ABSTRACT
Gastric cancer is the most common malignant tumor in the digestive system. However, the detection rate of early gastric cancer is low, resulting in delayed prognosis and poor outcomes. The identification of effective therapeutic targets for gastric cancer is, therefore, of profound significance. Recently, various lncRNAs have been shown to be biomarkers for different cancers. This study investigated the role of long non-coding RNA (lncRNA) TTTY15 in gastric cancer. The expression level of TTTY15, miR-98-5p, and cyclin D2 (CCND2) were evaluated by quantitative reverse transcription polymerase chain reaction (qRT-PCR) and Western blot assay using tumor and non-tumor tissues collected from 30 patients with gastric cancer, gastric cancer cell lines (AGS, SNU-5, and NCI-N87), and the normal gastric epithelial cell line GES-1. The interaction between TTTY15 and miR-98-5p and between miR-98-5p and CCND2 were predicted by bioinformatics and then further verified by dual-luciferase and RNA pull-down analyses. Cell proliferation was evaluated by 3-(4,5-dimethylthiazol-2-yl)-2,5-diphenyl-2 H-tetrazolium bromide (MTT) assay, and apoptosis was measured using flow cytometry and caspase-3 assay. The results indicate that TTTY15 and CCND2 expression increased and miR-98-5p expression decreased in gastric cancer tumor tissues and cell lines. TTTY15 knockdown inhibited gastric cancer cell proliferation but promoted apoptosis by sponging miR-98-5p, which acted as a tumor suppressor gene by reducing the expression of its target gene CCND2 in gastric cancer. In conclusion, lncRNA TTTY15 is a potential oncogene involved in gastric cancer and may be a novel therapeutic target for gastric cancer treatment.
GRAPHICAL ABSTRACT
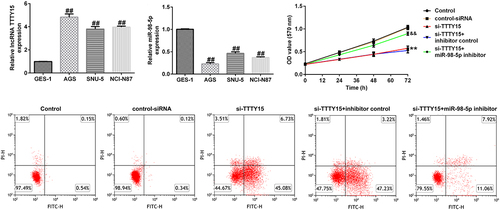
Introduction
Gastric cancer, a common type of malignancy, currently, has the third-highest incidence and fifth-highest mortality rate in the world [Citation1,Citation2]. In China, gastric cancer has become the second most common type of malignant tumor after lung cancer. An increasing number of studies have confirmed that gastric cancer is often caused by H. pylori infection, smoking, consumption of cured foods, and a high-salt diet [Citation3]. At present, surgery combined with radiotherapy, immunotherapy, and targeted therapy is still the most effective way to treat gastric cancer. However, due to atypical early symptoms and the relatively limited awareness of the importance of medical checkups, the detection rate of early gastric cancer is significantly lower in China than in Europe and America [Citation4]. The identification of effective therapeutic targets of gastric cancer is, therefore, of profound importance for its diagnosis and treatment.
Approximately 98% of the human genome is thought to not encode proteins and is hence classified as non-coding genes [Citation5]. Long non-coding RNAs (lncRNAs) are a class of non-coding genes that are more than 200 nucleotides in length [Citation6]. Accumulating evidence has shown that lncRNAs can modulate tumor formation and development in various cancers [Citation7,Citation8]. Moreover, the role and mechanism of lncRNAs in gastric cancer has become a prominent topic in academic research [Citation9,Citation10]. TTTY15 has been reported to be overexpressed and to promote tumor formation in esophageal squamous cell carcinoma [Citation11], colorectal cancer [Citation12], prostate cancer [Citation13], and lung cancer [Citation14]. However, how TTTY15 is involved in gastric cancer remains unclear.
MicroRNAs, a type of small non-coding RNAs, are implicated in regulation of cell differentiation, metabolism, and the cell cycle by reducing target gene expressions at the post-transcriptional level [Citation15,Citation16]. The study of miRNAs in oncology started with chronic granulocytic leukemia (CML), with miR-15a and miR-16-1 being reported to be down-regulated in CML [Citation17,Citation18]. Subsequently, scientists have analyzed and compared miRNA expression profiles in breast cancer [Citation19], lung cancer [Citation20], prostate cancer [Citation21], ovarian cancer [Citation22], and gastric cancer [Citation23]. Thus, miRNAs appear to play crucial regulatory roles in tumorigenesis and development. This study aimed to investigate the mechanism of miR-98-5p involvement in gastric cancer.
In this study, we hypothesized that TTTY15 plays a role in gastric cancer by regulating miR-98-5p/CCND2. Therefore, this study was designed to explore the role and molecular mechanisms of TTTY15 in gastric cancer cells.
Materials and Methods
Patient enrollment
A total of 30 paired tumor tissues and adjacent normal tissues that were more than 2 cm from the tumors [Citation24] were collected from patients who underwent surgical treatment for gastric cancer at the Third People’s Hospital of Hubei Province between January 2019 and March 2021. All excised specimens were stored at −80°C until further use. None of these patients had undergone adjuvant treatment such as chemotherapy or radiotherapy before surgery. All the patients signed the informed consent documents. The present study was approved by the Ethical Review Committee of the Third People’s Hospital of Hubei Province.
Cell culture
AGS, SNU-5, and NCI-N87 gastric cancer cell lines and the gastric epithelial cell line GES-1 were purchased from the Chinese Academy of Sciences (Shanghai, China). All cells were cultured in Roswell Park Memorial Institute (RPMI)-1640 medium containing 10% fetal bovine serum (FBS) in a humidified incubator at 37°C in the presence of 5% CO2.
Cell transfection
2 μM lncRNA TTTY15-siRNA (si-TTTY15), 2 μM control-siRNA, 50 nM miR-98-5p inhibitor, 100 nM miR-98-5p mimic, 50 nM inhibitor control, and 100 nM mimic control were synthesized using RiboBio Co. (Guangzhou, China). The adenovirus plasmid for overexpression of CCND2 (10 μl) and the negative control plasmid (10 μl) were obtained from Santa Cruz Biotechnology (USA). These oligonucleotides were transfected into AGS gastric cancer cells using Lipofectamine 3000 reagent (Thermo Fisher Scientific, Inc., Shanghai, China) according to the manufacturer’s instructions.
Binding site prediction
The online tool starBase was used to predict the potential targets of TTTY15 and the binding sites between TTTY15 and miR-98-5p [Citation25]. Similarly, the bioinformatics tool TargetScan (http://www.targetscan.org/) was used to predict the downstream targets of miR-98-5p and their binding sites [Citation26].
Dual-luciferase reporter assay
The TTTY15 sequences containing the potential miR-98-5p binding sites was amplified by PCR and subcloned into a pGL3 vector (Promega, USA), designated as WT-TTTY15 plasmid. Additionally, MUT-TTTY15, which did not contain the bindings sites, was constructed by GenePharma (Shanghai, China). AGS cells were seeded in 24-well plates and transfected with WT-TTTY15 or MUT-TTTY15 plasmids and miR-98-5p mimic or mimic control using Lipofectamine 2000 (Thermo Fisher Scientific, Inc., Shanghai, China) according to the manufacturer’s instructions. After 24 h of cultivation at 37°C with 5% CO2, the transfected cells were harvested and the luciferase signal was measured using a Promega Kit (Promega, USA) with a GloMax® 2020 Single Tube Luminometer instrument (Promega, USA). The ratio of firefly luciferase activity was normalized to Renilla luciferase [Citation27].
RNA pull-down assay
AGS cells were transfected with lncRNA TTTTY15 biotinylated at the 3′ end, or Oligo probe, and cultured at 37°C with 5% CO2. The transfected cells were then cultured in the presence of streptavidin-coated magnetic beads (Gzscbio, Guangzhou, China). Finally, the biotin-coupled RNA complex was used to determine the abundance of miR-98-5p by qRT-PCR analysis of the bound fractions [Citation28].
Quantitative reverse transcription polymerase chain reaction (qRT-PCR) analysis
Total RNA was extracted from all the samples using Trizol reagent. After measuring the RNA concentration using Nanodrop 2000, 2 μg RNA was reverse transcribed into cDNA using a kit from Takara Co. (RR003A; Japan). The cDNA obtained by reverse transcription was subjected to real-time fluorescence quantification of gene expression on StepOnePlus™ Real-Time PCR System (Applied Biosystems; Shanghai, China) using a Realtime PCR Master Mix kit (QPK-201; Toyobo, Japan). The PCR thermal cycles comprised initial denaturation at 95°C for 5 min; followed by 40 cycles of 15 sec at 95°C, 1 min at 60°C and 30 sec at 72°C; and a final extension for 10 min at 72°C. At the end of the PCR reaction. CT values were derived from the PCR software for relative quantification using the 2−ΔΔCT method [Citation29]. GAPDH/U6 was used as an internal reference. Primer sequences were listed as following:
TTTY15, forward 5′-TGAGGGAGGGAT GTAGCTTT-3′;
reverse 5′-GAAGTCAAGCAGGCAACTGA-3′;
miR-98-5p, forward 5′-TGAGGTAGTAGTTTGTGCTGTT-3′;
reverse 5′-GCGAGCACAGAATTAATACGAC-3′;
GAPDH, forward 5′-CATCATCCCTGCCTCTACTGG-3′;
reverse 5′-GTGGGTGTCGCTGTTGAAGTC-3′;
U6 S 5′-GGAACGATACAGAGAAGATTAGC-3′;
Stem-loop-R 5′-CTCAACTGGTGTCGTGGAGTC-3′;
PCNA, forward 5′- CCTGCTGGGATATTAGCTCCA-3′;
reverse 5′-CAGCGGTAGGTGTCGAAGC-3′;
CCND2, forward 5′-ACCTTCCGCAGTGCTCCTA-3′;
reverse 5′-CCCAGCCAAGAAACGGTCC-3′.
MTT assay
MTT assay was used to evaluate cell proliferation. Briefly, transfected AGS cells were seeded at 1 × 104 cells/well in 96-well plates and cultured at room temperature in a humid atmosphere with 5% CO2. At different time points (24, 48, and 72 h), 20 μL of MTT solution (Procell, Wuhan, China) was added to each well and the plates were incubated for 2 h at 37°C with 5% CO2. Then, 150 μL of dimethyl sulfoxide (Procell, Wuhan, China) was added to dissolve the formazan. Finally, the absorbance was measured at 570 nm using a microplate reader [Citation30].
Flow cytometry analysis
After transfection, 1 × 105 AGS cells/well were cultured in 96-well plates at 37°C with 5% CO2 for 24 h. Then, 5 μL of annexin-V FITC and 10 μL of propidium iodide staining solution (PI) were added to each well and incubated for 15 min at room temperature in the dark. The labeled cells were then placed on ice and subjected to flow cytometry using a BD FACSCalibur (San Diego, CA, USA) [Citation31]. The number of apoptotic cells was determined using ImageJ software.
Caspase-3 activity
After transfection, the AGS cells were seeded into 6-well plates and cultured at 37°C in the presence of 5% CO2. Then, the cells were harvested by centrifugation to collect the supernatant fluid. The caspase-3 activity in the supernatant fluid was assayed with a microplate reader at 405 nm using a caspase-3 activity detection kit (Beyotime Institute of Biotechnology, Shanghai, China) according to the supplier’s instructions [Citation32].
Western blot assay
Protein expressions were determined using Western blot assay [Citation33]. Total protein was extracted using RIPA cell lysate buffer containing a protease inhibitor cocktail. The protein concentration was then measured using the BCA method. The proteins were separated by SDS-PAGE gel electrophoresis and transferred to PVDF membranes. The membranes were then blocked with 5% BSA at room temperature for 50 min to eliminate nonspecific binding of primary and secondary antibodies. Primary antibody (cleaved-Caspase3 antibody, ab32042, 1:1000, Abcam; GAPDH antibody, ab9485, 1:1000, Abcam; PCNA antibody, ab18197, 1:1000, Abcam; CCND2 antibody, ab207604, 1:1000, Abcam;) was then added to the PVDF membrane and incubated overnight at 4°C. The next day, the membranes were probed with the corresponding HRP-labeled secondary antibody (ab7090, 1:1000, Abcam) by incubation at room temperature for 2 h. ECL chromogenic solution A and B were mixed, added dropwise to the position of the target band on the membrane, and photographed using ImageJ software.
Data analysis
All statistical analyses were performed using SPSS 20 statistical software (SPSS, Chicago, IL, USA) and GraphPad Prism 5 (GraphPad Software, La Jolla, CA, USA). Data are presented as means ± SD. Student’s t-test or one-way ANOVA analysis followed by Tukey’s test were used for the statistical analysis. A p-value of less than 0.05 (P < 0.05) was considered statistically significant.
Results
lncRNA TTTY15 sponged miR-98-5p in AGS cells
First, the starBase online tool was utilized to predict the potential targets of lncRNA TTTY15. This tool identified the potential binding sites between the 3′-UTR of TTTY15 and miR-98-5p (). Furthermore, using a dual-luciferase reporter assay, it was found that the luciferase activity was greatly decreased in the miR-98-5p mimic and WT-TTTY15 co-transfection groups compared with that in mimic control group (). Additionally, the RNA pull-down assay also demonstrated that the expression of miR-98-5p was greatly enhanced in AGS cells treated with lncRNA TTTTY15 probes compared with those treated with NC probes, (). These results suggest that lncRNA TTTY15 can sponge miR-98-5p in AGS cells.
Figure 1. lncRNA TTTY15 sponges miR-98-5p in gastric cancer.
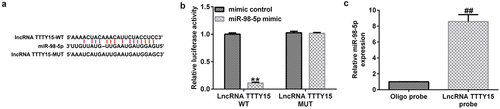
Enhanced lncRNA TTTY15 and decreased miR-98-5p expression in gastric cancer patients and cell lines
To further investigate the mechanisms underlying lncRNA TTTY15 and miR-98-5p involvement in gastric cancer, we used qRT-PCR analysis to distinguish aberrantly expressed lncRNA TTTY15 and miR-98-5p. lncRNA TTTY15 expression was greatly increased and miR-98-5p expression was down-regulated in tumor tissues compared with that in non-tumor adjacent tissues (). Similarly, lncRNA TTTY15 levels were increased and miR-98-5p levels were reduced in the gastric cancer cell lines AGS, SNU-5, and NCI-N87 compared with that in the normal gastric epithelial cell line GES-1, (). The changes in lncRNA TTTY15 and miR-98-5p levels were most obvious in AGS cells, which were hence chosen for the subsequent experiments.
Figure 2. Ectopic expressions of lncRNA TTTY5 and miR-98-5p in gastric cancer tissues and cells.
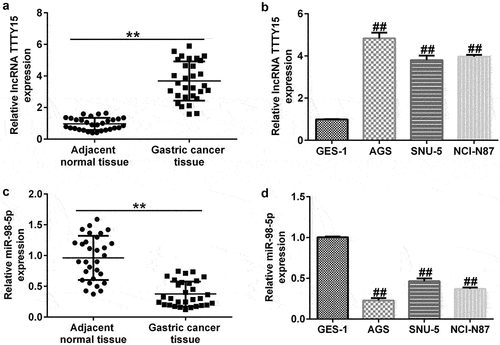
lncRNA TTTY15 silencing inhibited AGS cell proliferation by sponging miR-98-5p
The effects of lncRNA TTTY15 and its target miR-98-5p on AGS cell proliferation were investigated. As shown in , after transfection with si-TTTY15, lncRNA TTTY15 expression was clearly suppressed in AGS cell compared to that in control siRNA group, suggesting that the interference was successful. Similarly, miR-98-5p inhibitor transfection also down-regulated the level of miR-98-5p in AGS cells (). Loss- and gain-of-function experiments () then showed that si-TTTY15 treatment could enhance miR-98-5p expression in AGS cells compared to that in control siRNA group. However, this enhancement could be partially offset by co-transfection with miR-98-5p inhibitor. Comprehensively, the above results suggest that TTTY15 reduces miR-98-5p expression in gastric cancer cells.
Figure 3. lncRNA TTTY5 suppressed miR-98-5p expression in AGS cells to regulate AGS cell proliferation.
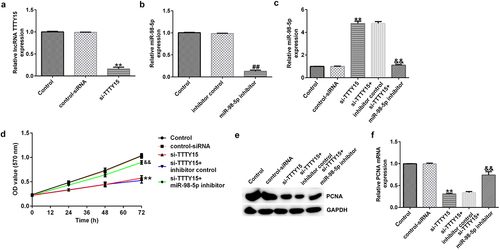
Furthermore, the MTT assay illustrated that lncRNA TTTY15 silencing greatly reduced AGS cell proliferation compared with that in negative control siRNA (). Additionally, the expression of proliferation-related protein PCNA was also decreased in AGS cells after si-TTTY15 transfection (). Conversely, the inhibitory effects of si-TTTY15 on AGS cell proliferation could be offset by the miR-98-5p inhibitor (). Collectively, these results indicate that lncRNA TTTY15 knockdown reduced AGS cell proliferation by targeting miR-98-5p.
lncRNA TTTY15 knockdown triggered AGS cell apoptosis by sponging miR-98-5p
The impact of lncRNA TTTY15/miR-98-5p axis on the regulation of AGS cell apoptosis was also investigated. As shown in , lncRNA TTTY15 knockdown significantly promoted AGS cell apoptosis and enhanced caspase-3 activity. Additionally, the pro-apoptosis protein cleaved-caspase-3 was also increased after knockdown of TTTY15 expression in AGS cells (). Conversely, the miR-98-5p inhibitor could reverse the promotive effects of lncRNA TTTY15 down-regulation on AGS cell apoptosis (). Collectively, these results indicate that lncRNA TTTY15 down-regulation promotes AGS cell apoptosis by inhibition of miR-98-5p expression.
Figure 4. lncRNA TTTY5 induced AGS cell apoptosis by sponging miR-98-5p.
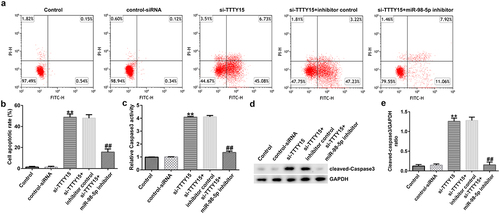
CCND2 was negatively modulated by miR-98-5p in gastric cancer
As predicted by TargetScan, CCND2 contains potential binding sites for miR-98-5p (). The results of the dual-luciferase reporter assay further confirmed that the miR-98-5p mimic could decrease luciferase activity in the CCND2-WT-transfected groups, while there was no significant change in the CCND2-MUT transfected groups ().
Figure 5. Identification of CCND2 as a downstream target of miR-98-5p in gastric cancer.
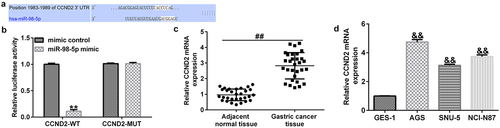
Moreover, the qRT-PCR analysis revealed that CCND2 expression was clearly up-regulated in gastric cancer tumor tissues compared to that in the paracancerous tissues (). Similarly, CCND2 level was enhanced in gastric cancer cell lines (AGS, SNU-5, and NCI-N87) compared with that in the GES-1 normal gastric epithelial cells. This implies that CCND2 may be an oncogene involved in gastric cancer ().
miR-98-5p negatively regulated CCND2 in AGS cells
In order to confirm the regulatory relationship between CCND2 and miR-98-5p, we transiently transfected AGS cells with miR-98-5p mimic and/or CCNDS-plasmid. The results shown in indicate that miR-98-5p was increased in the miR-98-5p mimic group compared to that in the mimic control group. Meanwhile, CCND2 expression was up-regulated in AGS cells transfected with CCND2-plasmid compared to that in control plasmid group (). Finally, the co-transfection experiments revealed that the miR-98-5p mimic could reduce the level of CCND2 mRNA and protein in AGS cells and that the inhibition of CCND2 expression induced by the miR-98-5p mimic was partially offset by CCND2-plasmid transfection (). Comprehensively, the above results illustrate that CCND2 was negatively regulated by miR-98-5p in AGS cells, thus suggesting that CCND2 functions as a downstream target for miR-98-5p.
Up-regulation of miR-98-5p induced AGS cell apoptosis and reduced proliferation by targeting CCND2
To explore the effects of miR-98-5p on AGS cells, AGS cells were transfected with mimic control, miR-98-5p mimic, miR-98-5p + control plasmid, or miR-98-5p mimic + CCND2-plasmid and cultured for 48 h. Then, a series of functional experiments were conducted to verify that the miR-98-5p/CCND2 axis is involved in the regulation of gastric cancer progression. As shown in , up-regulation of miR-98-5p inhibited AGS cell proliferation and reduced the level of the pro-proliferation protein PCNA in AGS cells. Moreover, the results in show that overexpression of miR-98-5p resulted in AGS cell apoptosis as well as an increase in caspase-3 activity and the level of the pro-apoptosis protein cleaved caspase-3. In contrast, the enhancing effect of the miR-98-5p mimic on AGS cell apoptosis was partially reversed by co-transfection with CCND2-plasmid (). Altogether, the above-mentioned results imply that miR-98-5p participates in the regulation of gastric cancer development by targeting CCND2.
Figure 7. miR-98-5p overexpression suppressed AGS cell proliferation and promoted apoptosis by targeting CCND2.
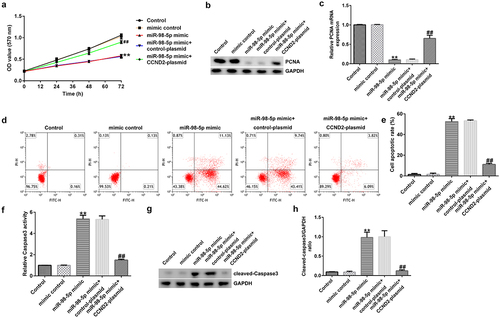
Discussion
The lncRNA TTTY15 coding sequence is located on the Y chromosome (chrY:12,537,650 ~ 12,860,839) and comprises 323,190 bases. As a newly discovered lncRNA, TTTY15 has been shown to play a crucial role in cardiovascular diseases. For instance, Zheng et al. [Citation34], Chen et al. [Citation35], Huang et al. [Citation36], Hao et al. [Citation37], and Zhang et al. [Citation38] have reported that TTTY15 silencing can sponge miR-186-5p, miR-374a-5p, miR-455-5p, miR-766-5p, and let-7i-5p to mitigate hypoxia-induced vascular endothelial cell injury. Moreover, dysregulation of TTTY15 has been observed in various cancers. In colorectal cancer, TTTY15 was clearly increased in cancerous tissues and cell lines, promoting colorectal cancer cell growth and metastasis by sponging miR-29-3p [Citation12]. In the study by Xiao et al. [Citation13], the TTTY15 coding sequence was found to be located on the Y chromosome and its expression was up-regulated in prostate cancer tissues, thus functioning as an oncogene. Wang et al. [Citation11] showed that TTTY15 levels were up-regulated in esophageal squamous cell carcinoma (ESCC) samples and cells, indicating that it may function as an oncogene in ESCC. On the other hand, another study revealed a tumor-suppressive role of TTTY15 in non-small cell lung cancer [Citation14]. In the present study, we investigated the role of TTTY15 in gastric cancer. First, we found that there was pronounced up-regulation of TTTY15 in gastric cancer tissues and cell lines. However, only 30 pairs of clinical samples were included in this study, and a larger sample size may make our results more convincing, which is a research limitation of this study. Then, using siRNA technology, we knocked down TTTY15 by transfection of si-TTTY15 into gastric cancer cells. Functional experiments showed that this silencing of TTTY15 inhibited gastric cancer cell proliferation and promoted apoptosis. In this study, only flow cytometry was used to analyze cell apoptosis, and the absence of detection of apoptosis using the TUNEL assay is another limitation of the study. Collectively, for the first time, we revealed an oncogenic role of TTTY15 in gastric cancer.
The miR-98-5p coding sequence has been reported to be located on chrX:53,556,223–53,556,341 and comprises 119 bases. Recently, miR-98-5p has been found to be involved in various aspects of tumor formation and development. For instance, miR-98-5p has been shown to act as a tumor suppressor in non-small cell lung cancer [Citation39], gastric cancer [Citation40], glioma cancer [Citation41], oral squamous cell carcinoma [Citation42], ovarian cancer [Citation43], and papillary thyroid carcinoma [Citation44]. Xu et al. [Citation45] observed down-regulation of miR-98-5p in gastric cancer. Meanwhile, another study showed that miR-98-5p could reduce gastric cancer cell stemness and paclitaxel chemosensitivity by targeting BCAT1 [Citation40]. Consistent with previous studies, our study first verified the binding relationship between TTTY15 and miR-98-5p by a dual-luciferase reporter assay and RNA pull-down analysis. Furthermore, rescue experiments demonstrated that the inhibitory effects of TTTY15 silencing on gastric cancer growth could be reversed by miR-98-5p inhibitor. Additionally, overexpression of miR-98-5p by transfection with miR-98-5p mimic reduced gastric cancer cell proliferation and promoted apoptosis, implying that TTTY15 could sponge miR-98-5p to participate in the regulation of gastric cancer development.
CCND2 was predicted by the TargetScan bioinformatics tool to have potential binding sites for miR-98-5p, and it was validated as a downstream target of miR-98-5p using a dual-luciferase assay. As a component of the cell cycle machinery, CCND2 (like Cyclin D1, Cyclin D3, and Cyclin E) is a cell cycle regulatory protein [Citation46]. Recent studies have reported that aberrant expression of CCND2 can lead to abnormal cell proliferation [Citation47–49]. Previous studies have shown that CCND2 is aberrantly expressed in a variety of tumor tissues, such as lung cancer [Citation50], breast cancer [Citation51], and gastric cancer [Citation52]. Consistent with previous research, our study found that miR-98-5p could promote gastric cancer cell apoptosis in addition to reducing cell proliferation, which could be partly offset by overexpression of CCND2. Thus, our research revealed that miR-98-5p can suppress gastric cancer development by targeting CCND2.
Conclusion
This study showed that knockdown of lncRNA TTTY15 can down-regulate CCND2 expression by sponging miR-98-5p, thereby reducing gastric cancer progression. Thus, the lncRNA TTTY15/miR-98-5p/CCND2 axis is a potential therapeutic target for gastric cancer, and our results provide new insights for clinical treatment approaches.
Availability of data and materials
The datasets used and/or analyzed during the current study are available from the corresponding author on reasonable request.
Research highlights
lncRNA TTTY15 sponged miR-98-5p in AGS cells.
lncRNA TTTY15 knockdown inhibited proliferation and triggered AGS cell apoptosis by sponging miR-98-5p.
Up-regulation of miR-98-5p induced AGS cell apoptosis and reduced proliferation by targeting CCND2.
Disclosure statement
No potential conflict of interest was reported by the author(s).
Additional information
Funding
References
- Rong GH, Zhang YX, Ma YC, et al. The Clinical and molecular characterization of gastric cancer patients in Qinghai-Tibetan plateau. Front Oncol. 2020;10:1033.
- Zhao QF, Cao L, Guan LL, et al. Immunotherapy for gastric cancer: dilemmas and prospect. Brief Funct Genomics. 2019;18(2):107–112.
- Correa P, Piazuelo MB, and Camargo MC. The future of gastric cancer prevention. Gastric Cancer 2004;73:9–16.
- Johnston FM, Beckman M. Updates on management of gastric cancer. Curr Oncol Rep. 2019;21(8):67.
- Lu SH, Zhang J, Lian XL, et al. A hidden human proteome encoded by ‘non-coding’ genes. Nucleic Acids Res. 2019;47(15):8111–8125.
- Wang JC, Su ZL, Lu SN, et al. LncRNA HOXA-AS2 and its molecular mechanisms in human cancer. Clin Chim Acta. 2018;485:229–233.
- Wang JY, Lu AQ, Chen LJ. LncRNAs in ovarian cancer. Clin Chim Acta. 2019;490:17–27.
- Logotheti S, Marquardt S, Gupta SK, et al. LncRNA-SLC16A1-AS1 induces metabolic reprogramming during bladder cancer progression as target and co-activator of E2F1. Theranostics. 2020;10(21):9620–9643.
- Liu XJ, Li SL, Li JS, et al. Long non-coding RNA ZEB1-AS1 is associated with poor prognosis in gastric cancer and promotes cancer cell metastasis. Eur Rev Med Pharmacol Sci. 2018;22(9):2624–2630.
- Zhuang M, Gao W, Xu J, et al. The long non-coding RNA H19-derived miR-675 modulates human gastric cancer cell proliferation by targeting tumor suppressor RUNX1. Biochem Biophys Res Commun. 2014;448:315–322.
- Wang W, Yang JB. Long noncoding RNA TTTY15 promotes growth and metastasis of esophageal squamous cell carcinoma by sponging microRNA-337-3p to upregulate the expression of JAK2. Anticancer Drugs. 2020;31(10):1038–1045.
- Zheng X-YX-Y, Cao MZ, Ba Y, et al. LncRNA testis-specific transcript, Y-linked 15 (TTTY15) promotes proliferation, migration and invasion of colorectal cancer cells via regulating miR-29a-3p/DVL3 axis. Cancer Biomark. 2021;31(1):1–11.
- Xiao GA, Ao JJ, Kong DP, et al. The long moncoding RNA TTTY15, which is located on the Y chromosome, promotes prostate cancer progression by sponging let-7. Eur Urol. 2019;76(3):315–326.
- Lai IL, Chang YS, Chan WL, et al. Male-specific long noncoding RNA TTTY15 inhibits non-small cell lung cancer proliferation and metastasis via TBX4. Int J Mol Sci. 2019;20(14):3473.
- Vienberg S, Geiger J, and Madsen S, et al. MicroRNAs in metabolism. Acta Physiol (Oxf). 2017;219(2):346–361.
- Wang F, Liang R, Tandon N, et al. H19X-encoded miR-424(322)/-503 cluster: emerging roles in cell differentiation, proliferation, plasticity and metabolism. Cell Mol Life Sci. 2019;76(5):903–920.
- Calin GA, Ferracin M, Cimmino A, et al. A MicroRNA signature associated with prognosis and progression in chronic lymphocytic leukemia. N Engl J Med. 2005;353(17):1793–1801.
- Cimmino A, Calin GA, Fabbri M, et al. miR-15 and miR-16 induce apoptosis by targeting BCL2. Proc Natl Acad Sci U S A. 2005;102(39):13944–13949.
- Sabit H, Cevik E, Tombuloglu H, et al. Triple negative breast cancer in the era of miRNA. Crit Rev Oncol Hematol. 2021;157:103196.
- Du XH, Zhang JT, Wang JP, et al. Role of miRNA in lung cancer-potential biomarkers and therapies. Curr Pharm Des. 2018;23(39):5997–6010.
- Fabris L, Ceder Y, and Chinnaiyan AM, et al. The potential of microRNAs as prostate cancer biomarkers. Eur Urol 2016;70(2) :312–322.
- Ghafouri-Fard S, Shoorei H, Taher M. miRNA profile in ovarian cancer. Exp Mol Pathol. 2020;113:104381.
- Zeng L, Liao Q, Zeng X, et al. Noncoding RNAs and hyperthermic intraperitoneal chemotherapy in advanced gastric cancer. Bioengineered. 2022;13(2):2623–2638.
- Gao M, Li W, Wang H, et al. The distinct expression patterns of claudin-10, −14, −17 and E-cadherin between adjacent non-neoplastic tissues and gastric cancer tissues. Diagn Pathol. 2013;8(1):205.
- Li JH, Liu S, Zhou H, et al. starBase v2.0: decoding miRNA-ceRNA, miRNA-ncRNA and protein-RNA interaction networks from large-scale CLIP-Seq data. Nucleic Acids Res. 2014;42(D1):D92–D97.
- Riffo-Campos ÁL, Riquelme I, Brebi-Mieville P. Tools for Sequence-Based miRNA Target Prediction: what to Choose? Int J Mol Sci. 2016;17:1987.
- Yu L, Qu H, Yu Y, et al. LncRNA-PCAT1 targeting miR-145-5p promotes TLR4-associated osteogenic differentiation of adipose-derived stem cells. J Cell Mol Med. 2018;22(12):6134–6147.
- Chen B, Wang H, Lv C, et al. Long non-coding RNA H19 protects against intracerebral hemorrhage injuries via regulating microRNA-106b-5p/acyl-CoA synthetase long chain family member 4 axis. Bioengineered. 2021;12(1):4004–4015.
- Livak KJ, Schmittgen TD. Analysis of relative gene expression data using real-time quantitative PCR and the 2(-Delta Delta C(T)) method. Methods. 2001;25(4):402–408.
- Kumar P, Nagarajan A, and Uchil PD. Analysis of Cell Viability by the MTT Assay. Cold Spring Harb Protoc. 2018;2018(6): 469–471.
- Telford WG. Multiparametric Analysis of Apoptosis by Flow Cytometry. Methods Mol Biol. 2018;1678:167–202.
- Ren H, Li Z, Tang Z, et al. Long noncoding MAGI2-AS3 promotes colorectal cancer progression through regulating miR-3163/TMEM106B axis. J Cell Physiol. 2020;235(5):4824–4833.
- Kim B. Western blot Techniques. Methods Mol Biol. 2017;1606:133–139.
- Zheng J, Zhuo YY, Zhang C, et al. LncRNA TTTY15 regulates hypoxia-induced vascular endothelial cell injury via targeting miR-186-5p in cardiovascular disease. Eur Rev Med Pharmacol Sci. 2020;24(6):3293–3301.
- Chen YQ, Yang X, Xu W, et al. Knockdown of lncRNA TTTY15 alleviates myocardial ischemia–reperfusion injury through the miR-374a-5p/FOXO1 axis. IUBMB Life. 2021;73(1):273–285.
- Huang SQ, Ao WQ, Guo ZF, et al. Suppression of long noncoding RNA TTTY15 attenuates hypoxia-induced cardiomyocytes injury by targeting miR-455-5p. Gene. 2019;701:1–8.
- Hao CG, Chen SB. Knockdown of lncRNA TTTY15 alleviates ischemia/reperfusion-induced inflammation and apoptosis of PC12 cells by targeting miR-766-5p. Exp Ther Med. 2021;21(5):511.
- Zhang H, Zou XF, Liu F. Silencing TTTY15 mitigates hypoxia-induced mitochondrial energy metabolism dysfunction and cardiomyocytes apoptosis via TTTY15/let-7i-5p and TLR3/NF-κB pathways. Cell Signal. 2020;76:109779.
- Liang F, Yu QH, Chu Y, et al. MicroRNA-98-5p inhibits proliferation and metastasis in non-small cell lung cancer by targeting TGFBR1. Int J Oncol. 2019;54(1):128–138.
- Zhan PP, Shu X, Chen M, et al. miR-98-5p inhibits gastric cancer cell stemness and chemoresistance by targeting branched-chain aminotransferases 1. Life Sci. 2021;276:119405.
- Li YB, Wang XR, Zhao ZH, et al. LncRNA NEAT1 promotes glioma cancer progression via regulation of miR-98-5p/BZW1. Biosci Rep. 2021;41(7):BSR20200767.
- Niu XY, Yang B, Liu F, et al. LncRNA HOXA11-AS promotes OSCC progression by sponging miR-98-5p to upregulate YBX2 expression. Biomed Pharmacother. 2020;121:109623.
- Dong LN, Cao XJ, Luo Y, et al. A Positive Feedback Loop of lncRNA DSCR8/miR-98-5p/STAT3/HIF-1α Plays a Role in the Progression of Ovarian Cancer. Front Oncol. 2020;10:1713.
- Qiu K, Xie QJ, Jiang S, et al. miR-98-5p promotes apoptosis and inhibits migration and cell growth in papillary thyroid carcinoma through Bax/Caspase-3 by HMGA2. J Clin Lab Anal. 2020;34(2):e23044.
- Xu QF, Peng HP, Lu XR, et al. Oleanolic acid regulates the Treg/Th17 imbalance in gastric cancer by targeting IL-6 with miR-98-5p. Cytokine. 2021;148:155656.
- Hille S, Dierck F, Kühl C, et al. Dyrk1a regulates the cardiomyocyte cell cycle via D-cyclin-dependent Rb/E2f-signalling. Cardiovasc Res. 2016;110(3):381–394.
- Wang J, Shu HZ, Guo SG. MiR-646 suppresses proliferation and metastasis of non-small cell lung cancer by repressing FGF2 and CCND2. Cancer Med. 2020;9(12):4360–4370.
- Park SY, Lee CJ, Choi JH, et al. The JAK2/STAT3/CCND2 Axis promotes colorectal Cancer stem cell persistence and radioresistance. J Exp Clin Cancer Res. 2020;38(1):399.
- Zhao CC, Liu J, Wu HZ, et al. Aberrant methylation-mediated downregulation of lncRNA CCND2 AS1 promotes cell proliferation in cervical cancer. J Biol Res (Thessalon). 2020;27(1):11.
- Jin M, Ren J, Luo M, et al. Long non-coding RNA JPX correlates with poor prognosis and tumor progression in non-small-cell lung cancer by interacting with miR-145-5p and CCND2. Carcinogenesis. 2020;41(5):634–645.
- Zhong H, Yang J, Zhang B, et al. LncRNA GACAT3 predicts poor prognosis and promotes cell proliferation in breast cancer through regulation of miR-497/CCND2. Cancer Biomark. 2018;22(4):787–797.
- Zhuo SS, Sun MM, Bai RM, et al. Long intergenic non-coding RNA 00473 promotes proliferation and migration of gastric cancer via the miR-16-5p/CCND2 axis and by regulating AQP3. Cell Death Dis. 2021;12(5):496.