ABSTRACT
Animal manure is the main source of bioenergy production by anaerobic digestion (AD). However, the pathogenic bacteria in manure may pose a high risk to human health by contaminating the environment if not effectively inactivated during AD. Worldwide, more than 20,000 biogas plants are running for the treatment of animal manure. AD has been playing the important role in establishing a circular economy in the agricultural sector and may contribute to the United Nations sustainable development goal (UN SDG). Nevertheless, whether AD is a reliable approach for pathogens inactivation has been challenged. A comprehensive understanding of the coping mechanisms of pathogens with adverse conditions and the challenges of establishing the AD process to inactivate effectively pathogens are yet to be analyzed. In this review, the diversity and resistance of pathogens in animal manure are summarized. The efficiencies and the difficulties of their inactivations in AD are also analyzed. In particular, three forms of pathogens i.e. sporing-forming pathogens, viable but non-culturable (VBNC) pathogens, and persistent pathogens are discussed. The factors influencing the pathogens’ inactivation and AD efficiencies are analyzed. The trade-off between energy production and pathogens inactivation in an AD system was consequently pointed out. This review concluded that the development of anaerobic processes should meet the goals of high efficient bioenergy production and deep hygienization.
1. Introduction
The World Health Organization (WHO) ranked infectious diseases caused by pathogens including viruses, bacteria, fungi, and parasites as the second leading cause of death [Citation1]. A major source of these pathogens is animal manure, which harbors pathogens, including disease-causing and non disease-causing pathogens. The use of animal manure as fertilizer to improve soil quality and fertility in agriculture has a long tradition. This causes the pathogen to spread from soil to water bodies, which becomes a potential source of environmental pollution and human infection [Citation2]. It was reported by WHO that there were 600 million illnesses and 420,000 deaths caused by 31 foodborne pathogens globally in 2010 [Citation3]. And during 2019, 25,866 cases of infection, 6164 hospitalizations, and 122 deaths were identified by the Foodborne Diseases Active Surveillance Network [Citation4]. Considering safety and health, hygienization treatment for animal manure should be mandatory. Historically, this is an issue of widespread international concern. The control and destruction of pathogens can be achieved via biological, physical, and chemical methods or a combination of these. Composting and AD are the most common hygienization methods.
AD is a well-established technology for the treatment of various organic wastes and wastewater for bioenergy recovery, organic fertilizer production, and environmental protection. And it is in consistency with UN SDG No. 7 (Affordable and Clean Energy) which advocates for the reduction of waste through recycling and reuse [Citation5]. As a part of bioengineering, AD contributes a bright future for the solution of the problems like resources, environment, and human health facing the world, and further provides sustainable agriculture with economic and social, and environmental benefits [Citation6]. AD has been widely used in the treatment of animal manure. There are about 1347 million tonnes of animal manure produced annually throughout Europe and 1200 million tonnes of animal manure in the EU, and the theoretic biogas potential of manure was estimated at 26 and 23 billion m3 biomethane in Europe and the EU, respectively [Citation7]. In the United States, there were more than 2100 biogas plants in 2017, of which 250 farm-based digestion plants used livestock manure [Citation8]. In Europe, biogas production from agricultural waste, manure, and energy crops accounts for about 74% of the primary biogas energy output [Citation9]. In China, there was 1.8 × 109 t livestock manure in 2017, and the amount of livestock manure used for biogas production is 9.5 × 108 t, and the biogas production potential is approximately 5.7 × 1010 m3 [Citation10]. The substitution of biogas for fossil fuels is driving the sustainable development of many countries.
Meanwhile, the biosafety of the residues generated from the AD plants is the basis for the application of this technology. In some countries, the level of pathogens in anaerobic residues is regulated, and the application of AD effluent on land is permitted upon meeting the environmental quality requirements. It should be stressed that even the level of indicator pathogens is below the detection limit, this cannot be directly translated to the absence of potential pathogenic risk due to the occurrence of other pathogens [Citation11]. It has been reported that most mesophilic biological treatment processes are not likely to reduce pathogen levels by 90%-99% [Citation12]. What’s more, some pathogens can even multiply in the biogas plant environment [Citation13]. Pathogens can be transported from manure-amended soils into water [Citation14], and pathogen infections occurring by the fecal-oral route account for a large proportion in water environments. For a long time, the main focus has been on the efficiency of AD, but less on the controlling of pathogens. The inactivation of pathogens requires a high temperature and high volatile fatty acids (VFAs) environment, but the current anaerobic process is difficult to meet this high-stress environment in bioreactor due to the main goal of methane production in AD process. It is a challenge to achieve simultaneously the efficient methanogenesis and effective sterilization in AD.
Literature reviews about pathogens in animal manure tend to focus on the classification and description of pathogens, the methods involved in the control of pathogens, factors affecting pathogens deactivation in AD, and the mechanisms, kinetic models for pathogen inactivation, and so on. In this review, both disease-causing bacteria and indicative pathogens were discussed. The challenges of pathogen inactivation in animal manure through AD from tolerant pathogens and the limitation of the anaerobic process were discussed emphatically. By combining the inactivation efficiency of pathogens and the energy production of the AD process, this review aims to guide the practical design of AD systems.
2. Existence phenomenon of pathogens in animal manure
2.1. The diversity of pathogens in animal manure
The types of pathogens mainly include bacteria, viruses, parasites, and fungi. Pathogenic bacteria cause diseases by the production of toxins. Bacillus, Clostridium, Escherichia, Staphylococcus, and Vibrio can produce enterotoxins. However, some bacteria are nonpathogenic and therefore harmless. Bacterial pathogens from 31 genera in the virulence factor database (VFDB) (http://www.mgc.ac.cn/VFs/main.htm) are shown in and the specific species that can cause diseases were summarized. This is helpful to strengthen the understanding of the pathogenicity of pathogens. And it will be more targeted to control pathogenic bacteria by distinguishing disease-causing bacteria. Viruses cause diseases by infecting cells and commandeering cell machinery to produce more viruses at a rapid rate. There are two main categories of parasites harmful to humans: pathogens that can cause parasitic diseases and vectors that can transmit diseases. Pathogenic fungi make people and other organisms sick even die.
Table 1. The main disease-causing bacteria
2.2 Resistance of pathogens in animal manure
A large number of pathogenic bacteria exist in animal intestines, being able to adhere to the manure. Pathogenic bacteria species and contents in different kinds of animal wastes may be different. Take Escherichia coli (E. coli) as an example, in the cattle manure, chicken manure, and swine manure, their contents are 107–108 colony-forming unit (CFU)/g, 2.3 × 104–2.3 × 107 CFU/g, and 102–106 CFU/g, respectively [Citation15–17]. Additional examples of pathogenic microorganisms likely to be found in manure are shown in , and the likely diseases or symptoms are also presented. From , there are more types of pathogens in cow manure, which may be related to the animal shape and intestinal characteristics. Among these pathogens, zoonotic pathogens should be paid more attention because zoonotic diseases can spread between animals and people and cause threats to human and animal health.
The physiological structures and characteristics of pathogens determine their resistance to adverse environmental conditions. Gram-negative bacteria are sensitive to high temperature and VFA levels and have low resistance against the stress environment due to their thin (single-layered) cell walls constituting only a lipid-rich outer membrane and a monolayer of peptidoglycan. Gram-positive bacteria have thick (multi-layered) cell walls constituting multi-layered peptidoglycan, which is the structure of the cell membrane and acts as a permeability barrier to prevent the toxic chemicals from penetrating the cell. In addition, spore-forming gram-positive bacteria has high heat resistance. In the normal environment, the spores can survive for many years [Citation13]. Many protozoan parasites can also protect themselves from environmental stressors due to their ability to form cysts and oocysts [Citation18]. Virus ribonucleic acid (RNA) and protein capsid are the main resistance mechanism.
Literature data about the efficiencies of AD process on pathogens inactivation in animal manure were summarized in . For E. coli and coliforms, some studies showed a good inactivation effect, and the content in the discharge was lower than the detection limit. However, some studies showed that pathogens as high as 102–103 most probable number (MPN)/g total solid (TS) or CFU/mL still exist in the discharge. For Enterococcus and Clostridium perfringens, which are difficult to kill, the log10 reduction is still low, mostly <2, even if they are in thermophilic condition.
Table 2. Literature data about the efficiency of AD process on pathogens inactivation in animal manure
3. Challenges of pathogens inactivation in the AD process
3.1. Selecting indicative pathogen
Assessing the biosafety of anaerobic residues is essential for purpose of the application in the fields. However, there are many kinds of pathogens in anaerobic residues, and a number of pathogens are present in low concentrations. Detection of all of the pathogens may be challenging or time-consuming. Therefore, indicator bacteria are used to detect the possible presence of fecal pathogens and to indicate the effect of hygienic treatment of biowaste. The commonly used indicator bacteria are total coliforms, fecal coliforms, E. coli, Enterococci, Salmonella, and others (). Due to their large numbers occurring naturally in human and animal intestinal tracts and the fact that they are easily detectable and countable, they are selected as pathogen indicators. It is important to note that indicator bacteria are not necessarily pathogenic bacteria. Indicator bacteria are the types of bacteria used to detect and estimate the level of fecal contamination of water. Their presence indicates contamination that could be pathogenic to humans thus is used to indicate the presence of a health risk.
Total coliforms, a group of aerobic or facultative anaerobic gram-negative budless bacillus, can ferment lactose at 37°C and produce acid and gas within 24 h. The group members include Escherichia, Klebsiella, Enterobacter, Serratia, Citrobactera, Edwardsiella. Fecal coliforms, also called thermotolerant coliforms, are a sub-group of total coliforms and distinguished from total coliform by the ability to ferment lactose at 44.5°C. E. coli and Klebsiella are the group members. E. coli, a sub-group of fecal coliforms, belongs to gram-negative bacteria. Some specific E. coli serotypes (i.e. O157:H7) are pathogenic to humans and animals and can cause severe diarrhea and septicemia. Salmonella, gram-negative, non-spore forming, aerobic, and facultative anaerobic organisms, belongs to the family of Enterobacteriaceae. Enterococci are suggested as the most suitable indicator bacteria to validate the hygienic treatment of biowaste in biogas plants. The genus Enterococcus is also called fecal streptococci. In addition, Clostridium perfringens, Helminth ova, and Parvovirus are also used to be indicator bacteria. But their standards are less common.
Many countries have set biosafety regulations for pathogenic bacteria in AD [Citation25]. And the standards vary in regulations from country to country. The indicator pathogenic organisms and their biosafety standards are shown in . In the EU, Ireland, and the UK, E. coli is required to be less than 103 CFU/g fresh matter, while in the USA and China, it is that fecal coliform rather than E. coli used as an indicator pathogen, and the requirement is less than 103 and 2 × 106 MPN/g TS in the USA’s Class A and Class B standards, respectively. While in China, fecal coliforms are required to be less than 104 MPN/g fresh matter in ambient and mesophilic AD (MAD) systems and less than 102 MPN/g fresh matter in thermophilic AD (TAD) systems. Salmonella is required to be absent in fresh matter or total solid among the enumerative countries. One peculiarity of the EU standards is that they require log10 reductions rather than specific amounts for Salmonella Senftenberg (≥ 5 log10 reductions), Parvovirus (≥ 3 log10 reductions), and Ascaris eggs (≥ 3 log10 reductions). The difference in national standards makes it difficult to compare horizontally.
Figure 3. Threshold levels of pathogens in anaerobic residues.
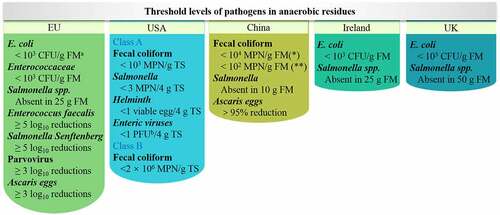
Only when the standard requirements are met can the digestate be allowed for land application. However, a potential problem is that even if the level of indicator pathogens meets the requirements, it cannot be directly interpreted as the absence of potential disease risk, because there may be other non-indicator pathogens. Therefore, it is necessary to strengthen and extend the study of pathogen indicator bacteria and improve the sanitary evaluation method.
3.2. Spore-forming pathogens
Bacterial species have different coping mechanisms with selective harsh environmental conditions. One of the most common coping mechanisms for bacteria is producing resistant forms called ‘spores’ to withstand extreme conditions of starvation, acidity, temperature, and desiccation. And those bacteria are called spore-forming bacteria. Typically, gram-positive bacteria can produce intracellular spores called endospores as a survival mechanism. Endospores are highly retractile and thick-walled structures formed inside the bacterial cells [Citation26], which are the dormant form of vegetative bacteria, carrying all the genetic material as is found in the vegetative form, but no an active metabolism. They are highly resistant to physical and chemical influences and act as a means of survival during hard times. When the environmental conditions turn favorable, endospores germinate back into vegetative cells (an active bacterial cell that undergoes metabolism). Bacteria can survive for many years in this dormant stage in the normal environment because of their spore characteristics.
Bacillu (aerobic) species as well as Clostridium (anaerobic) species are the most common spore-forming bacteria. Bacillus is a common source of contamination in food commodities. The ability of such bacteria to adapt to differences in temperature, pH, and nutrient sources promotes their multiplication in foods and their ability to cause food spoilage [Citation27]. B. cereus is a member of the Bacillus species and it is facultative aerobic bacteria, growing within the temperature range of 10–48°C (optimum temperature is 28–35°C). Clostridium is gram-positive bacteria and only sporulates anaerobically. Clostridium botulinum, Clostridium perfringens, and Clostridium difficile are species of widespread concern due to their pathogenic nature. Clostridium botulinum produces botulinum toxin and causes botulism. Infections with Clostridium perfringens can cause necrosis and gangrene. And Clostridium difficile can cause a series of diseases such as clinical manifestations with acquired diarrhea and pseudomembranous enteritis by producing multiple toxins [Citation28]. summarizes the characteristics of the three Clostridium species.
Table 3. The characteristics of Clostridium botulinum, Clostridium perfringens, and Clostridium difficile.
The cells of Bacillus and Clostridium are sensitive to thermophilic temperature but the spores are highly heat-resistant. It was reported that pathogens such as Salmonella and Mycobacteria paratuberculosis were inactivated within 24 h in TAD while weeks and even months were needed in MAD [Citation29]. However, spores of Clostridium perfringens type C and Bacillus cereus in cattle and pig slurry were not inactivated in MAD (35°C) or TAD (53°C) [Citation30]. Pasteurization heating is insufficient to destroy the Clostridium perfringens spores in cow manure. In the batch AD experiment of cow manure, Clostridium perfringens was reduced by 1.35 log10 reductions at 35°C after 15 days, and <1 log10 reduction was observed at 55°C (2 days) and 70°C (1 day) [Citation31]. It was reported that the decimal reduction time (DRT) of vegetative cells was 33.2 min at 50°C and 1 min at 60°C for Bacillus cereus,16.3 min at 55°C and 0.9 min at 65°C for Clostridium perfringens, respectively; while the DRT of Bacillus cereus spores was 32.1 min at 85°C and 2.0 min at 95°C, and the DRT of Clostridium perfringens spores was 34.2 min at 90°C and 2.2 min at 100°C [Citation32]. In most studies about AD, 0–1.8 log10 reductions were observed at the temperature of 24–55°C and HRT of 7–56 days [Citation25]. Sometimes the concentrations of Clostridium perfringens in the anaerobic residues were even higher than those in the substrates, with 0.12–1.3 log10 increases at the HRT of 15–90 days [Citation33–36]. The final concentrations in all of them were above 3 × 103 spores/g dry matter (DM).
3.2. Overlook of VBNC pathogens
VBNC state is a dormant-like state of bacteria, and many species of bacteria can enter this state when suffering stress conditions. It was considered a survival strategy for bacteria to avoid adverse conditions. This phenomenon was discovered by Xu et al. when they studied E. coli and Vibrio cholera in 1982 [Citation37]. Many factors including low or high temperature, extreme pH, oligotrophy, disinfectant, and high-energy rays can induce bacteria into VBNC state [Citation38,Citation39]. Compared with the normal growing cells, the metabolic activity of the VBNC bacteria was greatly reduced, the ability to divide and prolifize was decreased, and the ability to form colonies on the medium was lost. Most of the bacteria such as gram-negative bacteria which do not produce spores can enter the VBNC state to resist the external environmental pressure, to achieve the purpose of continued survival. Up to now, more than 80 species of bacteria have been reported to be able to enter the VBNC state under adverse environmental conditions. Pathogens in animal manure, such as E. coli, Salmonella, Shigella, Enterococcus, Listeria, are potential VBNC bacterial during AD. VBNC pathogens usually do not cause diseases, but they still keep virulence. They can resuscitate to culturable state and recover their pathogenicity when conditions become favorable. It was reported that E. coli entered the VBNC state in the MAD and TAD of sludge. During the subsequent centrifugation dehydration, culturable E. coli were found to revive and multiply [Citation40]. The relationship between normal pathogens and VBNC pathogens is shown in .
The count of E. coli in cow dung was measured by MPN and quantitative polymerase chain reaction (qPCR), and the result of the latter was 100 times higher than that of the former, indicating that most E. coli was nonculturable [Citation15]. There are more reports about the VBNC of pathogenic bacteria in sludge AD. In the TAD of sludge, the count result of E. coli obtained by the traditional culture method was 20 CFU/g dry solids (DS), while that obtained by competitive PCR (cPCR) was 7 × 105 CFU/g DS. The difference showed the existence of the VBNC [Citation41]. It was found that the total viable count of E. coli in sludge effluent was similar to the feed concentration of 9–10 log10 MPN/g DS through the MAD (35°C), TAD (55°C), and temperature-phased anaerobic digestion (TPAD) (55°C + 35°C). Further study found that the culturable E. coli increased by 2, 2, and 4 orders of magnitude immediately after dewatering for the MAD, TAD, and TPAD processes, respectively. The results of molecular quantifying methods indicated that the sudden increase of culturable pathogen content after dewatering is actually the reactivation of E. coli from the VBNC state to the culturable state [Citation40]. Due to the existence of pathogen VBNC state, AD thus mainly alters the culturable state of pathogens rather than killing them, which greatly limits the efficiency of AD in inactivating pathogens. The current evaluation of the inactivation effect of pathogens by AD is based on cultivable pathogens. However, VBNC pathogens cannot form colonies on the culture medium, resulting in false-negative test results and ultimately overestimating the inactivation performance. The true inactivation rate of viable pathogens in AD should be considered.
3.3. Persistent pathogens phenomenon
Persister cells are often a component of a stringent response of the microorganism to unfavorable conditions, especially antibiotics, which are among the most commonly used drugs worldwide due to their capacity of killing or inhibiting the growth of bacteria to fight bacterial infections [Citation42]. They are dormant variants of regular cells that form stochastically in microbial populations and are highly tolerant to antibiotics. Persister cells gain their resistance property protecting them from toxic compounds acting by entering a state of dormancy. In this state, they are metabolically less active cells. The remaining metabolic activities are mostly focused on energy production [Citation43]. However, they do not grow or die in the presence of antibiotics. In the absence of antibiotics stress, persister cells can return to sensitive and active growth phenotypes. Persister cells may be associated with chronic or recurrent infections [Citation44]. Bacteria with persistence have been reported, including Mycobacterium tuberculosis, Salmonella typhi, Chlamydia, Brucella, E. coli, and streptococcus [Citation45,Citation46].
In AD, the prevalence of antimicrobial resistance among bacteria isolated from influents and effluents has been reported. Antibiotic-resistant pathogens may proliferate through the environment and allow the spread of resistance genes through bacterial genetic recombination, thus influencing human and animal antimicrobial chemotherapy [Citation47]. A persistence of drug-resistant bacteria in thermophilic co-digestion of dairy manure and waste milk at 55°C until the end of the process was observed by Beneragama [Citation48].
4. Challenges in establishing strong inactivation AD process
The inactivation efficiency of pathogens is affected by many factors, including pathogen type, intermediate products, and operating conditions, especially temperature [Citation25]. The combined effects of these factors eventually led to the inactivation of pathogens. However, the resistance of pathogens causes obstacles to the effective inactivation of pathogens. In addition, it is reported that it’s more difficult to reduce pathogens for treatment on larger scales (e.g., full scale biogas plants) than that on laboratory scales [Citation49]. Therefore, how to strengthen the inactivation of pathogens in practical engineering should be further discussed.
4.1. Mesophilic vs thermophilic process
AD generally can be divided into TAD (50–55°C) and MAD (30–42°C). Most pathogens survive longer at mesophilic temperatures than at thermophilic temperatures. As the temperature increases (from 37 to 70°C), the fluidity and permeability of the cell membrane increase, which allows toxic chemicals to diffuse more rapidly into the cytoplasm and inhibits cell growth [Citation50]. In a mesophilic (35°C) small-scale AD, average T90 values, which is defined as the time for bacterial concentration to decrease by 90%, were 1.8 days for E. coli, 2.0 days for Salmonella dublin, and 0.9 days for Staphylococcus aureu. In a thermophilic (53°C) small-scale AD, T90 values of 0.4 h were determined for E. coli, 0.6 h for Salmonella dublin, and 0.5 h for Staphylococcus aureus [Citation30]. TAD has advantages over MAD in inactivating pathogens, but considering energy consumption, process stability, and diversity of anaerobic microorganisms, MAD is more widely applied to practice [Citation51]. Given this, the AD process should have greater innovation in inactivating pathogens.
4.2. Is multi-stage worthy for application?
Worldwide, single AD is more widely used than multi-stage process despite the latter having many advantages. Due to the characteristics of the substrate, pretreatment is needed to improve biogas production in single AD, and temperature is the parameter that had the greatest effect during AD [Citation52]. Pasteurization as a typical heat-treatment method, can be regarded as a stage in a multi-stage AD. In Swedish biogas plants, the undigested substrate is heated to 70°C in 60 min in a separate batch-wise step before AD. Germany and Austria also recommend that animal and poultry manure should be sterilized at 70°C for 20 or 30 min before entering AD tanks, to ensure the safety of biogas engineering [Citation53]. In Denmark, pasteurization (70°C in 60 min) may be replaced with a ‘similar method’ (a longer time at a lower temperature). This is combined with thermophilic or mesophilic digestion with regulated temperature and time [Citation13], called TPAD. However, pasteurization is always different from the first stage of multi-stage AD, and it is still not commonly used in underdeveloped areas or countries.
In the first stage of multi-stage AD, a high temperature or/and high VFAs concentration in the environment, which are considered as the main factors of pathogens inactivation, can be created by adjusting process parameters. VFAs are essential intermediates produced in the acidogenesis and acetogenesis step when organic materials are degraded during the AD process [Citation54], and free VFAs and ionized VFAs are the two forms of VFAs. Free VFAs are considered more toxic to pathogens than ionized VFAs, as they are lipophilic and can freely permeate the cell membrane [Citation55]. The toxicity of VFAs is dependent on the pH, which affects the distributions of free and ionized VFAs, thus affecting pathogen inactivation indirectly. Under the condition of low pH, VFAs mainly exist in a molecular state, which indicates that the high VFAs concentration and low pH are more conducive to inactivating pathogens. In an anaerobic digester for cattle dung with VFA levels of 5000 mg/L and pH 6.0, Salmonella typhi was completely eliminated within 12 d, whereas 26 d were required in the digester with VFA of 100 mg/L and pH 6.8. And T90 values for the two digesters were 2.44 d and 4.80 d, respectively [Citation56]. VFAs mainly include acetic, propionic, isobutyric, butyric, isovaleric, valeric, and caproic acid [Citation57]. Generally, the inhibitory effect of VFAs on pathogens increases with the increase of the organic acid chain length. A greater inactivation effect of n-caproic acid against Ascaris eggs compared with n-butyric acid was reported [Citation58]. Jiang et al. reported the inhibitory effect of VFAs against Salmonella was in the order of valeric acid > isovaleric acid ≥ propionic acid > butyric acid ≥ isobutyric acid > acetic acid [Citation59]. The first stage of multi-stage AD can effectively inactivate pathogens due to high temperature or VFA, and the second stage can realize the stable production of methane. Therefore, multi-stage AD should be considered to be popularized.
4.3. Tradeoff between energy production and pathogens inactivation
Bacterial inactivation due to temperature is related to time. In general, the longer the hydraulic retention time (HRT), the better the inactivation effect of pathogens. At the same temperature (35°C), a biogas plant with a longer HRT of 40 d was detected a significantly higher reduction of Campylobacter (87.4%) than 74.3% that in a biogas plant with HRT of 30 d [Citation60]. However, the extension of HRT reduces the volumetric gas production rate and energy production efficiency, which becomes contradictory with the sterilization effect. In addition, some studies have also pointed out that prolonged retention time has no significant effect on some pathogens. When the HRT was increased from 21 d to 41 d at MAD (39°C) of pig manure, the inactivation amount of E. coli increased slightly but not significantly, and the concentration was between 2.0 and 2.5 log CFU/g [Citation61]. When the HRT was increased from 7.4 d to 46.9 d, the reduction of E. coli in AD is in the range of 0.19 log10 to 2.99 log10, which is no correlation with HRT [Citation62]. Therefore, retention time is not a direct factor affecting the inactivation effect of pathogens and it works alongside other factors to inactivate pathogens. The specific inactivation mechanism needs to be further studied.
4.4. Double-edged sword of ammonia for fermentation and hygienization
Ammonia is the intermediate product of AD, which is released due to the degradation of organic molecules containing N such as proteins. Literature has reported the positive effect of ammonia on pathogen reduction in the mesophilic process at a total ammonia (TAN) concentration of 6–9 g/L [Citation63]. In addition, free ammonia (FA, NH3) is considered more toxic to pathogens than ammonium ion (NH4+) due to its lipophilic property, which can pass the cell membrane freely. Temperature, pH, and TAN determine the presence of FA, substantially [Citation64].
In a mesophilic anaerobic digester for pig manure, pathogens (Fecal coliform, E. coli, fecal streptococci, and Enterobacteriaceae) content and toxic-NH3 content were significant negative correlations [Citation33]. The NH3 concentration of 644 mg N/L (46 mM) caused 5 log10 reductions on Salmonella Typhimurium and Enterococcus faecalis after 1.6 and 4.2 days, respectively [Citation63]. There was a complete Salmonella reduction when the toxic-NH3 content was more than 560 mg NH3–N/L (40 mM) after 6 h, but 2520 (180 mM) mg NH3–N/L was needed to obtain >5 log10 reductions for E. coli O157:H7 after 6 h [Citation65]. It indicated that the higher concentration of ammonia nitrogen, the better the inactivation effect of pathogens.
However, the high concentration of ammonia nitrogen in an AD system will inhibit methanogenesis to some extent [Citation66]. It is generally believed that the lower limit concentration of ammonia nitrogen inhibiting methane fermentation is 3.0–4.0 g/L. But a successful biogas plant running under a much higher ammonia nitrogen level was reported [Citation67]. FA is freely membrane-permeable, which has been suggested to be the main reason for the inhibition [Citation68]. It was reported that FA concentrations of 150–400 mg/L are a cause of inhibition in TAD during the appraisal from a previous study [Citation69]. A 50% inhibition of methanogenesis was observed during TAD of pig manure at a FA concentration of 1450 mg/L [Citation70]. Therefore, in the anaerobic treatment of animal manure with high nitrogen content (such as chicken manure), high ammonia nitrogen has a positive effect on the inactivation of pathogens, but the possible adverse effects of high ammonia nitrogen on methane fermentation should also be considered. When reasonable organic load rate (OLR) and HRT were set, the AD methanogenesis system can also operate normally under a high ammonia nitrogen concentration of 6000 mg/L [Citation71].
5. Conclusions and prospects
AD is an effective way to realize the reduction treatment and resource recovery and utilization of animal manure, but it cannot completely inactive pathogenic bacteria. Pathogens have coping mechanisms with adverse conditions to survive to the highest extent, providing spore-forming pathogens and VBNC pathogens as the main forms. Conventional AD processes are thus difficult to effectively inactivate those patognes. High temperature, high VFAs, long retention time, and high ammonia concentrations have positive effects on inactivating pathogens. Those conditions can be achieved in an AD process and may be used to deeply inactivate pathogens. Multi-stage AD was consequently proposed as a potential approach to meet the objectives of high efficient methanogenesis and hygienization in AD.
Acknowledgements
This work was partially supported by the Key Laboratory of Environmental Biotechnology, CAS (kf2020012), and the State Key Joint Laboratory of Environment Simulation and Pollution Control (20K03ESPCT).
The authors declare that they have no known competing financial interests or personal relationships that could have appeared to influence the work reported in this paper.
Disclosure statement
No potential conflict of interest was reported by the author(s).
Additional information
Funding
References
- Thakur A, Mikkelsen H, Jungersen G. Intracellular pathogens: host immunity and microbial persistence strategies. J Immunol Res. 2019;2019:1356540.
- Manyi-Loh CE, Mamphweli SN, Meyer EL, et al. An overview of the control of bacterial pathogens in cattle manure. Int J Environ Res Public Health. 2016;13(9):843.
- WHO. WHO estimates of the global burden of foodborne diseases: foodborne disease burden epidemiology reference group 2007-2015. World Health Org. 2015.
- Marder EP, Griffin PM, Cieslak PR, et al. Preliminary incidence and trends of infections with pathogens transmitted commonly through food - foodborne diseases active surveillance network, 10 US sites, 2006-2017. Mmwr-Morbidity Mortality Weekly Rep. 2018;67(11):324–328.
- Akor CI, Osman AI, Farrell C, et al. Thermokinetic study of residual solid digestate from anaerobic digestion. Chem Eng J. 2021;406:127039.
- Yu X, Liu Y, Wang Y, et al. Role of bioengineering and laborers in integration of farmland resources toward to improve dimension of sustainable agriculture in China. Bioengineered. 2020;11(1):559–571.
- Scarlat N, Fahl F, Dallemand J-F, et al. A spatial analysis of biogas potential from manure in Europe. Renew Sust Energ Rev. 2018;94:915–930.
- USEPA. United States environmental protection agency.AgStar program biogas recovery in the agriculture sector. 2017.
- Eurostat. European Statistics. 2017.
- Lu J, Gao X. Biogas: potential, challenges, and perspectives in a changing China. Biomass Bioenergy. 2021;150:106127.
- Zhao Q, Liu Y. Is anaerobic digestion a reliable barrier for deactivation of pathogens in biosludge? Sci Total Environ. 2019;668:893–902.
- Manyi-Loh CE, Mamphweli SN, Meyer EL, et al. Inactivation of selected bacterial pathogens in dairy cattle manure by mesophilic anaerobic digestion (Balloon type digester). Int J Environ Res Public Health. 2014;11(7):7184–7194.
- Sahlström L. A review of survival of pathogenic bacteria in organic waste used in biogas plants. Bioresour Technol. 2003;87(2):161–166.
- Alegbeleye OO, Sant’Ana AS. Manure-borne pathogens as an important source of water contamination: an update on the dynamics of pathogen survival/transport as well as practical risk mitigation strategies. Int J Hyg Environ Health. 2020;227:113524.
- Klein M, Brown L, Tucker RW, et al. Diversity and abundance of zoonotic pathogens and indicators in manures of feedlot cattle in Australia. Appl Environ Microbiol. 2010;76(20):6947–6950.
- Thomas C, Idler C, Ammon C, et al. Inactivation of ESBL-/AmpC-producing Escherichia coli during mesophilic and thermophilic anaerobic digestion of chicken manure. Waste Manag. 2019;84:74–82.
- Massé D, Gilbert Y, Topp E. Pathogen removal in farm-scale psychrophilic anaerobic digesters processing swine manure. Bioresour Technol. 2011;102(2):641–646.
- Rousseau A, La Carbona S, Dumètre A, et al. Assessing viability and infectivity of foodborne and waterborne stages (cysts/oocysts) of Giardia duodenalis, Cryptosporidium spp., and Toxoplasma gondii: a review of methods. Parasite. 2018;25:14.
- Pandey PK, Soupir ML. Escherichia coli inactivation kinetics in anaerobic digestion of dairy manure under moderate, mesophilic and thermophilic temperatures. Amb Express. 2011;1(1):18.
- Smith SR, Lang NL, Cheung KHM, et al. Factors controlling pathogen destruction during anaerobic digestion of biowastes. Waste Manag. 2005;25(4):417–425.
- Popova TP, Zaharinov B, Kaleva MD, et al. Reduction of microorganisms in thermophilic process of anaerobic digestion of cattle manure. Int J Curr Microbiol Appl Sci. 2013;2:653–660.
- Varel VH, Wells JE, Shelver WL, et al. Effect of anaerobic digestion temperature on odour, coliforms and chlortetracycline in swine manure or monensin in cattle manure*. J Appl Microbiol. 2012;112(4):705–715.
- Fongaro G, Kunz A, Magri ME, et al. Evaluation of the effective inactivation of enteric bacteria and viruses from swine effluent and sludge at tropical temperatures. Water Air Soil Pollut. 2018;229(7). DOI:10.1007/s11270-018-3878-y.
- McCarthy G, Lawlor PG, Gutierrez M, et al. Assessing the biosafety risks of pig manure for use as a feedstock for composting. Sci Total Environ. 2013;463:712–719.
- Jiang Y, Xie SH, Dennehy C, et al. Inactivation of pathogens in anaerobic digestion systems for converting biowastes to bioenergy: a review. Renew Sust Energ Rev. 2020;120:109654.
- Basta M, Annamaraju P. Bacterial spores. StatPearls. 2021. Treasure Island (FL): StatPearls Publishing. https://www.ncbi.nlm.nih.gov/books/NBK556071/.
- Carlin F, Nguyen C. Pathogen update: bacillus species. In: Sofos J, editor. Part 4, Pathogen update: Bacillus species, advances in microbial food safety. England: Woodhead Publishing; 2013. p. 70–96.
- Wang J, Yang C, Zhang C, et al. Complete genome sequence of the Clostridium difficile LCL126. Bioengineered. 2021;12(1):745–754.
- Plymforshell L. Survival of salmonellas and ascaris suum eggs in a thermophilic biogas plant. Acta Vet Scand. 1995;36(1):79–85.
- Olsen JE, Larsen HE. Bacterial decimation times in anaerobic digestions of animal slurries. Biological Wastes. 1987;21(3):153–168.
- Watcharasukarn M, Kaparaju P, Steyer JP, et al. Screening Escherichia coli, enterococcus faecalis, and Clostridium perfringens as indicator organisms in evaluating pathogen-reducing capacity in biogas plants. Microb Ecol. 2009;58(2):221–230.
- Byrne B, Dunne G, Bolton DJ. Thermal inactivation of Bacillus cereus and Clostridium perfringens vegetative cells and spores in pork luncheon roll. Food Microbiol. 2006;23(8):803–808.
- Orzi V, Scaglia B, Lonati S, et al. The role of biological processes in reducing both odor impact and pathogen content during mesophilic anaerobic digestion. Sci Total Environ. 2015;526:116–126.
- Costa A, Gusmara C, Gardoni D, et al. The effect of anaerobic digestion and storage on indicator microorganisms in swine and dairy manure. Environ Sci Pollut Res. 2017;24(31):24135–24146.
- Lloret E, Pastor L, Pradas P, et al. Semi full-scale thermophilic anaerobic digestion (TAnD) for advanced treatment of sewage sludge: stabilization process and pathogen reduction. Chem Eng J. 2013;232:42–50.
- Salsali H, Parker WJ, Sattar SA. The effect of volatile fatty acids on the inactivation of Clostridium perfringens in anaerobic digestion. World J Microbiol Biotechnol. 2008;24(5):659–665.
- Xu HS, Roberts N, Singleton FL, et al. Survival and viability of nonculturable escherichia-coli and vibrio-cholerae in the estuarine and marine-environment. Microb Ecol. 1982;8(4):313–323.
- Colwell RR, Grimes DJ. Nonculturable microorganisms in the environment. Part I, Semantics and strategies. 2000:1–6.
- Pinto D, Santos MA, Chambel L. Thirty years of viable but nonculturable state research: unsolved molecular mechanisms. Crit Rev Microbiol. 2015;41(1):61–76.
- Fu B, Jiang Q, Liu HB, et al. Occurrence and reactivation of viable but non-culturable E-coli in sewage sludge after mesophilic and thermophilic anaerobic digestion. Biotechnol Lett. 2014;36(2):273–279.
- Higgins MJ, Chen YC, Murthy SN, et al. Reactivation and growth of non-culturable indicator bacteria in anaerobically digested biosolids after centrifuge dewatering. Water Res. 2007;41(3):665–673.
- Yang Q, Gao Y, Ke J, et al. Antibiotics: an overview on the environmental occurrence, toxicity, degradation, and removal methods. Bioengineered. 2021;12(1):7376–7416.
- Radzikowski JL, Vedelaar S, Siegel D, et al. Bacterial persistence is an active σS stress response to metabolic flux limitation. Mol Syst Biol. 2016;12(9):882.
- Humphreys GJ, Mcbain AJ. An introduction to the biology of biofilm recalcitrance, Chapter 14. Biofilms in Infection Prevention Control. 2014: 245–256. ISBN: 9780123977519.
- Monack DM, Mueller A, Falkow S. Persistent bacterial infections: the interface of the pathogen and the host immune system. Nat Rev Microbiol. 2004;2(9):747–765.
- Helaine S, Kugelberg E. Bacterial persisters: formation, eradication, and experimental systems. Trends Microbiol. 2014;22(7):417–424.
- Walsh JJ, Rousk J, Edwards-Jones G, et al. Fungal and bacterial growth following the application of slurry and anaerobic digestate of livestock manure to temperate pasture soils. Biol Fertil Soils. 2012;48(8):889–897.
- Beneragama N, Iwasaki M, Lateef SA, et al. The survival of multidrug-resistant bacteria in thermophilic and mesophilic anaerobic co-digestion of dairy manure and waste milk. Anim Sci J. 2013;84(5):426–433.
- Liu X, Lendormi T, Lanoisellé JL. Conventional and innovative hygienization of feedstock for biogas production: resistance of indicator bacteria to thermal pasteurization, pulsed electric field treatment, and anaerobic digestion. Energies. 2021;14(7):1938.
- Salsali HR, Parker WJ, Sattar SA. Impact of concentration, temperature, and pH on inactivation of Salmonella spp. by volatile fatty acids in anaerobic digestion. Can J Microbiol. 2006;52(4):279–286.
- Gavala HN, Yenal U, Skiadas IV, et al. Mesophilic and thermophilic anaerobic digestion of primary and secondary sludge. Effect of pre-treatment at elevated temperature. Water Res. 2003;37(19):4561–4572.
- Zhang S, Wang Y, Liu S. Process optimization for the anaerobic digestion of poplar (Populus L.) leaves. Bioengineered. 2020;11(1):439–448.
- Marañón E, Castrillón L, Fernández JJ, et al. Anaerobic mesophilic treatment of cattle manure in an upflow anaerobic sludge blanket reactor with prior pasteurization. J Air Waste Manage Assoc. 2006;56(2):137–143.
- Patinvoh RJ, Millati R, Sárvári-Horváth I, et al., Lukitawesa. Factors influencing volatile fatty acids production from food wastes via anaerobic digestion. Bioengineered. 2020;11(1):39–52.
- Puchajda B, Oleszkiewicz J, Sparling R, et al. Low-temperature inactivation of fecal coliforms in sludge digestion. Water Environ Res. 2006;78(7):680–685.
- Kunte DP, Yeole TY, Chiplonkar SA, et al. Inactivation of Salmonella typhi by high levels of volatile fatty acids during anaerobic digestion. J Appl Microbiol. 1998;84(1):138–142.
- Wainaina S, Kumar Awasthi M, Taherzadeh MJ. Bioengineering of anaerobic digestion for volatile fatty acids, hydrogen or methane production: a critical review. Bioengineered. 2019;10(1):437–458.
- Harroff LA, Liotta JL, Bowman DD, et al. Inactivation of Ascaris eggs in human fecal material through in situ production of carboxylic acids. Environ Sci Technol. 2017;51(17):9729–9738.
- Jiang Y, Dennehy C, Lawlor PG, et al. Inactivation of Salmonella during dry co-digestion of food waste and pig for manure. Waste Manag. 2018;82:231–240.
- Qi G, Pan Z, Yamamoto Y, et al. The survival of pathogenic bacteria and plant growth promoting bacteria during mesophilic anaerobic digestion in full-scale biogas plants. Anim Sci J. 2019;90(2):297–303.
- Dennehy C, Lawlor PG, McCabe MS, et al. Anaerobic co-digestion of pig manure and food waste; effects on digestate biosafety, dewaterability, and microbial community dynamics. Waste Manag. 2018;71:532–541.
- Huong LQ, Madsen H, Anh LX, et al. Hygienic aspects of livestock manure management and biogas systems operated by small-scale pig farmers in Vietnam. Sci Total Environ. 2014;470:53–57.
- Ottoson JR, Schnurer A, Vinneras B. In situ ammonia production as a sanitation agent during anaerobic digestion at mesophilic temperature. Lett Appl Microbiol. 2008;46(3):325–330.
- Scaglia B, D’Imporzano G, Garuti G, et al. Sanitation ability of anaerobic digestion performed at different temperature on sewage sludge. Sci Total Environ. 2014;466:888–897.
- Park GW, Diez-Gonzalez F. Utilization of carbonate and ammonia-based treatments to eliminate Escherichia coli O157: H7 and Salmonella Typhimurium DT104 from cattle manure. J Appl Microbiol. 2003;94(4):675–685.
- Bi S, Westerholm M, Qiao W, et al. Metabolic performance of anaerobic digestion of chicken manure under wet, high solid, and dry conditions. Bioresour Technol. 2020;296:122342.
- Bi S, Westerholm M, Hu W, et al. The metabolic performance and microbial communities of anaerobic digestion of chicken manure under stressed ammonia condition: a case study of a 10-year successful biogas plant. Renewable Energy. 2021;167:644–651.
- Chen Y, Cheng JJ, Creamer KS. Inhibition of anaerobic digestion process: a review. Bioresour Technol. 2008;99(10):4044–4064.
- Duan NN, Dong B, Wu B, et al. High-solid anaerobic digestion of sewage sludge under mesophilic conditions: feasibility study. Bioresour Technol. 2012;104:150–156.
- Sung S, Liu T. Ammonia inhibition on thermophilic anaerobic digestion. Chemosphere. 2003;53(1):43–52.
- Mahdy A, Bi S, Song Y, et al. Overcome inhibition of anaerobic digestion of chicken manure under ammonia-stressed condition by lowering the organic loading rate. Bioresour Technol Rep. 2020;9:100359.