ABSTRACT
In this study, we aimed to investigate the effect of Magnolol on Alzheimer’s disease (AD). After the model of streptozotocin-induced AD mice with brain insulin resistance was established, the mice were treated with Magnolol or miR-200c antagomiR. The abilities of ambulations, rearings, discrimination, spatial learning, and memory were evaluated by open-field test (OFT), novel object recognition (NOR), and morris water maze (MWM) tests. The levels of malondialdehyde (MDA), glutathione (GSH), superoxide dismutase (SOD), tumor necrosis factor-α (TNF-α), interleukin-6 (IL-6), C-reactive protein (CRP), and miR-200c in the mice hippocampus were evaluated by enzyme-linked immunosorbent assay, Western blot, or Quantitative real-time Polymerase Chain Reaction. In AD mice model, streptozotocin induced the locomotor impairment and cognitive deficit, up-regulated levels of MDA, TNF-α, IL-6, and CRP, while down-regulated levels of GSH, SOD, and miR-200c. Magnolol increased the rearings numbers and discrimination index of AD mice in OFT and NOR tests. Magnolol increased the number of entries in the target quadrant and time spent in the target quadrant and decreased the escape latency of AD mice in the MWM test. Magnolol also down-regulated the levels of MDA, TNF-α, IL-6, and CRP, and up-regulated the levels of GSH, SOD, and miR-200c in the hippocampus tissues of AD mice. However, miR-200c antagomiR did the opposite and further offset the effects of the Magnolol on AD mice. Magnolol attenuated the locomotor impairment, cognitive deficit, and neuroinflammatory in AD mice with brain insulin resistance via up-regulating miR-200c.
Introduction
Alzheimer’s disease (AD), the main cause of senile dementia, is a degenerative disease of the central nervous system characterized by cognitive dysfunction and behavioral impairment [Citation1,Citation2]. Patients with AD suffer from progressive memory loss and cognitive decline, which lead to a negative impact on their daily life, social activities, and work abilities, along with various neuropsychiatric symptoms and behavioral disorders [Citation3–6]. Brain insulin resistance is one of the key features of AD [Citation7]. Insulin shows neuromodulatory actions in the brain and insulin signaling is involved in neuronal plasticity, gene expression, energy production, and neuronal survival [Citation8]. Insulin signaling impairment and brain insulin resistance may lead to impaired memory and cognitive dysfunction in AD [Citation8,Citation9]. Therefore, to attenuate the locomotor and cognitive deficits caused by brain insulin resistance in AD might be one of the key treatments for AD.
Several bioactive substances isolated from natural products have been proved to possibly exert anti-AD effects according to a majority of preclinical and clinical trials [Citation10,Citation11]. For instance, Ellagic acid possesses the effects of neuroprotection and cognitive enhancement in sporadic AD [Citation12]. Chrysophyllum albidum fruit-supplemented diet can enhance memory function and prevent cognitive deficits induced by lipopolysaccharide by inhibiting oxidative stress-related processes, acetylcholinesterase activity and pro-inflammatory mediators [Citation13]. Naringin can protect the nerve center of mice from damage by enhancing cholinergic transmission, antioxidant defense system, and inhibiting lipid peroxidation and nitrification processes [Citation14]. Besides, Luteolin can attenuate memory impairment in AD model mice [Citation15], and Cryptotanshinone ameliorates inflammation in the mice by down-regulating pro-inflammatory pathways [Citation16].
Magnolol is one of the important active ingredients from Magnolia Officinalis [Citation17,Citation18]. Owing to the extensive and important uses of Magnolia Officinalis in clinical practice, scholars at home and abroad have conducted numerous studies on its active ingredients, including Magnolol [Citation17,Citation19]. With the deepening of the study, plentiful biological activities of Magnolol have been gradually uncovered, such as anti-cancer, anti-inflammation, and anti-oxidation [Citation20–22]. For example, Magnolol holds the capacity to suppress the growth of cancer cells and enhance apoptosis of the cells in esophagus cancer, osteosarcoma cancer, colorectal cancer, etc. [Citation22–24]. Moreover, Magnolol can mitigate neuroinflammation and oxidative stress in mice with prefrontal cortex [Citation25], and it also has the ability to mitigate oxidative stress in adipocytes [Citation21]. In addition, a recent research has proved that Magnolol can attenuate inflammation in the mice model of formalin-induced inflammatory pain and has no deteriorating effect on their locomotor and cognitive abilities [Citation9]. However, there is still no clue about the effect of Magnolol on the neuroinflammation, locomotor impairment, and cognitive deficit caused by AD. Considering that microRNA (miRNA, miR)-200 c was previously proved to possess a preventive effect against Aβ peptide-induced toxicity in an AD mice model [Citation26], and that Magnolol up-regulates the expression of miR-200c in cancer cells to exert its anti-cancer effect [Citation27], we speculated that Magnolol had a regulatory effect on AD, which was also associated with miR-200c.
Based on the current background, this study aimed to investigate the effects of Magnolol and miR-200c on neuroinflammation, locomotor impairment, and cognitive deficit in AD, and further explore the potential mechanism underlying the effect. The aim of this study was to reveal the effect of Magnolol on motor injury, cognitive deficits, and neuroinflammation in AD disease mice with brain insulin resistance by regulating miR-200c expression.
Materials and Methods
Animals and ethics statement
In the present study, 120 male BALB/c mice (Age: six-week-old, weighed 25–30 g) were bought from Jiangsu ALF Biotechnology (Jiangsu, China, http://www.jsalfei.com/). All mice were raised for 5 days in a SPF environment before the experiment. All animal experiments were approved by the Committee of Experimental Animals of the First Affiliated Hospital of Zhejiang Chinese Medical University (Zhejiang Provincial Hospital of Traditional Chinese Medicine) (Z20190712N).
Establishment of brain insulin resistance AD mice model
The brain insulin resistance AD mice model was established in the light of a previous publication [Citation28]. In brief, after the mice were anesthetized by intraperitoneal injection of 50 mg/kg sodium pentobarbital (1,507,002, MREDA, Beijing, China), their scalp was tightened with a stereotactic frame. Then a midline sagittal incision was made on the scalp with a scalpel blade. The coordinates of 0.8 mm behind bregma, 1.5 mm on the left and right sides, and 3.6 mm below the skull surface were adjusted digitally. A burr hole was subsequently drilled on the skulls on both sides of the lateral ventricle with a drilling machine (18,036–24, ZeRun, Shenzhen, China), after which a bilateral injection of 3 mg/kg streptozotocin (STZ; S0130, Sigma-Aldrich, St Louis, MO, USA) was slowly administered with a volume of around 4 µl for each side at a speed of almost 1 µl/min. After 2 min of injection, the mice were taken out of the stereotactic frame and the burr holes (the injection place) were filled with dental cement.After being dried, the dental cement was removed. The wounds in the head skin were also stitched with surgical thread, (12,051–11, ZeRun, Shenzhen, China) and the mice were then raised for further treatment.
Animal grouping
The animal experiment consisted of two parts. Fifty mice were involved in the first part and seventy mice were involved in the second part.
In the first part, the mice were randomly divided into five groups (n = 10 for each group): Control group, Model group, Magnolol 5 group, Magnolol 10 group, and Magnolol 15 group. In the second part, miR-200c AntagomiR and its negative control (AntagomiR-NC) were purchased from RIBOBIO (Guangzhou, China), and the mice were randomly divided into seven groups (n = 10 for each group): Model group, AntagomiR-NC group, AntagomiR group, AntagomiR-NC+Magnolol 10 group, AntagomiR-NC+Magnolol 15 group, AntagomiR+Magnolol 10 group, and AntagomiR+Magnolol 15 group.
Animal treatment
The mice in the Control group were normally raised without any treatment and set as normal control, whereas those in the Model group only underwent a surgery to establish AD model. Besides, the mice in the Magnolol 5 group, Magnolol 10 group, and Magnolol 15 group were intragastrically administered with 5 mg/kg, 10 mg/kg, and 15 mg/kg Magnolol (gnololtered with 5 mg/kg, 1 St Louis, MO, USA) respectively once a day for 21 days based on the Model group.
The mice in the Model group only underwent a surgery to establish AD model, whilst those in the AntagomiR-NC group and the AntagomiR group were intracranially injected with AntagomiR negative control and miR-200c AntagomiR respectively after the intracranial injection of STZ during the surgery to establish the AD model. In the AntagomiR-NC+Magnolol 10 group and the AntagomiR-NC+Magnolol 15 group, based on the AntagomiR-NC group, the mice were intraperitoneally injected with 10 mg/kg and 15 mg/kg Magnolol once a day for 21 days, respectively. In the AntagomiR+Magnolol 10 group and the AntagomiR+Magnolol 15 group, based on the AntagomiR group, the mice were intraperitoneally injected with 10 mg/kg and 15 mg/kg Magnolol once a day for 21 days, respectively. We chose the dose and treatment time of Magnolol according to the study of Yi-Ruu Lin et al [Citation29].
On the 14th day, the open-field test (OFT) was performed in all mice. On the 15th and 16th days, the novel object recognition (NOR) test was conducted in all mice. From the 17th to the 21st day, all mice received the morris water maze (MWM) test. After the completion of MWM test, all mice were anesthetized by intraperitoneal injection of 50 mg/kg sodium pentobarbital and sacrificed by cervical dislocation. Finally, the hippocampus tissues of all mice were taken out for further use.
OFT
On the 14th day, the OFT was performed in all mouse [Citation30]. The OFT was carried out in the open field test using a video analysis system (XR-XZ301, XinRuan information Technology Co., Ltd, Shanghai, China), and the experimental device consists of two parts as the open field (40 cm × 40 cm × 40 cm; central area: 20 cm × 20 cm) and the data automatic acquisition and processing system. The experiments were all performed at 9:30 am so as to keepa quiet experimental environment. In order to eliminate odors, the field was cleaned with 70% alcohol (E111991, Aladdin, Shanghai, China) before each mouse was tested. The mice were removed from the home cage, and placed in the center of the bottom in the box. The timing and photographed were started simultaneously and stopped 5 minutes (min) later. At the end of the experiment, the experimental data were analyzed by the video analysis system, including the crossings and rearings numbers.
NOR evaluation
The NOR test was conducted on the 15th day and the 16th day as previously described [Citation9] to evaluate the discrimination capacity of all mice for two different objects. The test was performed under a circular open feldspar with a diameter of 50 cm and a height of 40 cm. On the 15th day, the mice were trained for 2 min firstly under the circular open feldspar to adapt to the environment and familiarize themselves with the arena. Then two similar rectangular objects (10 cm high) were taped to the arena floor 10 cm above the ground. The distance between the two objects was nearly 20 cm. Each mouse was then placed in the circular arena, and it was considered that the mice explored the objects (the whole process of exploration was recorded on video) when the distance between the nose of mice and the object was less than or equal to 2 cm. Then the mice were taken out of the arena. The arena and two objects were cleaned with 75% ethanol (E885996, Macklin, Shanghai, China) to eliminate the olfactory cues of the mice.
On the 16th day, one of the two objects was replaced with a novel object of the same height but with completely different shape and color. The novel object was placed in the same position and fixed in the same way as the previous one. Each mouse was then placed in the circular arena again to explore the familiar and novel objects, and the whole process of exploration was recorded on a video camera (FDR-AX60, SONY) again. The time to explore the familiar object was named Tf and the time to explore the novel object was designated as Tn. Finally, the discrimination index was calculated by the formula: discrimination index = (Tn–Tf)/(Tn+Tf).
MWM test
The MWM test was conducted on the 17th day and the 21st day as previously described [Citation9] to measure the abilities of spatial learning and memory in all mice. Mice were trained during the first four days (from the 17th day to the 20th day) and subjected to a probe trial on the last day (the 21st day). The water maze was a circular pool with a diameter of 180 cm and a height of 60 cm. Briefly, 24 hours (h) before the spatial learning trial, the mice were familiarized with the water maze for 60 seconds (s). During the first four days of training, mice were trained to find the hidden platform which was 1 cm below the water and the time to reach the platform (escapes latency) was documented. During the trials, mice that failed to reach the hidden platform within 60 s were guided to the platform and stayed on the platform for 30 s. On the last day, a 60 s probe test was conducted without the hidden platform to evaluate the memory of mice, and the time spent in the target quadrant and the number of entries in the target quadrant were documented by a video camera (FDR-AX60, SONY, Tokyo, Japan) and analyzed by the SMART v.3.0 software system (Panlab, Barcelona, Spain).
Enzyme-linked immunosorbent assay[Citation31]
The MDA ELISA kit (Jianglai Biotechnology Co., Ltd., Shanghai, China; http://jianglai.foodmate.net/sell/itemid-266191.shtml) was used to evaluate the level of MDA in tissue samples. According to the operating instructions of the kit, the standards were diluted and the samples were prepared, then the prepared standards (50 μl) and tissue samples (50 μl) were added to a 96-well plate, after which the plate was incubated at 37°C for 30 min. Next, the plate was washed with wash buffer and added with 50 μl of Enzyme-labeled reagent, which was then incubated (37°C) for 30 min and washed again with wash buffer. Finally, the 50 μl of coloration buffer A and 50 μl of coloration buffer B were added into the plate for further 15 min of incubation in the dark. Finally, the 50 μl of stop buffer was added into the 96-well plate. The absorbance of each well was measured under the Imark microplate reader (Bio-Rad, Alfred Nobel Drive Hercules, CA, USA).
Mice ELISA kits for Glutathione (GSH; MM-44117 M2, MEIMIAN, China), superoxide dismutase (SOD; MM-0389 M2, MEIMIAN, China), tumor necrosis factor-α (TNF-α; MM-0132 M2, MEIMIAN, China), interleukin-6 (IL-6; MM-1011 M2, MEIMIAN, China), and C-reactive protein (CRP; MM-0074 M2, MEIMIAN, China) were collected. In brief, the homogenates of hippocampus tissues (50 μl) and standard samples (50 μl) were separately added into the 96-well plate provided in the ELISA kits. After being incubated at 37°C for 30 min, the plate was washed five times with wash buffer. Then 100 μl of working buffer was added into the 96-well plate and incubated at 37°C for 30 min. After washing five times with wash buffer, the 96-well plate was added with 100 μl of coloration buffer and incubated at 37°C for 10 min. Finally, the stop buffer was added into the 96-well plate and the absorbance of each well was measured under the Imark microplate reader.
Real-time fluorescent quantitative polymerase chain reaction
MiRNAs in the mice hippocampus tissues were extracted using a PureLink miRNA Isolation Kit (K157001, Invitrogen, MA, USA) for quantifying the expression of miR-200c. Then the reverse-transcription was conducted using a PrimeScript RT reagent Kit (RR047A, Takara, Tokyo, Japan). In brief, 2 μg of miRNA was mixed with 2 μl of gDNA Eraser Buffer, 1 μl of gDNA Eraser, and 5 μl of RNase Free dH2O, and the mixed solution was reacted at room temperature for 30 min. The solution was further mixed with 1 μl of PrimeScipt RT Enzyme Mix, 1 μl of RT Primer Mix, 4 μl of PrimeScript Buffer 2, and 4 μl of RNase Free dH2O. After reaction at 37°C for 15 min and at 85°C for 5 s, the cDNA solution was collected and stored at 4°C. Finally, the amplification of cDNA was conducted also using the PrimeScript RT reagent Kit. In short, 2 μl of cDNA solution was mixed with 12.5 μl of SYBR Premix Ex TaqII, 2 μl primers of miR-200c or U6, and 8.5 μl of dH2O. The mixed solution was further reacted in the QuantStudio6 system (Applied Biosystems, CA, USA) under the conditions as follows: at 95°C for 30 s, 95°C for 5 s, and 60°C for 35 s, for 40 cycles. After the reaction was completed, the expression level of miR-200c was quantified using the 2−ΔΔCt method [Citation32], with U6 serving as an internal control. The primers of miR-200c were: F: 5ʹ-CTTAAAGCCCCTTCGTCTCC-3ʹ, R: 5ʹ-ACCGATTTACCCACCCTCAT-3ʹ; and those of U6 were: F: 5ʹ-CTCGCTTCGGCAGCACA-3ʹ, R: 5ʹ-AACGCTTCACGAATTTGCGT-3ʹ.
Western blot[Citation33]
The homogenates of mice hippocampus were incubated with NP-40 (R21234, OKA, Beijing, China) for 15 min and centrifuged (14,000 × g) at 4°C for 30 min. Afterward, the total protein in the hippocampus tissues was collected and subsequently subjected to the detection of concentration using a BCA detection kit (P0012S, Beyotime, Shanghai, China). After denaturation, the protein was added into a 10% SDS-PAGE gel (CW0022, CWBIO, Beijing, China), electrophoresed for 1.5 h, and then transferred on the PVDF membrane (JKA40001, OKA). The membrane subsequently was blocked with 5% nonfat milk at room temperature for 1 h and incubated with the primary antibodies at 4°C overnight. After the membrane was further incubated with the secondary antibody at room temperature for 2 h, the signal of protein was detected and quantified by Image Lab 3.0 (Bio-Ras, CA, USA). In this test, all information of antibodies is listed in .
Table 1. All antibodies information and sources in Western blot in this study
Statistical analysis
In this study, SPSS 20.0 was used for statistical analysis of data. Multiple sets of data were analyzed by one-way ANOVA. Dunnett’s was used as a post-hoc test. P < 0.05 indicated that the data were statistically significant.
Results
Magnolol ameliorated the impairment of locomotor activity and cognitive function, and regulated the levels of oxidative-related factors in AD model mice with brain insulin resistance
In order to explore the effects of Magnolol on AD mouse model, we examined the effects of Magnolol on motor and cognitive functions of AD brain insulin resistant mice, as well as the expression levels of oxygen-related factors. The OFT was firstly conducted to evaluate the locomotor activity of the mouse, and the results showed that the numbers of crossings () and rearing () of the AD mice were significantly decreased as compared to the Control group (P < 0.05), while after the mice were treated with 10 mg/kg and 15 mg/kg Magnolol, the number of rearing was increased when compared with the Model group (P < 0.05). Then NOR test was conducted to evaluate the discrimination ability of the mouse. As exhibited in , the discrimination index of the AD mice was significantly reduced as compared to the Control group (P < 0.001), while an increased index was evidenced after the mouse were treated with 5 mg/kg, 10 mg/kg, and 15 mg/kg Magnolol when compared with the Model group (P < 0.001). Besides, the MWM test () was further performed to evaluate the spatial learning and memory ability of the mouse. When compared with the Control group, the escape latency () was increased and the number of entries in the target quadrant () and the time spent in the target quadrant () were decreased in AD mouse (P < 0.001). However, after mice in the model group were treated with different doses of magnolol compared to mice in the only model treatment group, the escape latency (P < 0.05) was decreased and the number of entries in the target quadrant (P < 0.001) and the time spent in the target quadrant (P < 0.001) were increased. All these phenomena suggested that Magnolol ameliorated the impairment of the locomotor activity and cognitive function of AD model mice with brain insulin resistance.
Figure 1. Magnolol ameliorated the impairment of locomotor activity and cognitive function, and regulated the levels of oxidative-related factors in AD model mice with brain insulin resistance. (a-b) OFT was performed to measure the locomotor activity of the mice. (c) NOR evaluation was conducted to measure the discrimination ability of the mice. (d-f) MWM test was used to evaluate the spatial learning and memory ability of the mice. (g-i) The secretions of MDA (g), GSH (h), and SOD (i) in hippocampus tissues of the mice were detected by ELISA. (*P < 0.05, ***P < 0.001, vs. Control; ^P < 0.05, ^^P < 0.01, ^^^P < 0.001, vs. Model). (AD: Alzheimer’s disease, OFT: open-field test, NOR: novel object recognition, MWM: morris water maze, MDA: malondialdehyde, GSH: glutathione, SOD: superoxide dismutase).
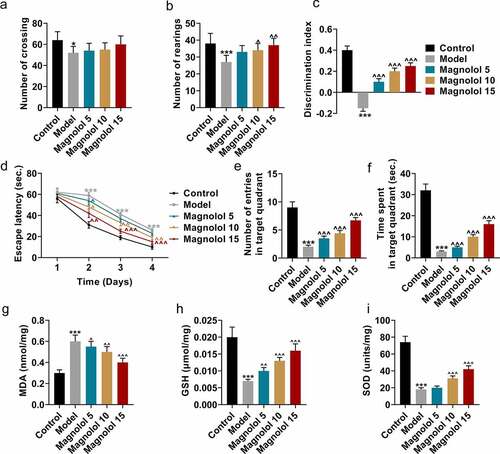
In view of the previous confirmation that the impairment of cognitive function in AD patients with brain insulin resistance was induced by the oxidative damage in the hippocampus [Citation9], the secretions of oxidative-related factors were detected by ELISA (). The results exhibited that in AD mice, the level of MDA () was up-regulated and those of GSH () and SOD () were down-regulated when compared with the Control group (P < 0.001), while Magnolol decreased the level of MDA (P < 0.05) and increased the levels of GSH and SOD as compared to the Model group (P < 0.01).
Magnolol down-regulated the levels of inflammation-related factors and up-regulated the level of miR-200c in AD model mice with brain insulin resistance
In order to explore the effect of Magnolol on inflammatory factors in AD model mice at the molecular biological level, we detected the expression level of miR-200c in magnolol-treated mice. Then the secretions of inflammation-related factors in hippocampus tissues were evaluated by ELISA (). As the data presented, the levels of TNF-α, IL-6, and CRP were obviously up-regulated in AD mice in comparison with the Control group (P < 0.001), while as compared to the Model group, the levels of TNF-α, IL-6, and CRP were down-regulated by 10 mg/kg and 15 mg/kg Magnolol (P < 0.001). Besides, the levels of TNF-α, IL-6, and CRP in hippocampus tissues were also detected by Western blot (), and the results further verified the finding of ELISA. Furthermore, when compared with the Control group, a down-regulated expression of miR-200c (P < 0.001; ) was found in the AD mice, which was restored by 5 mg/kg, 10 mg/kg, and 15 mg/kg Magnolol (P < 0.05) in comparison with the Model group.
Figure 2. Magnolol down-regulated the levels of inflammation-related factors and up-regulated the level of miR-200c in AD model mice with brain insulin resistance. (a-c) The secretions of TNF-α (a), IL-6 (b), and CRP (c) in hippocampus tissues of the mouse were detected by ELISA. (d-e) The expressions of TNF-α, IL-6, and CRP in hippocampus tissues of the mice were detected by Western blot. GAPDH was used as an internal control. (f) The expression of miR-200c in hippocampus tissues of the mouse was detected by RT-qPCR, and U6 was used as an internal control. (***P < 0.001, vs. Control; ^P < 0.05, ^^P < 0.01, ^^^P < 0.001, vs. Model). (AD: Alzheimer’s disease, TNF-α: tumor necrosis factor-α, IL-6: interleukin-6, CRP: C-reactive protein).
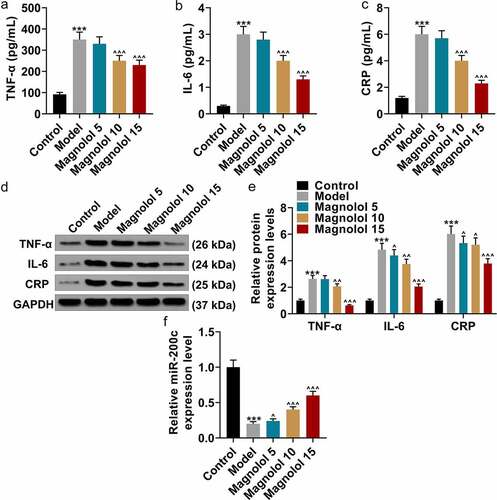
MiR-200c antagomiR offset the effects of Magnolol on locomotor activity and cognitive function and levels of oxidative-related factors in AD model mice with brain insulin resistance
Further, we analyzed the effects of Magnolol on motor activity, cognitive function and the levels of oxygen-related factors in AD model mice by up-regulation of miR-200c. To confirm that the effects of Magnolol in AD mice with brain insulin resistance were mediated by regulating miR-200c, another animal experiment was conducted with miR-200c antagomiR (AntagomiR). As shown in , miR-200c expression was down-regulated by AntagomiR (P < 0.001) and up-regulated by Magnolol (P < 0.001) when compared with the AntagomiR-NC group; however, after co-treatment with AntagomiR and Magnolol (the AntagomiR+Magnolol 10 group and the AntagomiR+ Magnolol 15 group), the effects of AntagomiR and Magnolol on miR-200c expression were offset by each other. In the OFT, the numbers of crossings () and rearings () were down-regulated by AntagomiR (P < 0.001), but these numbers were up-regulated by Magnolol (P < 0.001) when compared with the AntagomiR-NC group. In the AntagomiR+Magnolol 10 group and the AntagomiR+Magnolol 15 group, the numbers of crossings and rearings were increased, and the effects of AntagomiR and Magnolol on the number of rearings were neutralized by each other. In the NOR evaluation (), the discrimination index was down-regulated by AntagomiR (P < 0.001) and up-regulated by Magnolol (P < 0.001) when compared with the AntagomiR-NC group, while in the AntagomiR+Magnolol 10 group and the AntagomiR+ Magnolol 15 group, the effects of AntagomiR and Magnolol on the discrimination index were neutralized by each other. In the MWM test, when compared with the AntagomiR-NC group, AntagomiR increased the escape latency (P < 0.05; ) and decreased the number of entries in the target quadrant (P < 0.001; ) and the time spent in the target quadrant (P < 0.001; ). Magnolol, however, decreased the escape latency (P < 0.05) and increased the number of entries in the target quadrant (P < 0.001) and the time spent in the target quadrant (P < 0.001). Besides, in the AntagomiR+Magnolol 10 group and the AntagomiR+Magnolol 15 group, the effects of AntagomiR and Magnolol on escape latency, the number of entries in the target quadrant and the time spent in the target quadrant were neutralized by each other.
Figure 3. miR-200c antagomiR offset the effects of Magnolol on locomotor activity and cognitive function and levels of oxidative-related factors in AD model mice with brain insulin resistance. (a) The expressions of miR-200c in hippocampus tissues of the mice were quantified by RT-qPCR. U6 was used as an internal control. (b-c) OFT was performed to measure the locomotor ability of the mice. (d) NOR evaluation was employed to measure the discrimination ability of the mice. (e-g) MWM test was conducted to evaluate the spatial learning and memory ability of the mice. (h-j) The secretions of MDA (h), GSH (i), and SOD (j) in hippocampus tissues of the mice were detected by ELISA. (*P < 0.001, **P < 0.001, ***P < 0.001, vs. AntagomiR-NC; ^P < 0.05, ^^^P < 0.001, vs. AntagomiR; #P < 0.05, ##P < 0.01, ###P < 0.001, vs. AntagomiR-NC+ Magnolol 10; &P < 0.05, &&P < 0.01, &&&P < 0.001, vs. AntagomiR-NC+ Magnolol 15). (AD: Alzheimer’s disease, OFT: open-field test, NOR: novel object recognition, MWM: morris water maze, MDA: malondialdehyde, GSH: glutathione, SOD: superoxide dismutase, PCO: protein carbonylation).
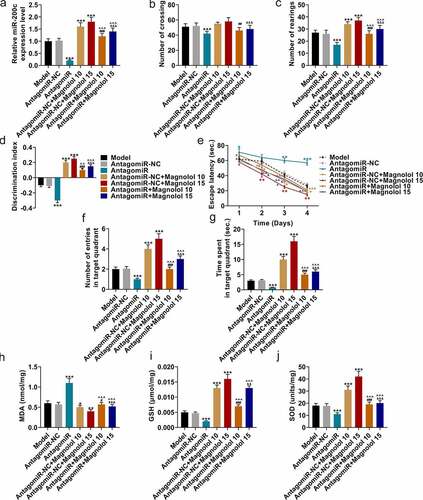
As for the secretions of oxidative-related factors (), when compared with the AntagomiR-NC group, AntagomiR increased the levels of MDA (P < 0.001; ) but decreased those of GSH (P < 0.001, ) and SOD (P < 0.001, ), while Magnolol inhibited the level of MDA (P < 0.05) but raised the levels of GSH (P < 0.001) and SOD (P < 0.001). Meanwhile, in the AntagomiR+Magnolol 10 group and the AntagomiR+Magnolol 15 group, the effects of AntagomiR and Magnolol on the levels of these oxidative-related factors were neutralized by each other. All the evidence suggested that the effect of Magnolol on AD mice with brain insulin resistance was mediated by regulating miR-200c.
MiR-200c antagomiR offset the effect of Magnolol on the levels of inflammation-related factors in AD model mice with brain insulin resistance
Meanwhile, we analyzed the effect of Magnolol on the levels of inflammation-related factors in AD model mice by upregulation of miR-200c. The levels of inflammation-related factors in hippocampus tissues were finally detected using both ELISA () and Western blot (). The levels of TNF-α, IL-6, and CRP were up-regulated by AntagomiR (P < 0.05) and down-regulated by Magnolol (P < 0.001) when compared with the AntagomiR-NC group, while the effects of AntagomiR and Magnolol on the levels of these factors were neutralized by each other after co-treatment with AntagomiR and Magnolol (the AntagomiR+ Magnolol 10 group and the AntagomiR+Magnolol 15 group). This discovery indicated that miR-200c antagomir offset the effect of Magnolol on the expressions of TNF-α, IL-6, and CRP in AD model mice with brain insulin resistance, which further revealed that the effect of Magnolol in AD mice with brain insulin resistance was mediated by regulating miR-200c.
Figure 4. miR-200c antagomiR offset the effect of Magnolol on the levels of inflammation-related factors in AD model mice with brain insulin resistance. (a-c) The secretions of TNF-α (a), IL-6 (b), and CRP (c) in hippocampus tissues of the mice were detected by ELISA. (d-e) The expressions of TNF-α, IL-6, and CRP in hippocampus tissues of the mice were detected by Western blot. GAPDH was used as an internal control. (*P < 0.001, ***P < 0.001, vs. AntagomiR-NC; ^^P < 0.05, ^^^P < 0.001, vs. AntagomiR; ##P < 0.01, ###P < 0.001, vs. AntagomiR-NC+ Magnolol 10; &&&P < 0.001, vs. AntagomiR-NC+ Magnolol 15). (AD: Alzheimer’s disease, TNF-α: tumor necrosis factor-α, IL-6: interleukin-6, CRP: C-reactive protein).
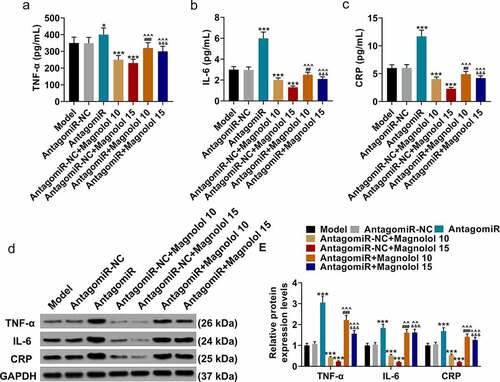
Discussion
In this study, streptozotocin (STZ) was firstly intracerebroventricularly injected into the mouse to mimic the pathological and behavioral alterations in sporadic AD patients with brain insulin resistance. The major symptoms of patients suffering from AD with brain insulin resistance are memory loss, cognitive decline, and locomotor impairment [Citation3–5], and in our study, the STZ-induced mice also exhibited impaired locomotor and cognitive capacities, which indicated that the brain insulin resistance AD mice model induced by STA was successfully established.
Magnolol, one of the important active ingredients from Magnolia Officinalis, possesses plentiful biological activities including anti-cancer, anti-inflammation, and anti-oxidation [Citation20–22]. A recent study has proved that Magnolol can attenuate inflammation in the formalin-induced inflammatory pain mice while causing no deteriorating effect on their locomotor and cognitive abilities [Citation9]. In this study, we observed the effects of Magnolol on the behavior of mouse with intracranial insulin resistance, and found that through the escape latency, the number of times to enter the target area and the time in the target area, Magnolol could attenuate locomotor impairment and cognitive deficit for the first time, although the specific effect and mechanism still needs more exploration.
In terms of molecular studies, it has been found that NEAT1 induces Mir-124-3p to inhibit sh-SY5Y cytotoxicity and reduce cellular inflammatory response, indicating that miRNA has a certain regulatory role in the development of AD [Citation34]. Since miR-200c was previously proved to possess a defensive effect against Aβ peptide-induced toxicity in an AD mice model [Citation26] and Magnolol achieves its anti-cancer effect via up-regulating the expression of miR-200c in cancer cells [Citation27], we speculated that the effect of Magnolol on AD mice with brain insulin resistance is also associated with miR-200c. Here, we further discovered that Magnolol enhanced the level of miR-200c in the hippocampus tissues of AD mice with brain insulin resistance, and miR-200c antagomiR not only exerted opposite effects but also offset the effect of Magnolol on the locomotor activity and cognitive function of AD mice with brain insulin resistance, which led us to the conclusion that the effects of Magnolol on AD mice with brain insulin resistance are also mediated by up-regulating miR-200c.
Changes in behavioral disorders and cognitive impairment are induced by brain oxidative stress damage in the synapses and hippocampus [Citation35–37]. The process of oxidative damage causes a range of intermediates and end products including MDA [Citation38,Citation39]. Besides, during oxidative damage, the levels and activities of antioxidant enzymes such as SOD and GSH were suppressed in AD [Citation39]. To enhance the reliability of the logic of our research, we then detected the levels of oxidative-related factors in the hippocampus tissues of AD mice with brain insulin resistance. We discovered that the up-regulated MDA in AD mice with brain insulin resistance were decreased by Magnolol and increased by miR-200c antagomiR, whereas the down-regulated SOD and GSH were increased by Magnolol and decreased by miR-200c antagomiR, in addition to the discovery that the effects of miR-200c antagomiR and Magnolol were offset by each other. These results indicated that Magnolol mitigated oxidative stress in AD mice with brain insulin resistance by regulating miR-200c.
In addition, neuronal dysfunction is the consequence of reactive oxygen species generation during oxidative stress damage [Citation9]. Neuroinflammation is the key feature of neuronal dysfunction and neurodegeneration in the STZ-induced AD model [Citation9,Citation40]. STZ results in the release of pro-inflammatory cytokines such as TNF-α, IL-6, and CRP by activating the microglia, and finally contributes to neuroinflammation [Citation6,Citation40,Citation41]. It was exhibited in our study that the up-regulated TNF-α, IL-6, and CRP in the hippocampus tissues of AD mice with brain insulin resistance were decreased by Magnolol and increased by miR-200c antagomiR. Likewise, the effects of miR-200c antagomiR and Magnolol were offset by each other. This evidence revealed that Magnolol attenuated neuroinflammation in AD mice with brain insulin resistance by regulating miR-200c.
Conclusion
In conclusion, our research demonstrated that Magnolol can alleviate the cognitive impairment, neuroinflammation and other symptoms of AD mice by up-regulating the expression of miR-200c.
Limitation
However, we should measure the brain insulin level in the AD mice for exploring the effects of Magnolol on the expression of miR- 200c in AD mice.
Future strategy
Therefore, in the next study, we will further examine the effect of Magnolol on the brain insulin level of mice.
Highlights
Magnolol attenuated the locomotor impairment of Alzheimer’s disease mice.
Magnolol attenuated cognitive deficit and neuroinflammatory of Alzheimer’s disease mice.
Magnolol up-regulating miR-200c in Alzheimer’s disease mice.
Availability of data and materials
The analyzed data sets generated during the study are available from the corresponding author on reasonable request.
Disclosure statement
No potential conflict of interest was reported by the author(s).
Additional information
Funding
References
- Sousa JCE, Santana ACF, Magalhães GJP. Resveratrol in Alzheimer’s disease: a review of pathophysiology and therapeutic potential. Arq Neuropsiquiatr. 2020. DOI:10.1590/0004-282x20200010
- Soria Lopez JA, González HM, Léger GC. Alzheimer’s disease. Handb Clin Neurol. 2019;167:231–255.
- Bao X, Liu G, Jiang Y, et al. Cell adhesion molecule pathway genes are regulated by cis-regulatory SNPs and show significantly altered expression in Alzheimer’s disease brains. Neurobiol Aging. 2015;36:2904.e1–7.
- Heneka MT, Carson MJ, El Khoury J, et al. Neuroinflammation in Alzheimer’s disease. Lancet Neurol. 2015;14:388–405.
- Verkhratsky A, Parpura V, Rodriguez-Arellano JJ, et al. Astroglia in Alzheimer’s disease. Adv Exp Med Biol. 2019;1175:273–324.
- Hansen DV, Hanson JE, Sheng M. Microglia in Alzheimer’s disease. J Cell Biol. 2018;217:459–472.
- Steen E, Terry BM, Rivera EJ, et al. Impaired insulin and insulin-like growth factor expression and signaling mechanisms in Alzheimer’s disease–is this type 3 diabetes? J Alzheimers Dis. 2005;7:63–80.
- de la Monte SM. Brain insulin resistance and deficiency as therapeutic targets in Alzheimer’s disease. Curr Alzheimer Res. 2012;9:35–66.
- Akhtar A, Dhaliwal J, Saroj P, et al. Chromium picolinate attenuates cognitive deficit in ICV-STZ rat paradigm of sporadic Alzheimer’s-like dementia via targeting neuroinflammatory and IRS-1/PI3K/AKT/GSK-3β pathway. Inflammopharmacology. 2020;28:385–400.
- Dey A, Bhattacharya R, Mukherjee A, et al. Natural products against Alzheimer’s disease: pharmaco-therapeutics and biotechnological interventions. Biotechnol Adv. 2017;35:178–216.
- Zhu Y, Peng L, Hu J, et al. Current anti-Alzheimer’s disease effect of natural products and their principal targets. J Integr Neurosci. 2019;18:327–339.
- Jha AB, Panchal SS, Shah A. Ellagic acid: insights into its neuroprotective and cognitive enhancement effects in sporadic Alzheimer’s disease. Pharmacol Biochem Behav. 2018;175:33–46.
- Ajayi AM. Chrysophyllum albidum (African star apple) fruit-supplemented diet enhances cognitive functions and attenuates lipopolysaccharide-induced memory impairment, oxidative stress, and release of proinflammatory cytokines. Nutrire. 2020;45:10–13.
- Ben-Azu B, Nwoke EE, Aderibigbe AO, et al. Possible neuroprotective mechanisms of action involved in the neurobehavioral property of naringin in mice. Biomed Pharmacothe. 2019;109:536–546.
- Wang H, Wang H, Cheng H, et al. Ameliorating effect of luteolin on memory impairment in an Alzheimer’s disease model. Mol Med Rep. 2016;13:4215–4220.
- Maione F, Piccolo M, De Vita S, et al. Down regulation of pro-inflammatory pathways by tanshinone IIA and cryptotanshinone in a non-genetic mouse model of Alzheimer’s disease. Pharmacol Res. 2018;129:482–490.
- Zhang J, Chen Z, Huang X, et al. Insights on the multifunctional activities of magnolol. Biomed Res Int. 2019;2019:1847130.
- Gao T, Xu H, Jia S, et al. Magnolol induces human Ewing sarcoma SK-ES-1 cell apoptosis via the mitochondrial and death receptor pathways. Am J Transl Res. 2020;12:1672–1682.
- Sarrica A, Kirika N, Romeo M, et al. Safety and toxicology of magnolol and honokiol. Planta Med. 2018;84:1151–1164.
- Chen H, Fu W, Chen H, et al. Magnolol attenuates the inflammation and enhances phagocytosis through the activation of MAPK, NF-κB signal pathways in vitro and in vivo. Mol Immunol. 2019;105:96–106.
- Parray HA, Lone J, Park JP, et al. Magnolol promotes thermogenesis and attenuates oxidative stress in 3T3-L1 adipocytes. Nutrition. 2018;50:82–90.
- Zhou S, Wen H, Li H. Magnolol induces apoptosis in osteosarcoma cells via G0/G1 phase arrest and p53-mediated mitochondrial pathway. J Cell Biochem. 2019;120:17067–17079.
- Chen Y, Huang K, Ding X, et al. Magnolol inhibits growth and induces apoptosis in esophagus cancer KYSE-150 cell lines via the MAP kinase pathway. J Thorac Dis. 2019;11:3030–3038.
- Chei S, Oh HJ, Song JH, et al. Magnolol suppresses TGF-β-induced epithelial-to-mesenchymal transition in human colorectal cancer cells. Front Oncol. 2019;9:752.
- Cheng J, Dong S, Yi L, et al. Magnolol abrogates chronic mild stress-induced depressive-like behaviors by inhibiting neuroinflammation and oxidative stress in the prefrontal cortex of mice. Int Immunopharmacol. 2018;59:61–67.
- Higaki S, Muramatsu M, Matsuda A, et al. Defensive effect of microRNA-200b/c against amyloid-beta peptide-induced toxicity in Alzheimer’s disease models. PLoS One. 2018;13:e0196929.
- Hagiwara K, Gailhouste L, Yasukawa K, et al. A robust screening method for dietary agents that activate tumour-suppressor microRNAs. Sci Rep. 2015;5:14697.
- Nassar SZ, Badae NM, Issa YA. Effect of amylin on memory and central insulin resistance in a rat model of Alzheimer’s disease. Arch Physiol Biochem. 2020;126:326–334.
- Lin YR, Chen HH, Ko CH, et al. Effects of honokiol and magnolol on acute and inflammatory pain models in mice. Life Sci. 2007;81:1071–1078.
- Ding B, Lin C, Liu Q, et al. Tanshinone IIA attenuates neuroinflammation via inhibiting RAGE/NF-kappaB signaling pathway in vivo and in vitro. J Neuroinflammation. 2020;17:302.
- Yang C, Xia W, Liu X, et al. Role of TXNIP/NLRP3 in sepsis-induced myocardial dysfunction. Int J Mol Med. 2019;44:417–426.
- Papadaki C, Monastirioti A, and Rounis K, et al. Circulating MicroRNAs regulating DNA damage response and responsiveness to cisplatin in the prognosis of patients with non-small cell lung cancer treated with first-line platinum chemotherapy. Cancers (Basel). 2020;12:1282.
- Taylor SC, Posch A. The design of a quantitative Western blot experiment. Biomed Res Int. 2014;2014:361590.
- Chen MY, Fan K, Zhao LJ, et al. Long non-coding RNA nuclear enriched abundant transcript 1 (NEAT1) sponges microRNA-124-3p to up-regulate phosphodiesterase 4B (PDE4B) to accelerate the progression of Parkinson’s disease. Bioengineered. 2021;12:708–719.
- Canever L, Freire TG, Mastella GA, et al. Changes in behavioural parameters, oxidative stress and neurotrophins in the brain of adult offspring induced to an animal model of schizophrenia: the effects of FA deficient or FA supplemented diet during the neurodevelopmental phase. Prog Neuropsychopharmacol Biol Psychiatry. 2018;86:52–64.
- Fukui K, Omoi NO, Hayasaka T, et al. Cognitive impairment of rats caused by oxidative stress and aging, and its prevention by vitamin E. Ann NY Acad Sci. 2002;959:275–284.
- Li Q, Song W, Tian Z, et al. Aminoguanidine alleviated MMA-induced impairment of cognitive ability in rats by downregulating oxidative stress and inflammatory reaction. Neurotoxicology. 2017;59:121–130.
- López-Riquelme N, Alom-Poveda J, Viciano-Morote N, et al. Apolipoprotein E ε4 allele and malondialdehyde level are independent risk factors for Alzheimer’s disease. SAGE Open Med. 2016;4:2050312115626731.
- Zabel M, Nackenoff A, Kirsch WM, et al. Markers of oxidative damage to lipids, nucleic acids and proteins and antioxidant enzymes activities in Alzheimer’s disease brain: a meta-analysis in human pathological specimens. Free Radic Biol Med. 2018;115:351–360.
- Luo H, Xiang Y, Qu X, et al. Apelin-13 suppresses neuroinflammation against cognitive deficit in a streptozotocin-induced rat model of alzheimer’s disease through activation of BDNF-TrkB signaling pathway. Front Pharmacol. 2019;10:395.
- Ng A, Tam WW, Zhang MW, et al. IL-1β, IL-6, TNF- α and CRP in elderly patients with depression or Alzheimer’s disease: systematic review and meta-analysis. Sci Rep. 2018;8:12050.