ABSTRACT
Osteoporosis (OP) is a systemic bone metabolic disease with complicated pathogenesis and is difficult to cure clinically. The regulatory mechanisms of OP are needed to be further investigated. In the present study, we focused on the role of myocardial infarction-associated transcript (MIAT) in OP development and examined the underlying mechanism. The serum expression levels of MIAT in samples from patients with OP and healthy controls were compared using quantitative reverse transcription-PCR (qRT-PCR). The dual-luciferase reporter assay was used to confirm the relationship between MIAT and its potential target microRNA, i.e., miR-150-5p. Moreover, bone marrow-derived mesenchymal stem cells (BMSCs) were cultured and transfected with MIAT shRNA, with or without miR-150-5p inhibitor. EdU staining and colony formation analysis were performed to determine the proliferation ability of these cells. Furthermore, the TUNEL assay and flow cytometry were used to assess BMSC apoptosis. Finally, RT-PCR and Western blot assays were employed to assess the expression of osteogenic differentiation biomarkers. Compared with controls, the expression of MIAT was significantly increased, whereas that of miR-150-5p was markedly decreased in patients with OP. MIAT and miR-150-5p expression levels exhibited a strong negative correlation. Furthermore, osteogenic differentiation indicators were suppressed in serum of OP patients. MIAT was downregulated, and miR-150-5p was upregulated in induced to osteogenic differentiation BMSCs. Furthermore, downregulation of MIAT dramatically promoted osteogenic differentiation, increased proliferation, and inhibited apoptosis in BMSCs; miR-150-5p inhibitor abrogated the effects of MIAT. In conclusion, lncRNA MIAT can regulate the proliferation, apoptosis, and osteogenic differentiation of BMSCs.
KEYWORDS:
1. Introduction
As the most prevalent progressive bone disease, osteoporosis (OP) dramatically limits patient activity and greatly increases the medical burden [Citation1]. OP is characterized by low bone mass, potentially resulting in bone rarefaction and fractures [Citation2]. It is well known that bone is a continuously and dynamically modified tissue, predominantly consisting of osteoblasts and osteoclasts. Osteoblasts promote bone formation, whereas osteoclasts can induce bone resorption [Citation3]. The imbalance between osteoblasts and osteoclasts has been indicated as the primary factor resulting in OP and other bone metabolic processes [Citation4]. Bone marrow-derived mesenchymal stem cells (BMSCs), a known source of osteoblasts, maintain a balance between bone formation and bone resorption [Citation5,Citation6]. Therefore, an in-depth investigation into the roles of BMSCs in OP is critical for early diagnosis, as well as disease management [Citation7].
Myocardial infarction-associated transcript (MIAT), a long non-coding RNA (lncRNA), reportedly plays a key role in the development of myocardial infarction [Citation8]. Recent studies have shown that MIAT is abnormally expressed in microvascular dysfunction [Citation9], paranoid schizophrenia [Citation10], and cancer [Citation11]. In contrast, MIAT was found to be downregulated in adipose tissue-derived stem cells (ASCs) undergoing osteogenic differentiation [Citation12]. For instance, MIAT knockdown obviously ameliorated diabetes mellitus-induced retinal microvascular dysfunction by regulating miR-150-5p [Citation9]. MIAT contributed to non-small cell lung cancer proliferation and metastasis through MMP9 activation [Citation11]. Moreover, MIAT silencing can induce the osteogenic process of mesenchymal stem cells (MSCs) [Citation13,Citation14]. Nevertheless, the role of MIAT in OP warrants further investigation.
LncRNAs are reportedly involved in exerting biological functions [Citation15]. Osteoblast differentiation and osteogenesis are regulated by different miRNAs [Citation16]. MIAT targets distinct miRNAs in different diseases. For instance, MIAT, which time-dependently increases during BMSC differentiation, induces BMSC differentiation into endothelial cells by targeting miR-200a [Citation17]. Moreover, MIAT sponges miR-150-5p to regulate oxidative stress and modulate the function of human lens epithelial cells [Citation18]. In contrast, miR-150 induces osteoblast differentiation [Citation19] and inhibits osteoclast growth [Citation20].
Accordingly, in the present study, we focused on the roles of MIAT and miR-150-5p in OP. Interaction between MIAT and miR-150-5p, and their effects on osteoblast differentiation of BMSCs were investigated, which may provide a novel treatment idea for OP.
2. Materials and Methods
2.1 Patients
Herein, we enrolled 30 healthy volunteers and 30 patients with OP, and took blood samples and isolated serum samples. Patients with OP presenting with hip fractures requiring surgical intervention, a history of cardiovascular diseases, cancers, diabetes, or other metabolic problems were excluded. Each participant provided informed consent before study initiation. The study protocol was approved by the Ethics Committee of the Liaohe Hospital.
2.2 Cell culture
HEK 293 T cells (ATCC, Manassas, VA) were cultured using Dulbecco’s Modified Eagle Medium (DMEM; Thermo Fisher Scientific, Inc., Waltham, MA, USA), supplemented with 10% fetal bovine serum (FBS; Gibco; Thermo Fisher Scientific, Inc.) and 1% penicillin/streptomycin (Invitrogen) [Citation21]. The cells were maintained at 95% humidity and 5% CO2 at 37°C.
2.3 Isolation and culture of BMSCs
Gradient centrifugation was performed to isolate MSCs from the bone marrow of rats. In brief, four male Sprague-Dawley rats were housed under standard conditions and allowed to access food and water ad libitum. Bone marrow aspirates were placed in MEM medium (Gibco), supplemented with 10% FBS for 24 h at 95% humidity and 5% CO2 at 37°C [Citation22]. Suspended cells were removed, and then cells were cultured for approximately 10 days. On reaching ~75% confluency, cells were harvested for subsequent experiments.
2.4 shRNA transfection
MIAT (shMIAT-1 5ʹ-GGTCAGGATTAGTGGTCATTC-3ʹ and shMIAT-2 5ʹ-GGTGATTACCGTGCACCTTGA-3ʹ) and the negative control (NC; TTCTCCGAACGTGTCACGTTTC) were purchased from GenePharma Co. (Suzhou, China). shRNA transfection was performed using Lipofectamine 2000 (Invitrogen) [Citation23].
2.5 Dual-luciferase reporter assay
The binding sites between miR-150 and MIAT were predicted using StarBase 3.0. To verify that MIAT targeted miR-150, HEK 293 T cells (2 × 104 cells/mL, 200 μL) were plated into 48-well plates. Then, cells were treated with 400 ng wild-type (WT) MIAT or mutant (MUT) MIAT 3ʹ-UTR. After incubation for 6 h, HEK 293 T cells were cultured in DMEM for an additional 48 h. Luciferase activity was determined using a commercial kit (Promega Corporation) [Citation24].
2.6 5-Ethynyl-2ʹ-deoxyuridine staining (EdU)
EdU was used to examine BMSC proliferation [Citation25]. Briefly, BMSCs were incubated with EdU (50 μM) for 2 h. Then, BMSCs were obtained and stained using a kit (Ribobio). Finally, images were visualized and photographed using a microscope (Olympus).
2.7 Colony formation assay
In brief, BMSCs were seeded into 6-well plates and cultured for 7 days, with the culture medium replaced every 2 days. Thereafter, BMSCs were stained with crystal violet (0.1%) for 10 min [Citation26]. Colonies were visualized and counted using a microscope (Nikon, Tokyo, Japan).
2.8 Alkaline phosphatase (ALP) assessment
The cells were fixed with 4% paraformaldehyde (PFA) for 10 min at room temperature. Then, cells were seeded and stained with ALP for 20 min [Citation27]. The images were captured using a scanner. An ALP Assay Kit was used to detect ALP activity in accordance with the manufacturer’s protocol.
2.9 Cell apoptosis
Cells were stained with the propidium iodide (PI)/Annexin V-FITC kit (Sigma) in the dark for 10 min at room temperature [Citation28]. Then, the cells were counted by flow cytometry (BD Biosciences).
2.10 Terminal-deoxynucleotidyl Transferase Mediated Nick End Labeling (TUNEL) staining
TUNEL staining was used to assess BMSC death [Citation28]. Briefly, BMSCs were fixed and treated with a TUNEL staining kit (Beyotime). Then, images from six randomly selected fields were captured using a microscope (Olympus).
2.11 Reverse transcription-polymerase chain reaction (qRT-PCR)
RNA samples were extracted from collected serum and BMSCs using a commercially available kit (Takara, Japan). cDNA was synthesized, and PCR was performed using a Real-Time PCR Detection System (Bio-Rad, USA). The relative levels of MIAT, ALP, osteocalcin (OCN), and RUNX2 were normalized to GAPDH. miR-150-5p expression was standardized to U6. The sequences of the primers were as follows: Human MIAT forward: 5ʹ-CATGGCCTCCGTAGTAACTCAC-3ʹ, reverse: 5ʹ-TCAAACCCCAGCCACTCTTC-3ʹ; Human GAPDH forward:5ʹ- CATCATCCCTGCCTCTACTGG-3ʹ, reverse 5ʹ- GTGGGTGTCGCTGTTGAAGTC-3ʹ; Rat OCN forward: 5ʹ- CCAGCGACTCTGAGTCTGACAA-3ʹ, reverse: 5ʹ-AACGGTGGTGCCATAGATGC-3ʹ; Rat ALP forward: 5ʹ-TGGACCTCATCAGCATTTGG-3ʹ, reverse: 5ʹ- GAGGGAAGGGTCAGTCAGGTT-3ʹ; Rat RUNX2 forward: 5ʹ-TACTCTGCCGAGCTACGAAATG-3ʹ, reverse: 5ʹ-TGAAACTCTTGCCTCGTCCG-3ʹ; Rat MIAT forward: 5ʹ-GCGAGCAATCTGAAGATCCTG-3ʹ, reverse: 5ʹ-GCTCTACCCCATCTCCAGAGAC-3ʹ; Rat GAPDH forward: 5ʹ-CGCTAACATCAAATGGGGTG-3ʹ, reverse: 5ʹ-TTGCTGACAATCTTGAGGGAG-3ʹ; hsa-miR-150-5p forward: 5ʹ- TCCCAACCCTTGTACCAGTG-3ʹ, reverse 5ʹ- CTCAACTGGTGTCGTGGAGTC-3ʹ; Human U6 forward: 5ʹ- CTCGCTTCGGCAGCACAT-3ʹ, reverse: 5ʹ- AACGCTTCACGAATTTGCGT-3ʹ; rno-miR-150-5p forward: 5ʹ- TCCCAACCCTTGTACCAGTG-3ʹ, reverse: 5ʹ- CTCAACTGGTGTCGTGGAGTC-3ʹ; Rat U6 forward: 5ʹ- CCTGCTTCGGCAGCACAT-3ʹ, reverse: 5ʹ- AACGCTTCACGAATTTGCGT-3ʹ.
2.12 Western blotting
Protein extracts (15 μg/lane) were subjected to 10% sodium dodecyl sulfate gel electrophoresis. The protein extracts were then transferred to a polyvinylidene fluoride membrane (Millipore) and incubated with primary antibodies at 4°C overnight. On day 2, the membrane was incubated with secondary antibodies for 2 h at room temperature. Protein bands were visualized using an ECL Western blot Kit (CWBIO). Each protein level was normalized to that of GAPDH.
2.13 Statistical analysis
Statistical analyses were performed using GraphPad Prism 7.0 (GraphPad Software, La Jolla, CA, USA). Data are expressed as mean ± standard deviation (SD). Comparison of two groups was performed using Student’s t-tests. Comparison of more than two groups was performed using analysis of variance. Statistical significance was set at P < 0.05.
3. Results
3.1 MIAT and miR-50-5p were aberrant expressed in patients with OP
Based on the qRT-PCR analysis, we observed that MIAT expression was increased (, p < 0.01), while miR-150-5p was decreased (, p < 0.01) in serum samples from patients with OP. Additionally, correlation analysis revealed that MIAT and miR-150-5p expression levels were negatively correlated (, r = - −0.4941, p = 0.0055). Furthermore, osteogenic differentiation-related proteins including Runx2, ALP, and OCN were dramatically lower in serum of patients with OP than those in healthy control group (, p < 0.001).
Figure 1. Expression level of MIAT and miR-150-5p in serum samples of OP patients and healthy controls. MIAT was increased (a) and miR-150-5p was decreased (b) in the serum from patients with postmenopausal OP compared with healthy volunteers. A significant negative correlation was found between the levels of MIAT and miR-150-5p in serum of the OP patients (c). Osteogenic differentiation indicators RUNX2, ALP and OCN were evaluated in the serum from patients with postmenopausal OP compared with healthy volunteers (d-f). **p < 0.01, ***p < 0.001.
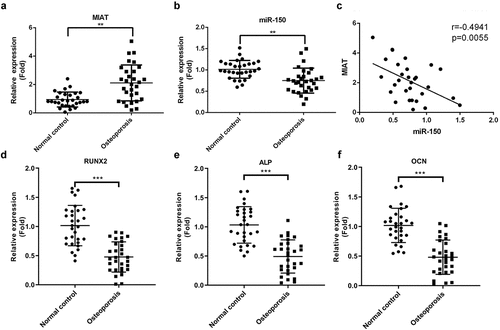
3.2 MIAT targets miR-150-5p
Using bioinformatics tools, MIAT was predicted to target miR-150-5p (). As shown in , luciferase reporter analysis revealed that miR-150-5p inhibitor markedly upregulated luciferase activity in the WT-MIAT group while demonstrating no significant effect in the MUT-MIAT group (, p < 0.05).
3.3 MIAT is downregulated during osteogenic differentiation of BMSCs
The expression level of MIAT in BMSCs was determined by qRT-PCR. As shown in , osteogenic differentiation of BMSCs was confirmed by increased levels of osteogenic markers (). On the contrary, the expression of MIAT was decreased (), while miR-150-5p was increased during osteogenic differentiation in a time-dependent manner ().
Figure 3. MIAT is significantly downregulated during the osteogenic differentiation of BMSCs. Osteogenic differentiation of BMSCs was evidenced by the increased expression of RUNX2, ALP and OCN (a-c). MIAT was decreased and miR-150-5p was increased time-dependently during the osteogenic differentiation of BMSCs (c and d). *p < 0.05, **p < 0.01, ***p < 0.001.
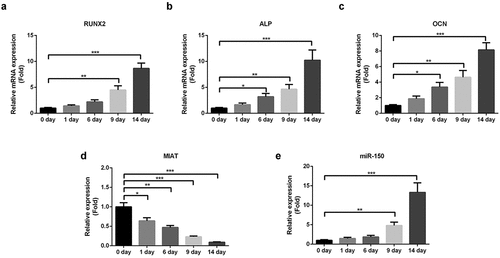
3.4 Transfection of shMIAT and miR-150-5p inhibitor in BMSCs
Compared with the shNC group, shMIAT-1 and shMIAT-2 markedly decreased the expression level of MIAT in BMSCs, especially in shMIAT-1 treated cells (, p < 0.01). Therefore, shMIAT-1 was used in the present study. In contrast, the miR-150-5p inhibitor dramatically downregulated miR-150-5p expression in BMSCs (, p < 0.01).
3.5 MIAT promotes the proliferation of BMSCs by targeting miR-150-5p
Based on EdU staining () and the colony formation assay (), shMIAT infection significantly enhanced BMSC proliferation in the shMIAT group when compared with the shNC group; the miR-150-5p inhibitor partially inhibited the proliferative behaviors of shMIAT.
Figure 5. MIAT can regulate the cell proliferation of BMSCs by targeting miR-150-5p. EdU staining (a and b) and colony formation (c and d) showed that compared with shNC group, the proliferation of BMSCs was significantly increased in shMIAT group, which was reversed by miR-150-5p inhibitor. *p < 0.05, **p < 0.01, ***p < 0.001. Note: The petri dish is 35 mm in diameter. The scale length is 50 μm.
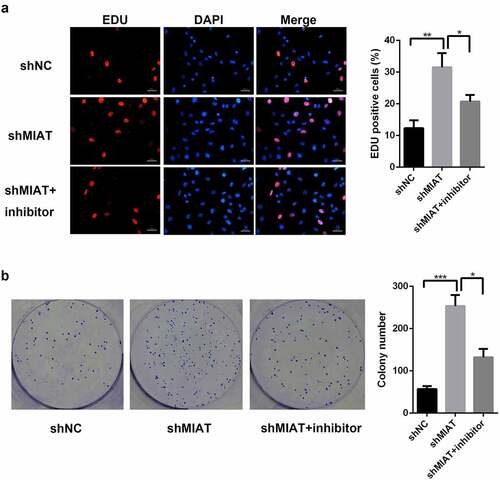
3.6 MIAT inhibits the apoptosis of BMSCs by targeting miR-150-5p
TUNEL staining () and flow cytometry () showed that apoptosis of BMSCs was markedly decreased in the shMIAT group. Notably, the miR-150-5p inhibitor partially blocked the anti-apoptotic effects of shMIAT.
Figure 6. MIAT can regulate the apoptosis of BMSCs by targeting miR-150-5p. TUNEL staining (a and b) and flow cytometry (c and d) showed that compared with shNC group, the cell apoptosis of BMSCs was significantly decreased in shMIAT group, which was reversed by miR-150-5p inhibitor. *p < 0.05, **p < 0.01, ***p < 0.001. Note: The scale length is 50 μm.
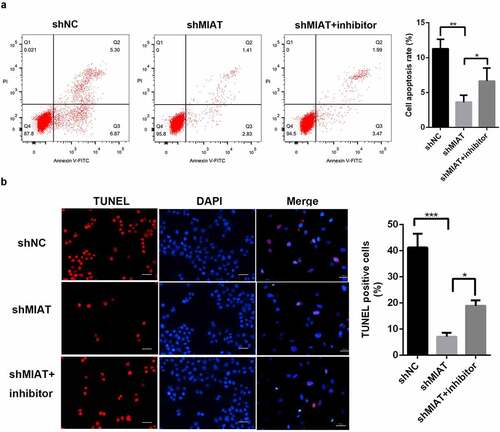
3.7 MIAT may regulate osteogenic differentiation of BMSCs by targeting miR-150-5p
ALP staining and ALP activity assay were conducted to evaluate osteogenic differentiation of BMSCs. Based on ALP staining and ALP activity assay, ALP activity was significantly increased in the shMIAT group when compared with the shNC group. Moreover, transfection of miR-150-5p inhibitor abrogated the MIAT-induced effects on ALP activity (). Finally, the role of MIAT in the expression of osteogenic markers was evaluated by RT-PCR and Western blotting. Compared with shNC, shMIAT significantly increased the BMSC expression of osteogenic markers, RUNX2 and ALP (mRNA expression () and protein expression () at day 14, and the osteogenic effects of MIAT were partially abrogated by transfection with miR-150-5p.
Figure 7. MIAT can regulate the osteogenic differentiation of BMSCs by targeting miR-150-5p. Compared with shNC group, the ALP activity was significantly increased in shMIAT group, which was reversed by miR-150-5p inhibitor (a and b). Compared to shNC, shMIAT significantly increased the mRNA expression of RUNX2 and ALP (c), which were reversed by miR-150-5p inhibitor. MIAT knockdown significantly promoted the protein expression of RUNX2 and ALP by targeting miR-150-5p (d). *p < 0.05, **p < 0.01, ***p < 0.001.
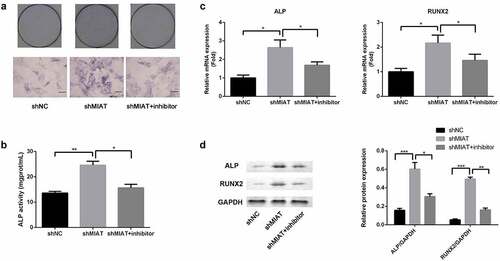
4. Discussion
In the present study, we reported that lncRNA MIAT was significantly increased in patients with OP and could affect the growth, cell death, and osteogenic differentiation of BMSCs by regulating miR-150-5p expression.
It is well-established that BMSCs differentiate into osteoblasts [Citation29]. Therefore, the induction of BMSC proliferation and osteogenic differentiation is crucial for preventing and treating OP [Citation20]. Notably, lncRNA MIAT is a novel regulator of multiple human disorders [Citation30–32]. For example, MIAT is involved in myocardial infarction [Citation33] and microvascular dysfunction [Citation34]. In addition, MIAT can impact the osteogenic differentiation of human ASCs [Citation12]. The silencing of MIAT reportedly promotes the osteogenesis of MSCs [Citation14]. Moreover, MIAT sponges miR-150-5p in human lens epithelial cells [Citation18]. Meanwhile, miR-150 induces osteoblast differentiation [Citation19] and suppresses osteoclast differentiation [Citation20]. However, it remains unclear whether MIAT regulates miR-150 in BMSCs to participate in the pathogenesis of OP.
Herein, we observed that MIAT targeted miR-150-5p, which is in line with previous study [Citation18]. In addition, the expression of MIAT was increased, while that of miR-150-5p was decreased in patients with OP. Osteogenic differentiation indicators were also increased in serum of patients with OP. Furthermore, the expression of MIAT was decreased, while miR-150-5p was increased in BMSCs during osteogenic differentiation in a time-dependent manner. Thence, we speculated that MIAT and miR-150-5p may participate in osteogenic differentiation of OP.
Runx2 is an osteogenic marker related to osteoblast activation during osteogenesis [Citation35], and ALP is a marker for early osteogenic differentiation of BMSCs and plays a role in the in vitro calcification of BMSCs [Citation36]. In present study, MIAT was decreased and miR-150-5p was increased time-dependently during the osteogenic differentiation of BMSCs. Furthermore, MIAT knockdown significantly promoted osteogenic differentiation of BMSCs. Interestingly, co-transfection of miR-150-5p abrogated the osteogenic ability of MIAT shRNA. Therefore, our findings revealed that MIAT might affect the osteogenic differentiation of BMSCs by targeting miR-150-5p.
Abnormal bone reconstruction [Citation37,Citation38] is another important factor in the development of OP. In the present study, MIAT knockdown significantly increased BMSC growth and decreased apoptosis, while miR-150-5p partially blocked the proliferative and anti-apoptotic effects of MIAT shRNA. Collectively, our results indicate that MIAT can affect the growth and apoptosis of BMSCs by targeting miR-150-5p.
There are some limitations in this study. Firstly, the clinical samples were not ethnically diverse, and only Han was included. In future experiments, the sample size should be further expanded and the number of ethnic groups should be increased to verify the results of this study. Secondly, whether MIAT could regulate OP process in vivo by binding with miR-150 should be further investigated. Finally, the downstream gene of miR-150 that affect osteogenic differentiation of BMSCs should be studied.
Conclusions
In summary, MIAT was downregulated while miR-150-5p was upregulated in a time-dependent manner during the osteogenic differentiation of BMSCs. Furthermore, MIAT knockdown significantly increased ALP activity, proliferation, osteogenic differentiation, and osteogenesis and inhibited apoptosis in BMSCs by targeting miR-150-5p. These results suggest the potential role of MIAT in the treatment of OP.
Research Highlights
Compared with controls, the expression of lncRNA MIAT is significantly increased in patients with osteoporosis, whereas that of miR-150-5p is markedly decreased.
LncRNA MIAT can bind with miR-150-5p, and the expression levels of each other exhibited a strong negative correlation.
Downregulation of lncRNA MIAT dramatically promotes osteogenic differentiation, increases proliferation, and inhibits apoptosis in BMSCs by sponging miR-150-5p
Authors’ contributions
Fei Wang performed most of the experiments and wrote part of the manuscript, Huimin Deng and Jimin Chen performed some of the experiments, Zhaobin Wang performed the statistical analysis, Ruofeng Yin designed the studied, wrote and revised most parts of the manuscript.
Data Availability Statement
All data generated or analyzed during this study are available from the corresponding author upon reasonable request.
Disclosure statement
No potential conflict of interest was reported by the author(s).
Additional information
Funding
References
- Cosman F, de Beur SJ, LeBoff MS, et al. Clinician’s guide to prevention and treatment of osteoporosis. Osteoporos Int. 2014;25(10):2359–2381.
- Ge DW, Wang WW, Chen HT, et al. Functions of microRNAs in osteoporosis. Eur Rev Med Pharmacol Sci. 2017;21:4784–4789.
- Mundy GR, Elefteriou F. Boning up on ephrin signaling. Cell. 2006;126:441–443.
- Pagani F, Francucci CM, Moro L. Markers of bone turnover: biochemical and clinical perspectives. J Endocrinol Invest. 2005;28(10 Suppl):S8–S13.
- Zhang RF, Wang Q, Zhang AA, et al. Low-level laser irradiation promotes the differentiation of bone marrow stromal cells into osteoblasts through the APN/Wnt/β‑catenin pathway. Eur Rev Med Pharmacol Sci. 2018;22:2860–2868.
- Wang R, Xu B, Xu HG. Up-Regulation of TGF‑β promotes Tendon-to-Bone healing after anterior cruciate ligament reconstruction using Bone Marrow-D erived mesenchymal stem cells through the TGF-β/MAPK signaling pathway in a New Zealand White Rabbit model. Cell Physiol Biochem. 2017;41:213–226.
- Fan JZ, Yang L, Meng GL, et al. Estrogen improves the proliferation and differentiation of hBMSCs derived from postmenopausal osteoporosis through notch signaling pathway. Mol Cell Biochem. 2014;392:85–93.
- Ishii N, Ozaki K, Sato H, et al. Identification of a novel non-coding RNA, MIAT, that confers risk of myocardial infarction. J Hum Genet. 2006;51:1087–1099.
- Yan BA, Yao J, Liu JY, et al. lncRNA-miat regulates microvascular dysfunction by functioning as a competing endogenous RNA. Circ Res. 2015;116(7):1143–1156.
- Rao SQ, Hui HL, Ye N, et al. Genetic variants in long non-coding RNA MIAT contribute to risk of paranoid schizophrenia in a Chinese Han population. Schizophr Res. 2015;166:125–130.
- Lai IL, Yang CA, Lin PC, et al. Long noncoding RNA MIAT promotes non-small cell lung cancer proliferation and metastasis through MMP9 activation. Oncotarget. 2017;8:98148–98162.
- Jin C, Zheng Y, Huang Y, et al. Long non-coding RNA MIAT knockdown promotes osteogenic differentiation of human adipose-derived stem cells. Cell Biol Int. 2017;41(1):33–41.
- Ishii N, Ozaki K, Sato H, et al. Identification of a novel non-coding RNA, MIAT, that confers risk of myocardial infarction. J Hum Genet. 2006;51(12):1087–1099.
- Fang B, Li Y, Chen C, et al. Huo Xue Tong Luo capsule ameliorates osteonecrosis of femoral head through inhibiting lncRNA-Miat. J Ethnopharmacol. 2019;238:111862.
- Jia J, Tian Q, Ling S, et al. MiR-145 suppresses osteogenic differentiation by targeting Sp7. FEBS Lett. 2013;587:3027–3031.
- Chen L, Holmstrom K, Qiu W, et al. MicroRNA-34a inhibits osteoblast differentiation and in vivo bone formation of human stromal stem cells. Stem Cells. 2014;32:902–912.
- Wang H, Ding XG, Yang JJ, et al. LncRNA MIAT facilitated BM-MSCs differentiation into endothelial cells and restored erectile dysfunction via targeting miR-200a in a rat model of erectile dysfunction. Eur J Cell Biol. 2018;97:180–189.
- Shen Y, Dong LF, Zhou RM, et al. Role of long noncoding RNA MIAT in proliferation, apoptosis and migration of lens epithelial cells: a clinical and in vitro study. J Cell Mol Med. 2016;20(3):537–548.
- Dong CL, Liu HZ, Zhang ZC, et al. The influence of MicroRNA-150 in Osteoblast Matrix Mineralization. J Cell Biochem. 2015;116(12):2970–2979.
- Choi SW, Lee SU, Kim EH, et al. Osteoporotic bone of miR-150-deficient mice: possibly due to low serum OPG-mediated osteoclast activation. Bone Rep. 2015;3:5–10.
- Mao Y, Yan R, Li A, et al. Lentiviral Vectors Mediate Long-Term and High Efficiency Transgene Expression in HEK 293T cells. Int J Med Sci. 2015;12(5):407–415.
- Zhang L, Tang Y, Zhu X, et al. Overexpression of MiR-335-5p Promotes Bone Formation and Regeneration in Mice. J Bone Miner Res. 2017;32(12):2466–2475.
- Chen L, Zhang D, Yu L, et al. Targeting MIAT reduces apoptosis of cardiomyocytes after ischemia/reperfusion injury. Bioengineered. 2019;10(1):121–132.
- Chen J, Chen T, Zhu Y, et al. circPTN sponges miR-145-5p/miR-330-5p to promote proliferation and stemness in glioma. J Exp Clin Cancer Res. 2019;38(1):398.
- Zhang X, Wang S, Wang H, et al. Circular RNA circNRIP1 acts as a microRNA-149-5p sponge to promote gastric cancer progression via the AKT1/mTOR pathway. Mol Cancer. 2019;18(1):20.
- Zhang SF, Zhang K, Cheng HM, et al. Comparative transcriptomics reveals colony formation mechanism of a harmful algal bloom species Phaeocystis globosa. Sci Total Environ. 2020;719:137454.
- Nakamura T, Nakamura-Takahashi A, Kasahara M, et al. Tissue-nonspecific alkaline phosphatase promotes the osteogenic differentiation of osteoprogenitor cells. Biochem Biophys Res Commun. 2020;524(3):702–709.
- Liang J, Chen C, Liu H, et al. Gossypol Promotes Bone Formation in Ovariectomy-Induced Osteoporosis through Regulating Cell Apoptosis. Biomed Res Int. 2018;2018:3635485.
- Xu R, Shi G, Xu L, et al. Simvastatin improves oral implant osseointegration via enhanced autophagy and osteogenesis of BMSCs and inhibited osteoclast activity. J Tissue Eng Regen Med. 2018;12:1209–1219.
- Wang R, Xu B, Xu HG. Up-Regulation of TGF-β promotes Tendon-to-Bone healing after anterior cruciate ligament reconstruction using Bone Marrow-D erived mesenchymal stem cells through the TGF-β/MAPK signaling pathway in a New Zealand White Rabbit model. Cell Physiol Biochem. 2017;41:213‑226.
- Wapinski O, Chang HY. Long noncoding RNAs and human disease. Trends Cell Biol. 2011;21:354–361.
- Esteller M. Non-coding RNAs in human disease. Nat Rev Genet. 2011;12:861–874.
- Liao J, He Q, Li M, et al. LncRNA MIAT: myocardial infarction associated and more. Gene. 2016;578(2):158–161.
- Yan B, Yao J, Liu JY, et al. LncRNA-MIAT regulates microvascular dysfunction by functioning as a competing endogenous RNA. Circ Res. 2015;116(7):1143–1156.
- Mundy GR, Elefteriou F. Boning up on ephrin signaling. Cell. 2006;126:441–443.
- Bai Y, Yin G, Huang Z, et al. Localized delivery of growth factors for angiogenesis and bone formation in tissue engineering. Int Immunopharmacol. 2013;16:214–223.
- Qiu J, Huang G, Na N, et al. MicroRNA‑ 214‑5p/TGF‑β/Smad2 signaling alters adipogenic differentiation of bone marrow stem cells in postmenopausal osteoporosis. Mol Med Rep. 2018;17:6301‑6310.
- Abdi J, Mutis T, Garssen J, et al. Toll‑like receptor (TLR)‑1/2 triggering of multiple myeloma cells modulates their adhesion to bone marrow stromal cells and enhances bortezomib‑induced apoptosis. PLoS One. 2014;9:e96608.