ABSTRACT
Monocrotaline (MCT)-induced pulmonary artery hypertension (PAH) has been reported to cause right heart failure (RHF). Moreover, Right heart diseases have been determined to cause ventricular arrhythmia (VA). So we can conclude that MCT-induced PAH increases the incidence of VA. In addition, Previous studies have determined the benefits of Dapagliflozin (DA) on the cardiac system, but the responses of MCT-induced RHF to DA are not fully reported. So the present study sought to evaluate the effects of DA on the MCT-induced PAH. A dose intraperitoneal injection of MCT (60 mg/kg) was carried out to induce a rat model with PAH. DA (60 mg/l) was administered for 4 weeks following MCT injection. Echocardiography, body weight, blood pressure, blood glucose, electrophysiological study, and Western blot were performed. Four weeks after the MCT injection, MCT-treated rats decreased body weight, blood glucose and blood pressure. In addition, MCT caused the formation of PAH and RHF. Moreover, MCT-induced PAH rats increased the incidence of VA, prolonged action potential duration (APD), and shortened effective refractory period (ERP). Additionally, PAH rats significantly prevented the activated expressions of Ion channel proteins such as potassium channel (Kv1.5, Kv2.1, Kv4.2, Kv4.3) and L-type Ca channel (Cav1.2). As we expected, these changes above in PAH rats were reversed when DA was administered. Mechanistically, DA significantly reduced the levels of toll-like receptor (TLR4), the nuclear factor kappa B (NF-κB) in MCT-treated rats. In conclusion, these findings determine that DA reduces the vulnerability of VA in PAH rats through the TLR4/NF-κB signaling pathway.
1. Introduction
Pulmonary arterial hypertension (PAH), characterized by excessive vasoconstriction and vascular remodeling, causes right heart failure (RHF) and death [Citation1–3]. Moreover, RHF is one of major causes of death in patients with PAH. Although there have been advances in the causes of HF and related devices and pharmacological therapeutics, the mortality rate of people with HF remains high, with almost up to 50% of sudden death in patients [Citation4,Citation5]. Ventricular arrhythmia, including ventricular fibrillation (VF) and ventricular tachycardia (VT), accounts for nearly 60% of mortality in patients with HF [Citation6–9].
According to previous reports, the electrical and structural remodeling of the ventricle are the basic mechanisms of VA. Ventricular hypertrophy and fibrosis belong to the structural remodeling [Citation10,Citation11]. The electrical remodeling includes prolonged APD, shorted ERP and ion channels remodeling [Citation12,Citation13]. Previous study suggested that structural and electrical remodeling were the major reasons for ventricular arrhythmia in PAH rats [Citation14]. As previous reports suggest, activated TLR4/NF-κB signaling pathway promotes the ventricular electrical remodeling and structural remodeling, which increase the vulnerability of VA [Citation15–17]. So the treatment for ventricular remodeling is beneficial for PAH-induced ventricular arrhythmia.
Dapagliflozin (DA), as one of sodium-glucose cotransporter 2 (SGLT2) inhibitors, has recently been determined to reduce worsening heart failure and ventricular arrhythmia [Citation18–21]. However, few studies have assessed whether DA alleviates PAH-induced ventricular arrhythmia.
The present study aims to determine the effects of DA on MCT-induced PAH and whether it reduces the vulnerability of VA induced by PAH.
2. Materials and animals
An expanded Methods section describing all procedures and protocols can be found in the Supplemental Methods section.
2.1 Animal treatments
Eighty male Sprague Dawley (SD) rats (300–350 g) carried out in all experiments were provided by Renmin Hospital of Wuhan University’s animal experiment center. All animal procedures followed the Guide for the care and the use of Laboratory Animals, issued by the National Institutes of Health in 1996. The SD Rats were housed in conditions with 22 ± 2°C and a 12:12-h light/dark cycle, and water with food ad libitum were enough to be provided.
All rats were randomized into control (CTL) (n = 20), control+DA (CTL+DA) (n = 20), PAH (n = 20) or (PAH+DA) groups (n = 20). The PAH group and the PAH+DA group received a dose (60 mg/kg) Intraperitoneal injection of MCT [Citation22]. Monocrotaline (MCT) (98% purity, C835791) was obtained from Macklin Biochemical Co., Ltd (Shanghai, China). Simultaneously, the PAH+DA group and the CTL+DA group received DA administered by dissolving Dapagliflozin in drinking water at a concentration of 60 mg/L [Citation23] for 4 weeks While the other rats were fed normally. Dapagliflozin was purchased from AstraZeneca AB.
2.2 Blood glucose measurement
We measured the blood glucose of rats in per group weekly for a fixed time, using glucometer (ACCU-CHEK produced by Roche Diabetes Care GmbH) [Citation24]. And the blood samples were collected from the tail vein of 8–10 rats each group.
2.3 Blood pressure measurement
We measured the blood pressure of 8–10 rats in each group at the end of the fourth week through Blood Pressure Meter (BP-2010A, Softron Beijing Biotechnology) [Citation25].
2.4 Echocardiography
Eight to ten rats from each group were placed on an electrical heating pad under anesthesia (2% isoflurane/100% oxygen) with the supine position, and the echocardiographic assessment was conducted using the VINNO 6VET ultrasound system (Vinno Technology, Suzhou, Jiangsu, China), equipped with an 18-MHz linear array transducer (Vinno Technology, Suzhou, Jiangsu, China). We measured the cardiac function as previously described [Citation26]. We recorded the left ventricular ejection fraction (LVEF), left ventricular fractional shortening (LVFS) and so on. All the recorded results were shown in Supplementary Material Table S1.
2.5 Electrophysiological studies
Langendorff-perfused hearts were prepared according to our previously published methods [Citation24]. We analyzed and recorded APD and ERP. In addition, we used burst pacing to imply the vulnerability of ventricular arrhythmia. The APD was measured at 20% (APD20), 50% (APD50) and 90% (APD90) repolarization. All the procedures [Citation24] were shown in Supplementary Material.
2.6 Histology and histochemistry analysis: HE(hematoxylin-eosin) & Masson staining
H&E (hematoxylin-eosin) staining was conducted following previous protocols [Citation27]. In short, after the murine hearts were fixed with 4% formaldehyde overnight, they were embedded in paraffin and made into 5-μm sections. Cardiac paraffin sections were dewaxed in xylene for 5–10 minutes, and then dehydrated with anhydrous ethanol, 95% ethanol, 85% ethanol and 75% ethanol for 5 minutes each. After staining by Hematoxylin solution (Harris) for 3–5 minutes, the tissues were washing by 1% dilute lithium carbonate water solution back to blue. And then dyed the tissues in eosin (alcohol soluble) for 1–2 seconds without washing. Finally, the tissues were added into 95% ethanol directly and dehydrated in absolute ethanol for 1–2 minutes.
Masson staining was conducted according to previous methods [Citation28]. The tissues of the rats were quickly removed under anesthesia, and the blood was washed with PBS. The ventricular tissues and lung tissues were isolated and fixed with 4% of paraformaldehyde. The sample was imbedded into paraffin and cut into 3-mm-thick slices. The slices were stained with Masson’s trichrome staining and observed under an optical microscope (CX31, Olympus, Tokyo, Japan). The samples were observed at × 400, and three visual fields were tested in each sample. Results were analyzed with the Image-Pro 6.0 software.
2.7 Immunohistochemical analysis of lung tissues
Three-millimeter-thick sections were incubated overnight at 4°C, through using alpha-smooth muscle actin (α-SMA) (1:200 dilution for staining). The sections were washed 3 times at room temperature for 5 minutes each time, and it was incubated with a secondary antibody for 2 hours at room temperature after incubation with the primary antibodies. The samples were observed under the optical microscope (CX31, Olympus, Tokyo, Japan) at ×400, and three visual fields were tested randomly in each sample [Citation28]. Results were analyzed with the Image-Pro 6.0 software.
2.8 Western blot Assay of ventricular tissues
The Western blot Assay was conducted as previously described [Citation24]. Total proteins were extracted from the frozen heart tissues. Protein concentrations were determined and normalized using the Bicinchoninic Acid (BCA) Protein Assay Kit (G2026, Servicebio, Wuhan, China). Next, proteins (28 mg) were separated by sodium dodecylsulphate (SDS)-polyacrylamide gel electrophoresis (PAGE), then transferred onto a polyvinylidene difluoride (PVDF) membrane and incubated with primary antibodies overnight at 4°C (39.2°F). Finally, the secondary antibodies were incubated with the membranes for 60 minutes at room temperature. Enhanced chemiluminescence (BL523B, Biosharp, Anhui, China) was used to visualize the signals. The protein and gene expression levels of target genes were normalized to the internal reference gene GAPDH. Kv4.2 (1:1000 dilution for Western blot, ab123543) were purchased from Abcam (Cambridge, UK) while Kv1.5 (1:1000 dilution for Western blot, PA5-77,573), Kv2.1 (1:1000 dilution for Western blot, PA5-77,580), Kv4.3 (1:500 dilution for Western blot, PA5-77,583), and Cav1.2 (1:400 dilution for Western blot, PA5-77,297) were also obtained from Abcam. Besides, NF-κB (p65) (1:1000 dilution for Western blot, 8242S), P-NF-κB (P-p65) (1:1000 dilution for Western blot, 3031S), GAPDH (1:1000 dilution for Western blot, 7074S). And TLR4 (1:1000 dilution for Western blot, AF7017) were purchased from Affinity Biosciences (OH, USA).
2.9 Statistical analysis
We performed statistical analyses using GraphPad Prism software. Continuous variables are expressed as means ± the standard error of the mean (SEM). The student’s t-test determined statistical differences between two groups. Statistical comparisons among multiple groups were performed with a one-way analysis of variance (ANOVA), followed by Tukey’s post hoc test. Categorical data were reported as percentages and were analyzed by Fisher’s exact test. Significance was assumed when p < 0.05 or p < 0.01.
3. Results
The current study determined the effects of Dapagliflozin on PAH-induced ventricular arrhythmia and its underlying mechanism. Through our experiments, we found that DA reversed the structural and electrical remodeling of ventricles by preventing the activated signal pathway of TLR4/NF-κB, reducing the vulnerability of ventricular arrhythmia caused by PAH.
3.1 DA improved systolic function and improved the survival rate of PAH rats
During the 4 weeks, six rats from the PAH group (30%) and 3 from PAH+DA group (15%) were dead. There was no statistical difference between PAH group and PAH+DA group (p = 0.4506 > 0.05; ). During the formation of PAH, we found that rats changed for bad conditions. PAH rats eat food and drink water less than normal, so, the death rate was as expected. showed the analyzed morphometric parameters. The PAH group presented higher ventricular hypertrophy (heart weight and heart/tibia), RVW (right ventricular weight), (LV+S)W (left ventricular weight and interventricular weight), RVW/tibia, and (LV+S)W/tibia (p < 0.05 vs. CTL group). To our surprise, these changes were inhibited in PAH+DA group (p < 0.05 vs. PAH group). listed body weight ()), blood glucose ()) and blood pressure ()) of rats. We could find there were significant statistical differences between PAH group (p < 0.05 vs. CTL group) and PAH+DA group (p < 0.05 vs. PAH group). In addition, we found that DA down-regulated the body weight, blood pressure and blood glucose. The formation of PAHs also caused rats to down-regulate their body weight, blood pressure and blood glucose. Above all, we found that DA decreased the body weight but lessened the down-regulation of blood pressure or blood glucose. In Table S1, we listed the changes from ventricle to pulmonary vessel. We could find easily that the rats from the PAH group had formation of PAH and right heart failure. However, with the administration of DA, rats from PAH+DA group had a lot of improvement in cardiac function and pulmonary vessel. From all of the above, after the use of MCT, the hearts of PAH group rats changed seriously. By the way, these results suggested that the administration of Dapagliflozin lessened PAH-related symptoms. At the same time, from the above-mentioned data, we noticed that the hearts of PAH group became hypertrophied, which increased the incidence of ventricular arrhythmia.
Table 1. Dapagliflozin improved the ventricular hypertrophy
Figure 1. Effects of Dapagliflozin on percent survival of PAH’s rats (n = 20 per group). Data are presented as mean as ±SEM. *P < 0.05 vs. CTL group, #P < 0.05 vs. PAH group. **P < 0.01 vs. CTL group, ##P < 0.01 vs. PAH group.
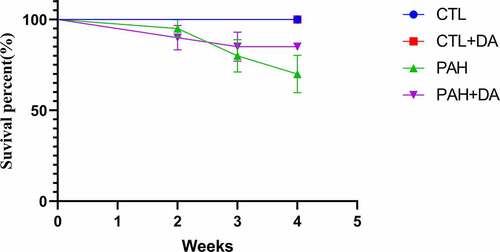
Figure 2. Effects of Dapagliflozin on the body weight, blood glucose and blood pressure. The body weight (a), The blood glucose (b), and the blood pressure (c) (n = 8–10 per group). Data are presented as mean as ±SEM. *P < 0.05 vs. CTL group, #P < 0.05 vs. PAH group. **P < 0.01 vs. CTL group, ##P < 0.01 vs. PAH group. Abbreviations: SBP, systolic blood pressure; DBP, diastolic blood pressure.
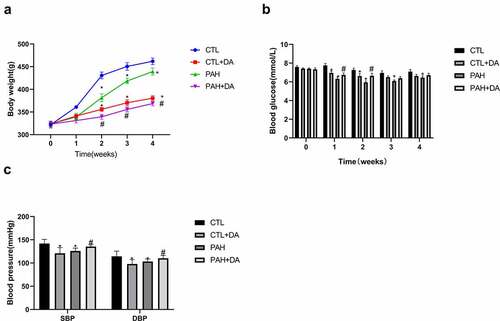
3.2 Dapagliflozin reduced the vulnerability of ventricular arrhythmia in the PAH rats
To identify the role of Dapagliflozin administration on VA’s vulnerability leaded by PAH, we used the burst pacing to stimulate rats. As we expected, there was no VA happening in the CTL group and the CTL+DA group ()). Despite this, it was obvious that VA was induced in both PAH group and PAH+DA group. From , there were still significant differences existing in the rate of VA ()) and the duration of VA ()) between PAH group and CTL group (80% vs. 0%, p < 0.05; 17.39 ± 2.759 vs. 0, p < 0.05). And the PAH+DA had improved a lot compared with PAH group (30% vs. 80%, p < 0.05; 2.567 ± 0.9609 vs. 17.39 ± 2.759, p < 0. 05). Therefore, from these results, we could see that the PAH increased the vulnerability of VA. And, the administration of Dapagliflozin prevented these changes.
Figure 3. Effects of Dapagliflozin on the vulnerability of ventricular arrhythmia in PAH’s rats. Representative images of burst pacing (a). Ratio of VA induced by burst pacing (b). Duration of VA in rats (c) (n = 8–10 each group). Data are presented as mean as ±SEM. *P < 0.05 vs. CTL group, #P < 0.05 vs. PAH group. **P < 0.01 vs. CTL group, ##P < 0.01 vs. PAH group. Abbreviations: NSR, normal sinus rhythm; VA, ventricular arrhythmia.
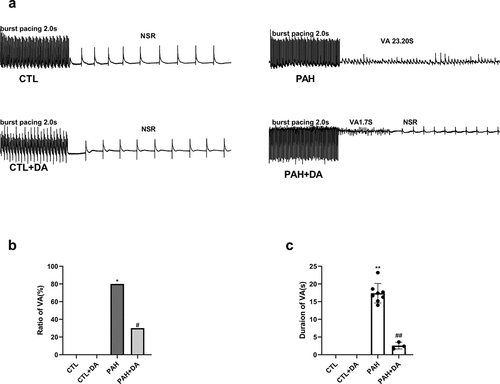
3.3 Effects of Dapagliflozin administration on PAHs rats in electrophysiological properties
We analyzed the ventricular electrophysiological remodeling in vivo hearts of rats. As we could see, there were no differences in CTL group and CTL+DA group, comparing APD20, APD50 and APD90 (for all, p > 0.05). As we imaged, from , we found that there were significant differences between APD20 (6.875 ± 1.356 vs. 19.63 ± 0.7440, p < 0.05), APD50 (15.25 ± 0.7071 vs. 43.50 ± 1.195, p < 0.05) and APD90 (64.0 ± 2.517 vs. 138.6 ± 5.350, p < 0.05) between CTL group and PAH group. In addition, we compared the rats from PAH group and the PAH+DA group. Surprisingly, we found the Dapagliflozin administrated shorted the APD20 (14.13 ± 0.6409 vs. 19.63 ± 0.7440, p < 0.05), APD50 (23.25 ± 1.165 vs. 43.50 ± 1.195, p < 0.05) and APD90 (91.14 ± 4.180 vs. 138.6 ± 5.350, p < 0.05). From ), we found the PAH group had a shorted ERP than CTL group (37.88 ± 2.1 vs. 61.63 ± 2.264, p < 0.05). With our expected, the Dapagliflozin administration improved these (46.25 ± 1.669 vs. 37.88 ± 2.1, p < 0.05). From these results above, we found that Dapagliflozin administration shorted the APD and prolonged the ERP. Above all these results, we can find with no difficult that Dapagliflozin administration prevented the electrical remodeling induced by PAH.
Figure 4. Effects of Dapagliflozin administration on the electrophysiological properties in PAH’s rats. Representative images of S1S1 stimulation (a). Analysis of APD20 (b), APD50 (c), and APD90 (d). Representative examples of ERP and analysis of ERP (e-f) (n = 8–10 per group). Data are presented as mean as ±SEM. *P < 0.05 vs. CTL group, #P < 0.05 vs. PAH group. **P < 0.01 vs. CTL group, ##P < 0.01 vs. PAH group. Abbreviations: APD20, action potential duration 20; APD50, action potential duration 50; APD90, action potential duration 90; ERP, effective refractory period.
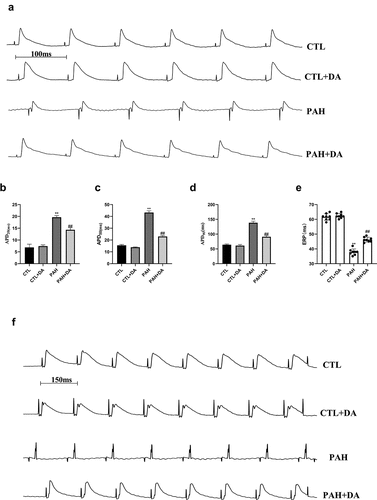
3.4 Dapagliflozin increased the expressions of ion channel proteins.
We can directly see the expressions of the ion channel proteins in rats from each group in ). And we also quantified the expressions of the channels of Cav1.2 ()), Kv1.5 ()), Kv2.1 ()), Kv4.2 ()) and Kv4.3 (). As we imaged, there were no statistical differences in CTL group and CTL+DA group. But, we found that the expression of all channels mentioned above had statistically different changes between PAH group and PAH+DA group (p < 0.05, for all). Dapagliflozin administration increased the expressions of the ion channel proteins.
Figure 5. Effects of Dapagliflozin administration on Ion channel proteins in PAH’s rats. Representative Western blots (a). Quantitative results of the Cav1.2 (b), Kv1.5 (c), Kv2.1 (d), Kv4.2 (e), Kv4.3 (f) (n = 3 per group). Data are presented as mean as ±SEM. *P < 0.05 vs. CTL group, #P < 0.05 vs. PAH group. **P < 0.01 vs. CTL group, ##P < 0.01 vs. PAH group.
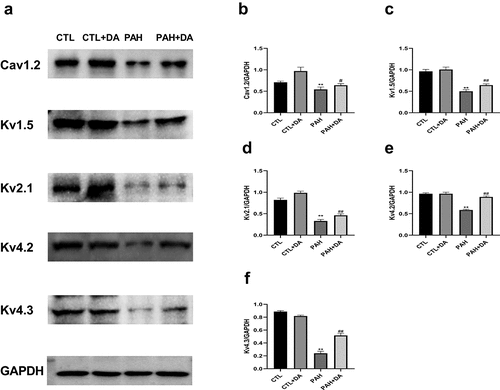
3.5 Dapagliflozin relieved the degrees of ventricular hypertrophy and fibrosis
From , it was clear that the PAH group had thickening of the pulmonary artery walls and more serious degree of the fibrosis, compared with the CTL group. These forcefully determined the conclusion of the results from and Table S1 that rats from PAH group had formed PAH. As we expected, when comparing PAH group with PAH+DA group, we found the amended results due to the use of Dapagliflozin. In ), it was obvious that the PAH group had more serious levels of hypertrophy and fibrosis, not only in the right ventricle but also in the left ventricle than in the CTL group. In addition, compared with PAH group, PAH+DA group had changes for the better. To analyze intuitively these results, we quantified these results. With no surprise, there were significant statistical differences in the CTL, PAH and PAH+DA groups (p < 0.05, for all). These further suggested that rats in the PAH group had ventricular hypertrophy and fibrosis during the formation of PAH induced by MCT. And, Dapagliflozin administration prevented these changes.
Figure 6. Effects of Dapagliflozin on the left pulmonary artery and the left superior pulmonary artery. Representative images of HE, Masson and α-SMA staining (a-b). Quantified results of Masson and α-SMA staining (c-f) (n = 3 per group). Data are presented as mean as ±SEM. *P < 0.05 vs. CTL group, #P < 0.05 vs. PAH group. **P < 0.01 vs. CTL group, ##P < 0.01 vs. PAH group. Abbreviations: HE, hematoxylin-eosin staining; Mas, Masson staining; α-SMA, alpha-smooth muscle actin; LPA, left pulmonary artery; LSPA, left superior pulmonary artery.
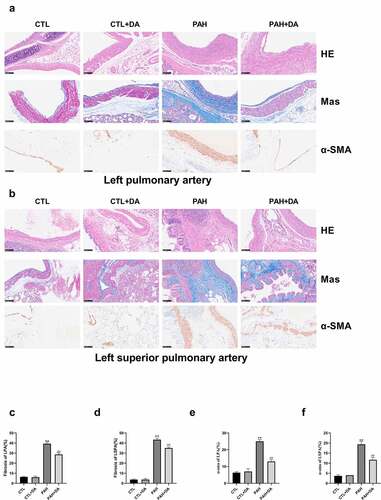
Figure 7. Effects of Dapagliflozin on the left ventricle and right ventricle. Representative results of HE and Masson staining (a). Quantitative results of Mas in right ventricle (b) and left ventricle (c) (n = 3 each group). Data are presented as mean as ±SEM. *P < 0.05 vs. CTL group, #P < 0.05 vs. PAH group. **P < 0.01 vs. CTL group, ##P < 0.01 vs. PAH group. Abbreviations: RV, right ventricle; LV, left ventricle.
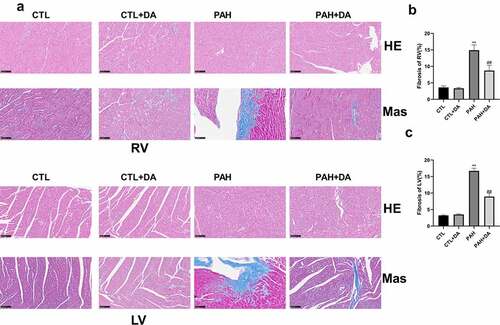
3.6 Dapagliflozin administration down-regulated the TLR4/NF-κB pathway
To determine the underlying pathway of the Dapagliflozin, we designed next experiment procedures. From ), we could find that the TLR4/NF-KB pathway was actively expressed in the PAH group. To better analyze the differences in each group, we quantified the expressions of TLR4 ()), P65 ()) and P-p65 (). From these figures, it was obvious that the expression of the pathway in PAH group was more active than the CTL group (p < 0.05, for all). Also, the PAH+DA group had a lower expression than the PAH group (p < 0.05, for all). Therefore we got the conclusion that Dapagliflozin administration reduced the incidence of VA induced by PAH by inhibiting the signaling pathway of TLR4/NF-κB.
Figure 8. Effects of Dapagliflozin on the expression of TLR4/P65 signal pathway. Representative Western blots (a). Quantitative results of TLR4 (b), P65 (c), P-p65 (d), p65/P-p65 (e) (n = 3 each group). Data are presented as mean as ±SEM. *P < 0.05 vs. CTL group, #P < 0.05 vs. PAH group. **P < 0.01 vs. CTL group, ##P < 0.01 vs. PAH group. Abbreviations: TLR4, toll-like receptor 4; p65, nuclear factor kappa B; P-p65, P-nuclear factor kappa B.
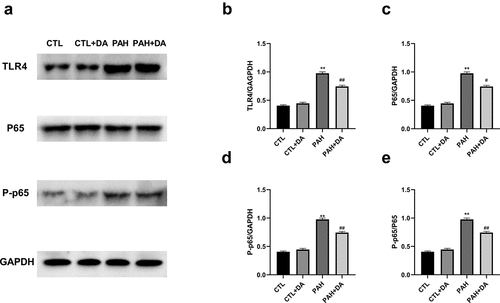
4. Discussion
In our study, we determined the effects and the mechanisms of Dapagliflozin on the vulnerability of ventricular arrhythmia in PAH rats. The major findings of our study were concluded below: 1) Under 28 days, rats in PAH group formed right heart failure, but, Dapagliflozin prevented the formation; 2) Dapagliflozin lessened the body weight but, up-regulated the blood glucose and the blood pressure in PAH’s rats; 3) Dapagliflozin reduced the vulnerability of ventricular arrhythmia and shortened the duration of VA-induced by burst pacing; 4) Dapagliflozin shorted the APD and prolonged the ERP; 5) Dapagliflozin up-regulated the expressions of the ion channel proteins; 6) Dapagliflozin prevented the ventricular hypertrophy and fibrosis; 7) Dapagliflozin inhibited the activation of the TLR4/NF-κB signaling pathway.
To inquire into the mechanisms of Dapagliflozin on the vulnerability of VA-induced by PAH, MCT-induced PAH models were usually used according to previous reports [Citation29–35]. As reported previously, the PAH caused ventricular hypertrophy, ventricular fibrosis, and right heart failure. In the present study, we found the formation of PAH and right heart failure in the rats from the PAH group, which suggested that the establishment of our study model was successful. It was similar to other reports [Citation36,Citation37]. According to previous reports, Dapagliflozin could down-regulate body weight, blood glucose and blood pressure in normal conditions [Citation38,Citation39]. In our present study, we found that Dapagliflozin also down-regulated body weight, blood pressure and blood glucose by comparing the CTL group with CTL+DA group, which were highly similar to previous studies. As we all know, PAH could lead to bad conditions for rats, which caused the down-regulation of body weight, blood pressure, and blood glucose. However, Dapagliflozin made the conditions of rats changing for the better, which may prevent the down-regulation caused by PAH. So, by comparing PAH group with PAH+DA group, we found that Dapagliflozin administration up-regulated the blood glucose and blood pressure but lessened the body weight.
Like previous reports, Dapagliflozin had a 27% reduction of VA [Citation40], which provided powerful support for our study. More and more emerging clinical data investigated that Dapagliflozin could improve vascular endothelial function and decreased oxidative stress [Citation41]. In addition, it also ameliorated myocardial fibrosis and improved cardiac diastolic and systolic functions [Citation42–44]. According to a previous meta-analysis, Dapagliflozin decreased 27% rate of the induction of VT [Citation40]. In our study, we also found that Dapagliflozin obviously reduced the vulnerability of VA induced by PAH, determining that Dapagliflozin was beneficial for preventing VA caused by PAH.
According to previous reports, low expressions of ion channel proteins like Kv1.5, Kv2.1, Kv4.2, Kv4.3 and Cav1.2 may cause prolonged APD and shorted ERP, which lead to the conduction abnormalities and reentry [Citation15,Citation17,Citation24]. In the present study, with Dapagliflozin administered, these electrical changes were reversed.
Fibrosis and hypertrophy are hallmarks of pathological ventricular remodeling, which contributed cardiac dysfunction and arrhythmogenesis [Citation45,Citation46]. According to previous reports, Dapagliflozin could prevent hypertrophy and fibrosis [Citation47,Citation48]. In the present study, we also found that DA obviously inhibited the ventricular hypertrophy and fibrosis.
It is well known that TLR4 is involved in the development of ventricular remodeling and heart failure [Citation49,Citation50]. In addition, previous study has proven that TLR4 knockout improved cardiac functions and prevented the ventricular remodeling [Citation51]. As a downstream mediator of TLR4, NF-κB is also related with the ventricular remodeling [Citation24]. Our current studies have suggested that it could reduce the vulnerability of VA through preventing TLR4/NF-κB signaling pathway [Citation15,Citation24]. In the present study, Dapagliflozin significantly reduced the levels of TLR4/NF-κB pathway. Therefore, these results determined that Dapagliflozin reversed the structural and electrical remodeling of ventricle through inhibiting TLR4/NF-κB pathway.
A limitation of our study is that we could not explore further the mechanisms in vitro due to the conditions of laboratory. The exploration of Nav1.2 and another ion channel proteins will be shown in our next studies. Additionally, different signal pathways are also worth determining.
5. Conclusion
Dapagliflozin could prevent the activated expressions of TLR4/NF-κB signal pathway, reversing the structural and electrical remodeling. These suggested that Dapagliflozin may reduce the vulnerability of ventricular arrhythmia through regulation of TLR4/NF-κB signal pathway.
Highlights
MCT-induced PAH caused the formation of RHF.
RHF increased the vulnerability of VA.
DA inhibited the electrical and structural remodeling.
DA may protect these changes against the activation of TLR4/NF-κB signaling pathway.
Author contribution
HH designed the experiment and supervised the project. TQ and BK performed the experiments and wrote the draft. WS participated in the discussion, and revision of the draft. JF, CD, and ZX edited the language and figure. All authors contributed to the article and approved the submitted version.
Supplemental Material
Download Zip (17.4 KB)Disclosure statement
No potential conflict of interest was reported by the author(s).
Supplementary Material
Supplemental data for this article can be accessed here.
Additional information
Funding
References
- McLaughlin VV, Archer SL, Badesch DB, et al. ACCF/AHA 2009 expert consensus document on pulmonary hypertension: a report of the American college of cardiology foundation task force on expert consensus documents and the American Heart Association: developed in collaboration with the American college of chest physicians, American thoracic society, Inc., and the pulmonary hypertension association. Circulation. 2009;119(16):2250–2294.
- Stacher E, Graham BB, Hunt JM, et al. Modern age pathology of pulmonary arterial hypertension. Am J Respir Crit Care Med. 2012;186(3):261–272.
- McLaughlin VV, Shah SJ, Souza R, et al. Management of pulmonary arterial hypertension. J Am Coll Cardiol. 2015;65(18):1976–1997.
- Fang JC, Ewald GA, Allen LA, et al. Advanced (stage D) heart failure: a statement from the Heart Failure Society of America guidelines committee. J Card Fail. 2015;21(6):519–534.
- Fleisher LA, Fleischmann KE, Auerbach AD, et al. ACC/AHA guideline on perioperative cardiovascular evaluation and management of patients undergoing noncardiac surgery: executive summary: a report of the American College of Cardiology/American Heart Association task force on practice guidelines. Circulation. 2014;130(24):2215–2245.
- Carson P, Anand I, et al. Mode of death in advanced heart failure: the comparison of medical, pacing, and defibrillation therapies in heart failure (COMPANION) trial. J Am Coll Cardiol. 2005;46(12):2329–2334.
- Cygankiewicz I, Zareba W, Vazquez R, et al. Heart rate turbulence predicts all-cause mortality and sudden death in congestive heart failure patients. Heart Rhythm. 2008;5(8):1095–1102.
- Huikuri HV, Castellanos A, Myerburg RJ. Sudden death due to cardiac arrhythmias. N Engl J Med. 2001;345(20):1473–1482.
- Teerlink JR, Jalaluddin M, Anderson S, et al. Ambulatory ventricular arrhythmias in patients with heart failure do not specifically predict an increased risk of sudden death. PROMISE (Prospective randomized Milrinone survival evaluation) investigators. Circulation. 2000;101(1):40–46.
- Brilla CG, Maisch B, Weber KT. Myocardial collagen matrix remodelling in arterial hypertension. Eur Heart J. 1992;13(Suppl D):24–32.
- Burchfield JS, Xie M, Hill JA. Pathological ventricular remodeling: mechanisms: part 1 of 2. Circulation. 2013;128(4):388–400.
- Wasson S, Reddy HK, Dohrmann ML. Current perspectives of electrical remodeling and its therapeutic implications. J Cardiovasc Pharmacol Ther. 2004;9(2):129–144.
- Yang KC, Nerbonne JM. Mechanisms contributing to myocardial potassium channel diversity, regulation and remodeling. Trends Cardiovasc Med. 2016;26(3):209–218.
- Martin B, Vanderpool RR, Henry BL, et al. Relaxin inhibits ventricular arrhythmia and asystole in rats with pulmonary arterial hypertension. Front Cardiovasc Med. 2021;8:668222.
- Jiang X, Kong B, Shuai W, et al. Loss of MD1 exacerbates myocardial ischemia/reperfusion injury and susceptibility to ventricular arrhythmia. Eur J Pharmacol. 2019;844:79–86.
- Wang Y, Hu H, Yin J, et al. TLR4 participates in sympathetic hyperactivity post-MI in the PVN by regulating NF-κB pathway and ROS production. Redox Biol. 2019;24:101186.
- Shuai W, Kong B, Fu H, et al. Loss of MD1 increases vulnerability to ventricular arrhythmia in diet-induced obesity mice via enhanced activation of the TLR4/MyD88/CaMKII signaling pathway. Nutr Metab Cardiovasc Dis. 2019;29(9):991–998.
- McMurray J, Solomon SD, Inzucchi SE, et al. Dapagliflozin in patients with heart failure and reduced ejection fraction. N Engl J Med. 2019;381(21):1995–2008.
- Packer M, Anker SD, Butler J, et al. Cardiovascular and renal outcomes with Empagliflozin in heart failure. N Engl J Med. 2020;383(15):1413–1424.
- Zannad F, Ferreira JP, Pocock SJ, et al. SGLT2 inhibitors in patients with heart failure with reduced ejection fraction: a meta-analysis of the EMPEROR-Reduced and DAPA-HF trials. Lancet. 2020;396(10254):819–829.
- Curtain JP, Docherty KF, Jhund PS, et al. Effect of dapagliflozin on ventricular arrhythmias, resuscitated cardiac arrest, or sudden death in DAPA-HF. Eur Heart J. 2021;42(36):3727–3738.
- Hiram R, Naud P, Xiong F, et al. Right atrial mechanisms of atrial fibrillation in a rat model of right heart disease. J Am Coll Cardiol. 2019;74(10):1332–1347.
- Joannides CN, Mangiafico SP, Waters MF, et al. Dapagliflozin improves insulin resistance and glucose intolerance in a novel transgenic rat model of chronic glucose overproduction and glucose toxicity. Diabetes Obes Metab. 2017;19(8):1135–1146.
- Yang HJ, Kong B, Shuai W, et al. Shensong Yangxin protects against metabolic syndrome-induced ventricular arrhythmias by inhibiting electrical remodeling. Front Pharmacol. 2020;11:993.
- Shuai W, Kong B, Fu H, et al. The effect of MD1 on potassium and L-type calcium current of cardiomyocytes from high-fat diet mice. Channels (Austin). 2020;14(1):181–189.
- Wang HB, Yang J, Shuai W, et al. Deletion of microfibrillar-associated protein 4 attenuates left ventricular remodeling and dysfunction in heart failure. J Am Heart Assoc. 2020;9(17):e015307.
- Guo Y, Wang L, Ma R, et al. JiangTang XiaoKe granule attenuates cathepsin K expression and improves IGF-1 expression in the bone of high fat diet induced KK-Ay diabetic mice. Life Sci. 2016;148:24–30.
- Lv X, Li J, Hu Y, et al. Overexpression of miR-27b-3p targeting Wnt3a regulates the signaling pathway of Wnt/β-Catenin and attenuates atrial fibrosis in rats with atrial fibrillation. Oxid Med Cell Longev. 2019;2019:5703764.
- Iles L, Pfluger H, Lefkovits L, et al. Myocardial fibrosis predicts appropriate device therapy in patients with implantable cardioverter-defibrillators for primary prevention of sudden cardiac death. J Am Coll Cardiol. 2011;57(7):821–828.
- Kasahara Y, Kiyatake K, Tatsumi K, et al. Bioactivation of monocrotaline by P-450 3A in rat liver. J Cardiovasc Pharmacol. 1997;30(1):124–129.
- Schultze AE, Roth RA. Chronic pulmonary hypertension–the monocrotaline model and involvement of the hemostatic system. J Toxicol Environ Health B Crit Rev. 1998;1(4):271–346.
- Henriques-Coelho T, Roncon-Albuquerque Júnior R, Lourenço AP, et al. Ghrelin reverses molecular, structural and hemodynamic alterations of the right ventricle in pulmonary hypertension. Rev Port Cardiol. 2006;25(1):55–63.
- Henriques-Coelho T, Oliveira SM, Moura RS, et al. Thymulin inhibits monocrotaline-induced pulmonary hypertension modulating interleukin-6 expression and suppressing p38 pathway. Endocrinology. 2008;149(9):4367–4373.
- Correia-Pinto J, Henriques-Coelho T, Roncon-Albuquerque R Jr, et al. Time course and mechanisms of left ventricular systolic and diastolic dysfunction in monocrotaline-induced pulmonary hypertension. Basic Res Cardiol. 2009;104(5):535–545.
- Hardziyenka M, Campian ME, Reesink HJ, et al. Right ventricular failure following chronic pressure overload is associated with reduction in left ventricular mass: evidence for atrophic remodeling. J Am Coll Cardiol. 2011;57(8):921–928.
- Xu Y, Gu Q, Liu N, et al. PPARγ alleviates right ventricular failure secondary to pulmonary arterial hypertension in rats. Int Heart J. 2017;58(6):948–956.
- Umar S, Nadadur RD, Li J, et al. Intralipid prevents and rescues fatal pulmonary arterial hypertension and right ventricular failure in rats. Hypertension. 2011;58(3):512–518.
- Serenelli M, Böhm M, Inzucchi SE, et al. Effect of dapagliflozin according to baseline systolic blood pressure in the Dapagliflozin and prevention of adverse outcomes in heart failure trial (DAPA-HF). Eur Heart J. 2020;41(36):3402–3418.
- Dhillon S. Dapagliflozin: a review in Type 2 diabetes. Drugs. 2019;79(10):1135–1146.
- Li HL, Lip GH, Feng Q, et al. Sodium-glucose cotransporter 2 inhibitors (SGLT2i) and cardiac arrhythmias: a systematic review and meta-analysis. Cardiovasc Diabetol. 2021;20(1):100.
- Lee DM, Battson ML, Jarrell DK, et al. SGLT2 inhibition via dapagliflozin improves generalized vascular dysfunction and alters the gut microbiota in type 2 diabetic mice. Cardiovasc Diabetol. 2018;17(1):62.
- Lin B, Koibuchi N, Hasegawa Y, et al. Glycemic control with empagliflozin, a novel selective SGLT2 inhibitor, ameliorates cardiovascular injury and cognitive dysfunction in obese and type 2 diabetic mice. Cardiovasc Diabetol. 2014;13:148.
- Joubert M, Jagu B, Montaigne D, et al. The sodium-glucose cotransporter 2 inhibitor Dapagliflozin prevents cardiomyopathy in a diabetic lipodystrophic mouse model. Diabetes. 2017;66(4):1030–1040.
- Hammoudi N, Jeong D, Singh R, et al. Empagliflozin improves left ventricular diastolic dysfunction in a genetic model of Type 2 diabetes. Cardiovasc Drugs Ther. 2017;31(3):233–246.
- Spinale FG. Myocardial matrix remodeling and the matrix metalloproteinases: influence on cardiac form and function. Physiol Rev. 2007;87(4):1285–1342.
- Massare J, Berry JM, Luo X, et al. Diminished cardiac fibrosis in heart failure is associated with altered ventricular arrhythmia phenotype. J Cardiovasc Electrophysiol. 2010;21(9):1031–1037.
- Trujillo JM, Nuffer WA. Impact of sodium-glucose Cotransporter 2 inhibitors on nonglycemic outcomes in patients with Type 2 diabetes. Pharmacotherapy. 2017;37(4):481–491.
- Arow M, Waldman M, Yadin D, et al. Sodium-glucose cotransporter 2 inhibitor Dapagliflozin attenuates diabetic cardiomyopathy. Cardiovasc Diabetol. 2020;19(1):7.
- Parizadeh SM, Ghandehari M, Heydari-Majd M, et al. Toll-like receptors signaling pathways as a potential therapeutic target in cardiovascular disease. Curr Pharm Des. 2018;24(17):1887–1898.
- Xu GR, Zhang C, Yang HX, et al. Modified citrus pectin ameliorates myocardial fibrosis and inflammation via suppressing galectin-3 and TLR4/MyD88/NF-κB signaling pathway. Biomed Pharmacother. 2020;126:110071.
- Riad A, Jäger S, Sobirey M, et al. Toll-like receptor-4 modulates survival by induction of left ventricular remodeling after myocardial infarction in mice. J Immunol. 2008;180(10):6954–6961.