ABSTRACT
Circular RNAs (CircRNAs) have attracted increasing attention in the diagnosis and treatment of human cancers. CircUBAP2 has been identified to promote the progression of triple-negative breast cancer (TNBC), but the function of circUBAP2 in the cisplatin (DDP) resistance of TNBC remains obscure. Our investigation showed that circUBAP2 was significantly upregulated in DDP-resistant TNBC and TNBC sensitivity to DDP could be enhanced by silencing of circUBAP2. Moreover, circUBAP2 was revealed to be a ceRNA for miR-300 to upregulate the expression of anti-silencing function 1B histone chaperone (ASF1B). The effect of circUBAP2/miR-300/ASF1B axis on DDP resistance of TNBC was evaluated by rescue experiments, which demonstrated that circUBAP2 inhibited TNBC sensitivity to DDP through miR-300/ASF1B axis. Furthermore, it was discovered that ASF1B activated PI3K/AKT/mTOR signaling to facilitate the DDP resistance of TNBC cells. In summary, this research revealed a novel regulatory mechanism that circUBAP2 functioned as ceRNA of miR-300 to upregulate ASF1B, which further triggered the PI3K/AKT/mTOR (PAM) signaling to enhance the DDP resistance of TNBC.
Graphical Abstract
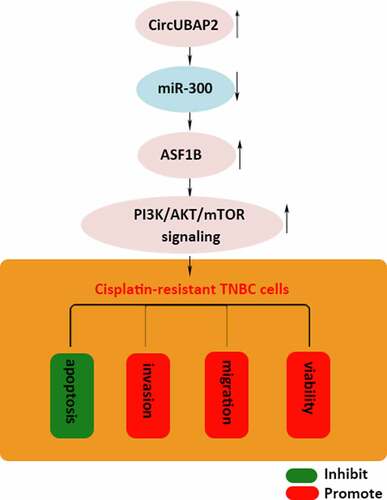
Introduction
Breast cancer (BC) has the highest incidence and the leading mortality rate among all the malignant tumors in women worldwide [Citation1]. With the progression of diagnostic techniques and specialized treatments, the mortality rate of breast cancer has shown a decreasing tendency in recent years [Citation2]. Triple-negative breast cancer (TNBC) is a heterogeneous subtype of BC negative with the expression of estrogen receptor, progesterone receptor, or human epidermal growth factor receptor 2 (HER2) [Citation3]. Currently, patients diagnosed with TNBC mainly receive non-personalized treatments like radiotherapy and chemotherapy [Citation4]. Cisplatin (DDP) is an anti-cancer drug commonly used in BC [Citation5] and has been demonstrated to improve the pathological complete response of TNBC [Citation6]. However, the efficacy of chemotherapy is often limited by the emergence of DDP resistance during the treatment of TNBC.
Circular RNAs (circRNAs) are a type of non-coding RNAs with a stable circular loop structure [Citation7] and have been uncovered to regulate the biological processes in various cancers [Citation8]. Besides, circRNAs have been revealed to mediate the drug resistance of different types of cancers, including hepatocellular carcinoma [Citation9], non-small cell lung cancer [Citation10], and gastric cancer [Citation11]. Circular RNA UBAP2 (circUBAP2) has been previously demonstrated to participate in the development of human cancers. For instance, circUBAP2 promotes the malignancy of colorectal cancer through miR-199a/VEGFA axis [Citation12]. Knockdown of circUBAP2 suppresses the development of papillary thyroid cancer cells by elevating the expression of miR-370-3p [Citation13]. CircUBAP2 exacerbates osteosarcoma cell malignancy through miR‑204‑3p/HMGA2 pathway [Citation14]. More importantly, circUBAP2 has been identified to be upregulated in TNBC and predict poor prognosis [Citation15]. Nevertheless, the role and function of circUBAP2 in DDP-resistant TNBC are still unclear.
The current research is designed to investigate the molecular mechanism of circUBAP2 in the DDP resistance of TNBC. We hypothesized that circUBAP2 could be involved in the DDP resistance of TNBC. Our findings might offer a novel treatment strategy for DDP-resistant TNBC patients.
Materials and methods
Tissue specimens
A total of 57 pairs of TNBC tissues and para-cancerous tissues were obtained from TNBC patients at The Affiliated Shuyang Hospital of Xuzhou Medical University. The samples were put in liquid nitrogen immediately after collection and stored at −80°C for analysis. All protocols were approved by the Ethical Committee of The Affiliated Shuyang Hospital of Xuzhou Medical University. Written consent was signed by all patients involved.
Cell culture and construction of DDP-resistant cells
Human TNBC cell lines (HCC1937, BT-549 and MDA-MB-436) and normal human breast epithelial cell line MCF10A were obtained from ATCC (Manassas). MCF10A cells were cultured in DMEM (Gibco) containing 5% horse serum (Life Technologies), 20 ng/mL human epidermal growth factor (hEGF) (R&D Systems), 0.5 mg/mL hydrocortisone (Sigma), 10 µg/mL insulin, 100 U/mL penicillin, and 100 U/mL streptomycin. TNBC cell lines were cultured in DMEM with 10% heat-inactivated fetal bovine serum (FBS) (Gibco), 100 U/mL penicillin, 100 U/mL streptomycin, and 2 mmol/L L-glutamine. All cells were cultured at 37°C in a humidified 5% CO2 atmosphere cell incubator. DDP-resistant TNBC cells were constructed by culturing TNBC cells with increasing concentration of DDP for 24 h and then collected for subsequent experiments.
Cell transfection
Short hairpin RNA (shRNA) against circUBAP2 (shcircUBAP2#1 and shcircUBAP2#2), ASF1B (shASF1B), shNC, miR-300 mimics (miR-300 mimics) and miR-300 inhibitor (miR-300 inhibitor) with their negative controls (NC mimics and NC inhibitor), circUBAP2 and ASF1B over expression plasmid (pcDNA3.1/circUBAP2 and pcDNA3.1/ASF1B) and empty pcDNA3.1 vector were obtained from GenePharma (Shanghai). Transfection of these vectors into TNBC cells was conducted via Lipofectamine 2000 (Invitrogen).
Luciferase reporter assay
The wild-type (WT) and mutant (Mut) sequences of circUBAP2 and ASF1B were cloned into the pmirGLO vector (Promega Corporation). Following that, these vectors with shNC, shcircUBAP2, NC mimics and miR-300 mimics were co-transfected into TNBC cells using Lipofectamine 2000 (Invitrogen).
RT-qPCR
Total RNA from TNBC tissues or cells was separated using TRIzol reagent (Invitrogen). Briefly, cDNA was synthesized with a PrimeScript RT reagent kit (Takara). RT-qPCR was implemented with a SYBR Premix Ex Taq kit (TaKaRa) on an ABI 7500 RT-PCR system (Applied Biosystems). Relative gene expressions were normalized to GAPDH/U6 using the 2–ΔΔCT method.
Western blotting
Briefly, total proteins were isolated by RIPA buffer (Thermo Fisher Scientific). Then the proteins were separated by SDS-PAGE and transferred to PVDF membranes (Millipore) which were sealed with 5% nonfat milk and incubated with primary antibodies against p-AKT, AKT p-mTOR, mTOR, ASF1B and GAPDH. Secondary antibodies were added for incubation for 1 h. Protein signals were visualized with an ECL kit (Thermo Fisher Scientific) [Citation16].
CCK-8 assay
The cells (BT-549/DDP and MDA-MB-436/DDP) were seeded into 96-well plates (1x104 cells/well) and cultured for 0, 24, 48, and 72 h at 37°C and 5% CO2. Following that, 10 µl CCK-8 reagent (Beyotime) was added to each well and then incubated for an extra 4 h. Cell viability was measured with a microplate reader (Thermo Fisher Scientific) at 450 nm absorbance [Citation17]. IC50 values were calculated by treating the indicated cells with different concentrations of DDP for 24 h.
TUNEL assay
DDP-resistant TNBC cells (BT-549/DDP and MDA-MB-436/DDP) were washed with PBS and fixed in 4% paraformaldehyde solution for 1 h at 4°C. After permeabilization with 0.25% Triton-X 100, the cells were incubated in TUNEL enzyme for 1 h at 37°C. Subsequently, the TUNEL-stained cells were counterstained with DAPI at room temperature. Images of apoptotic cells were captured from ≥5 fields of view under a fluorescence microscope.
Wound-healing assay
Transfected cells were seeded into six-well plates and cultured up to ~100% confluence. After a 6 h starvation, wounds were created with a sterile 200-μL pipette tip. Then the cells were cultured for 24 h after washing. Wound closing images were observed before and after the 24-h culture.
Transwell invasion assay
Briefly, BT-549/DDP and MDA-MB-436/DDP cells were cultured in a serum-free medium in the top chamber precoated with Matrigel. Meanwhile, 600 μL complete medium was added to the bottom chambers. Following 48 h incubation, the invaded cells on the surface of the bottom chamber were stained with Harris hematoxylin solution (Sigma) and recorded by a light microscope [Citation18].
Statistical analysis
All experiments were repeated independently at least three times and data were analyzed using GraphPad Prism 5.0. One-way ANOVA or Student’s t-test was employed to analyze the difference between groups. Data were presented as mean ± standard deviation (SD). P values of < 0.05 were considered statistically significant.
Results
Our study aimed to investigate the expression, biological function, and downstream mechanism of circUBAP2 in DDP-resistant TNBC cells. Through a series of experiments, we demonstrated that circUBAP2 enhanced the DDP resistance of TNBC via miR-300/ASF1B/PI3K/AKT/mTOR axis.
circUBAP2 is upregulated in DDP-resistant TNBC cells
To explore the roles of circUBAP2 in the DDP-resistance of TNBC, the expression pattern of circUBAP2 was explored. Firstly, results from 2 GEO databases (GSE101123 and GSE101124) indicated that circUBAP2 was upregulated in BC compared with that in normal tissues (). RT-qPCR detected a significantly higher level of circUBAP2 in TNBC tissue samples () and cell lines (HCC1937, BT-549 and MDA-MB-436) () in comparison with para-cancerous tissues and MCF10A cells, respectively. Subsequently, circUBAP2 expression was compared between DDP-resistant TNBC cells and their parental cells. The increased IC50 value indicated successfully constructed BT-549/DDP and MDA-MB-436/DDP cells (). RT-qPCR indicated markedly elevated levels of circUBAP2 in DDP-resistant cells (). Several studies have identified that circUBAP2 is derived from the UBAP2 gene (chr9:33,953,282-33,973,235) by head-to-tail splicing [Citation19–21]. To verify the circular characteristics of circUBAP2, total RNA from BT-549/DDP and MDA-MB-436/DDP cells was treated with or without RNase R and we found that compared to with linear form of UBAP2, circUBAP2 was resistant to RNase R (). In addition, the results of nuclear-cytoplasmic fractionation indicated that circUBAP2 was preferentially localized in the cytoplasm (). Overall, it was demonstrated that circUBAP2 expression was upregulated in TNBC, especially in DDP-resistant TNBC cells.
Figure 1. CircUBAP2 was abundantly expressed in TNBC tissues and cells, particularly in DDP-resistant cells. (a) GEO databases showed the expression of circUBAP2 in normal and BC tissues. (b and c) RT-qPCR compared the expression of circUBAP2 between TNBC tissues and normal tissues (b) and between TNBC cell lines and normal human breast epithelial cells (c). (d) IC50 value was compared between TNBC cells (BT-549 and MDA-MB-436) and their matched DDP-resistant cells. (e) CircUBAP2 expression was detected in both DDP-resistant and nonresistant TNBC cells. (f) Relative expression of circUBAP2 and UBAP2 mRNA in DDP-resistant cells was detected by RT-qPCR in the presence or absence of RNase R. (g) circUBAP2 was mainly located in the cytoplasm by nuclear-cytoplasmic fractionation assay. *p < 0.05; ** p < 0.01.
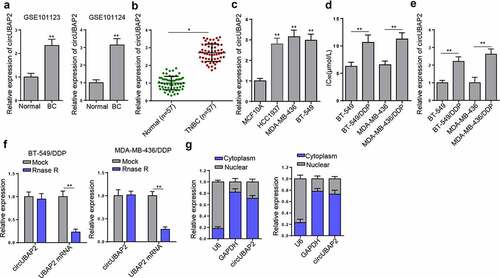
circUBAP2 augments DDP resistance of TNBC cells
To verify the hypothesis that circUBAP2 could regulate the DDP-resistance of TNBC cells, shcircUBAP2 was transfected into BT-549/DDP and MDA-MB-436/DDP cells (). Subsequently, CCK-8 assay detected reduced IC50 value and cell viability following circUBAP2 knockdown (). Wound healing and Transwell assays witnessed markedly retarded cell migration and invasion abilities following circUBAP2 depletion (). Finally, TUNEL assay indicated that circUBAP2 deficiency noticeably increased cell apoptosis ( and g). All in all, the sensitivity of TNBC cells to DDP could be attenuated by silencing circUBAP2.
Figure 2. CircUBAP2 knockdown sensitized TNBC cells to DDP. (a) CircUBAP2 expression before and after transfection of shcircUBAP2#1/2 in BT-549/DDP and MDA-MB-436/DDP cells. (b-g) The influence of circUBAP2 deficiency on the IC50 (b), cell proliferation (c), cell migration (d), cell invasion (e), and cell apoptosis (f and g) was evaluated by CCK-8, wound healing, Transwell, and TUNEL assays. *p < 0.05; ** p < 0.01.
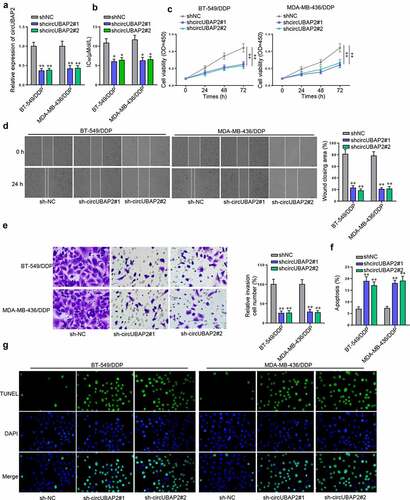
circUBAP2 serves as a ceRNA for miR-300 to upregulate ASF1B
Next, we explored the downstream mechanism of circUBAP2. Starbase website predicted that circUBAP2 might target miR-300 and three databases (microT, PITA, and TargetScan) predicted that miR-300 possibly interacted with ASF1B (). To verify, RT-qPCR demonstrated that miR-300 overexpression efficiently reduced the expression of circUBAP2 and ASF1B in BT-549/DDP and MDA-MB-436/DDP cells (). In addition, circUBAP2 knockdown in these two cell lines greatly elevated the level of miR-300 but downregulated the expression of ASF1B ( and e), implicating that circUBAP2 might sponge miR-300 to upregulate ASF1B expression. Moreover, miR-300 overexpression or circUBAP2 knockdown significantly downregulated the protein levels of ASF1B (). Subsequently, luciferase reporter assay was performed to validate the interactions. As illustrated in , miR-300 mimics attenuated the luciferase activity of circUBAP2-WT. Meanwhile, cells treated with miR-300 mimics or shcircUBAP2 both exhibited decreased luciferase activity of ASF1B-WT (). Moreover, downregulated miR-300 expression and upregulated ASF1B expression were observed in TNBC tissues by RT-qPCR (). Pearson’s analysis further identified the negative association between circUBAP2 and miR-300 or between miR-300 and ASF1B expressions as well as the positive correlation between circUBAP2 and ASF1B levels in TNBC tissues (). Taken together, circUBAP2 could upregulate ASF1B by absorbing miR-300.
Figure 3. CircUBAP2 regulates the miR-300/ASF1B axis in TNBC. (a) Binding sites predicted by Starbase between CircUBAP2 and miR-300 or between miR-300 and ASF1B. (b and c) mRNA levels of circUBAP2 and ASF1B in BT-549/DDP and MDA-MB-436/DDP cells following miR-300 overexpression. (d and e) mRNA levels of miR-300 and ASF1B in BT-549/DDP and MDA-MB-436/DDP cells after silencing circUBAP2. (f) Protein levels of ASF1B in BT-549/DDP and MDA-MB-436/DDP cells following miR-300 overexpression or circUBAP2 knockdown (g and h) The interaction among circUBAP2, miR-300, and ASF1B was verified by Luciferase reporter assay. (i) RT-qPCR results of miR-300 and ASF1B expressions in TNBC tissues. (j) Correlation analysis of the expressions of circUBAP2, miR-300, and ASF1B in TNBC tissues. *p < 0.05; ** p < 0.01.
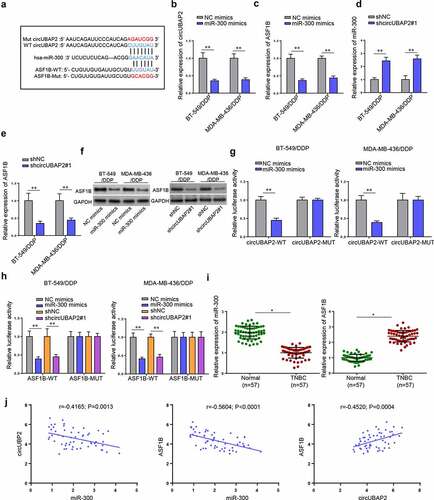
circUBAP2 regulates TNBC sensitivity to DDP through miR-300/ASF1B axis
To confirm the role of circUBAP2/miR-300/ASF1B pathway in the DDP resistance of TNBC cells, rescue experiments were conducted by co-transfecting shcircUBAP2#1 and miR-300 inhibitor into BT-549/DDP and MDA-MB-436/DDP cells. Following the transfection, it was observed that miR-300 inhibitor rescued the IC50 value suppressed by shcircUBAP2#1 (). Functional assays further indicated that miR-300 deficiency revived the blocked viability, migration, and invasion caused by shcircUBAP2#1, while suppressed the augmented cell apoptosis induced by circUBAP2 knockdown (). On the contrary, transfection of pcDNA3.1/ASF1B into BT-549/DDP and MDA-MB-436/DDP cells abrogated the decrease of IC50 value, cell viability, cell migration, and cell invasion as well as the increase of cell apoptosis resulted from miR-300 abundance (). To summarize, circUBAP2 enhanced DDP resistance of TNBC cells by suppressing miR-300 to increase the level of ASF1B.
Figure 4. circUBAP2 knockdown could enhance the sensitivity of TNBC cells to DDP through miR-300/ASF1B pathway. (a-e) After transfection of shNC, shcircUBAP2, and shcircUBAP2 + miR-300 inhibitor, the IC50 value (a), proliferation (b), migration (c), invasion (d), and apoptosis (e) of BT-549/DDP and MDA-MB-436/DDP cells were detected. (f-j) After transfection of NC mimics, miR-300 mimics, and miR-300 mimics + pcDNA3.1/ASF1B, the IC50 value (f), viability (g), migration (h), invasion (i), and apoptosis (j) of BT-549/DDP and MDA-MB-436/DDP cells were detected. *p < 0.05; ** p < 0.01.
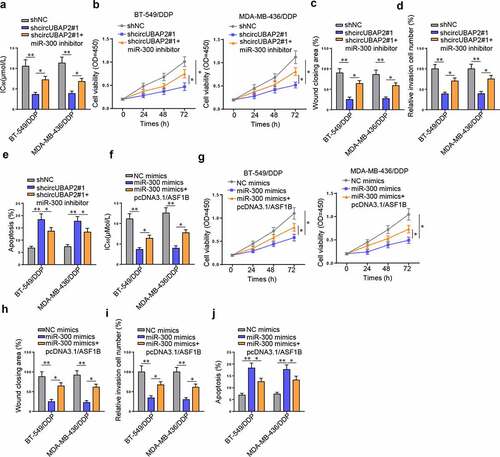
ASF1B activates PI3K/AKT/mTOR (PAM) pathway to promote DDP resistance of TNBC cells
PAM pathway was reported to participate in the tumorigenesis and chemoresistance of TNBC [Citation22,Citation23]. To verify the impact of ASF1B on the PAM pathway in DDP-resistant TNBC cells, ASF1B was silenced in BT-549/DDP and MDA-MB-436/DDP cells (). Next, Western blot showed that ASF1B depletion markedly decreased the levels of p-AKT and p-mTOR, but that of AKT and mTOR were not affected, indicating the inhibitory effect of ASF1B silencing on the PAM pathway (). Subsequently, Western blot confirmed that the introduction of 740Y-P re-elevated p-AKT and p-mTOR protein levels suppressed by ASF1B depletion in BT-549/DDP and MDA-MB-436/DDP cells (). Besides, 740Y-P rescued the reduced IC50 value caused by shASF1B (). CCK-8, wound healing, and Transwell assays further demonstrated that 740Y-P overturned the inhibitory effect of shASF1B on the viability, migration, and invasion of DDP-resistant TNBC cells (). TUNEL assay indicated that the introduction of 740Y-P annulled the promotive effect of shASF1B on cell apoptosis (). In sum, ASF1B facilitated the resistance of TNBC cells to DDP by activating the PAM signaling.
Figure 5. The promotive effect of PAM pathway on the DDP-resistance of TNBC cells was activated by ASF1B. (a) Transfection efficiency of shASF1B into BT-549/DDP and MDA-MB-436/DDP cells. (b, c) Western blot detected the expressions of p-AKT, AKT p-mTOR, mTOR after silencing ASF1B (b) or co-transfected with shASF1B and 740Y-P (c) in BT-549/DDP and MDA-MB-436/DDP cells. (d-h) the IC50 value (d), viability (e), migration (f), invasion (g), and apoptosis (h) of BT-549/DDP and MDA-MB-436/DDP cells treated with shNC, shASF1B, and shASF1B + 740Y-P were detected. *p < 0.05; ** p < 0.01.
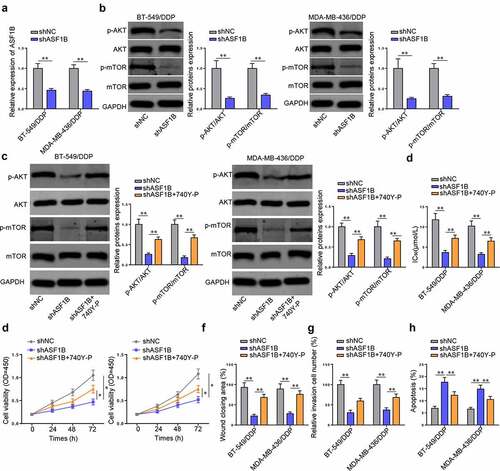
Discussion
Congenital or acquired drug resistance has greatly reduced the therapeutic effect for TNBC patients [Citation24]. Even though the drug-resistant mechanism in TNBC has been extensively investigated by researchers [Citation25–27], few studies focused on the resistance of TNBC to DDP. In this study, we explored the DDP resistance of TNBC on a molecular level and revealed that circUBAP2 was greatly elevated in TNBC tissues and DDP-resistant TNBC cells. By silencing circUBAP2, the resistance of TNBC cells to DDP was inhibited, indicating that circUBAP2 could act as a potential biomarker to enhance the sensitivity of TNBC cells to DDP therapies.
With the advancement in bioinformatics and high-throughput sequencing technologies, circRNAs have come into the spotlight [Citation28] and their roles in human cancers have gradually been revealed [Citation29]. To date, circRNAs have been reported to mediate the progression and chemoresistance of human cancers by sponging miRNAs in many cases [Citation30–32]. MiR-300 has been revealed as an oncogene or a tumor suppressor in various human cancers. For example, miR-300 targets ROS1 to inhibit cell viability and mobility in laryngeal squamous cell carcinoma [Citation33]. MiR‑300 suppresses FOXO1 expression to promote cell proliferation of liver cancer [Citation34]. MiR-300 inhibits epithelial-to-mesenchymal transition of oral squamous cell carcinoma and attenuates tumor malignancy [Citation35]. Herein, we identified that circUBAP2 directly targeted miR-300 and the suppressive effect of miR-300 on DDP resistance of TNBC cells could be reversed by circUBAP2.
Anti-silencing function 1 (ASF1) is an important histone H3–H4 chaperone that involves in the regulation of DNA replication, DNA repair, and transcription [Citation36]. ASF1A and ASF1B are the two paralogs of ASF1 [Citation37]. ASF1A primarily facilitates DNA repair and cell senescence, whereas ASF1B preferentially participates in cell proliferation [Citation38]. Interestingly, the function of ASF1B in malignant tumors has been revealed by multiple reports. For example, ASF1B stabilized CDK9 to promote cervical cancer progression [Citation39]. ASF1B accelerated the viability and mobility of clear cell renal cell carcinoma cells by activating the AKT pathway [Citation40]. ASF1B silence could inhibit the progression of prostate cancer through suppressing PI3K/Akt signaling [Citation41]. Particularly, ASF1B was reported as a predictor of the clinical outcomes of BC [Citation42]. Our findings demonstrated that the expression of ASF1B in TNBC was mediated by circUBAP2/miR-300 axis and circUBAP2 promoted TNBC cell resistance to DDP by upregulating ASF1B through suppressing miR-300.
The PAM pathway has been uncovered as a promising target in cancer treatment due to its frequent activation in multiple human cancers, including ovarian cancer [Citation43], non-small cell lung cancer [Citation44], and prostate cancer [Citation45]. Moreover, the PAM pathway has been revealed to interfere in the drug resistance of BC. For example, Li et al. reported that tumor-associated macrophages activated the PAM pathway to facilitate tumor progression and induce tamoxifen resistance in BC [Citation46]. Zong et al. reported that SIK2 knockdown could inhibit glycolysis and PAM signaling to improve the sensitivity of BC cells to DDP [Citation47]. Zhang et al. reported that ghrelin suppressed BC cell sensitivity to DDP through the PAM pathway [Citation48]. Particularly, the therapeutic significance of PAM signaling in TNBC was confirmed by multiple authors. For instance, Costa et al examined the clinical progress achieved in TNBC by targeting the PAM pathway [Citation49]. Khan et al. discovered that the activation of PAM signaling in TNBC could enhance resistance to HER2-targeted treatment, hormone treatment, and chemotherapy [Citation50]. Wu et al. reported that rhizoma amorphophalli inhibited TNBC cell proliferation, migration, invasion, and metastasis through the PAM pathway [Citation51]. In our research, it was uncovered that PAM pathway was regulated by ASF1B, and the knockdown of ASF1B could inhibit PAM signaling to enhance the sensitivity of TNBC cells to DDP therapies.
Conclusion
Our investigation revealed the functional role of circUBAP2/miR-300/ASF1B/PAM pathway in DDP-resistant TNBC. It was discovered that circUBAP2 targeted miR-300 to regulate the expression of ASF1B, which further triggered the PAM pathway to inhibit the sensitivity of TNBC cells to DDP. These findings suggested that circUBAP2 might be a promising therapeutic target for overcoming the DDP resistance of TNBC.
Highlights
CircUBAP2 is upregulated in DDP-resistant TNBC cells
CircUBAP2 augments DDP resistance of TNBC cells
CircUBAP2 regulates TNBC sensitivity to DDP through miR-300/ASF1B axis
ASF1B activates PAM pathway to promote DDP resistance of TNBC cells.
Disclosure statement
No potential conflict of interest was reported by the author(s).
Additional information
Funding
References
- Li HN, Li XR, Lv ZT, et al. Elevated expression of FREM1 in breast cancer indicates favorable prognosis and high-level immune infiltration status. Cancer Med. 2020;9(24):9554–9570.
- Corbeau I, Thezenas S. Inflammatory blood markers as prognostic and predictive factors in early breast cancer patients receiving neoadjuvant chemotherapy. Cancers. 2020;12(9):2666.
- Katayama H, Tsou P, Kobayashi M, et al. A plasma protein derived TGFβ signature is a prognostic indicator in triple negative breast cancer. NPJ Precis Oncol. 2019;3:10.
- Liu Y, Zhou Y, Ma X, et al. Inhibition lysosomal degradation of clusterin by protein kinase D3 promotes triple-negative breast cancer tumor growth. Advanced Science (Weinheim, Baden-Wurttemberg, Germany). 2021;8(4):2003205.
- You F, Li J, Zhang P, et al. miR106a promotes the growth of transplanted breast cancer and decreases the sensitivity of transplanted tumors to cisplatin. Cancer Manage Res. 2020;12:233–246.
- Subramani R, Estrada A, Dixon M, et al. Pregnancy inhibits mammary carcinogenesis by persistently altering the hypothalamic-pituitary axis. Cancers. 2021;13(13):3207.
- Qian C, Chen S, Li S, et al. Circ_0000003 regulates glutamine metabolism and tumor progression of tongue squamous cell carcinoma via the miR‑330‑3p/GLS axis. Oncol Rep. 2021;45(4). DOI:10.3892/or.2021.7996
- Lei M, Zheng G, Ning Q, et al. Translation and functional roles of circular RNAs in human cancer. Mol Cancer. 2020;19(1):30.
- Xu J, Ji L, Liang Y, et al. CircRNA-SORE mediates sorafenib resistance in hepatocellular carcinoma by stabilizing YBX1. Signal Transduction and Targeted Therapy. 2020;5(1):298.
- Hong W, Xue M, Jiang J, et al. Circular RNA circ-CPA4/ let-7 miRNA/PD-L1 axis regulates cell growth, stemness, drug resistance and immune evasion in non-small cell lung cancer (NSCLC). J Exp Clin Cancer Res. 2020;39(1):149.
- Huang X, Li Z, Zhang Q, et al. Circular RNA AKT3 upregulates PIK3R1 to enhance cisplatin resistance in gastric cancer via miR-198 suppression. Molecular Cancer. 2019;18(1):71.
- Dai J, Zhuang Y, Tang M, et al. CircRNA UBAP2 facilitates the progression of colorectal cancer by regulating miR-199a/VEGFA pathway. Eur Rev Med Pharmacol Sci. 2020;24(15):7963–7971.
- Xiong H, Yu J, Jia G, et al. Emerging roles of circUBAP2 targeting miR-370-3p in proliferation, apoptosis, and invasion of papillary thyroid cancer cells. Hum Cell. 2021;34(6):1866–1877.
- Ma W, Xue N, Zhang J, et al. circUBAP2 regulates osteosarcoma progression via the miR‑204‑3p/HMGA2 axis. Int J Oncol. 2021;58(3):298–311.
- Wang S, Li Q, Wang Y, et al. Upregulation of circ-UBAP2 predicts poor prognosis and promotes triple-negative breast cancer progression through the miR-661/MTA1 pathway. Biochem Biophysical Res Commun. 2018;505(4):996–1002.
- He W, Shi X, Guo Z, et al. Circ_0019693 promotes osteogenic differentiation of bone marrow mesenchymal stem cell and enhances osteogenesis-coupled angiogenesis via regulating microRNA-942-5p-targeted purkinje cell protein 4 in the development of osteoporosis. Bioengineered. 2022;13(2):2181–2193.
- Xu L, Tan Y, Xu F, et al. Long noncoding RNA ADIRF antisense RNA 1 upregulates insulin receptor substrate 1 to decrease the aggressiveness of osteosarcoma by sponging microRNA-761. Bioengineered. 2022;13(2):2028–2043.
- Xie F, Xiong Y, Yan J, et al. Circular RNA circ_0048764 promotes the development of breast cancer by regulating microRNA-1296-5p/tripartite motif containing 14 axis. Bioengineered. 2022;13(2):1963–1974.
- Liu B, Tian Y, Chen M, et al. CircUBAP2 promotes MMP9-mediated oncogenic effect via sponging miR-194-3p in hepatocellular carcinoma. Front Cell Dev Biol. 2021;9:675043.
- Chen F, Guo L, and Di J, et al., Circular RNA ubiquitin-associated protein 2 enhances autophagy and promotes colorectal cancer progression and metastasis via miR-582-5p/FOXO1 signaling. Yi chuan xue bao. 2021;48(12):1091-1103 .
- Qi T, Zhang D, Shi X, et al. Decreased circUBAP2 expression is associated with preeclampsia by limiting trophoblast cell proliferation and migration. Reproductive Sciences (Thousand Oaks, Calif.). 2021;28(8):2237–2245.
- Fan S, Yan S, Yang Y, et al. Actin-like protein 8 promotes the progression of triple-negative breast cancer via activating PI3K/AKT/mTOR pathway. Oncol Targets Ther. 2021;14:2463–2473.
- Hu H, Zhu J, Zhong Y, et al. PIK3CA mutation confers resistance to chemotherapy in triple-negative breast cancer by inhibiting apoptosis and activating the PI3K/AKT/mTOR signaling pathway. Ann Transl Med. 2021;9(5):410.
- Lopes N, Correia VG. Cracking the breast cancer glyco-code through glycan-lectin interactions: targeting immunosuppressive macrophages. Int J Mol Sci. 2021;22(4):1972.
- Liu XY, Jiang W, Ma D, et al. SYTL4 downregulates microtubule stability and confers paclitaxel resistance in triple-negative breast cancer. Theranostics. 2020;10(24):10940–10956.
- Lee KM, Giltnane JM, Balko JM, et al. MYC and MCL1 cooperatively promote chemotherapy-resistant breast cancer stem cells via regulation of mitochondrial oxidative phosphorylation. Cell Metabol. 2017;26(4):633–647.e637.
- Haga Y, Higashisaka K, Yang L, et al. Inhibition of Akt/mTOR pathway overcomes intrinsic resistance to dasatinib in triple-negative breast cancer. Biochem Biophysical Res Commun. 2020;533(4):672–678.
- Liang M, Yao W, Shi B. Circular RNA hsa_circ_0110389 promotes gastric cancer progression through upregulating SORT1 via sponging miR-127-5p and miR-136-5p. Cell Death & Disease. 2021;12(7):639.
- Yu T, Wang Y, Fan Y, et al. CircRNAs in cancer metabolism: a review. Journal of Hematology & Oncology. 2019;12(1):90.
- Wang J, Zhao X, Wang Y, et al. circRNA-002178 act as a ceRNA to promote PDL1/PD1 expression in lung adenocarcinoma. Cell Death Dis. 2020;11(1):32.
- Sang Y, Chen B, Song X, et al. circRNA_0025202 regulates tamoxifen sensitivity and tumor progression via regulating the miR-182-5p/FOXO3a axis in breast cancer. Mol Ther. 2019;27(9):1638–1652.
- Guan Y, Zhang Y, Hao L, et al. CircRNA_102272 promotes cisplatin-resistance in hepatocellular carcinoma by decreasing miR-326 targeting of RUNX2. Cancer Manage Res. 2020;12:12527–12534.
- Ge W, Han C, Wang J, et al. MiR-300 suppresses laryngeal squamous cell carcinoma proliferation and metastasis by targeting ROS1. Am J Transl Res. 2016;8(9):3903–3911.
- Chang Y, Zhou C, Fan L, et al. Upregulation of microRNA‑300 induces the proliferation of liver cancer by downregulating transcription factor FOXO1. Oncol Rep. 2018;40(6):3561–3572.
- Kang Y, Zhang Y, Sun Y, et al. MicroRNA-300 suppresses metastasis of oral squamous cell carcinoma by inhibiting epithelial-to-mesenchymal transition. Oncol Targets Ther. 2018;11:5657–5666.
- Zhang W, Gao Z, Guan M, et al. ASF1B promotes oncogenesis in lung adenocarcinoma and other cancer types. Front Oncol. 2021;11:731547.
- Mbianda J, Bakail M, André C. Optimal anchoring of a foldamer inhibitor of ASF1 histone chaperone through backbone plasticity. Science Advances. 2021;7(12). DOI:10.1126/sciadv.abd9153
- Hu X, Zhu H, Zhang X, et al. Comprehensive analysis of pan-cancer reveals potential of ASF1B as a prognostic and immunological biomarker. Cancer Medicine. 2021;10(19):6897–6916.
- Liu X, Song J, Zhang Y, et al. ASF1B promotes cervical cancer progression through stabilization of CDK9. Cell Death & Disease. 2020;11(8):705.
- Jiangqiao Z, Tao Q, Zhongbao C, et al. Anti-silencing function 1B histone chaperone promotes cell proliferation and migration via activation of the AKT pathway in clear cell renal cell carcinoma. Biochem Biophysical Res Commun. 2019;511(1):165–172.
- Han G, Zhang X, Liu P, et al. Knockdown of anti-silencing function 1B histone chaperone induces cell apoptosis via repressing PI3K/Akt pathway in prostate cancer. Int J Oncol. 2018;53(5):2056–2066.
- Corpet A, De Koning L, Toedling J, et al. Asf1b, the necessary Asf1 isoform for proliferation, is predictive of outcome in breast cancer. EMBO J. 2011;30(3):480–493.
- Ediriweera MK, Tennekoon KH, Samarakoon SR. Role of the PI3K/AKT/mTOR signaling pathway in ovarian cancer: biological and therapeutic significance. Semin Cancer Biol. 2019;59:147–160.
- Tan AC. Targeting the PI3K/Akt/mTOR pathway in non-small cell lung cancer (NSCLC). Thorac Cancer. 2020;11(3):511–518.
- Shorning BY, Dass MS, Smalley MJ. The PI3K-AKT-mTOR pathway and prostate cancer: at the crossroads of AR, MAPK, and WNT signaling. International Journal of Molecular Sciences. 2020;21(12). DOI:10.3390/ijms21124507
- Li D, Ji H, Niu X, et al. Tumor-associated macrophages secrete CC-chemokine ligand 2 and induce tamoxifen resistance by activating PI3K/Akt/mTOR in breast cancer. Cancer Science. 2020;111(1):47–58.
- Zong S, Dai W, Fang W, et al. SIK2 promotes cisplatin resistance induced by aerobic glycolysis in breast cancer cells through PI3K/AKT/mTOR signaling pathway. Biosci Rep. 2020. DOI:10.1042/BSR20201302
- Zhang J, Xie T. Ghrelin inhibits cisplatin-induced MDA-MB-231 breast cancer cell apoptosis via PI3K/Akt/mTOR signaling. Exp Therap Med. 2020;19(3):1633–1640.
- Costa RLB, Han HS, Gradishar WJ. Targeting the PI3K/AKT/mTOR pathway in triple-negative breast cancer: a review. Breast Cancer Res Treat. 2018;169(3):397–406.
- Khan MA, Jain VK, Rizwanullah M, et al. PI3K/AKT/mTOR pathway inhibitors in triple-negative breast cancer: a review on drug discovery and future challenges. Drug Discov Today. 2019;24(11):2181–2191.
- Wu C, Qiu S, Liu P, et al. Rhizoma Amorphophalli inhibits TNBC cell proliferation, migration, invasion and metastasis through the PI3K/Akt/mTOR pathway. J Ethnopharmacol. 2018;211:89–100.