ABSTRACT
Ischemic stroke is one of the leading causes of morbidity and mortality in humans. Cerebral ischemia-reperfusion (CIR) injury serves as a leading cause of stroke. Schisandra chinensis is a well-known Chinese traditional medicine. In this study, we explored the role of Gomisin J (GJ), a compound of S. chinensis, in CIR using a middle cerebral artery occlusion/reperfusion rat model and the possible mechanisms. We identified that GJ reduced neurological scores, cerebral infarction, and water content in the I/R rat brain. Importantly, GJ rescued I/R treatment-reduced neuron survival in the hippocampus, inhibited apoptosis of ischemic tissues in I/R rats, increased B-cell lymphoma-extra-large (Bcl-XL) expression, and reduced the levels of cleaved caspase-3, Bax, cyclooxygenase-2, nuclear factor kappa-B, and nitric oxide in I/R rat brain tissues. Furthermore, GJ treatment enhanced nuclear factor E2 related factor 2 (Nrf2) translocation, heme oxygenase-1 (HO-1) expression, superoxide dismutase and glutathione peroxidase activities, and glutathione level. Overall, GJ treatment GJ attenuates CIR injury by inducing anti-apoptotic, antioxidant, and anti-inflammatory effects in vivo.
1. Introduction
Stroke, a general cause of long-course of serious disorders and mortality globally [Citation1,Citation2], is usually provoked by cerebral ischemia due to embolism occlusion of prominent brain aorta or thromboembolism [Citation3]. To intervene stroke, reperfusion is often performed for blood restoration. However, the procedure easily leads to cerebral ischemia/reperfusion (CIR) injury [Citation4] due to hypoxia following the short-course restoration of blood reperfusion [Citation5]. Increasing studies have indicated that CIR injury usually comprises neural phenomena, such as inflammatory response, oxidative stress, and hypoxia [Citation6], which eventually results in necrosis, apoptosis, and acute autophagy in the ischemic cerebrum [Citation7]. Currently, tissue plasminogen activator (tPA) is the only practical approach to manage CIR injury [Citation8]. Therefore, it is urgent and necessary to recognize novel and useful therapeutic targets and develop effective agents for CIR injury treatment for patients with stroke.
In recent years, various novel traditional Chinese medicines have been formulated to treat cerebrovascular disorders [Citation9–11]. For example, Radix Scrophulariae has been identified to attenuate CIR injury by modulating MAPK signaling [Citation12]. Schisandra Chinensis is a wood herb native to China, Japan, and Korea [Citation13]. S. Chinensis has been recognized as a common herb medicine to treat and alleviate many disorders [Citation14,Citation15]. It comprises various active chemical components, including gomisins, pregomisin, deoxyschisandrin, schisandrin, and lignans and is involved in many signaling pathways to modulate various biological processes and exert various biological roles [Citation16–18], such as anti-cancer, obesity, adipogenesis, oxidative stress, inflammation, fibrosis, and vascular contractility. Gomisin J (GJ) is a major component of S. Chinensis and has been identified to present numerous biological activities, including antiretroviral activity, anti-inflammation, defensive impact on angiotensin II-related hypertension, anti-hepatic effects on cardiovascular symptoms, and vascular relaxation [Citation19–21]. All these imply that GJ may possess potential functions in modulating cerebrovascular disorders. However, the effect of GJ on CIR injury is still elusive. Therefore, we constructed a middle cerebral artery occlusion/reperfusion (MCAO/R) rat model and explored the role of GJ in CIR injury. Our data showed that GJ relieved neuronal injury, attenuated neurological loss of the hippocampus, repressed apoptosis of ischemic tissues, induced anti-inflammatory and antioxidant effects, and decreased lipid peroxidation. Our results indicated that GJ might perform a novel function in inhibiting CIR injury.
2. Materials and methods
2.1. Experimental animals and grouping
Male Wistar rats (n = 180, body weight 250–300 g) from Shandong Pengyue Experimental Animal Company were used in the study. They were kept under the restriction of a controlled temperature (25 ±1°C) and light cycles (12 h light/12 h dark) and allowed free access to water and food. Rats were randomly divided into sham (n = 18), I/R(n = 30), and I/R plus GJ groups. Rats in the I/R plus GJ group were further divided into 5 mg/kg GJ (n = 18), 10 mg/kg GJ group (n = 18), 20 mg/kg GJ group (n = 18), 40 mg/kg GJ group (n = 18), and 80 mg/kg GJ group (n = 30).
2.2. MCAO/R rat model
To analyze CIR injury, the MCAO/R model was constructed as previously reported [Citation22]. Briefly, rats were placed in a fixed frame and quickly anesthetized using sodium pentobarbital (30 mg/kg). Then, the blood was occluded from the common carotid artery through external carotid artery by inserting a 4–0 nylon filament into the internal carotid artery for 2 h. After that, the blood flow was restored for 3, 6, 12, 24, or 48 h. Rats in the sham group received the same operation without MCAO/R occlusion. Neurological function score was recorded after reperfusion, and brain tissues were obtained for subsequent analysis. All procedures and animal care were authorized by the Animal Ethics Committee of The Second Affiliated Hospital of Kunming Medical University.
2.3. Drug administration
GJ (purity ≥ 99%, MCE, USA) was dissolved in PBS with 0.1% dimethyl sulfoxide (DMSO) and 1% hydroxyethyl cellulose. GJ was intraperitoneally injected into rats at 5, 10, 20, 40, and 80 mg/kg body weights [Citation23,Citation24] in the I/R plus GJ group before cerebral reperfusion. Rats in the I/R and sham groups were intraperitoneally injected with PBS with 0.1% dimethyl sulfoxide (DMSO) and 1% hydroxyethyl cellulose.
2.4. Brain damage analysis
The neurological deficits of rats after MCAO/R operation were evaluated by behavioral inspection and scored from 0 to 5 points by standard criteria [Citation25]. Rats without neurologic deficit were scored 0 point; Rats with mild focal neurologic deficit and failure to extend left forepaw fully were scored 1 point; Rats with moderate focal neurologic deficit and circling to the left were scored 2 points; Rats with severe focal deficit and falling to the left were scored 3 points; Rats with depressed consciousness and unable to walk spontaneously were scored 4 points; and rats that died during experiments were scored 5 points. 2,3,5-Triphenyltetrazolium chloride (TTC, Sigma, USA) was used to evaluate cerebral infarction. At 24 h after operation, 6 rats from each group were sacrificed, and the whole brains were rapidly removed. In addition, the infarct volumes of rats in the 80 mg/kg GJ and the I/R groups were estimated at 3, 6, 12, and 48 h after reperfusion. The brain samples were coronally sectioned into 2 μm slices, incubated with 2% TCC solution for 20 min, fixed using 4% paraformaldehyde, and observed under a microscope. The normal tissues were stained red with TCC, while the infarcted sections appeared white. The infarcted volume was calculated [Citation26] as previously described. The brain water content (BWC) was determined by the difference in infarct hemisphere weight before and after being dried [Citation12]. Hematoxylin and eosin staining was used to analyze the numbers of surviving neurons of the hippocampus in the rats.
2.5. TUNEL assay
Samples were fixed in 10% formalin for 24 h, dehydrated in gradient ethanol solutions, and prepared as 5 μm paraffin sections using a microtome. The sections were dewaxed, incubated with 0.1 M PBS containing 0.3% Triton X-100 for 30 min, and stained with TUNEL reagents (Roche, Germany) for 1 h. In addition, ventricular samples were dyed using DAPI (Sigma, USA) after TUNEL staining. TUNEL-positive cells were detected using a fluorescence microscope.
2.6. Lipid peroxidation analysis
Lipid peroxidation in the rats was analyzed by measuring the levels of malondialdehyde (MDA) using the thiobarbituric acid reaction. In short, rat brains were removed after reperfusion and homogenized. The tissue homogenates were incubated with 10% trichloroacetic acid (TCA) solution and 0.67% thiobarbituric acid (TBA) solution at 100°C for 30 min. The supernatants were transferred into 96-well plates, and the absorption at 532 nm was measured using a microplate reader. The MDA concentration was calculated based on a standard curve.
2.7. NO production analysis
NO production was analyzed using the Griess assays. In short, the brain tissues were entirely removed and homogenized in a saline solution on ice. The supernatants were reacted with a solution containing 0.1% N-1-naphthyl ethylenediamine dihydrochloride, 5% H3PO4, and 1% sulfanilamide at room temperature in 96-well plates in the dark. After that, the supernatants were transferred into 96-well plates, and absorptions at 532 nm were measured using a microplate reader (BioTek Instruments, USA).
2.8. Activity measurement of antioxidant enzymes
Brain tissues were removed and homogenized in a saline solution on ice. Superoxide dismutase (SOD) activity, glutathione (GSH) content, and glutathione peroxidase (GSH-Px) activity were determined using a SOD assay kit, a GSH assay kit (Cayman Company, USA), and a GSH-Px assay kit (Cayman Company, USA), respectively, by measuring absorptions at 450 nm, 405 nm, and 340 nm, respectively, with a microplate reader (BioTek Instruments, Winooski, USA).
2.9. Western blot analysis
Total proteins were extracted, quantified using a BCA kit, separated by SDS-PAGE, and transferred onto PVDF membranes. The membranes were incubated overnight with primary antibodies against caspase-3, Bax, and Bcl-2 at dilution 1:1000 from Cell Signaling Technology, USA, and p-p65, COX-2, Nrf2, Lamin B1, HO-1 and β-actin at dilution 1:1000 from Abcam, USA. The membranes were then incubated with proper second antibodies for 1 h. The signals were visualized using Odyssey CLx Infrared Imaging System and quantified using ImageJ software.
2.10. Statistical analysis
Data were expressed as mean ± SD and quantitatively analyzed using Graphpad prism 7. The neurobehavioral scores were analyzed using a non-parametric (Mann-Whitney) U test. Differences among multiple groups and between two groups were analyzed using one-way ANOVA and unpaired Student’s t-test, respectively. P < 0.05 were considered statistically significant.
3. Results
The study explored whether GJ, a compound of S. chinensis, plays a role in attenuating CIR injury in the middle cerebral artery occlusion/reperfusion rat model. We found that GJ relieved neuronal injury, attenuated neurological loss of the hippocampus, repressed apoptosis of ischemic tissues, exerted an anti-inflammatory and antioxidant effect, and inhibited I/R injury-induced lipid peroxidation.
3.1. GJ relieves neuronal injury in I/R rats
To understand GJ’s role in modulating CIR injury, we constructed a MCAO/R rat model. shows the GJ structure () and experimental flowchart (). The neurological scores of rats were significantly increased by the I/R treatment at 24 hours after reperfusion, and GJ treatment dose-dependently reduced this increase (P < 0.01, ). Meanwhile, TTC staining showed that GJ treatment inhibited I/R-induced cerebral infarction (P < 0.01, )) and reduced brain water content (P < 0.01, ) in rats in a dose-dependent manner. Furthermore, we found that 80 mg/kg GJ treatment decreased the infarct volume in I/R rats at 6 h, 12 h, and 48 h of reperfusion. Altogether, these suggest that GJ relieves neuronal injury in I/R rats.
Figure 1. GJ relieves I/R rat neuronal injury. (a) GJ chemical structure. (b) Experimental protocol schematic diagram. (c-f) MCAO/R rats were treated with GJ at indicated doses (n = 6). (c) Rat neurological deficit scores were measured after 24 h of reperfusion. (d) TC staining of cerebral infarction in rats. (e) Rat brain water content was calculated after 24 h of reperfusion. (f) TC staining of cerebral infarction after reperfusion in the rats. ** P < 0.01, # P < 0.05, ## P < 0.01. Data are presented as mean ± SD.
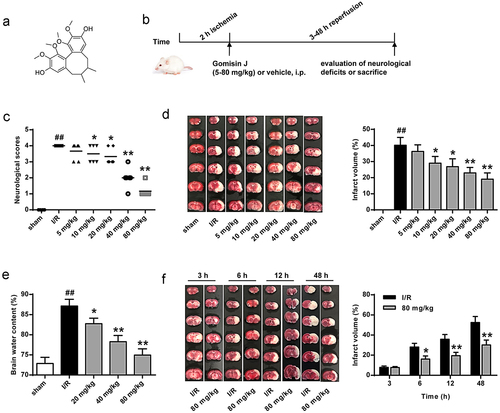
3.2. GJ attenuates neurological loss in I/R rat hippocampus
Next, we further explored GJ’s neuroprotective function by evaluating neurons from the hippocampus in the I/R rat model. Hematoxylin and eosin staining revealed that I/R treatment reduced the survival of neurons in the hippocampus, and GJ treatment dose-dependently rescued this phenotype (, p < 0.01). These suggest that GJ attenuates neurological loss in I/R rat hippocampus.
3.3. GJ represses I/R rat ischemic tissues apoptosis
We then assessed the role of GJ in modulating apoptosis of ischemic tissues in I/R rats using TUNEL staining. The number of TUNEL positive cells was increased in the cerebral cortex tissues of I/R rats, whereas GJ treatment blocked this elevation (P < 0.01, ). In addition, the levels of pro-apoptotic cleaved caspase-3 and Bax expression were enhanced, while the level of anti-apoptotic Bcl-XL expression was reduced in the I/R rats, and these changes were reversed by GJ treatment (, p < 0.01). Altogether, these indicate that GJ represses the apoptosis of ischemic tissues in I/R rats.
Figure 3. GJ represses apoptosis of ischemic tissues in I/R rats. MCAO/R rats were constructed and treated with GJ at indicated doses (n = 6). The apoptosis of cerebral cortex tissues was analyzed by TUNEL staining. * P < 0.05, ** P < 0.01, ## P < 0.01. Data are presented as mean ± SD.
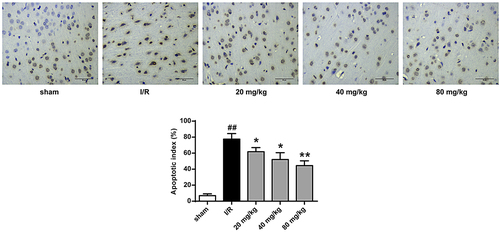
Figure 4. GJ regulates apoptotic proteins in I/R rat ischemic tissues. (a-d) MCAO/R rats were constructed and treated with GJ at indicated doses (n = 6). β-actin, cleaved caspase-3, Bax, and Bcl-XL levels was analyzed by Western blot. * P < 0.05, ** P < 0.01, ## P < 0.01. Data are presented as mean ± SD.
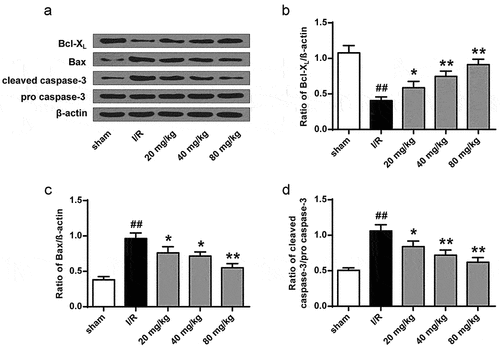
3.4. GJ inhibits oxidative stress and inflammation in I/R rats
Next, to further explore the anti-inflammatory effect of GJ, we measured p-p65 of NF-κB and COX-2 levels in I/R rat brain tissues. Significantly, I/R treatment enhanced p-p65 and COX-2 levels in rats, and these enhancements were reduced by GJ treatment in a dose-dependent manner (P < 0.01, ). Meanwhile, the NO level in I/R rat brain was reduced by GJ treatment in a dose-dependent manner (P < 0.01, ). Together, these data suggest that GJ treatment reduces inflammation in I/R rats.
Figure 5. GJ inhibits inflammation in I/R rats. (a-d) MCAO/R rats were constructed and treated with GJ at indicated doses (n = 6). (a-c) NF-κB (p-p65), COX-2, and β-actin expression was measured. (d) NO levels were analyzed in rats. * P < 0.05, ** P < 0.01, ## P < 0.01. Data are presented as mean ± SD.
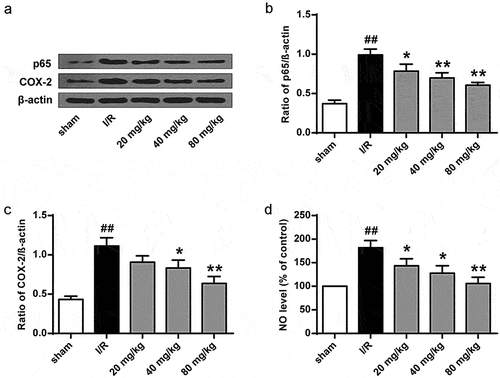
To further investigate GJ’s antioxidant function in I/R rats, we examined Nrf2 nuclear translocation and HO-1 expression. The results showed that Nrf2 nuclear translocation and HO-1 expression were significantly enhanced by GJ in a dose-dependent manner in I/R rats (P < 0.01, ). Meanwhile, the activities of antioxidant enzymes SOD () and GSH-Px () and the level of GSH () were reduced by I/R treatment in rats (P < 0.01), while GJ treatment dose-dependently reversed these reductions. These results indicate that GJ exerts an antioxidant role in I/R rats.
Figure 6. GJ inhibits oxidative stress in I/R rats. (a-f) MCAO/R rats were constructed and treated with GJ at indicated doses (n = 6). (a-c) Nucleus Nrf2, Lamin B1, HO-1, and β-actin expression was measured by Western blot. (d-f) SOD and GSH-Px activities and GSH content were analyzed. * P < 0.05, ** P < 0.01, ## P < 0.01. Data are presented as mean ± SD.
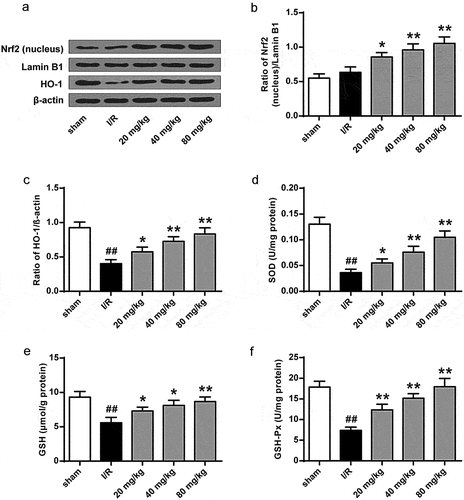
3.5. GJ decreases lipid peroxidation in I/R rats
To further investigate GJ’s function in I/R injury-induced lipid peroxidation, we measured MDA levels in I/R rat brain tissues. Our data showed that I/R significantly increased MDA level in the rats, and GJ treatment dose-dependently reduced this enhancement (P < 0.01, ). These data suggest that GJ represses lipid peroxidation in I/R rats.
4. Discussion
CIR injury is one of the most severe cerebrovascular disorders [Citation4] and is correlated with inflammatory response, oxidative stress, and hypoxia [Citation6]. Recently, natural compounds of traditional Chinese herbs have been used to treat cerebrovascular diseases [Citation9–11]. GJ, a principal constituent of S. Chinensis, has presented multiple biomedical activities, including anti-inflammatory effects and vascular relaxation [Citation19–21]. In this study, we found that GJ treatment attenuates CIR injury by inducing anti-apoptotic, anti-inflammatory, and antioxidant effects in rats.
In recent years, natural compounds have been identified to modulate CIR injury. For instance, hexahydrocurcumin prevents CIR injury by inhibiting oxidant stress and inflammation in the stroke rat model [Citation27]. YiQi Tongluo Granule attenuates CIR injury through regulating CaMKII and GluN2B via NMDAR-ERK1/2 signaling in rats [Citation28]. Trametenolic acid B reduces CIR injury by modulating the microRNA-10a/PI3K/Akt/mTOR axis [Citation29]. Ginkgo diterpene lactones repress CIR-induced inflammation response via the TLR4/NF-κB signaling in rat astrocytes [Citation30]. Qingkailing injection protects against CIR injury and regulates the AMPK/NLRP3 inflammation signaling [Citation31]. Here, we first identified that GI could relieve CIR-induced neuronal injury and neurological loss of the hippocampus in I/R rats, suggesting that GI protects against CIR-related brain dysfunction. Our study provides valuable evidence for GI’s function in cerebrovascular disease development.
Inhibiting inflammation, apoptosis, oxidative stress, and lipid peroxidation can attenuate CIR injury. For instance, RTN1-C interferes CIR injury by regulating apoptosis and ER stress processes [Citation22]. Trim47 inhibits CIR injury by modulating inflammation and apoptosis [Citation32]. DUSP14 relieves CIR injury through inhibiting apoptosis and inflammation by activating Nrf-2 [Citation33]. MicroRNA-106b-5p reduces CIR injury by repressing apoptosis and oxidative stress in rats [Citation34]. Nodal reduces CIR injury by inhibiting inflammation and oxidative stress [Citation35]. Theaflavin inhibits CIR injury by suppressing oxidative stress [Citation36]. Biochanin A induces neuroprotection against CIR injury by inhibiting oxidative stress and inflammation signaling in rats [Citation37]. Monosialotetrahexosylganglioside protects CIR injury by inhibiting lipid peroxidation [Citation38]. Moreover, as a natural component of S. Chinensis, GJ is well known to have an anti-cancer effect [Citation39]. It has been found that GJ is able to inhibit inflammation and oxidative stress [Citation20,Citation40,Citation41]. Our study showed that GJ inhibits inflammation, apoptosis, oxidative stress, and lipid peroxidation in I/R rats. Our study has some limitations. First, we mainly used young animals instead of old animals. Our results need to be further evaluated in old animals in the future [Citation42]. In addition, our study did not explore the effect of GJ treatment on transport proteins [Citation43]. Moreover, the effects of GJ treatment on some key immune cells, such as leukocytes and neutrophils, will be explored in future studies [Citation44].
5. Conclusions
In conclusion, we identified that GJ attenuates CIR injury by inducing anti-apoptotic, anti-inflammatory, and antioxidant effects in vivo. This study provides new insight into GJ’s function in stroke-related CIR injury and indicates that GJ may serve as a potential candidate for stroke-related CIR injury treatment.
Availability of supporting data
The data that support the findings of this study are available on request from the corresponding author.
Authors’ contributions
Xiaoli Min and Linping Zhao: study concepts, literature research, clinical studies, data analysis, experimental studies, manuscript writing and review; Xiaoli Min and Ying Shi: study design, literature research, experimental studies and manuscript editing; Jian Wang and Hongling LV: definition of intellectual content, clinical studies, data acquisition and statistical analysis; Xiaoxiao Song and Qunyuan Zhao: data acquisition, manuscript preparation and data analysis; Qing Zhao, Rui Jing, and Jiayi Hu: data acquisition and statistical analysis.
All authors have read and approved the submission of the manuscript.
Ethical approval and consent to participate
All procedures were approved by The Second Affiliated Hospital of Kunming Medical University Animal Ethics Committee (Approval No. KMU26532) and completed in line with the standards set out in the principles on ethical animal research outlined in the Second Affiliated Hospital of Kunming Medical University of Health Laboratory Animal Care and Use Guidelines.
Acknowledgements
We thank the Natural Science Foundation of China (No.81960219) and Yunnan Province Applied Basic Research Foundation Chinese Medicine Joint Project (No.2018FF001(-013)) for supporting and approving.
Disclosure statement
No potential conflict of interest was reported by the author(s).
Additional information
Funding
References
- Zhang A, Deng W, Zhang B, et al. Association of lipid profiles with severity and outcome of acute ischemic stroke in patients with and without chronic kidney disease. Neurol Sci. 2020 Oct 13;42(6):2371–2378.
- Chen B, Yang L, Chen J, et al. Inhibition of Connexin43 hemichannels with Gap19 protects cerebral ischemia/reperfusion injury via the JAK2/STAT3 pathway in mice. Brain Res Bull. 2019;146:124–135.
- Li HF, Hu SM, Song HQ. Door-to-Needle time and long-term outcomes in patients with stroke. Jama. 2020;324(14):1467.
- Zou X, Xie L, Wang W, et al. FK866 alleviates cerebral pyroptosis and inflammation mediated by Drp1 in a rat cardiopulmonary resuscitation model. Int Immunopharmacol. 2020;89(Pt A):107032.
- Zuo G, Zhang D, Mu R, et al. Resolvin D2 protects against cerebral ischemia/reperfusion injury in rats. Mol Brain. 2018;11(1):9.
- Wu XJ, Sun XH, Wang SW, et al. Mifepristone alleviates cerebral ischemia-reperfusion injury in rats by stimulating PPAR gamma. Eur Rev Med Pharmacol Sci. 2018;22(17):5688–5696.
- Liang W, Lin C, Yuan L, et al. Preactivation of Notch1 in remote ischemic preconditioning reduces cerebral ischemia-reperfusion injury through crosstalk with the NF-kappaB pathway. J Neuroinflammation. 2019;16(1):181.
- Kahl A, Blanco I, Jackman K, et al. Cerebral ischemia induces the aggregation of proteins linked to neurodegenerative diseases. Sci Rep. 2018;8(1):2701.
- Sun K, Fan J, Han J. Ameliorating effects of traditional Chinese medicine preparation, Chinese materia medica and active compounds on ischemia/reperfusion-induced cerebral microcirculatory disturbances and neuron damage. Acta Pharm Sin B. 2015;5(1):8–24.
- Liu X, Tan W, Yang F, et al. Shengmai injection reduces apoptosis and enhances angiogenesis after myocardial ischaemia and reperfusion injury in rats. Biomed Pharmacother. 2018;104:629–636.
- Sezen ŞC, Kucuk A, Ozer A, et al. Assessment of the effects of levosimendan and thymoquinone on lung injury after myocardial ischemia reperfusion in rats. Drug Des Devel Ther. 2018;12:1347–1352.
- Meng X, Xie W, Xu Q, et al. Neuroprotective Effects of Radix Scrophulariae on Cerebral Ischemia and Reperfusion Injury via MAPK Pathways. Molecules. 2018;23(9):9.
- Choo SH, Sung HH, Chae MR, et al. Effects of Schisandra chinensis extract on the relaxation of isolated human prostate tissue and smooth muscle cell. J Ethnopharmacol. 2014;156:271–276.
- Zhao W, Geng D, Li S, et al. LncRNA HOTAIR influences cell growth, migration, invasion, and apoptosis via the miR-20a-5p/HMGA2 axis in breast cancer. Cancer Med. 2018;7(3):842–855.
- Zhang M, Xu L, Yang H. Schisandra chinensis fructus and its active ingredients as promising resources for the treatment of neurological diseases. Int J Mol Sci. 2018;19:7.
- Sowndhararajan K, Deepa P, Kim M, et al. An overview of neuroprotective and cognitive enhancement properties of lignans from Schisandra chinensis. Biomed Pharmacother. 2018;97:958–968.
- Nowak A, Zaklos-Szyda M, Blasiak J, et al. Potential of Schisandra chinensis (Turcz.) Baill. in Human Health and Nutrition: a Review of Current Knowledge and Therapeutic Perspectives. Nutrients. 2019;11(2):333.
- Oh SY, Kim YH, Bae DS, et al. Anti-inflammatory effects of gomisin N, gomisin J, and schisandrin C isolated from the fruit of Schisandra chinensis. Biosci Biotechnol Biochem. 2010;74(2):285–291.
- Park JY, Choi YW, Yun JW, et al. Gomisin J from Schisandra chinensis induces vascular relaxation via activation of endothelial nitric oxide synthase. Vascul Pharmacol. 2012;57(2–4):124–130.
- Ye BH, Lee SJ, Choi YW, et al. Preventive effect of gomisin J from Schisandra chinensis on angiotensin II-induced hypertension via an increased nitric oxide bioavailability. Hypertens Res. 2015;38(3):169–177.
- Kim M, Lim SJ, Lee H-J, et al. Gomisin J Inhibits Oleic Acid-Induced Hepatic Lipogenesis by Activation of the AMPK-Dependent Pathway and Inhibition of the Hepatokine Fetuin-A in HepG2 Cells. J Agric Food Chem. 2015;63(44):842–855.
- Gong L, Tang Y, An R, et al. RTN1-C mediates cerebral ischemia/reperfusion injury via ER stress and mitochondria-associated apoptosis pathways. Cell Death Dis. 2017;8(10):e3080.
- Hu YW, Li HT, Li RL, et al. Protective effects of Schisandrin B against D-GalN-induced cell apoptosis in human hepatocyte (L02) cells via modulating Bcl-2 and Bax. Bioengineered. 2021;12(1):7205–7214.
- Du HY, Wang R, Li JL, et al. Ligustrazine induces viability, suppresses apoptosis and autophagy of retinal ganglion cells with ischemia/reperfusion injury through the PI3K/Akt/mTOR signaling pathway. Bioengineered. 2021;12(1):507–515.
- Longa EZ, Weinstein PR, Carlson S, et al. Reversible middle cerebral artery occlusion without craniectomy in rats. Stroke. 1989;20(1):84–91.
- Li L, Yu Q, Liang W. Use of 2,3,5-triphenyltetrazolium chloride-stained brain tissues for immunofluorescence analyses after focal cerebral ischemia in rats. Pathol Res Pract. 2018;214(1):174–179.
- Wicha P, Tocharus J, Janyou A, et al. Hexahydrocurcumin protects against cerebral ischemia/reperfusion injury, attenuates inflammation, and improves antioxidant defenses in a rat stroke model. PLoS One. 2017;12(12):e0189211.
- Wu SP, Li D, Wang N, et al. YiQi Tongluo Granule against Cerebral Ischemia/Reperfusion injury in rats by freezing GluN2B and CaMK II through NMDAR/ERK1/2 Signaling. Chem Pharm Bull (Tokyo). 2019;67(3):244–252.
- Wang J, Wang A, He H, et al. Trametenolic acid B protects against cerebral ischemia and reperfusion injury through modulation of microRNA-10a and PI3K/Akt/mTOR signaling pathways. Biomed Pharmacother. 2019;112:108692.
- Fu X, Zhang J, He X, et al. Circular RNA MAN2B2 promotes cell proliferation of hepatocellular carcinoma cells via the miRNA-217/MAPK1 axis. J Cancer. 2020;11(11):3318–3326.
- Ma C, Wang X, Xu T, et al. Qingkailing injection ameliorates cerebral ischemia-reperfusion injury and modulates the AMPK/NLRP3 Inflammasome Signalling pathway. BMC Complement Altern Med. 2019;19(1):320.
- Hao MQ, Xie LJ, Leng W, et al. Trim47 is a critical regulator of cerebral ischemia-reperfusion injury through regulating apoptosis and inflammation. Biochem Biophys Res Commun. 2019;515(4):651–657.
- Jianrong S, Yanjun Z, Chen Y, et al. DUSP14 rescues cerebral ischemia/reperfusion (IR) injury by reducing inflammation and apoptosis via the activation of Nrf-2. Biochem Biophys Res Commun. 2019;509(3):713–721.
- Li P, Shen M, Gao F, et al. An Antagomir to MicroRNA-106b-5p ameliorates cerebral ischemia and reperfusion injury in rats via inhibiting apoptosis and oxidative stress. Mol Neurobiol. 2017;54(4):2901–2921.
- Cui Y, Wang JQ, Shi XH, et al. Nodal mitigates cerebral ischemia-reperfusion injury via inhibiting oxidative stress and inflammation. Eur Rev Med Pharmacol Sci. 2019;23(13):5923–5933.
- Li R, Li X, Wu H, et al. Theaflavin attenuates cerebral ischemia/reperfusion injury by abolishing miRNA1283pmediated Nrf2 inhibition and reducing oxidative stress. Mol Med Rep. 2019;20(6):4893–4904.
- Guo M, Lu H, Qin J, et al. Biochanin A provides neuroprotection against cerebral Ischemia/Reperfusion Injury by Nrf2-Mediated inhibition of oxidative stress and inflammation signaling pathway in rats. Med Sci Monit. 2019;25:8975–8983.
- Li M, Liao YJ, Hou GH, et al. Monosialotetrahexosylganglioside protect cerebral ischemia/reperfusion injury through upregulating the expression of tyrosine hydroxylase by inhibiting lipid peroxidation. Biomed Pharmacother. 2016;84:1923–1929.
- Wang DY, Li N, Cui YL. Long Non-coding RNA CCAT1 Sponges miR-454 to promote chemoresistance of ovarian cancer cells to cisplatin by regulation of surviving. Cancer Res Treat. 2020;52(3):798–814.
- Yoshikawa A, Saito Y, Maruyama K. Lignan compounds and 4,4’-dihydroxybiphenyl protect C2C12 cells against damage from oxidative stress. Biochem Biophys Res Commun. 2006;344(1):394–399.
- Ryu EY, Park SY, Kim SG, et al. Anti-inflammatory effect of heme oxygenase-1 toward Porphyromonas gingivalis lipopolysaccharide in macrophages exposed to gomisins A, G, and J. J Med Food. 2011;14(12):1519–1526.
- Popa-Wagner A, Dumitrascu DI, Capitanescu B, et al. Dietary habits, lifestyle factors and neurodegenerative diseases. Neural Regen Res. 2020;15(3):394–400.
- Dazert P, Suofu Y, Grube M, et al. Differential regulation of transport proteins in the periinfarct region following reversible middle cerebral artery occlusion in rats. Neuroscience. 2006;142(4):1071–1079.
- Wang C, Borger V, Sardari M, et al. Mesenchymal Stromal cell-derived small extracellular vesicles induce ischemic neuroprotection by modulating leukocytes and specifically neutrophils. Stroke. 2020;51(6):1825–1834.