019;40(6):1481–1499.
- Pstrag N, Ziemnicka K, Bluyssen H, et al. Thyroid cancers of follicular origin in a genomic light: in-depth overview of common and unique molecular marker candidates. Mol Cancer. 2018;17(1):116.
- Lauretti E, Dabrowski K, Pratico D. The neurobiology of non-coding RNAs and alzheimer’s disease pathogenesis: pathways, mechanisms and translational opportunities. Ageing Res Rev. 2021;71:101425.
- Luo Q, Guo F, Fu Q, et al. hsa_circ_0001018 promotes papillary thyroid cancer by facilitating cell survival invasion, G1/S cell cycle progression, and repressing cell apoptosis via crosstalk with miR-338-3p and SOX4. Mol Ther Nucleic Acids. 2021;24:591–609.
- Chu, J , Tao, L , and Yao, T. Circular RNA circRUNX1 promotes papillary thyroid cancer progression and metastasis by sponging MiR-296-3p and regulating DDHD2 expression. Cell Death Dis. 2021;122: 112.
- Ye M, Hou H, Shen M, et al. Circular RNA circFOXM1 plays a role in papillary thyroid carcinoma by sponging miR-1179 and regulating HMGB1 expression. Mol Ther Nucleic Acids. 2020;19:741–750.
- Yu F, Yu C, Li F, et al. Wnt/beta-catenin signaling in cancers and targeted therapies. Signal Transduct Target Ther. 2021;6(1):307.
- Tewari D, Bawari S, Sharma S, et al. Targeting the crosstalk between canonical Wnt/beta-catenin and inflammatory signaling cascades: a novel strategy for cancer prevention and therapy. Pharmacol Ther. 2021;227:107876.
- Dai W, Jin X, Han L, et al. Exosomal lncRNA DOCK9-AS2 derived from cancer stem cell-like cells activated Wnt/beta-catenin pathway to aggravate stemness, proliferation, migration, and invasion in papillary thyroid carcinoma. Cell Death Dis. 2020;11(9):743.
- Ma J, Huang X, Li Z, et al. FOXE1 supports the tumor promotion of Gli2 on papillary thyroid carcinoma by the Wnt/β-catenin pathway. J Cell Physiol. 2019;234(10):17739–17748.
- Bi W, Huang J, Nie C, et al. CircRNA circRNA_102171 promotes papillary thyroid cancer progression through modulating CTNNBIP1-dependent activation of beta-catenin pathway. J Exp Clin Cancer Res. 2018;37(1):275.
- Gu H, Xu Z, Zhang J, et al. circ_0038718 promotes colon cancer cell malignant progression via the miR-195-5p/Axin2 signaling axis and also effect Wnt/beta-catenin signal pathway. BMC Genomics. 2021;22(1):768.
- Xi J, Xi Y, Zhang Z, et al. Hsa_circ_0060937 accelerates non-small cell lung cancer progression via modulating miR-195-5p/HMGB3 pathway. Cell Cycle. 2021;20(19):2040–2052.
- Zhou M, Wang Y, Zhang C, et al. MicroRNA‑195‑5p suppresses the proliferation, migration, invasion and epithelial‑mesenchymal transition of laryngeal cancer cells in vitro by targeting E2F3. Exp Ther Med. 2021;22(4):1078.
- Shen D, Li J, Tao K, et al. Long non-coding RNA MCM3AP antisense RNA 1 promotes non-small cell lung cancer progression through targeting microRNA-195-5p. Bioengineered. 2021;12(1):3525–3538.
- Yan B, Ren Z, Sun J, et al. IGF2-AS knockdown inhibits glycolysis and accelerates apoptosis of gastric cancer cells through targeting miR-195/CREB1 axis. Biomed Pharmacother. 2020;130:110600.
- Liu S, Li B, Li Y, et al. Circular RNA circ_0000228 promotes the malignancy of cervical cancer via microRNA-195-5p/ lysyl oxidase-like protein 2 axis. Bioengineered. 2021;12(1):4397–4406.
- Li S, Lv C, Li J, et al. LncRNA LINC00473 promoted colorectal cancer cell proliferation and invasion by targeting miR-195 expression. Am J Transl Res. 2021;13(6):6066–6075.
- Wu J, Xu W, Ma L, et al. Formononetin relieves the facilitating effect of lncRNA AFAP1-AS1-miR-195/miR-545 axis on progression and chemo-resistance of triple-negative breast cancer. Aging (Albany NY). 2021;13(14):18191–18222.
- Wang A, Zhang T, Wei W, et al. The Long Noncoding RNA LINC00665 facilitates c-myc transcriptional activity via the miR-195-5p MYCBP axis to promote progression of lung adenocarcinoma. Front Oncol. 2021;11:666551.
- Yang B, Li L, Tong G, et al. Circular RNA circ_001422 promotes the progression and metastasis of osteosarcoma via the miR-195-5p/FGF2/PI3K/Akt axis. J Exp Clin Cancer Res. 2021;40(1):235.
- Li C, Zhu L, Fu L, et al. CircRNA NRIP1 promotes papillary thyroid carcinoma progression by sponging mir-195-5p and modulating the P38 MAPK and JAK/STAT pathways. Diagn Pathol. 2021;16(1):93.
- Xu J, Li Z, Su Q, et al. Suppression of long noncoding RNA LINC00324 restricts cell proliferation and invasion of papillary thyroid carcinoma through downregulation of TRIM29 via upregulating microRNA-195-5p. Aging (Albany NY). 2020;12(24):26000–26011.
- Gui X, Li Y, Zhang X, et al. Circ_LDLR promoted the development of papillary thyroid carcinoma via regulating miR-195-5p/LIPH axis. Cancer Cell Int. 2020;20(1):241.
- Yin Y, Hong S, Yu S, et al. MiR-195 inhibits tumor growth and metastasis in papillary thyroid carcinoma cell lines by targeting CCND1 and FGF2. Int J Endocrinol. 2017;2017:6180425.
- Guryanov I, Tennikova T, and Urtti A. Peptide inhibitors of vascular endothelial growth factor A: current situation and perspectives. Pharmaceutics. 2021; 13(9) ;1337.
- Guo X, Yi H, and Li TC, et al. Role of Vascular Endothelial Growth Factor (VEGF) in human embryo implantation: clinical Implications. Biomolecules. 2021;11(2) ;253.
- Ren S, Tan X, Fu MZ, et al. Downregulation of miR-375 contributes to ERBB2-mediated VEGFA overexpression in esophageal cancer. J Cancer. 2021;12(23):7138–7146.
- Ciccarese C, Iacovelli R, Porta C, et al. Efficacy of VEGFR-TKIs plus immune checkpoint inhibitors in metastatic renal cell carcinoma patients with favorable IMDC prognosis. Cancer Treat Rev. 2021;100:102295.
- Wang R, Ma Q, Ji L, et al. miR-622 suppresses tumor formation by directly targeting VEGFA in papillary thyroid carcinoma. Onco Targets Ther. 2018;11:1501–1509.
- Looi QH, Foo JB, Lim MT, et al. How far have we reached in development of effective influenza vaccine? Int Rev Immunol. 2018;37(5):266–276.
- Yu AM, Tu MJ. Deliver the promise: rNAs as a new class of molecular entities for therapy and vaccination. Pharmacol Ther. 2021;107967. doi:10.1016/j.pharmthera.2021.107967