ABSTRACT
LncRNA down syndrome cell adhesion molecule antisense 1 (DSCAM-AS1) plays an important role in tumor progression, but its function in pancreatic cancer is unknown. DSCAM-AS1 level was evaluated by in situ hybridization (ISH) assay and qRT-PCR. DSCAM-AS1 was knocked down in pancreatic cancer cells, and its impacts on cell proliferation, invasion, and migration were detected. The binding relationship among DSCAM-AS1, miR-136-5p, and pre-B-cell leukemia homeobox 3 (PBX3) was investigated by bioinformatic analysis and luciferase reporter assay. An in vivo animal model was constructed to determine the role of DSCAM-AS1 in tumor growth. Our results showed that DSCAM-AS1 was elevated in tumor tissues of pancreatic cancer patients and cell lines. DSCAM-AS1 knockdown efficiently inhibited PANC-1 cell proliferation, migration, and invasion and suppressed tumor growth. DSCAM-AS1 could promote PBX3 expression by sponging miR-136-5p, and its function in pancreatic cancer was partially mediated by the miR-136-5p/PBX3 axis. Overall, DSCAM-AS1 knockdown inhibits pancreatic cancer progression by modulating the miR-136-5p/PBX3 axis.
KEYWORDS:
Introduction
Pancreatic cancer is a common invasive malignancy with approximate 227,000 deaths annually worldwide [Citation1]. Although great advances have been achieved in pancreatic cancer diagnosis and treatment [Citation2,Citation3], the overall five-year survival rate is still low (about 6%) [Citation4]. Hence, identifying novel diagnostic and therapeutic targets for pancreatic cancer is urgently needed.
LncRNAs are emerging as novel regulators in human cancer with more than 200 nt in length [Citation5]. In pancreatic cancer, more and more lncRNAs with important roles in cancer progression have been identified, such as HOXA-AS2 [Citation6], BX111 [Citation7], ZEB2-AS1 [Citation8], and HOTTIP [Citation9]. LncRNA down syndrome cell adhesion molecule antisense 1 (DSCAM-AS1) is a newly identified lncRNA [Citation10] with a significant impact on the development of various human cancers. For instance, DSCAM-AS1 is highly expressed in osteosarcoma tumor tissues and aggravates tumor cell proliferative rate and invasive ability [Citation11]. Moreover, DSCAM-AS1 is involved in G(1)/S cell cycle transition of breast cancer and promotes tumor growth [Citation10]. Although the crucial functions of DSCAM-AS1 in various cancers are known, its role in pancreatic cancer is still lacking.
MicroRNAs (miRNAs) are another group of small molecule RNAs that exert important functions in tumor progression through modulating mRNA translation and degradation [Citation12]. MiR-136-5p is reported as a tumor suppressor in various human cancers, including bladder cancer [Citation13], laryngeal squamous cell carcinoma [Citation14], and retinoblastoma [Citation15]. Moreover, previous studies have reported that pre-B-cell leukemia homeobox 3 (PBX3) is a molecular target of miR-136-5p in multiple tumors, and this axis plays an essential role in cancer progression. For example, miR-136-5p prevents retinoblastoma progression by targeting PBX3 [Citation16]. Previous studies reported that PBX3 is notably upregulated in pancreatic cancer tissues, and high PBX3 level is positively correlated to the tumor progression of AsPC-1 and BxPC-3 cells [Citation17].
Here, we hypothesized that lncRNA DSCAM-AS1 might play a role in pancreatic cancer progression, investigated its expression and potential function in pancreatic cancer, and revealed that miR-136-5p/PBX axis mediated the role of DSCAM-AS1 in pancreatic cancer.
Materials and methods
Tissue samples
A total of 30 patients with pancreatic cancer were recruited at West China Hospital, Sichuan University. Tumor tissues and normal pancreatic adjacent tissues were obtained by surgical resection. This study protocol was approved by the Ethics Committees of West China Hospital affiliated to Sichuan University (No. 9632). All patients signed the written informed consent. The clinical characteristics of pancreatic cancer patients are shown in Supplementary .
Table 1. Sequences of primers used in qRT-PCR
Cell culture
Human pancreatic cancer cell lines SW1990, BXPC-3, PANC-1, and PaCa-2, HEK-293 T, and HPDE6-C7 were purchased from American Type Culture Collection (ATCC, Rockville, MD, USA) and grown in DMEM medium containing 10% FBS at 37°C with 5% additional CO2.
Cell transfection
Two specific small interfering RNA targeting DSCAM-AS1 were obtained from GenePharma (Shanghai, China). MiR mimics (5ʹ-ACUCCAUUUGUUUUGAUGAUGG-3ʹ), NC mimics (5ʹ-TTCTCCGAACGTGTCACGT-3ʹ), miR-136-5p inhibitor (5ʹ-CCAUCAUCAAAACAAAUGGAGU-3ʹ), and NC inhibitor (5ʹ-UUCUCCGAACGUGUCACGUTT-3ʹ). For DSCAM-AS1 and PBX3 overexpression, the full length of DSCAM-AS1 and PBX3 was cloned into pcDNA3.1 (GenePharma, Shanghai, China), respectively, and the recombinant vectors were named by pDSCAM-AS1 and pPBX3. For establishing stable DSCAM-AS1 knockdown cells, short hairpin RNA against DSCAM-AS1 (sh-DSCAM-AS1, 5ʹ-GGAGATCACAGCCAAGGAA-3ʹ) and negative control (sh-NC, 5ʹ-TTCTCCGAACGTGTCACGTTT-3ʹ) were transfected into PANC-1 cells, and stable DSCAM-AS1 knockdown cells were screened out as previously reported [Citation18].
QRT-PCR
Total RNA was extracted with TRIzol reagent (Invitrogen, Grand Island, NY) and reversely transcribed into cDNA using Superscript III transcriptase (Invitrogen). Gene expression was detected using PCR on an ABI 7500 qPCR system with specific primers listed in . Data were analyzed using the 2−ΔΔCt method [Citation19].
Western blot
The expression of interest proteins was detected by Western blot as described in the previous study [Citation20]. Total protein was isolated with RIPA lysis buffer with 1% proteinase inhibitor (PMSF, Boster, China). After separation on 8% SDS-PAGE, protein samples were transferred onto PVDF membrane and incubated with antibodies against PBX3 (47 kDa, Abcam, ab109173, 1: 500) or GAPDH (36 kDa, Abcam, ab9485, 1: 1000) overnight at 4 ℃. Subsequently, the membranes were incubated with HRP-conjugated anti-rabbit IgG secondary antibodies (1:3000, Boster, Wuhan, China) at 37°C for 1 h. The protein signals were observed with an ECL detection system.
Luciferase reporter assay
The wild-type (WT-) or mutant (MUT-) binding sites of DSCAM-AS1 and PBX3 were cloned into luciferase reporter vector psiCheck2. The recombinant luciferase reporter vectors and miR-136-5p mimics or mimics NC were co-transfected into HEK293T cells using Lipofectamine 2000. After two days, the luciferase activities were measured using a Dual-Glo Luciferase Assay System (Promega Corporation). The relative luciferase activity was calculated as the ratio of firefly/Renilla luciferase activity [Citation21].
Immunofluorescence
Transfected PANC-1 cells were incubated with anti-PBX3 antibodies (Abcam, ab233136, 1:500) overnight at 4°C. After incubation with FITC-conjugated secondary antibodies (Abcam, ab6717, 1:1000) for 1 h and DAPI for nuclei, images were taken using a Zeiss LSM510 microscope.
ISH assay
The tumor tissues were fixed in 4% polyoxymethylene, embedded in paraffin, and cut into 4 μm sections. The ISH assay was performed using the Enhanced Sensitive ISH Detection Kit (POD) (Boster Biotechnology) with Digoxin-labeled DSCAM-AS1 probes from Life Technologies (Shanghai, China) [Citation22]. The images were obtained using a Zeiss LSM510 microscope.
In vivo model
The animal model was conducted as previously reported [Citation23]. 5 × 106 stable sh-DSCAM-AS1-transfected PANC-1 cells were subcutaneously inoculated into BALB/c nude mice. Tumor volumes were assessed every week for five weeks based on length×width2/2. After that, mice were sacrificed, and solid tumors were weighted. To detect the proliferative rate in vivo, immunohistochemistry (IHC) assay was performed using anti-Ki67 (ab15580, 1:500, Abcam) as previously described [Citation24]. The study was approved by the Animal Research Committee of West China Hospital, Sichuan University.
Statistical analysis
Data were analyzed using Graphpad Prism7 software and expressed as the mean ± standard deviation (SD). Differences between two groups and among multiple groups were compared using two-tailed unpaired Student’s t-test or ANOVA with Scheffe’s post hoc test, respectively. A p < 0.05 represents statistical significance.
Results
DSCAM-AS1 was up-regulated in pancreatic cancer
For investigating the role of DSCAM-AS1 in pancreatic cancer progression, we first detected its expression in pancreatic cancer tumor tissues and cell lines. ISH () and qRT-PCR assay () showed that DSCAM-AS1 was significantly upregulated in pancreatic cancer tissues (p < 0.01), and high DSCAM-AS1 level was positively correlated with tumor progression in patients (Supplementary ). In addition, we found that DSCAM-AS1 levels were higher in the four cancer cell lines than in HPDE6-C7 cells, with the highest level in PANC-1 (). Thus, PANC-1 cells were used for the subsequent studies. Overall, these results suggested that DSCAM-AS1 was highly expressed in pancreatic cancer tumor tissues and cell lines.
DSCAM-AS1 knockdown inhibited pancreatic cancer progression in vitro
After detecting DSCAM-AS1 expression, we knocked DSCAM-AS1 down in PANC-1 cells by transfecting two DSCAM-AS1 siRNAs to investigate its function (both p < 0.01, ). DSCAM-AS1 knockdown significantly decreased viability (p < 0.01, ), colony numbers (p < 0.01, ), migration (p < 0.01, ), and invasion (p < 0.01, ) of PANC-1 cells, suggesting that DSCAM-AS1 knockdown exerted an inhibitory role in pancreatic cancer cell proliferation, migration, and invasion.
Figure 2. DSCAM-AS1 inhibition efficiently prevented pancreatic cancer progression in vitro. PANC-1 cells were transfected with two siRNAs targeting DSCAM-AS1. (a) DSCAM-AS1 level. (b) CCK-8 assay. (c) Colony formation assay. (d) Migration assay. (e) Invasion assay. ×200, scale bar = 100 μm. ** p < 0.01 vs. si-NC.
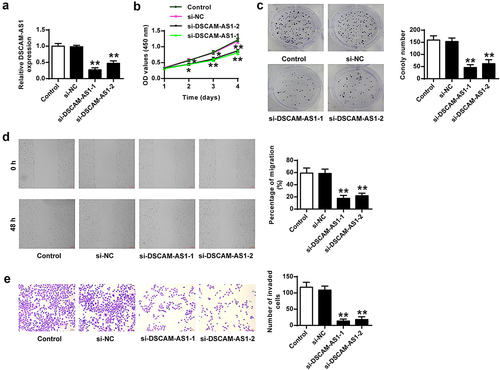
DSCAM-AS1 knockdown prevented tumor growth
Subsequently, we established an in vivo animal model by subcutaneously inoculating mice with stable sh-DSCAM-AS1-transfected PANC-1 cells to examine the function of DSCAM-AS1 in pancreatic cancer growth. We found that DSCAM-AS1 knockdown significantly reduced DSCAM-AS1 level in tumor tissues (p < 0.01, ) and notably inhibited tumor growth (p < 0.01) (). qRT-PCR analysis showed that DSCAM-AS1 knockdown also significantly upregulated miR-136-5p (p < 0.01) and downregulated PBX3 (p < 0.01) in tumor tissues (). Western blot assay confirmed the reduction of PBX3 in tumor tissues by DSCAM-AS1 knockdown (). Furthermore, IHC assay showed that DSCAM-AS1 knockdown obviously decreased Ki-67 positive cells in mice (p < 0.01, ). Overall, DSCAM-AS1 knockdown slowed pancreatic tumor growth in vivo.
Figure 3. DSCAM-AS1 knockdown inhibited tumor growth in vivo. (a) DSCAM-AS1 level in tumor tissues by qRT-PCR. (b) Representative tumor images. (c) Tumor volume. (d) Tumor weight. (e) MiR-136-5p and PBX3 levels in tumor tissues by qRT-PCR. (f) PBX3 level in tumor tissues. (g) Ki-67 levels in tumor tissues by IHC staining assay. ×200, scale bar = 100 μm.
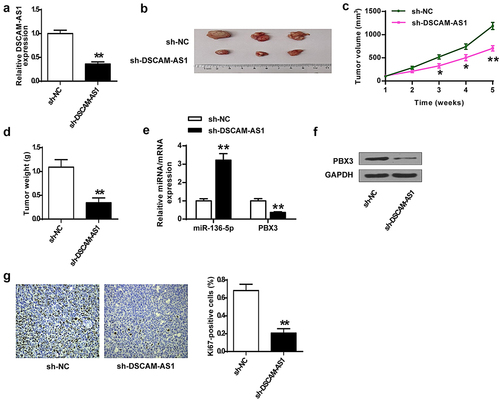
DSCAM-AS1 directly bound to miR-136-5p
Current studies revealed multiple action mechanisms of DSCAM-AS1 in tumor progression, including regulating gene expression by sponging miRNAs to disrupt their functions. Thus, we first confirmed the effects of miR-136-5p mimics and miR-136-5p inhibitor on miR-136-5p levels (both p < 0.01, ) and predicted the potential binding between DSCAM-AS1 and miR-136-5p via bioinformatics analysis using Starbase software (). To confirm the prediction, we performed luciferase reporter assays in PNAC-1 cells co-transfected with DSCAM-AS1 3ʹ-UTR WT/Mut with miR-136-5p mimics/NC mimics. The results showed that miR136-5p overexpression notably reduced the luciferase activity of DSCAM-AS1 3ʹ-UTR WT (p < 0.01) but had no effect on DSCAM-AS1 3ʹ-UTR MUT (). Furthermore, DSCAM-AS1 knockdown significantly elevated miR-136-5p level in PNAC-1 cells (both p < 0.01, ). Moreover, miR-136-5p mimics significantly reduced DSCAM-AS1 level (p < 0.01) while miR-136-5p inhibitor enhanced DSCAM-AS1 level (p < 0.01) in PNAC-1 cells (). Furthermore, miR-136-5p levels were significantly reduced in tumor tissues (p < 0.01, ) and had a significantly negative correlation with DSCAM-AS1 level (p < 0.01, ). We further investigated the correlation of miR-136-5p expression with patients’ clinicopathological parameters. The results showed that high miR-136-5p levels were negatively correlated with tumor progression in patients (Supplementary Table 2). Taken together, these results suggested that DSCAM-AS1 directly targeted miR-136-5p in pancreatic cancer, and miR-136-5p was downregulated in pancreatic tumor tissues.
Figure 4. DSCAM-AS1 directly bound to miR-136-5p. (a) The transfection efficiency of miR-136-5p mimics or inhibitor was confirmed. (b) The putative interaction between DSCAM-AS1 and miR-136-5p by Starbase. (c) Luciferase reporter assay. (d) PNAC-1 cells were transfected with si-DSCAM-AS1-1 and si-DSCAM-AS1-1, and miR-136-5p expression was evaluated. (e) DSCAM-AS1 level in PNAC-1 cells after transfection with miR-136-5p mimics or inhibitor. (f) MiR-136-5p level in tumor tissues. (g) The correlation between DSCAM-AS1 and miR-136-5p levels in tumor tissues.
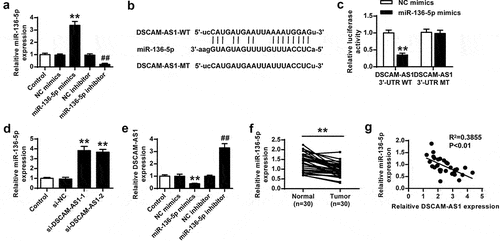
MiR-136-5p mimics inhibited pancreatic cancer behaviors, possibly via regulating PBX3 in vitro
To investigate the function of miR-136-5p in pancreatic cancer, miR-136-5p mimics were transfected into tumor cells. The results showed that miR-136-5p mimics significantly inhibited viability (p < 0.05), colony formation (p < 0.01), migration (p < 0.01), and invasion (p < 0.01) of PANC-1 cells compared with NC mimics (). We further investigated the downstream pathway of miR-136-5p using Starbase and found that PBX3 might be a downstream gene of miR-136-5p (). Luciferase reporter assay showed that miR-136-5p overexpression significantly reduced the relative luciferase activity of PBX3 3ʹ-UTR WT (p < 0.01) but had no impact on PBX3 3ʹ-UTR MUT (). PBX3 expression was significantly increased in pancreatic cancer tissues compared with normal controls (). Furthermore, high PBX3 were positively correlated with tumor progression in patients (Supplementary Table 3). Next, we performed rescue experiments by transfecting DSCAM-AS1 overexpression vector into PANC-1 cells (p < 0.01, ) and found that miR-136-5p mimics reduced PBX3 expression (p < 0.01) and co-transfection of DSCAM-AS1 with miR-136-5p mimics attenuated the inhibitory impact of miR-136-5p mimics on PBX3 expression (p < 0.01, ). Immunofluorescence staining also confirmed that DSCAM-AS1 overexpression relieved the inhibitory effect of miR-136-5p mimics on PBX3 in PANC-1 cells (). Taken together, these results suggested that miR-136-5p played a tumor suppressor role in pancreatic cancer via targeting PBX3.
Figure 5. MiR-136-5p mimics inhibited pancreatic cancer progression, possibly via PBX3 in vitro. (a) CCK-8 assay. (b) Colony formation assay. (c) Migration assay. (d) Invasion assay. (e) The putative interaction between miR-136-5p and PBX3 by Starbase. (f) Luciferase reporter assay. (g) The transfection efficiency of DSCAM-AS1 overexpressing vector. (h and i) PBX3 level by qRT-PCR (h) and Western blot (i). (j) PBX3 level in PANC-1 cells by immunofluorescence. ×200, scale bar = 100 μm.
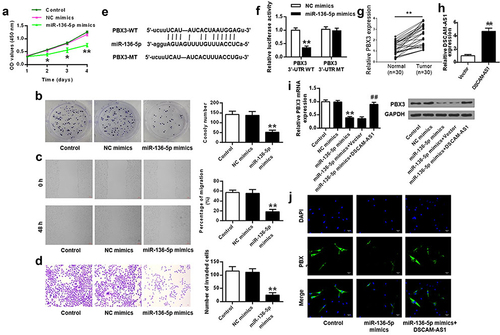
DSCAM-AS1 regulated pancreatic cancer progression via modulating miR-136-5p/PBX3
We further performed rescue experiments to investigate the role of miR-136-5p/PBX3 axis in the function of DSCAM-AS1. PANC-1 cells were transfected in vitro with miR-136-5p mimics, or co-transfected with miR-136-5p mimics and DSCAM-AS1 overexpressing vector (both p < 0.01, ). DSCAM-AS1 overexpression notably reversed the inhibitory impacts of miR-136-5p mimics on pancreatic cancer cell proliferation, invasion, and migration (all p < 0.01, ), suggesting that miR-136-5p mediated the pro-tumor function of DSCAM-AS1.
Figure 6. MiR-136-5p mediated the impact of DSCAM-AS1 in pancreatic cancer cells in vitro. (a) DSCAM-AS1 level by qRT-PCR. (b) CCK-8 assay. (c) Colony formation assay. (d) Migration assay. (e) Invasion assay. ×200, scale bar = 100 μm.
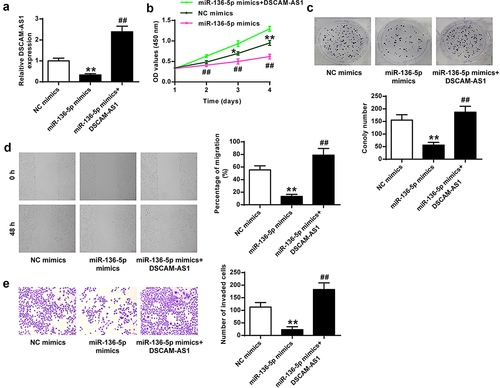
To investigate the regulatory axis of DSCAM-AS1 and PBX3, we constructed a PBX3 overexpressing vector and confirmed that its transfection significantly increased PBX3 at mRNA and protein levels (p < 0.01, and b). Then we transfected PANC-1 cells with si-DSCAM-AS1-1 or co-transfected with si-DSCAM-AS1-1 and PBX3 overexpressing vector and found that si-DSCAM-AS1-1 significantly reduced PBX3 level (p < 0.01) and co-transfection of PBX3 overexpressing vector obviously abolished the effect of si-DSCAM-AS1-1 on PBX3 level (p < 0.01) ( and d). The function assays showed that PBX3 overexpression efficiently reversed the inhibitory impacts of si-DSCAM-AS1-1 on the phenotype of PANC-1 cells (). Taken together, these results suggested that miR-136-5p/PBX3 axis mediated the pro-tumor function of DSCAM-AS1-1 in pancreatic cancer.
Figure 7. DSCAM-AS1 regulated pancreatic cancer progression via modulating PBX3. (a and b) The transfection efficiency by qRT-PCR (a) and Western blot (b). (c and d) PBX3 level by qRT-PCR (c) and Western blot (d). (e) CCK-8 assay. (f) Colony formation assay. (g) Migration assay. (h) Invasion assay. ×200, scale bar = 100 μm.
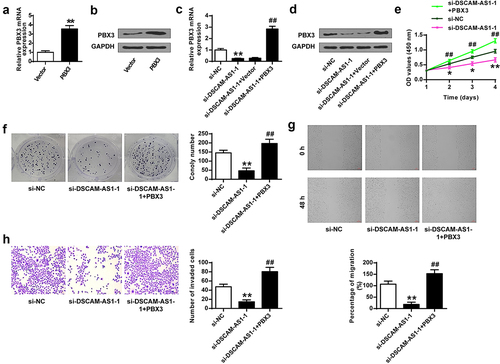
Discussion
The study investigated the expression and function of lncRNA DSCAM-AS1 in pancreatic cancer. We found that lncRNA DSCAM-AS1 was downregulated in pancreatic cancer tissues and cell lines and directly targeted MiR-136-5p to mediate its oncogenic role in the tumorigenesis and progression of pancreatic cancer. Moreover, miR-136-5p bound to PBX3 to downregulate its expression to further promote tumor proliferation, migration, and invasion. Our findings extended our knowledge of lncRNA mechanism in pancreatic cancer progression and provided a potential therapeutic target.
LncRNAs play fundamental roles in carcinogenesis. As a typical lncRNA, lncRNA DSCAM-AS1 is first identified in screening differentially expressed transcripts between breast cancer cells and benign breast samples [Citation25]. Subsequent studies have demonstrated that lncRNA DSCAM-AS1 is upregulated and plays a pro-tumor role in lung cancer, colorectal cancer, osteosarcoma, hepatocellular carcinoma, melanoma, and cervical cancer [Citation26]. For example, DSCAM-AS1 is upregulated in many human cancers and functions via miR-384/AKT3 in colorectal cancer [Citation27], miR-877-5p/ATXN7L3 in cervical cancer [Citation28], and miR577/HMGB1 in non-small cell lung cancer [Citation29]. This study investigated its role in pancreatic cancer and found that DSCAM-AS1 was also notably upregulated in pancreatic cancer. In addition, its high expression in tumor tissues was associated with malignant tumor progression in patients, implying that DSCAM-AS1 might be a prognostic biomarker for pancreatic cancer. Other studies also showed that serum DSCAM-AS1 level was increased in patients with hepatocellular carcinoma than in healthy controls [Citation30]. In the future, we will investigate the potential of serum DSCAM-AS1 as a prognostic marker for patients with pancreatic cancer. DSCAM-AS1 knockdown efficiently inhibited tumor progression, including proliferation, migration, invasion, and in vivo growth, consistent with previous reports. Recent studies have shown that non-coding RNAs (ncRNAs), including lncRNAs, are enriched in exosomes, and exosome treatment has become a promising therapeutic strategy [Citation31]. Hence, identifying DSCAM-AS1 inhibitor-related drugs and how to deliver them to the human body is the next stage of our work.
MiRNAs represent a category of non-coding RNAs with ~22 bp in length. Tumor molecular studies have demonstrated that multiple miRNAs play important roles in tumor progression [Citation32–36]. For example, microRNA-409 is demonstrated to act as a tumor suppressor in pancreatic carcinoma and is associated with a good prognosis in tumor patients [Citation37]. LncRNAs have been identified as specific sponges for miRNAs and inhibit their expression [Citation38]. DSCAM-AS1 was reported to participate in tumor progression by sponging several miRNA targets, such as miR-101-3p in gastric cancer [Citation39], miR-216b in colorectal adenocarcinoma [Citation40], and miR-338-3p in hepatocellular carcinoma [Citation30]. Our study revealed a negative correlation between DSCAM-AS1 and miR-136-5p levels in tumor tissues and confirmed their binding relationship. Moreover, the rescue experiments further confirmed the regulatory network of DSCAM-AS1 and miR-136-5p in pancreatic cancer. MiR-136-5p is a well-known tumor suppressor miRNA in multiple tumor types. For example, miR-136-5p is downregulated in hepatocellular carcinoma tissues compared with para-non-tumor tissues, and its high expression is associated with a good prognosis [Citation41]. Exosomal miR-136-5p derived from anlotinib-resistant NSCLC cells confers anlotinib resistance in non-small cell lung cancer through targeting PPP2R2A [Citation42]. However, as far as we know, there is no study exploring the role of miR-136-5p in the progression of pancreatic cancer. In addition, whether one or more downstream miRNA targets mediate the role of DSCAM-AS1 in pancreatic cancer needs to be investigated in the future, which will contribute to our understanding of the functions of DSCAM-AS1 in pancreatic cancer.
Previous studies have indicated that miRNAs could regulate gene expression by binding to the 3ʹ untranslated region (3ʹ-UTR) of their targeted genes in cancer [Citation43]. Here, we predicted the downstream targets of miR-136-5p and found that PBX3 might be a target of miR-136-5p. DSCAM-AS1 could positively regulate PBX3 by modulating miR-136-5p. Low PBX3 level due to si-DSCAM-AS1-1 transfection suppressed tumor cell progression, and PBX3 overexpression reversed the inhibitory effect of si-DSCAM-AS1-1. This phenotype was consistent with a previous finding that high PBX3 levels were closely related to the aggravated progression of pancreatic cancer in vitro [Citation17]. A series of rescue experiments revealed the regulatory axis of DSCAM-AS1/miR-136-5p/PBX3 in pancreatic cancer. However, a single miRNA might play its essential role in human cancers by targeting one or more downstream genes [Citation44,Citation45]. For example, miR-136-5p could target several downstream genes, including CBX4 in cervical cancer [Citation46], BCL2 in non-small cell lung cancer [Citation47], ROCK1 in head and neck cancer [Citation48], MMP2 in hepatocellular carcinoma [Citation49], and XIAP in ovarian tissues [Citation50]. Hence, other potential regulatory axises for miR-136-5p in pancreatic cancer should be explored.
Although our study revealed a regulatory axis in pancreatic cancer, whether manipulating miR-136-5p and PBX3 in vivo could affect the function of DSCAM-AS1 should be demonstrated in subsequent experiments.
Conclusion
DSCAM-AS1 promoted pancreatic cancer progression by regulating the miR-136-5p/PBX3 axis, suggesting that this axis could be a novel target for pancreatic cancer treatment.
Research Highlights
DSCAM-AS1 plays a pro-tumor role in pancreatic cancer.
DSCAM-AS1 promotes PBX3 expression via sponging miR-136-5p.
DSCAM-AS1/miR-136-5p/PBX3 participates in pancreatic cancer progression.
Ethical Approval and Consent to participate
All patients signed the written informed consent. All procedures were approved by West China Hospital, Sichuan University Ethics Committee and Animal Ethics Committee. Procedures operated in this research were completed in keeping with the standards set out in the Announcement of Helsinki and laboratory guidelines of research in China and the principles on ethical animal research outlined in the Basel Declaration.
Availability of supporting data
The data that support the findings of this study are available on request from the corresponding author.
Supplemental Material
Download Zip (25.8 KB)Acknowledgements
We thank the financial support from 1.3.5 project for disciplines of excellence, West China Hospital, Sichuan University(ZYJC18027)and Sichuan Provincial Department of Science and Technology Supporting Project(2018SZ0381)
Disclosure statement
No potential conflict of interest was reported by the author(s).
Supplementary Material
Supplemental data for this article can be accessed here
Additional information
Funding
References
- Vincent A, Herman J, Schulick R, et al. Pancreatic cancer. Lancet. 2011 Aug 13;378(9791):607–620.
- Chu LC, Goggins MG, Fishman EK. Diagnosis and detection of pancreatic cancer. Cancer J. 2017 Nov/Dec;23(6):333–342.
- Gupta R, Amanam I, Chung V. Current and future therapies for advanced pancreatic cancer. Journal of Surgical Oncology. 2017 Jul;116(1):25–34.
- Ilic M, Ilic I. Epidemiology of pancreatic cancer. World J Gastroenterol. 2016 Nov 28;22(44):9694–9705.
- Peng WX, Koirala P, Mo YY. LncRNA-mediated regulation of cell signaling in cancer. Oncogene. 2017 Oct 12;36(41):5661–5667.
- Wang J, Su Z, Lu S, et al. LncRNA HOXA-AS2 and its molecular mechanisms in human cancer. Clin Chim Acta. 2018 Oct;485:229–233.
- Deng SJ, Chen HY, Ye Z, et al. Hypoxia-induced LncRNA-BX111 promotes metastasis and progression of pancreatic cancer through regulating ZEB1 transcription. Oncogene. 2018 Nov;37(44):5811–5828.
- Gao H, Gong N, Ma Z, et al. LncRNA ZEB2-AS1 promotes pancreatic cancer cell growth and invasion through regulating the miR-204/HMGB1 axis. Int J Biol Macromol. 2018 Sep;116:545–551.
- Fu Z, Chen C, Zhou Q, et al. LncRNA HOTTIP modulates cancer stem cell properties in human pancreatic cancer by regulating HOXA9. Cancer Lett. 2017 Dec 1;410:68–81.
- Liang WH, Li N, Yuan Z-Q. DSCAM-AS1 promotes tumor growth of breast cancer by reducing miR-204-5p and up-regulating RRM2. Molecular Carcinogenesis. 2019 Apr;58(4):461–473.
- Yu CL, Xu NW, Jiang W, et al. LncRNA DSCAM-AS1 promoted cell proliferation and invasion in osteosarcoma by sponging miR-101. Eur Rev Med Pharmacol Sci. 2020 Jul;24(14):7709–7717.
- Hammond SM. An overview of microRNAs. Advanced drug delivery reviews. Advanced Drug Delivery Reviews. 2015 Jun 29;87:3–14.
- Kang W, Wang Q, Dai Y, et al. Hypomethylation of PlncRNA-1 promoter enhances bladder cancer progression through the miR-136-5p/Smad3 axis. Cell Death Dis. 2020 Dec 7;11(12):1038.
- Wang Z, Huang C, Zhang A, et al. Overexpression of circRNA_100290 promotes the progression of laryngeal squamous cell carcinoma through the miR-136-5p/RAP2C axis. Biomed Pharmacothe. 2020 May;125:109874.
- Yang XL, Hao YJ, Wang B, et al. Long noncoding RNA NORAD promotes the progression of retinoblastoma by sponging miR-136-5p/PBX3 axis. Eur Rev Med Pharmacol Sci. 2020 Jan;24(3):1278–1287.
- Yang XL, Hao YJ, Wang B, et al. Long noncoding RNA NORAD promotes the progression of retinoblastoma by sponging miR 136-5p/PBX3 axis. Eur Rev Med Pharmacol Sci. 2020 Apr;24(8):4055.
- Qiu Z, Wang X, Shi Y, et al. miR-129-5p suppresses proliferation, migration, and induces apoptosis in pancreatic cancer cells by targeting PBX3. Acta Biochim Biophys Sin (Shanghai). 2019 Sep 6;51(10):997–1007.
- Chen F, Mo J, Zhang L. Long noncoding RNA BCAR4 promotes osteosarcoma progression through activating GLI2-dependent gene transcription. Tumour Biol. 2016 Oct;37(10):13403–13412.
- Livak KJ, Schmittgen TD. Analysis of relative gene expression data using real-time quantitative PCR and the 2(T)(-Delta Delta C) method. Methods. 2001 Dec;25(4):402–408.
- Luo J, Wang K, Yeh S, et al. LncRNA-p21 alters the antiandrogen enzalutamide-induced prostate cancer neuroendocrine differentiation via modulating the EZH2/STAT3 signaling. Nat Commun. 2019 Jun 12;10(1). DOI:10.1038/s41467-019-09784-9.
- Bai T, Liu Y, Li B. LncRNA LOXL1-AS1/miR-let-7a-5p/EGFR-related pathway regulates the doxorubicin resistance of prostate cancer DU-145 cells. Iubmb Life. 2019 Oct;71(10):1537–1551.
- Wang Z, Liu Z, Wu S. Long non-coding RNA CTA sensitizes osteosarcoma cells to doxorubicin through inhibition of autophagy. Oncotarget. 2017 May 9;8(19):31465–31477.
- Zeng Z, Xu FY, Zheng H, et al. LncRNA-MTA2TR functions as a promoter in pancreatic cancer via driving deacetylation-dependent accumulation of HIF-1α. Theranostics. 2019;9(18):5298–5314.
- Jin X, Fang R, Fan P, et al. PES1 promotes BET inhibitors resistance and cells proliferation through increasing c-Myc expression in pancreatic cancer. J Exp Clin Cancer Res. 2019 Nov 12;38(1):463.
- Liu D, Rudland PS, Sibson DR, et al. Identification of mRNAs differentially-expressed between benign and malignant breast tumour cells. Br J Cancer. 2002 Aug 12;87(4):423–431.
- Ghafouri-Fard S, Khoshbakht T, Taheri M, et al. A review on the carcinogenic roles of DSCAM-AS1. Front Cell Dev Biol. 2021 Oct 11;9. DOI:10.3389/fcell.2021.758513
- Li B, Sun H, Zhang J. LncRNA DSCAM-AS1 promotes colorectal cancer progression by acting as a molecular sponge of miR-384 to modulate AKT3 expression. Aging (Albany NY). 2020 May 26;12(10):9781–9792.
- Liang J, Zhang S, Wang W, et al. Long non-coding RNA DSCAM-AS1 contributes to the tumorigenesis of cervical cancer by targeting miR-877-5p/ATXN7L3 axis. Biosci Rep. 2020 Jan 31;40(1). DOI:10.1042/BSR20192061.
- Qiu Z, Pan XX, You DY. LncRNA DSCAM-AS1 promotes non-small cell lung cancer progression via regulating miR-577/HMGB1 axis. Neoplasma. 2020 Jul;67(4):871–879.
- Ji D, Hu G, Zhang X, et al. Long non-coding RNA DSCAM-AS1 accelerates the progression of hepatocellular carcinoma via sponging miR-338-3p. Am J Transl Res. 2019;11(7):4290–4302.
- Cheng J, Meng J, Zhu L, et al. Exosomal noncoding RNAs in Glioma: biological functions and potential clinical applications. Mol Cancer. 2020 Mar 25;19(1):66.
- Wang W, Hong G, Wang S, et al. Tumor-derived exosomal miRNA-141 promote angiogenesis and malignant progression of lung cancer by targeting growth arrest-specific homeobox gene (GAX). Bioengineered. 2021 Jan 1;12(1):821–831.
- Lu N, Liu J, Ji C, et al. MiRNA based tumor mutation burden diagnostic and prognostic prediction models for endometrial cancer. Bioengineered. 2021 Jan 1;12(1):3603–3620.
- Jiang H, Ge R, Chen S, et al. miRNA-204-5p acts as tumor suppressor to influence the invasion and migration of astrocytoma by targeting ezrin and is downregulated by DNA methylation. Bioengineered. 2021 Nov 1;12(2):9301–9312.
- Hu S, Zhang J, Fang X, et al. Identification of microRNA hsa-miR-30c-5p as an inhibitory factor in the progression of hepatocellular carcinoma and investigation of its regulatory network via comprehensive analysis. Bioengineered. 2021 Jan 1;12(1):7165–7177.
- Fan W, Yu X, Li K, et al. The alerting expression of microRNA-411 predicts clinical prognosis and regulates tumor progression of glioblastoma. Bioengineered. 2021 Jan 1;12(1):8650–8657.
- Long K, Dong Q, Zeng W. The clinical significance of microRNA-409 in pancreatic carcinoma and associated tumor cellular functions. Bioengineered. 2021 Jan 1;12(1):4633–4642.
- Chan JJ, Tay Y. Noncoding RNA:RNA Regulatory Networks in Cancer. Int J Mol Sci. 2018 Apr 27;19(5):1310.
- Wang B, Liu H, Wang Z, et al. Long non-coding RNA DSCAM-AS1 promotes the progression of gastric cancer via targeting miR-101-3p. Panminerva Med. 2019 Dec 17. DOI:10.23736/S0031-0808.19.03814-X.
- Liu F, Jia J, Sun L, et al. lncRNA DSCAM-AS1 downregulates miR-216b to promote the migration and invasion of colorectal adenocarcinoma cells. Onco Targets Ther. 2019;12:6789–6795.
- Ding H, Ye Z-H, Wen D-Y, et al. Downregulation of miR-136-5p in hepatocellular carcinoma and its clinicopathological significance. Mol Med Rep. 2017 Oct;16(4):5393–5405.
- Gu G, Hu C, Hui K, et al. Exosomal miR-136-5p derived from anlotinib-resistant NSCLC cells confers anlotinib resistance in non-small cell lung cancer through targeting PPP2R2A. Int J Nanomedicine. 2021;16:6329–6343.
- Lee YS, Dutta A. MicroRNAs in cancer. Annu Rev Pathol. 2009;4(1):199–227.
- Ji Q, Xu X, Song Q, et al. miR-223-3p inhibits human osteosarcoma metastasis and progression by directly targeting CDH6. Mol Ther. 2018 May 2;26(5):1299–1312.
- Xu J, Yao Q, Hou Y, et al. MiR-223/Ect2/p21 signaling regulates osteosarcoma cell cycle progression and proliferation. Biomed Pharmacothe. 2013 Jun;67(5):381–386.
- Zhao J, Yang T, Li L. LncRNA FOXP4-AS1 is involved in cervical cancer progression via regulating miR-136-5p/CBX4 axis. Onco Targets Ther. 2020;13:2347–2355.
- Geng Y, Bao Y, Zhang W, et al. Circular RNA hsa_circ_0014130 inhibits apoptosis in non-small cell lung cancer by sponging miR-136-5p and upregulating BCL2. Mol Cancer Res. 2020 May;18(5):748–756.
- Yang B, Zang J, Yuan W, et al. The miR-136-5p/ROCK1 axis suppresses invasion and migration, and enhances cisplatin sensitivity in head and neck cancer cells. Exp Ther Med. 2021 Apr;21(4):317.
- Liu D, Kang H, Gao M, et al. Exosome-transmitted circ_MMP2 promotes hepatocellular carcinoma metastasis by upregulating MMP2. Molecular Oncology. 2020 Jun;14(6):1365–1380.
- Lu X, Gao H, Zhu B, et al. Circular RNA circ_RANBP9 exacerbates polycystic ovary syndrome via microRNA-136-5p/XIAP axis. Bioengineered. 2021 Jan 1;12(1):6748–6758.