ABSTRACT
Recent studies suggested that propofol, one of the most widely used anesthetics, may cause neurotoxicity in the developing brain, leading to cognitive deficits in adults. However, the underlying mechanisms remain unclear. In this study, we aimed to evaluate the mechanisms of propofol neurotoxicity in the neural stem cells (NSCs). The mRNA and protein expression levels of microRNA-9-5p (miR-9-5p) and chemokine CXC receptor 4 (CXCR4) were determined by quantitative reverse transcription-polymerase chain reaction and Western blotting analyses. Cell viability and apoptosis were evaluated using the cell counting kit-8 and Hoechst staining kits. The levels of apoptosis-related proteins B-cell lymphoma 2 (Bcl-2), Bcl-2-associated X protein, and caspase-3 were detected by Western blotting analysis. These results confirmed that propofol activated cell apoptosis in a dose-dependent manner. A significant increase in the miR-9-5p and CXCR4 expression was observed in the propofol-treated cells. The overexpression of miR-9-5p induced apoptosis in NSCs, accompanied by elevated apoptosis-related protein activity. Furthermore, mitigated CXCR4 expression reduced propofol-induced cell apoptosis. We conclude that propofol induces cell death in NSCs, and overexpression of miR-9-5p/CXCR4 contributes to propofol-induced cell apoptosis, which might be a target for developing novel strategies to treat propofol neurotoxicity.
Introduction
Propofol is one of the most widely used intravenous anesthetics in clinical anesthesia and intensive care units. Additionally, due to its characteristic of rapid onset and smooth induction process, it is often used in pediatrics for anesthesia and sedation [Citation1]; it has a neuroprotective function that is used to treat hypoxic brain diseases [Citation2]. However, FDA has warned that long-term or repeated exposure to certain anesthetics, including propofol, in late pregnancy or children under 3 years of age could cause brain damage to the developing brain [Citation3]. Propofol has been found to cause growth cone collapse, axonal transport impairment, loss of synaptic connectivity, and behavioral deficits in neonatal mice [Citation4]. Therefore, propofol neurotoxicity has attracted significant attention.
Neural stem cells (NSCs) existing in the sub-granular area of the hippocampal gyrus of children’s brains can self-renew and generate the following three main cell forms in the central nervous system: neurons, astrocytes, and oligodendrocytes [Citation5]. It has been found that propofol can interfere with the neurogenesis of NSCs by affecting apoptosis, proliferation, or differentiation, the potential mechanisms related to Pink1, Ca2+, or microRNA (miRNAs) [Citation6–9].
miRNAs are endogenous short-stranded (19–22 nucleotides) non-coding RNAs that control gene expression through RNA silencing or post-transcriptional regulation. The microRNA-9 (miR-9), expressed in the central nervous system, is involved in a complex relationship between brain functions and disorders [Citation10]. Delaloy et al. found that the absence of miR-9 decreased proliferation but increased migration of the human neural progenitor cells during neurogenesis [Citation11]. In an Alzheimer’s disease (AD) cell model, the overexpression of miR-9-5p impairs autophagic activity and promotes the formation of amyloid plaques in the SH-SY5Y cells, while the application of miR-9-5p antagonist can improve amyloid β-protein clearance rate and cognitive ability and activity in AD mice in the late stage of AD [Citation12]. The expression of miR-9-5p was upregulated in an in vitro model of Parkinson’s disease, and miR-9-5p inhibitor alleviated 1-methyl-4-phenylpyridinium-induced neurotoxicity in SH-SY5Y cells [Citation13]. These investigations suggest that miR-9-5p is crucial to the physiological and pathological processes of the nervous system.
Rabenstein et al. found the positive effects of osteopontin on the survival, proliferation, migration, and neuronal differentiation of NSCs were achieved by upregulating chemokine CXC receptor 4 (CXCR4) expression [Citation14]. Hu et al. reported that CXCR4 gene expression decreased after propofol exposure in lung cancer cells [Citation15]. In addition, studies showed that the miR-9-5p/CXCR4 signaling pathway, induced by high glucose, is involved in angiogenesis and can damage the human umbilical vascular endothelial cells [Citation16]. However, the exact role of the miR-9-5p/CXCR4 signaling pathway in propofol neurotoxicity remains unclear.
In this study, we aimed to demonstrate the role of the miR-9-5p/CXCR4 signaling pathway in propofol neurotoxicity in the developing brain. In vitro, we found that the expression of miR-9-5p and CXCR4 increased after treatment with propofol. Further experiments found that miR-9-5p caused the death of NSCs by increasing the expression of CXCR4, and reducing the expression of CXCR4 could reverse the damage of propofol to neural stem cells. This study found that miR-9-5p can be used as a diagnostic marker to observe the neurotoxicity of propofol, and its downstream factor CXCR4 can be used as a drug target to reverse the propofol neurotoxicity.
Material and methods
C17.2 neural stem cell culture
The mouse NSC line C17.2 was cultured in a cell culture medium consisting of 89% Dulbecco’s modified eagle’s medium (DMEM; Gibco), 10% fetal bovine serum (FBS; Hyclone), and 1% 100 U/ ml penicillin and streptomycin (Gibco). All cultures were maintained in a 37°C humid environment containing 5% CO2 [Citation17].
Propofol exposure
Propofol (Sigma-Aldrich) was dissolved in dimethyl sulfoxide (DMSO; Solarbio) for the in vitro assays. According to previous methods, the dose of propofol ranges from approximately 10–100 μM [Citation17]. Thus, the propofol NSCs group were exposed to clinically relevant concentrations of propofol (10, 50, or 100 μM) for 6 h, with the concentration of DMSO < 0.05%. We selected the condition that NSCs exposed to 100 μM propofol for 6 h were subjected to the following mechanism experiments.
Cell viability assay
According to the manufacturer’s guidelines, the cell counting kit-8 (CCK-8 kit, Dojindo Laboratories) was used to determine cell viability. NSCs were plated in 96-well plates (8 × 103/well) for 24 h. Subsequently, the cells were treated with propofol (100 μM) for 6 h, and then 10 μl CCK-8 reagent was added to the wells. A microplate reader (Promega Corp) was used to determine the cell viability at 450 nm after an incubation of 2 h [Citation18].
Hoechst staining
NSCs (5 × 104) were transfected or treated with propofol and stained with the Hoechst stain (cell apoptosis Hoechst staining kit, Beyotime) [Citation19]. Briefly, these cells were removed from the culture medium and were fixed in 0.5 ml of the fixative solution for 10 min, washed twice with PBS (3 min each time). 0.5 ml of Hoechst 33258 staining solution was added and allowed to stain for 5 min. It was subsequently washed twice with PBS (3 min each time). Finally, the samples were photographed using a fluorescence microscope (OLYMPUS FV1000).
Cell transfection
Mouse miR-9-5p mimics (miR-9-5p), mimics NC (miR-NC), CXCR4 siRNA (siCXCR4), and scrambled siRNAs (siNC), all acquired from Hanbio Co., Ltd, were transiently transfected into the cells for 24 h at a concentration of 100 nM using jetPRIME (Polyplus Transfection) following the manufacturer’s instructions [Citation20].
Real-time polymerase chain reaction
Total RNA was extracted using the TRIzol reagent (Invitrogen). The reverse transcriptase-PCR and PCR primers for miR-9-5p, CXCR4, U6, and glyceraldehyde 3-phosphate dehydrogenase were obtained from General Biosystems Co., Ltd., and the primer sequences are shown in . The RNA concentration was measured using NanoDrop 2000 (ThermoFisher). The transcriptor first-strand cDNA synthesis kit (Roche, Basel, Switzerland) was used for cDNA synthesis. The following settings were used for each PCR cycle: 20 s at 50°C, 5 s at 95°C, and 30 s at 60°C for a total of 40 cycles. Relative quantification was calculated using the 2− ΔΔCt method [Citation21]. Quantitative reverse transcription-polymerase chain reaction (RT-qPCR) was performed using the SYBR Green kit (Roche) on an ABI Illumina instrument (StepOnePlusTM) according to the manufacturer’s instructions.
Table 1. Primer sequences of RT-qPCR
Western blotting
Cells were placed in the radioimmunoprecipitation assay lysis buffer (Beyotime) containing protease inhibitors (PMSF, Beyotime) then collected and lysed [Citation22]. The bicinchoninic acid assay kit (Beyotime) was used to determine the concentration of protein, and protein was transferred to the polyvinylidene fluoride membranes (Roche). The membranes were blocked using 5% nonfat milk in Tris-buffered saline, supplemented with 0.1% Tween-20 (TBST) to block membranes [Citation23]. The polyvinylidene fluoride membrane was incubated overnight at 4°C with primary antibodies specific for Bax (A00183, 1:1000, BOSTER), caspase-3 (PB9188, 1:1000, BOSTER), Bcl-2 (3498 T, 1:1000, CST), CXCR4 (ab181020, 1:1000, Abcam), and β-actin (TA-09, 1:1000, ZSGB-BIO). The membranes were incubated with secondary antibodies conjugated to horseradish peroxidase (ZB-2301 and ZB-2305, 1:5000, ZSGB-BIO) for 1 h at room temperature. Finally, the membranes were washed three times with TBST and visualized using the ECL detection reagent (Beyotime).
Statistical analysis
All trials were conducted in triplicates. GraphPad 9.0 (GraphPad Software) was used for statistical analysis. All experimental data are presented as the mean ± SD. We used the student’s t-test to analyze the differences between control and treated groups and the one-way analysis of variance for comparing multiple groups. Significance level was set at P< 0.05.
Result
The purpose of this study was to investigate the role of the miR-9-5p/CXCR4 signaling pathway in the neurotoxicity of the developing brain induced by propofol. We found that propofol-induced NSCs apoptosis by increasing miR-9-5p and CXCR4 expression. Additionally, we observed that reducing the CXCR4 expression can reverse the apoptosis of NSCs induced by propofol.
Propofol administration increased the miR-9-5p and CXCR4 levels in NSCs
NSCs were exposed to various concentrations of propofol for 6 h, and cell proliferation was estimated using the CCK-8 assay kit. The results showed that the inhibitory effect of propofol on NSC proliferation was dose-dependent, with significant inhibitory effects at high doses (100 μM for 6 h) (). Additionally, both cellular and animal experiments have indicated that propofol has certain neurotoxic effects at high doses [Citation24]. Therefore, we chose the high dose (100 μM) as the final concentration for the subsequent studies. To determine the expression of miR-9-5p and CXCR4, RT-qPCR and Western blot analyses were performed. The levels of miR-9-5p and CXCR4 were substantially higher in the propofol-treated NSCs (). According to the data of Western blot analysis, we found that propofol-treated NSC underwent apoptosis, which was confirmed by the decrease in the expression of anti-apoptotic factors such as Bcl-2 and the increase in the expression of apoptotic factors such as Bax and caspase-3 (P < 0.05) ().
Figure 1. Up-regulated expression of miR-9-5p and CXCR4 are found in NSCs under propofol treatment. (a) The viability of NSCs after 6 h of propofol treatment was examined by the CCK-8 assay kit. (b and c) The mRNA expression of miR-9-5p and CXCR4 in NSCs after 6 h of propofol treatment was examined by RT-qPCR. (d) The protein bands of CXCR4 and β-actin and protein expression of CXCR4 in NSCs after 6 h of propofol treatment were examined by Western blot analysis. (e) The protein expressions of Bax, caspase-3, and Bcl-2 were measured by Western blot analysis. The experiment was repeated three times; the significance of difference between means was analyzed for multiple comparisons by the analysis of variance; the data between two groups was analyzed using the student’s t-test, *P < 0.05; **P < 0.01, ***P< 0.001, ****P< 0.0001; CCK-8 kit, Cell Counting Kit-8; NSCs, neural stem cells; CXCR4, CXC chemokine receptor-4; miR-9-5p, microRNA-9-5p; RT-qPCR, reverse transcription-quantitative polymerase chain reaction; Bax, Bcl-2-associated X protein; Bcl-2, B-cell lymphoma 2.
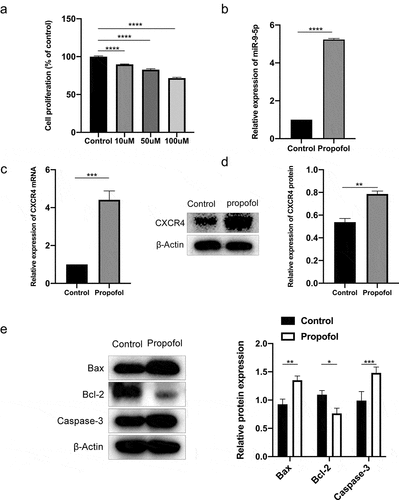
Propofol regulates CXCR4 expression through miR-9-5p
To explore and investigate the interactive relationship between miR-9-5p and CXCR4, we transfected NSCs with the NC mimic and miR-9-5p mimic. We then determined whether propofol-induced CXCR4 expression was dependent on miR-9-5p expression. The interference efficiency was then tested using RT-qPCR. We found that there was a significant increase in the miR-9-5p expression (P< 0.05) (), which was transfected with the miR-9-5p mimic. At the same time, when the expression of miR-9-5p increased, the expression of CXCR4 mRNA and protein also increased ( and ). These results indicated that propofol upregulates the CXCR4 expression by increasing the miR-9-5p expression.
Figure 2. miR-9-5p dependent induction of CXCR4 by propofol. (a and b) The expression of miR-9-5p and CXCR4 were examined by RT-qPCR. (c) The protein bands of CXCR4 and β-actin and protein expression of CXCR4 were examined by Western blot analysis. The experiment was repeated three times; the significance of the difference between means was analyzed for multiple comparisons by the analysis of variance; the data between two groups were analyzed using the student’s t-test, **P < 0.01, ***P < 0.001.
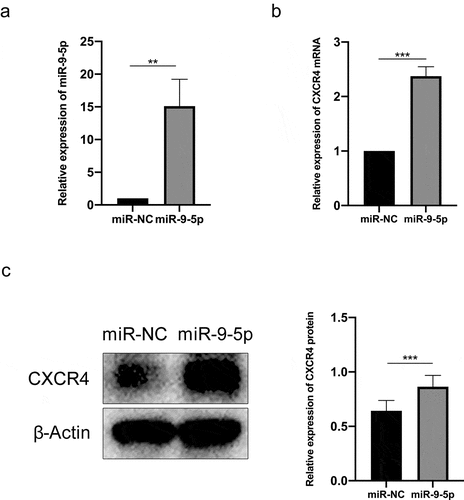
Propofol induces apoptosis of NSCs by upregulating miR-9-5p
The effect of miR-9-5p in propofol-mediated apoptosis of NSCs was explored by cell transfection with the NC mimic and miR-9-5p mimic. The results of the cell viability assay indicated that NSCs survival decreased when the expression of miR-9-5p increased (P< 0.05) (). The numbers of apoptotic NSCs increased in the miR-9-5p group, which mainly showed nuclear staining, nuclear concentration, and fragmentation (P < 0.05) (). Likewise, Western blot analysis further verified that the protein expression of Bax and caspase-3 increased through the over-expression of miR-9-5p, whereas the protein expression of Bcl-2 decreased (P < 0.05) (). These results indicate that propofol induces NSC apoptosis by upregulating miR-9-5p.
Figure 3. miR-9-5p promotes the apoptosis of propofol-treated NSCs. (a) The viability of propofol-treated NSCs after the overexpression of miR-9-5p was examined by a CCK-8 assay. (b) The effect of miR-9-5p overexpression on apoptosis of propofol-treated NSCs was examined by Hoechst staining. (c) the protein expression of Bax, caspase-3and Bcl-2 measured by Western blot analysis. The experiment was repeated three times; the significance of the difference between means was analyzed for multiple comparisons by the analysis of variance; the data between two groups were analyzed using the student’s t-test, *P< 0.05, ***P< 0.001, ****P < 0.0001.
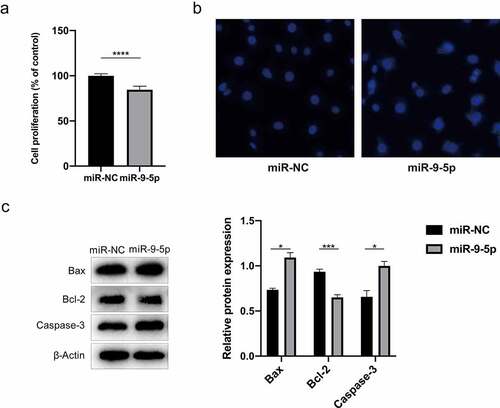
Knockdown of CXCR4 partially attenuates the propofol-induced NSCs Death
To explore the role of CXCR4 in propofol-induced NSC apoptosis, we transfected CXCR4 siRNAs or scrambled siRNAs into the c17.2 cells for 24 h to knock down CXCR4 or to create a scramble group. We assessed the knockdown efficiency using qRT-PCR. As shown in and , CXCR4 expression decreased in the NSCs compared to in the control group when using the CXCR4 siRNA. (P< 0.05).
Figure 4. CXCR4 silencing inhibits the apoptosis of propofol-treated NSCs. (a) The mRNA expression of CXCR4 after CXCR4 silencing was examined by RT-qPCR. (b) The protein expression of CXCR4 after CXCR4 silencing was examined by Western blot analysis. (c) Viability of propofol-treated NSCs after CXCR4 silencing was examined by the CCK-8 assay kit. (d) The apoptosis of propofol-induced NSCs after CXCR4 silencing was examined by Hoechst staining. (e) The protein expressions of Bax, caspase-3, and Bcl-2 were measured by Western blot analysis. The experiment was repeated three times; the significance of the difference between means was analyzed for multiple comparisons by the analysis of variance; the data between two groups were analyzed using the student’s t-test, **P< 0.01, ***P< 0.001, ****P< 0.0001.
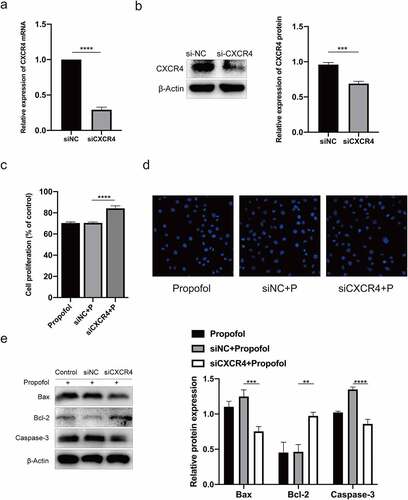
To further assess the role of CXCR4 in propofol-induced apoptosis, NSCs were exposed to 100 μM propofol for 6 h after being transfected with CXCR4 or scramble siRNA for 24 h. CCK-8 assay showed that the survival rate of NSCs in the CXCR4 siRNA group was enhanced when compared with the NC siRNA group (). Moreover, Hoechst staining and Western blot assay were used to test the effect of CXCR4 siRNA on the apoptosis of propofol-treated NSCs. It also revealed that the apoptosis rate in propofol-treated NSCs that were treated with CXCR4 siRNA decreased. Meanwhile, the protein expression of Bax and caspase-3 reduced, and Bcl-2 protein expression increased (P < 0.05) ( and ). In general, the above results demonstrated that CXCR4 siRNA inhibited apoptosis of propofol-treated NSCs.
As a specific antagonist of CXCR4, plerixafor (AMD3100) can also protect NSCs from propofol-induced neurotoxicity. NSCs were pretreated with 20 μM of AMD3100 for 1 h, and 100 μM of propofol was then added for 6 h, according to the protocol by Gao et al [Citation25]. After AMD3100 administration, the CCK-8 assay revealed that the survival rate of NSCs increased (). Meanwhile, Hoechst staining and Western blot assay demonstrated that the amount of apoptosis decreased, along with a reduction in the CXCR4, Bax, and caspase-3 expression; an increase in the Bcl-2 in protein expression was also observed ( and ). These results indicate that AMD3100 can alleviate apoptosis in propofol-treated NSCs.
Figure 5. Pretreatment of AMD3100 promotes the inhibition of apoptosis in propofol-treated NSCs. (a) Viability of propofol-induced NSCs after AMD3100 pretreating was examined by the CCK-8 assay kit. (b) The apoptosis of propofol-induced NSCs after AMD3100 pretreating was examined by Hoechst staining. (c) The protein expressions of CXCR4, Bax, caspase-3, and Bcl-2 were measured by Western blot analysis. The experiment was repeated three times; the significance of the difference between means was analyzed for multiple comparisons by the analysis of variance ; the data between two groups were analyzed using the student’s t-test, *P < 0.05, **P < 0.01, ***P < 0.001, ****P < 0.0001.
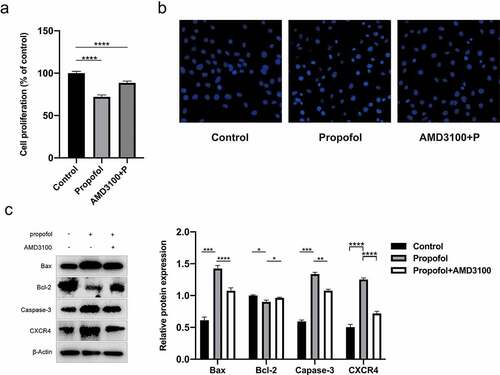
Discussion
This study provides some novel findings. First, propofol induces NSC neurotoxicity via the miR-9-5p/CXCR4 signaling pathway. Second, the inhibition of CXCR4 by siRNA or its specific inhibitor AMD3100, which can protect NSCs from the propofol-induced neurotoxicity. In short, the findings of our study not only help us understand the potential mechanism of propofol neurotoxicity in NSCs but also provide us with potential drug target sites that can be used to prevent propofol-induced neurotoxicity.
Studies have shown that late learning disorders can be caused by anesthetics, including propofol, early in life [Citation4,Citation26,Citation27]. Additionally, compared to control cells, sufficient cell death was induced when cultured neonatal rat primary hippocampal neurons were exposed for 6 h at 50 μM propofol [Citation28]. Additionally, studies have indicated that this phenomenon occurs in pregnant mice with single intraperitoneal injection propofol. Neural stem cells mainly exist in the developing brain and have the function of self-renewal and multi-functional differentiation. When stem cells stop renewing themselves and differentiating into specific types of neurons [Citation29], they first show up in the developing brain, in a process called neurogenesis that can be precisely regulated to produce the complex structures in the brain. Propofol impairs NSC proliferation and differentiation 6 h after administration [Citation30]. Exposure of rat NSCs to 20 μg/ml propofol for 6 h significantly inhibited rat NSC proliferation, neuronal differentiation, and migration [Citation8]. In this study, we treated NSCs with 10 μM, 50 μM, and 100 μM propofol for 6 h. It was found that the survival rate of neural stem cells decreased significantly at 100 μM propofol concentration, which was consistent with the experimental results of Huang et al [Citation17].
MicroRNAs are non-coding RNAs that participate in many physiological and pathological processes, and nearly 70% of miRNAs exist in the central nervous system [Citation31]. miR-184 participates in the process of cerebral ischemia by acting on phosphatidic acid phosphatase type 2 B [Citation32]. MicroRNA-378 regulates the proliferation and differentiation of NSCs by regulating the expression of Tailless [Citation33]. Many studies have shown that miRNAs are involved in propofol-induced neurotoxicity. MiR-363-3p was reported to participate in increasing the neuronal oxidative stress and apoptosis induced by propofol by targeting cyclic adenosine monophosphate response element-binding protein [Citation34]. Twaroski et al. found that propofol damages the human stem cell-derived neurons through the STAT3/miR-21/Sprouty 2/Akt pathway [Citation35]. In addition, miR-206 is involved in propofol-induced neurotoxicity in the human embryonic stem cells by regulating the p53-upregulated modulator of apoptosis [Citation36]. In this study, we found that propofol treatment increased the expression of miR-9-5p. When miR-9-5p mimics were added to neural stem cells, the expression of apoptotic proteins such as caspase-3 and Bax increased, the expression of anti-apoptotic protein Bcl-2 decreased, and the cell survival rate decreased. These results suggest that miR-9-5p mimics have similar effects to propofol; propofol damages NSCs through miR-9-5p. Our findings are consistent with those of previous reports, which showed that miR-9 played a similar role in the inhibition of embryonic stem cell self-renewal by isoflurane [Citation37].
CXCR4 is a G protein-coupled receptor located on the surface of the cell membrane. Studies have found that CXCR4 exists in the NSCs and participates in maintaining the stemness of NSCs together with CXCL12 [Citation38]. In addition, CXCR4 is involved in traumatic brain injury, where blocking CXCR4 improved cognition in mice [Citation39]. Lung cancer cells that were treated with 4 μg/mL propofol for 2 h significantly inhibited the lung cancer cell proliferation and led to a decrease in cell viability, which is related to the decrease in CXCR4 expression [Citation15]. In this study, we found that CXCR4 was markedly upregulated following its exposure to 100 μM propofol for 6 h, and overexpression of miR-9-5p also increased the expression of CXCR4 in neural stem cells, suggesting that propofol increases CXCR4 expression through miR-9-5p. In addition, targeting CXCR4 by siRNA transfection or AMD3100 pretreatment reversed decreased cell survival, increased number of apoptotic cells, increased the protein expression of Bax and caspase-3, and decreased Bcl-2 protein expression induced by propofol in NSCs. These results suggest that propofol causes neural stem cell apoptosis through the miR-9-5p/CXCR4 pathway, and inhibition of CXCR4 can reverse the propofol-induced neurotoxicity.
In summary, our study found that propofol application substantially increased the expression of miR-9-5p and CXCR4. miR-9-5p overexpression induces NSCs death, and CXCR4 knockdown has a protective effect on the neurotoxicity induced by propofol on NSCs. These results demonstrate that the miR-9-5p/CXCR4 pathway is crucial for NSC death.
Conclusion
Our study showed that the expression of miR-9-5p and CXCR4 increased after propofol treatment in c17.2 cells, and the neurotoxic effect of propofol can be reversed by reducing CXCR4. In the future, miR-9-5p may serve as a diagnostic marker of propofol neurotoxicity. In addition, CXCR4 can be used as an intervention target to reduce cognitive impairment caused by propofol.
Highlights
Propofol induces NSCs apoptosis through miR-9-5p/CXCR4 signaling pathway.
Overexpression of miR-9-5p can aggravate the apoptosis of NSCs induced by propofol.
Inhibition of CXCR4 expression can reverse the apoptosis of NSCs induced by propofol.
AMD3100 is a promising therapeutic drug to inhibit the neurotoxicity of propofol.
Acknowledgements
Thanks for all the contributors and participants.
Disclosure statement
No potential conflict of interest was reported by the author(s).
Additional information
Funding
References
- Chidambaran V, Costandi A, D’Mello A. Correction to: propofol: a review of its role in pediatric anesthesia and sedation. CNS Drugs. 2018;32:873.
- Chang J, Yan X, Zeng Y. RETRACTED: propofol weakens hypoxia-aroused apoptosis and autophagy via elevating microRNA-137 in neurocytes. Exp Mol Pathol. 2020;112:104327. doi:10.1016/j.yexmp.2019.104327.
- Olutoye OA, Baker BW, Belfort MA, et al. FDA warning on anesthesia and brain development: implications for obstetric and fetal surgery. Am J Clin Exp Obstet Gynecol. 2017;218(1):98–102. doi:10.1016/j.ajog.2017.08.107.
- Pearn ML, Schilling JM, Jian M, et al. Inhibition of RhoA reduces propofol-mediated growth cone collapse, axonal transport impairment, loss of synaptic connectivity, and behavioural deficits. Br J Anaesth. 2018;120:745–760.
- Choi H, Choi NY, Park HH, et al. Sublethal doses of zinc protect neural stem cells against hypoxia through activation of the PI3K pathway. Stem Cells Dev. 2019. DOI:10.1089/scd.2018.0138
- Liang C, Du F, Cang J, et al. Pink1 attenuates propofol-induced apoptosis and oxidative stress in developing neurons. J Anesth. 2018;32:62–69.
- Cao J, Li Y, Zeng F, et al. Propofol exposure disturbs the differentiation of rodent neural stem cells via an miR-124-3p/Sp1/Cdkn1b axis. Front Cell Dev Biol. 2020;8. DOI:10.3389/fcell.2020.00838
- Jiang Q, Wang Y, Shi X. Propofol inhibits neurogenesis of rat neural stem cells by upregulating MicroRNA-141-3p. Stem Cells Dev. 2017;26:189–196.
- Hu Q, Huang L, Zhao C, et al. Ca2+-PKCα-ERK1/2 signaling pathway is involved in the suppressive effect of propofol on proliferation of neural stem cells from the neonatal rat hippocampus. Brain Res Bull. 2019. DOI:10.1016/j.brainresbull.2019.04.005.
- Packer AN, Yi X, Harper SQ, et al. The bifunctional microRNA miR-9/miR-9* regulates REST and CoREST and is downregulated in Huntington’s disease. J Neurosci. 2008;28:14341–14346.
- Delaloy C, Lei L, Lee JA, et al. MicroRNA-9 coordinates proliferation and migration of human embryonic stem cell-derived neural progenitors. Cell Stem Cell. 2010;6:323–335.
- Chen ML, Hong CG, Yue T, et al. Inhibition of miR-331-3p and miR-9-5p ameliorates Alzheimer’s disease by enhancing autophagy. Theranostics. 2021;11:2395–2409.
- Wang Z, Sun L, Jia K, et al. miR-9-5p modulates the progression of Parkinson’s disease by targeting SIRT1. Neurosci Lett. 2019;701:226–33 .
- Monika R, Joerg H, Antje W, et al. Osteopontin mediates survival, proliferation and migration of neural stem cells through the chemokine receptor CXCR4. Stem Cell Res Ther. 2015;6:99 .
- Hu C, Iwasaki M, Liu Z, et al. Lung but not brain cancer cell malignancy inhibited by commonly used anesthetic propofol during surgery: implication of reducing cancer recurrence risk. J Adv Res. 2021. DOI:10.1016/j.jare.2020.12.007
- Yi J, Gao ZF. MicroRNA-9-5p promotes angiogenesis but inhibits apoptosis and inflammation of high glucose-induced injury in human umbilical vascular endothelial cells by targeting CXCR4. Int J Biol Macromol. 2019. DOI:10.1016/j.ijbiomac.2019.02.003
- Huang J, Jing S, Chen X, et al. Propofol administration during early postnatal life suppresses hippocampal neurogenesis. Mol Neurobiol. 2016;53:1031–1044.
- Yao Y, Li X, Cheng L, et al. Circular RNA FAT atypical cadherin 1 (circFAT1)/microRNA-525-5p/spindle and kinetochore-associated complex subunit 1 (SKA1) axis regulates oxaliplatin resistance in breast cancer by activating the notch and Wnt signaling pathway. Bioengineered. 2021;12:4032–4043.
- Cui Y, Pu R, Ye J, et al. LncRNA FAM230B promotes gastric cancer growth and metastasis by regulating the miR-27a-5p/TOP2A axis. Dig Dis Sci. 2021;66:2637–50 .
- Dateki M, Imamura O, Arai M, et al. A novel strategy for selective gene delivery by using the inhibitory effect of blue light on jetPRIME‐mediated transfection. Biotechnol Bioeng. 2016;113:1560–1567.
- Kjl A, Tds B. Analysis of relative gene expression data using real-time quantitative PCR and the 2 ΔΔ C T method. Methods. 2001;25:402–408.
- Dai L, Jie S, Bi S, et al. Angiopoietin-2 silence alleviates lipopolysaccharide-induced inflammation, barrier dysfunction and endoplasmic reticulum stress of intestinal epithelial cells by blocking Notch signaling pathway. Bioengineered. 2021. DOI:10.1080/21655979.2021.1985341
- Qiong L, Yin J. Orosomucoid 1 promotes epirubicin resistance in breast cancer by upregulating the expression of matrix metalloproteinases 2 and 9. Bioengineered. 2021. DOI:10.1080/21655979.2021.1987067
- Jiang L, Yang F, Zhao Q, et al. MicroRNA-665 mediates propofol-induced cell apoptosis in human stem cell-derived neurons. Bioengineered. 2019;10:493–500.
- Gao M, Dong Q, Yao H, et al. Induced neural stem cells modulate microglia activation states via CXCL12/CXCR4 signaling. Brain Behav Immun. 2017;59:288–99.
- Wang X, Dong Y, Zhang Y, et al. Sevoflurane induces cognitive impairment in young mice via autophagy. PLoS ONE. 2019;14:e0216372.
- Liu J, Zhao Y, Yang J, et al. Neonatal repeated exposure to isoflurane not sevoflurane in mice reversibly impaired spatial cognition at juvenile-age. Neurochem Res. 2017;42:595.
- Zhu X, Li H, Tian M, et al. miR-455-3p alleviates propofol-induced neurotoxicity by reducing EphA4 expression in developing neurons. Biomarkers. 2020;25:685–692.
- Zhao C, Sun GQ, Li S, et al. MicroRNA let-7b regulates neural stem cell proliferation and differentiation by targeting nuclear receptor TLX signaling. Proc Natl Acad Sci U S A. 2010;107:1876–1881.
- Chao L, Fang J, Wang J, et al. Propofol regulates neural stem cell proliferation and differentiation via calmodulin-dependent protein kinase II/AMPK/ATF5 signaling axis. Anesth Analg. 2019;129:608–17 .
- Wu J, He J, Tian X, et al. microRNA‐9‐5p alleviates blood–brain barrier damage and neuroinflammation after traumatic brain injury. J Neurochem. 2020;153:710–26.
- Yang H, Zhang Y, Chen H, et al. Mir-184 contributes to brain injury through targeting PPAP2B following ischemic stroke in male rats. Front Mol Neurosci. 2021;14:613887.
- Huang Y, Liu X, Wang Y. MicroRNA-378 regulates neural stem cell proliferation and differentiation invitro by modulating tailless expression. Biochem Biophys Res Commun. 2015;466:214–220.
- Yao Y, Zhang J. Propofol induces oxidative stress and apoptosis in vitro via regulating miR-363-3p/CREB signalling axis. Cell Biochem Funct. 2020;38:1119–1128.
- Twaroski D, Yan Y, Olson J, et al. Down-regulation of microRNA-21 is involved in the propofol-induced neurotoxicity observed in human stem cell-derived neurons. Anesthesiology. 2014;121:786–800.
- Li Y, Jia C, Zhang D, et al. Propofol-induced neurotoxicity in hESCs involved in activation of miR-206/PUMA signal pathway. Cancer Biomarkers. 2017;20:175–181.
- Lei Z, Ying Z, Hu R, et al. Isoflurane inhibits embryonic stem cell self-renewal and neural differentiation through miR-9/E-cadherin signaling. Stem Cells Dev. 2015;24:1912.
- Ho SY, Ling TY, Lin HY, et al. SDF-1/CXCR4 signaling maintains stemness signature in mouse neural stem/progenitor cells. Stem Cells Int. 2017 Mar 20;2017:2493752.
- Friedman-Levi Y, Liraz-Zaltsman S, Chen S, et al. Pharmacological blockers of CCR5 and CXCR4 improve recovery after traumatic brain injury. Exp Neurol. 2021;338:113604.