ABSTRACT
Melatonin (MEL) is an effective therapeutic choice for thyroid cancer treatment. In this study, we aimed to explored the potential effect of MEL upon the drug sensitivity of cancer cells and the according underlying mechanisms. Thyroid cancer mice were established as a control group and a MEL group to observe the in vivo effect of MEL. Tumor size and weight in nude mice were detected to evaluate the effect of MEL on tumor growth. Immunohistochemistry assay (IHC) and Western blot were performed to analyze the expression of PTEN protein in tumor cells or tumor cells. After 32 days of cancer cell implantation, MEL was found to significantly repress tumor growth in nude mice approximately by half. Moreover, MEL also suppressed tumor cell proliferation, while apparently activating the apoptosis of tumor cells. In addition, hydrogen sulfide (H2S) production was obviously elevated by MEL treatment. Mechanistically, the expression of phosphatase and tensin homolog (PTEN) was remarkably activated by MEL treatment in tumor tissues of implanted TPC-1 and BCPaP cells in nude mice. Meanwhile, MEL inhibited the expression of miR-21 and miR-30e and promoted the expression of lncRNA-cancer susceptibility candidate 7 (CASC7). Both miR-21 and miR-30e could suppress PTEN expression, while miR-21 could also inhibit the expression of lncRNA-CASC7. In conclusion, the results demonstrated that the MEL administration could downregulate the expression of miR-21 and miR-30e, which resulted in increased expression of PTEN, a pro-apoptotic tumor suppressor, to promote the apoptosis of thyroid cancer cells.
Graphical Abstract
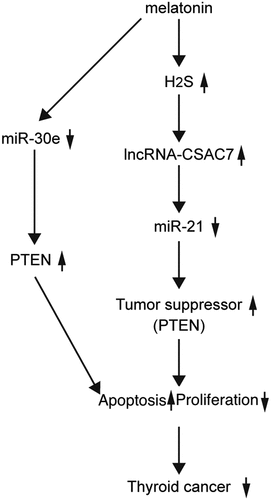
Introduction
Thyroid cancer is one of the most frequently diagnosed endocrine cancer, with a rapidly growing yearly occurrence rate worldwide [Citation1,Citation2]. In 2015, about 100,000 new thyroid cancer cases were reported in China [Citation3]. Differentiated thyroid cancer (DTC), which includes both follicular thyroid cancer and papillary thyroid cancer, accounts for most of thyroid cancer cases and typically reacts well to surgical procedure in conjunction with radioiodine (131-I) treatment [Citation4]. On the other hand, inadequately differentiated or undifferentiated thyroid cancer such as anaplastic thyroid cancer (ATC) makes up virtually 50% of all thyroid cancer associated mortality [Citation5].
LncRNAs are specified as a type of transcripts with no protein coding ability that contain greater than 200 nucleotides. LncRNAs were first recognized as transcriptional noises [Citation6]. Nevertheless, current evidence shows that lncRNAs are associated with various features including epigenetic control of transcription [Citation7]. In contrast, miRNAs are a type of tiny and single-stranded RNAs associated with the control of different intracellular activities, including cell growth, survival, proliferation, inflammatory reaction, as well as tumorigenesis [Citation8]. LncRNAs are typically thought as noncoding RNAs (ncRNAs) which manage gene expression. For example, lncRNA-CASC7 was shown to inhibit the expansion of colon cancer cells by suppressing miR-21 expression [Citation9]. Moreover, bioinformatic evaluation found a miR-21 binding site on CASC7 as well as on the 3-untranslated region (3’-UTR) of PTEN. As a result, in individuals with asthma resistant to corticosteroid treatment, CASC7 may target miR-21 to upregulate the expression of PTEN while raising the sensitivity level of airway smooth muscle cells (ASMCs) to glucocorticoid via hindering the signaling of PI3K/AKT.
PTEN is recognized as one of the most essential targets of miR-21 in cancer advancement, and an inverted relationship was discovered between the degrees of miR-21 expression and the intensity of PTEN signals [Citation10,Citation11]. The upregulation of miR-21 and the downregulation of PTEN are validated to lead to higher incidence of non-small-cell lung carcinoma (NSCLC). Therefore, these results show that miR-21 is featured as a tumorigenic element by negatively controlling the expression of PTEN in human NSCLC. The degree of miR-21 expression is also negatively associated with the degrees of PTEN protein as well as high degrees of miR-21 expression and a much shorter DFS. Certain proof has actually showed that PTEN is a gene suppressing tumor growth. Actually, homozygous PTEN deletions were seen in a number of tumors [Citation12–14]. In addition, PTEN has the ability to reduce cell proliferation, while the increased PTEN expression prevents the transition from the S phase in cell lines derived from thyroid tumors [Citation15,Citation16].
It has been reported that MEL increased the production of H2S, which may upregulate the expression of lncRNA-CSAC7 to downregulate the expression of miR-21 [Citation17,Citation18]. Meanwhile, MEL also downregulates the expression of miR-30e [Citation19,Citation20]. In this study, we aimed to explore the role of MEL in the regulation of the drug sensitivity of thyroid cancer cells. As PTEN is a shared target gene of miR-21 and miR-30e, we hypothesized that MEL may suppress the proliferation and promote the apoptosis of thyroid cancer cells via regulating the signaling pathways of H2S/CSAC7/microRNA-21/PTEN and microRNA-30e/PTEN. To test the above hypothesis, we incubated thyroid cancer cells with MEL and then implanted those cells into thyroid cancer mice. This study for the first time purposed H2S as a potential factor to participate in the signaling pathway of CSAC7, and investigated two different miRNA-involved signaling pathway to study the mechanism of MEL’s therapeutic effect upon thyroid cancer.
Materials and methods
Cell culture and transfection
To establish a cellular model of thyroid cancer, TPC-1 (Sigma Aldrich, St Louis, MO; cat number: SCC147) and BCPaP cells (DSMZ, Braunschweig, Germany; cat number: ACC273) were kept in a DMEM medium (Gibco, Thermo Fisher Scientific, Waltham, MA) supplemented with 10% (v/v) of FBS (Sigma Aldrich, St Louis, MO) as well as Pen (5000 units/mL)/Strep (5000 µg/mL) (Gibco, Thermo Fisher Scientific, Waltham, MA). All cells were cultured at 37°C in 5% carbon dioxide and saturated humidity. When the cells reached exponential growth, they were divided into 2 group, i.e., 1. Control group (Untreated cells), and 2. 5 mM MEL group (cells treated with 5 mM of melatonin). Melatonin concentration was chosen according to previous published articles and was prepared using DMSO solution. The cells were treated under respective conditions for 48 hrs. At 48 hrs after treatment, the cells were harvested for later analyses.
Animal model establishment
BALB/C female nude mice (about 4 to 6 weeks old) were bought from Charles River (Beijing) Laboratory (Beijing, China) and adapted to the environment in the animal facility for 7 days. Then, 1 × 106 TPC-1 and BCPaP cells were diluted in 100 μl of chilled PBS (PH = 7.4) and injected subcutaneously to the right forelimb of each mouse to establish a tumor model of thyroid cancer. After the successful establishment of the thyroid cancer model, the mice were arbitrarily divided into two groups, i.e., 1. Control group (thyroid cancer mice not treated with MEL) and 2. MEL (thyroid cancer mice treated with a daily dose of 25 mg/kg of MEL for 32 days). At the same time, all mice in both groups were irradiated with a radiation dose of 2 Gy two times weekly. During the entire treatment of 25 days, the growth dimension of tumor in each mouse was detected twice a week by using a Vernier caliper, so that the tumor volume could be approximated in accordance with the formula: tumor volume = 0.5 × W × L, in which L represented the largest tumor diameter while W is the perpendicular diameter of the tumor. Throughout the radiation treatment, the mouse was placed under a plumbum mold and the tumor was exposed to the radiation ray. After 32 days of therapy in conjunction with or without MEL, the tumor tissues were taken out from dissected mice and made into paraffin embedded section for subsequent analyses. Institutional ethical committee of Zhumadian Central Hospital has approved the protocol of this study (Approval ID: ZMDCH20170731B).
RNA isolation and real-time PCR
Collected tissue and cell samples were treated in a Trizol reagent (Invitrogen, Carlsbad, CA) to isolate the total RNA content, which was quantified and 1 μg of the total RNA from each sample was reversely transcribed into cDNA transcripts by making use of universal primers as well as a SuperScript III First-Strand Synthesis Assay Kit (Invitrogen, Carlsbad, CA) in accordance with the experimental protocols provided by the assay manufacturer. In the next step, real-time PCR was carried out on a QuantStudio 3 Real-Time PCR Instrument (Applied Biosystems, Foster City, CA) as well as a Power SYBR Green Master Real-Time PCR Assay kit (Applied Biosystems, Foster City, CA) in accordance with the experimental protocols provided by the assay manufacturer. All experimental data was evaluated by using the QuantStudio Software equipped on the QuantStudio 3 Real-Time PCR Instrument (Applied Biosystems, Foster City, CA). Finally, the expression of miR-30e (Forward primer: 5’-GTAAACATCCTTGACTGG-3’; Reverse primer: 5’-GAACATGTCTGCGTATCTC-3’), lncRNA-CASC7 (Forward primer: 5’-ATCAACGTCAAGCTGGGAGG-3’; Reverse primer: 5’-CTTGTCCCCCGCTCGTTC-3’), miR-21 (Forward primer: 5’-GCTTATCAGACTGATGTTG-3’; Reverse primer: 5’-GAACATGTCTGCGTATCTC-3’), and PTEN mRNA (Forward primer: 5’-TGAGTTCCCTCAGCCGTTACCT-3’; Reverse primer: 5’-GAGGTTTCCTCTGGTCCTGGTA-3’) in each sample was calculated by utilizing the ΔΔCt approach.
Vector construction, mutagenesis, and luciferase assay
The results of our preliminary sequence analysis showed potential binding sites of miR-21 and miR-30e in the gene sequences of lncRNA-CASC7 and PTEN, respectively. Thus, a luciferase assay was performed to explore the regulatory relationship between lncRNA-CSAC7/miR-21, miR-21/PTEN, as well as miR-30e/PTEN. At first, the sequences of CASC7 and PTEN containing respective miR-21/miR-30e binding sites were cloned and inserted into pcDNA 3.1 luciferase vectors (Promega, Madison, WI) to generate wild-type plasmids of CASC7 and PTEN, respectively. At the same time, site-directed mutagenesis (nucleotide sequence changes indicated in ) by utilization of a QuikChange II Site-directed mutagenesis kit (Agilent Technologies, Inc., Santa Clara, CA) was carried out to mutate the miR-21/miR-30e binding sites in the sequences of CASC7 and PTEN to generate mutant-type plasmids of CASC7 and PTEN, respectively. In the next step, either mutant type or wild type of CASC7 or PTEN plasmids were co-transfected into TPC-1 and BCPaP cells (6 × 103 cells per well) in conjunction with miR-21/miR-30e mimics or a scramble control by using Lipofectamine 3000 transfection reagent (Invitrogen, Carlsbad, CA) in accordance with the experimental protocols provided by the assay manufacturer. At 48 h after transfection, the cells were harvested and the luciferase activity of transfected cells was measured by using a Bright Glo luciferase assay (Promega, Madison, WI) in accordance with the experimental protocols provided by the assay manufacturer.
Figure 7. Luciferase assays showed that miR-21 and miR-30e suppressed the expression of PTEN and miR-21 while inhibiting the expression of lncRNA-CASC7 (* P value < 0.05, vs. control group).
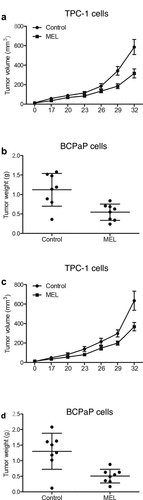
Cell proliferation assay
When the cells undergoing various treatments grew to 70% confluence, they were rinsed two times by PBS, trypsinized by using 0.25% trypsin, and seeded into 96-well tissue culture plates at 6 × 103 cells per well in 200 μl of cell suspension. Then, the proliferation status of the cells was measured by using an MTT assay kit (Sigma Aldrich, St Louis, MO) in accordance with the experimental protocols provided by the assay manufacturer.
Western blot
The total protein content in collected tissue (5 mg) and cell samples was homogenized with lysis buffer using electric homogenizer and was subsequently isolated by utilizing a protease inhibitor-supplemented radioimmunoprecipitation assay (RIPA) buffer (Thermo Fisher Scientific, Waltham, MA) for 2 h at 4°C. After that, the protein content was quantified by utilizing a BCA assay kit (Thermo Fisher Scientific) in accordance with the experimental protocols provided by the assay manufacturer. Then, the protein (50 μg/lane) in each lysate sample was resolved by using 10% SDS-PAGE, blotted onto a polyvinylidene difluoride (PVDF) membrane, and blocked for 1 h at ambient temperature in Tris-buffered saline containing 5% nonfat milk and Tween-20 (50 mmol/L of Tris-HCl, 150 mmol/L of NaCl, and 0.1% of Tween-20). In the next step, the membrane was incubated at 4°C overnight with anti-PTEN antibody (dilution: 1:1000; cat no: EP2138Y; OriGene, Rockville, MD), washed with Tris-buffered saline supplemented with 0.1% Tween 20 (TBST), and then incubated for 1 h at ambient temperature with appropriate horseradish peroxidase-conjugated secondary antibodies (dilution: 1: 2000; cat no: 32,460; Thermo Fisher Scientific, Waltham, MA) before the relative protein expression of PTEN in each sample was detected by using an enhanced chemiluminescence (ECL) reagent kit (Pierce™ Fast Western blot Kit, Thermo Fisher Scientific, Waltham, MA) in accordance with the experimental protocols provided by the assay manufacturer.
Apoptosis analysis
The proliferation of cells (6 × 103 cells per well) was measured by making use of a Guava Nexin Annexin V staining assay kit (Millipore, New York City, NY) in accordance with the experimental protocols provided by the assay manufacturer on a BD FACS Caliber flow cytometer (BD, San Jose, CA).
IHC assay
Immunofluorescence staining of PTEN protein in collected tumor tissue samples was carried out to measure the relative expression of PTEN in each sample. In brief, tissue sections (10 μm in thickness) were treated for 1 hour with PBS added with 5% BSA as well as 0.05% of Triton-X100. EDTA (Invitrogen, Carlsbad, CA) was utilized to retrieve antigen and endogenous peroxidase activity was blocked in a 0.5% hydrogen peroxide methanol solution. Then, the sections were incubated with primary anti-PTEN antibody (dilution: 1:1000; cat no: SC-7974; Santa Cruz Biotechnology, Santa Cruz, CA) for 2 h and suitable Alexa-594 labeled secondary antibodies (dilution:1:1000; cat no: SC-8432; Santa Cruz Biotechnology, Santa Cruz, CA) for 30 min consecutively. The slides were counter-stained with DAPI for 5 min and washed with PBS. Neutral resin was used as the mounting medium and the slides were observed under a Olympus fluorescence microscope (excitation: 450 nm; emission: 590 nm; Olympus Corporation, Tokyo, Japan) to evaluate the positive expression of PTEN in each sample.
ELISA
Concentrations of H2S in various samples were assayed by using a commercially available ELISA kit (NeoBioscience, Beijing, China) in accordance with the experimental protocols provided by the assay manufacturer.
Statistical analysis
SPSS version 20.0 was utilized for statistical analyses of all experimental data. Each experiment was repeated in triplicate and all data is presented as mean ± standard deviation. The normality test of experimental data was carried out by using an independent-sample T-test. The level of statistical significance was set to P = 0.05. All inter-group comparisons were carried out using Student’s t tests.
Results
MEL treatment suppressed the tumor cell growth in nude mice
In this study, we aimed to explore the role of MEL in the regulation of the drug sensitivity of thyroid cancer cells. As PTEN is a shared target gene of miR-21 and miR-30e, we hypothesized that MEL may suppress the proliferation and promote the apoptosis of thyroid cancer cells via regulating the signaling pathways of H2S/CSAC7/microRNA-21/PTEN and microRNA-30e/PTEN. To test the above hypothesis, we incubated thyroid cancer cells with MEL and then implanted those cells into thyroid cancer mice.
TPC-1 cells were implanted into nude mice and the growth of tumor in mice undergoing MEL treatment was monitored for 1 month. The size of tumor tissues in nude mice undergoing MEL treatment was significantly smaller than that in control mice (). Moreover, the weight of tumor tissues in mice administrated with MEL was remarkably decreased in comparison to that in the untreated control mice (). Furthermore, we implanted BCPaP cells into nude mice and observed similar results ().
Figure 1. MEL treatment suppressed tumor cell growth in nude mice.
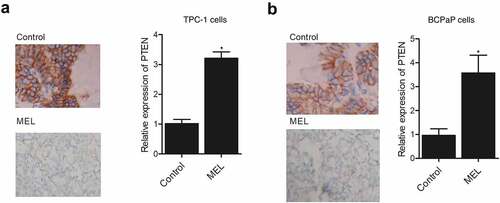
MEL treatment enhanced the expression of PTEN in the tumor tissues in nude mice
IHC assay was performed on the tumor tissues harvested from mice implanted with TPC-1 and BCPaP cells. The expression of PTEN was compared between the two groups. The expression of PTEN in the TPC-1 () and BCPaP () cells collected from MEL-treated mice was obviously elevated when compared with that in the control. These results demonstrated that the expression of PTEN was activated by MEL treatment.
Figure 2. IHC analysis showed that the expression of PTEN protein in tumor tissues in nude mice was activated by MEL treatment (* P value < 0.05, vs. control group).
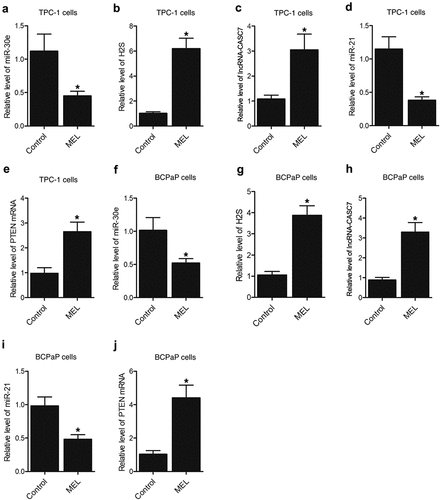
MEL treatment suppressed the expression of miR-30e and miR-21 while enhancing the lncRNA-CASC7 and PTEN mRNA expression along with H2S production in the tumor tissues in nude mice
Furthermore, the expression of miR-30e, miR-21, lncRNA-CASC7, and PTEN mRNA as well as the H2S production was examined in the tumor tissues of nude mice. The expression of miR-30e () and miR-21 () was notably repressed by MEL treatment when compared with that in the control. In contrast, the expression of lncRNA-CASC7 () and PTEN mRNA () was remarkably elevated by MEL treatment. The production of H2S was apparently elevated in the tumor tissues from MEL-treated nude mice (). Meanwhile, the expression of miR-30e, miR-21, lncRNA-CASC7, and PTEN mRNA as well as the H2S production in the tumor tissues of nude mice implanted with BCPaP cells showed a similar trend ().
Figure 3. MEL treatment suppressed the expression of miR-30e and miR-21 while enhancing the lncRNA-CASC7 and PTEN mRNA expression as well as H2S production in the tumor tissues of nude mice (* P value < 0.05, vs. control group).
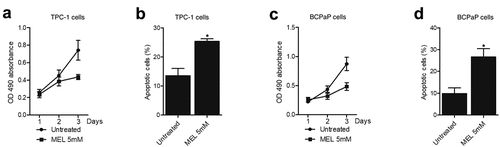
MEL inhibited the proliferation and activated the apoptosis of TPC-1 and BCPaP cells
MTT assay and flow cytometry were performed to examine the proliferation and apoptosis of TPC-1 and BCPaP cells treated with 5 mM MEL. The proliferation of TPC-1 () and BCPaP () cells was effectively repressed by MEL treatment. Meanwhile, the apoptosis of TPC-1 () and BCPaP () cells was obviously activated by MEL treatment. These data suggested that MEL treatment could effectively inhibit the proliferation and promote the apoptosis of tumor cells.
Figure 4. MEL inhibited the proliferation and activated the apoptosis of TPC-1 and BCPaP cells (* P value < 0.05, vs. untreated cells).
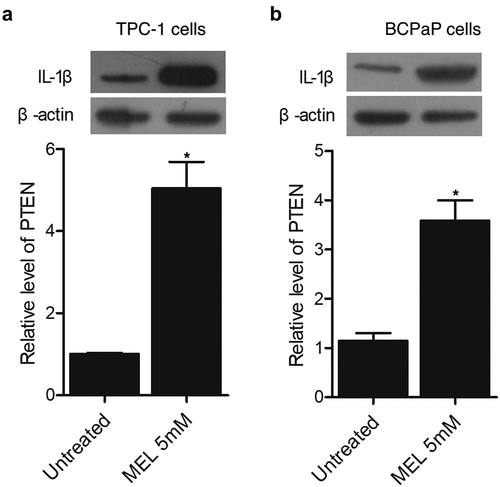
MEL increased the expression of PTEN protein in TPC-1 and BCPaP cells
Western blot was carried out to evaluate the expression of PTEN protein in TPC-1 and BCPaP cells treated with 5 mM MEL. The expression of PTEN protein was evidently increased in TPC-1 () and BCPaP () cells undergoing MEL treatment.
MEL treatment suppressed the expression of miR-30e and miR-21 while enhancing the lncRNA-CASC7 and PTEN mRNA expression as well as H2S production in TPC-1 and BCPaP cells
Furthermore, the expression of miR-30e, miR-21, lncRNA-CASC7, and PTEN mRNA as well as H2S production was also evaluated in TPC-1 and BCPaP cells undergoing 5 mM MEL treatment. Significant inhibition of miR-30e () and miR-21 () expression was observed in MEL-treated TPC-1 and BCPaP cells. In contrast, the expression of lncRNA-CASC7 () and PTEN mRNA () was activated in TPC-1 and BCPaP cells treated with MEL. The production of H2S () was also facilitated by MEL treatment in TPC-1 and BCPaP cells.
Figure 6. MEL treatment suppressed the expression of miR-30e and miR-21 while enhancing the lncRNA-CASC7 and PTEN mRNA expression as well as H2S production in TPC-1 and BCPaP cells (* P value < 0.05, vs. untreated cells).
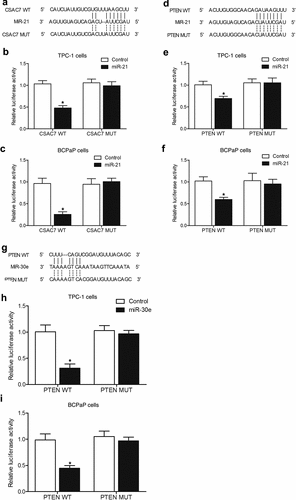
MiR-21 and miR-30e suppressed the expression of PTEN while miR-21 inhibited the expression of lncRNA-CASC7 through 3’ UTR binding
Sequence analysis showed potential binding of miR-21 to lncRNA-CASC7 () and PTEN (), and luciferase assay was performed to explore the regulatory relationship between miR-21 and its potential targets. Luciferase vectors containing wild-type and mutant lncRNA-CASC7 and PTEN were established and co-transfected into TPC-1 and BCPaP cells with miR-21. The luciferase activities of wild-type lncRNA-CASC7 () and PTEN () were significantly inhibited in TPC-1 and BCPaP cells. We also found that PTEN was a potential target of miR-30e (). Wild type and mutant PTEN was co-transfected into TPC-1 and BCPaP cells with miR-30e. The luciferase activity of wild-type PTEN vectors was remarkably inhibited by miR-30e (), indicating that miR-30e suppressed the expression of PTEN.
In summary, our results indicated that MEL treatment activated H2S production and lncRNA-CASC7 expression in tumor cells, subsequently inhibiting the expression of miR-21. Meanwhile, MEL treatment inhibited the expression of miR-30e, while the down-regulation of miR-21 and miR-30e resulted in the up-regulated expression of PTEN, which in turn inhibited the growth and activated the apoptosis of tumor cells to alleviate the severity of cancer.
Discussion
Melatonin is generated by pineal gland throughout the evening under the regulation of the body clock, the suprachiasmatic nuclei located in hypothalamus. Melatonin is likewise manufactured by immune competent cells to contribute in the monitoring as well as recuperation from infection [Citation21–23]. The interaction in between body defense and timing is a fine tuned and controlled procedure that entails melatonin-mediated constraint of leukocyte movement from the blood flow to tissues [Citation24,Citation25]. Melatonin also has complex functions including the role to scavenge free radicals. It was actually been revealed that melatonin treatment can reprogram H2S pathways to avoid high blood pressure. In this study, we implanted TPC-1 and BCPaP cells in nude mice, and detected the size and weight of tumor. We found that tumor growth in MEL-treated nude mice was significantly inhibited when compared with that in the control mice. In addition, we performed qPCR, IHC and Western blot to analyze the expression of PTEN mRNA and protein in tumor tissues derived from implanted TPC-1 and BCPaP cells in nude mice that were treated with MEL. The expression of PTEN was significantly increased by MEL treatment in TPC-1 and BCPaP cells or mouse tumor tissues. It is acknowledged that BCPaP is differed from TPC-1 by the presence of a BRAFV600E mutation. However, even though we utilized these two different cells lines in our study, the presence of BRAFV600E mutation seemed to exhibit no significant influence upon our findings.
H2S was actually thought as the 3rd signaling molecule in the gaseous phase that exerts essential effects on the development of cancer [Citation26–28]. Thyroid cancers are known for high rates of global occurrence [Citation29]. A research shows that diallyl sulfide can reduce the proliferation of cells while causing their apoptosis through the mitochondrial signaling [Citation30]. It was actually revealed that the expression of CASC7 was subdued while the expression of miR-21 as well as AKT activity was enhanced in ASMC collected from people with acute bronchial asthma. CASC7 enhanced PTEN expression by directly hindering miR-21 expression. The over-expression of CASC7 subdued the PI3K/AKT signaling while enhancing the impacts of dexamethasone on cytokine secretion through targeting miR-21.
It was actually discovered that miR-21-5p expression was low in TPC-1 cells to prevent their expansion as well as intrusion into normal tissues. Concordant with the above result, Dai et al. showed that the expression of miR-21 was dramatically reduced in people with papillary thyroid cancer [Citation31]. On the other hand, miR-21 was upregulated in TPC-1 [Citation32,Citation33]. Zhang et al. confirmed that miR-21 substantially improved cell proliferation as well as metastasis via targeting programmed apoptosis 4. The deregulation of miRNAs is crucial in human PTC [Citation33–35]. MiR‑21 transfection right into PTC led to a raised rate of cell expansion as well as a lowered rate of apoptosis [Citation36]. MiR‑21 works by interacting with PTEN, which in turn reduces the malignancy of cancer by de-phosphorylating threonine protein kinase (Akt)/RAC‑α serine [Citation37–39]. In another research, the downregulated miR‑21 expression influenced the Akt/PTEN signaling to inhibit the proliferation and induce G1 cycle arrest of leukemic cells in myelodysplastic syndromes [Citation40]. In this study, we performed qPCR to evaluate the expression of miR-30e, miR-21 and lncRNA-CASC7 in tumor tissues from nude mice and in TPC-1 and BCPaP cells treated with MEL. The expression of miR-30e and miR-21 was remarkably inhibited by MEL treatment, whereas the expression of lncRNA-CASC7 was notably elevated by MEL treatment. Furthermore, we performed luciferase assay to explore the regulatory relationships between miR-21 and lncRNA-CASC7/PTEN as well as between miR-30e and PTEN. The luciferase activity of lncRNA-CASC7 was significantly inhibited by miR-21. The luciferase activity of PTEN was also significantly repressed by miR-21 and miR-30e.
PTEN works as one of miR-21 targets by de-phosphorylating its target genes [Citation41]. PTEN mutations were actually observed in ~5% of breast cancer patients. PTEN inactivation can cause the loss of its ability as a lipid phosphatase while promoting the buildup of phosphate-dylinositol-3,4,5-trisphosphate (PIP3), leading to Akt phosphorylation [Citation42]. It was additionally shown that the rescue of expression of PTEN in thyroid tumor subdues tumor development. Particularly, it seems that the induced expression of PTEN in thyroid tumor does not cause the apoptosis of tumor cells. Instead, PTEN obstructs tumor development via cell cycle arrest and preventing the entry of tumor cells into the S phase. Additionally, past research offered proof that p27kip1, a kinase whose function is dependent on cyclin, is involved in the role of PTEN in the progression of cell cycles. As a matter of fact, PTEN expression can up-regulate the expression of p27kip1 in thyroid cancer, while the antisense against p27kip1 eliminates the effects of PTEN on cell cycle arrest (FB-1). In this study, we performed MTT and flow cytometry assays to examine the proliferation and apoptosis of tumor cells in nude mice and in culture. The proliferation of the cells was obviously repressed by MEL treatment, while the apoptosis of the cells was apparently activated by MEL treatment.
Conclusion
The findings of this study demonstrated that melatonin increased the production of H2S, which subsequently upregulated the expression of lncRNA-CSAC7 to downregulate the expression of miR-21. Meanwhile, melatonin also suppressed the expression of miR-30e, and the downregulation of both miR-21 and miR-30e resulted in increased expression of PTEN, a pro-apoptotic tumor suppressor. Therefore, we validated through the findings of this study that melatonin could promote the apoptosis of thyroid cancer cells. However, the study is limited due to the lack of clinical observations. To further validate the potential of melatonin in the treatment of thyroid cancer in patients, clinical study, as well as animal study with larger sample sized, is expected.
Author Contributions Statement
HJ and WS wrote the main manuscript text and HJ, WS, XBL and WHX prepared -8. All authors reviewed the manuscript.
Statement
The data that support the findings of this study are available from the corresponding author upon reasonable request.
Supplemental Material
Download Zip (1.3 MB)Disclosure statement
No potential conflict of interest was reported by the author(s).
Supplementary material
Supplemental data for this article can be accessed here
Additional information
Funding
References
- Siegel RL, Miller KD, Fuchs HE, et al. Cancer Statistics, 2021. CA Cancer J Clin. 2021Jan;71(1):7–33. Epub 2021 Jan 12. Erratum in: CA Cancer J Clin. 2021 Jul;71(4):359.
- Geng X, Sun Y, Fu J, et al. MicroRNA-17-5p inhibits thyroid cancer progression by suppressing Early growth response 2 (EGR2). Bioengineered. 2021 Dec;12(1):2713–2722.
- Chen W, Zheng R, Baade PD, et al. Cancer statistics in China, 2015. CA Cancer J Clin. 2016;66(2):115–132.
- Czarniecka A, Zeman M, Wozniak G, et al. Therapeutic Strategy in Low-Risk Papillary Thyroid Carcinoma – long-Term Results of the First Single-Center Prospective Non-Randomized Trial Between 2011 and 2015. Front Endocrinol (Lausanne). 2021;12:718833.
- Nagaiah G, Hossain A, Mooney CJ, et al. Anaplastic thyroid cancer: a review of epidemiology, pathogenesis, and treatment. J Oncol. 2011;2011:542358.
- Brosius J. Waste not, want not – transcript excess in multicellular eukaryotes. Trends Genet. 2005;21(5):287–288.
- Mercer TR, Dinger ME, Mattick JS. Long non-coding RNAs: insights into functions. Nat Rev Genet. 2009;10(3):155–159.
- Paladini L, Fabris L, Bottai G, et al. Targeting microRNAs as key modulators of tumor immune response. J Exp Clin Cancer Res. 2016;35(1):103.
- Wang G, Duan P, Liu F, et al. Long non-coding RNA CASC7 suppresses malignant behaviors of breast cancer by regulating miR-21-5p/FASLG axis. Bioengineered. 2021;12(2):11555–11566.
- Bao L, Yan Y, Xu C, et al. MicroRNA-21 suppresses PTEN and hSulf-1 expression and promotes hepatocellular carcinoma progression through AKT/ERK pathways. Cancer Lett. 2013;337(2):226–236.
- Yang S-M, Huang C, Li X-F, et al. Li J: miR-21 confers cisplatin resistance in gastric cancer cells by regulating PTEN. Toxicology. 2013;306:162–168.
- Li J, Yen C, Liaw D, et al. PTEN, a Putative Protein Tyrosine Phosphatase Gene Mutated in Human Brain, Breast, and Prostate Cancer. Science. 1997;275(5308):1943–1947.
- Steck PA, Pershouse MA, Jasser SA, et al. Tavtigian SV: identification of a candidate tumour suppressor gene, MMAC1, at chromosome 10q23.3 that is mutated in multiple advanced cancers. Nat Genet. 1997;15(4):356–362.
- Rasheed BK, Stenzel TT, McLendon RE, et al. PTEN gene mutations are seen in high-grade but not in low-grade gliomas. Cancer Res. 1997;57(19):4187–4190.
- Furnari FB, Lin H, Huang H-JS, et al. Growth suppression of glioma cells by PTEN requires a functional phosphatase catalytic domain. Proc Natl Acad Sci U S A. 1997;94(23):12479–12484.
- Cheney IW, Neuteboom ST, Vaillancourt MT, et al. Adenovirus-mediated gene transfer of MMAC1/PTEN to glioblastoma cells inhibits S phase entry by the recruitment of p27Kip1 into cyclin E/CDK2 complexes. Cancer Res. 1999;59(10):2318–2323.
- Li Y-T, Tian X-T, Wu M-L, et al. Resveratrol Suppresses the Growth and Enhances Retinoic Acid Sensitivity of Anaplastic Thyroid Cancer Cells. Int J Mol Sci. 2018;19(4). 10.3390/ijms19041030
- Liu J-H, Li C, Zhang C-H, et al. LncRNA-CASC7 enhances corticosteroid sensitivity via inhibiting the PI3K/AKT signaling pathway by targeting miR-21 in severe asthma. Pulmonology. 2019;26(1):18–26.
- Fernandes JCR, Aoki JI, Maia Acuna S, et al. Melatonin and Leishmania amazonensis Infection Altered miR-294, miR-30e, and miR-302d Impacting on Tnf, Mcp-1, and Nos2 Expression. Front Cell Infect Microbiol. 2019;9:60.
- Wu X, Ji H, Wang Y, et al. Melatonin Alleviates Radiation-Induced Lung Injury via Regulation of miR-30e/NLRP3 Axis. Oxid Med Cell Longev. 2019;2019:4087298.
- Markus RP, Fernandes PA, Kinker GS, et al. Immune-pineal axis - acute inflammatory responses coordinate melatonin synthesis by pinealocytes and phagocytes. Br J Pharmacol. 2018;175(16):3239–3250.
- Markus RP, Ferreira ZS, Fernandes PACM, et al. The immune-pineal axis: a shuttle between endocrine and paracrine melatonin sources. Neuroimmunomodulation. 2007;14(3–4):126–133.
- Carrillo-Vico A, Guerrero JM, Lardone PJ, et al. A review of the multiple actions of melatonin on the immune system. Endocrine. 2005;27(2):189–200.
- Lotufo CMC, Lopes C, Dubocovich ML, et al. Melatonin and N-acetylserotonin inhibit leukocyte rolling and adhesion to rat microcirculation. Eur J Pharmacol. 2001;430(2–3):351–357.
- Ren D-L, Li Y-J, Hu -B-B, et al. Melatonin regulates the rhythmic migration of neutrophils in live zebrafish. J Pineal Res. 2015;58(4):452–460.
- Szabo C, Coletta C, Chao C, et al. Tumor-derived hydrogen sulfide, produced by cystathionine-β-synthase, stimulates bioenergetics, cell proliferation, and angiogenesis in colon cancer. Proc Natl Acad Sci U S A. 2013;110(30):12474–12479.
- De Cicco P, Panza E, Ercolano G, et al. ATB-346, a novel hydrogen sulfide-releasing anti-inflammatory drug, induces apoptosis of human melanoma cells and inhibits melanoma development in vivo. Pharmacol Res. 2016;114:67–73.
- Wu D, Li M, Tian W, et al. Hydrogen sulfide acts as a double-edged sword in human hepatocellular carcinoma cells through EGFR/ERK/MMP-2 and PTEN/AKT signaling pathways. Sci Rep. 2017;7(1):5134.
- Xing M, Haugen BR, Schlumberger M. Progress in molecular-based management of differentiated thyroid cancer. Lancet. 2013;381(9871):1058–1069.
- Shin HA, Cha YY, Park MS, et al. Diallyl sulfide induces growth inhibition and apoptosis of anaplastic thyroid cancer cells by mitochondrial signaling pathway. Oral Oncol. 2010;46(4):e15–18.
- Dai L, Wang Y, Chen L, et al. MiR-221, a potential prognostic biomarker for recurrence in papillary thyroid cancer. World J Surg Oncol. 2017;15(1):11.
- Guo Z, Hardin H, Montemayor-Garcia C, et al. In Situ Hybridization Analysis of miR-146b-5p and miR-21 in Thyroid Nodules: diagnostic Implications. Endocrine Pathology. 2015;26(2):157–163.
- Suresh R, Sethi S, Ali S, et al. Differential Expression of MicroRNAs in Papillary Thyroid Carcinoma and Their Role in Racial Disparity. J Cancer Sci Ther. 2015;7(5):145–154.
- Liu J, Ni S. Association between genetic polymorphisms in the promoters of let-7 and risk of cervical squamous cell carcinoma. Gene. 2018;642:256–260.
- Perdas E, Stawski R, Nowak D, et al. The Role of miRNA in Papillary Thyroid Cancer in the Context of miRNA Let-7 Family. Int J Mol Sci. 2016;17(6):909.
- Zhang J, Yang Y, Liu Y, et al. MicroRNA-21 regulates biological behaviors in papillary thyroid carcinoma by targeting programmed cell death 4. J Surg Res. 2014;189(1):68–74.
- Meng F, Henson R, Wehbe–Janek H, et al. MicroRNA-21 regulates expression of the PTEN tumor suppressor gene in human hepatocellular cancer. Gastroenterology. 2007;133(2):647–658.
- Qi L, Bart J, Tan LP, et al. Expression of miR-21 and its targets (PTEN, PDCD4, TM1) in flat epithelial atypia of the breast in relation to ductal carcinoma in situ and invasive carcinoma. BMC Cancer. 2009;9(1):163.
- Zhu S, Wu H, Wu F, et al. MicroRNA-21 targets tumor suppressor genes in invasion and metastasis. Cell Res. 2008;18(3):350–359.
- Li G, Song Y, Li G, et al. Downregulation of microRNA‑21 expression inhibits proliferation, and induces G1 arrest and apoptosis via the PTEN/AKT pathway in SKM‑1 cells. Mol Med Rep. 2018Sep;18(3):2771–2779. Epub 2018 Jul 5. PMID: 30015844; PMCID: PMC6102657.
- Yu G, Li H, Wang J, et al. Xu H: miRNA-34a suppresses cell proliferation and metastasis by targeting CD44 in human renal carcinoma cells. J Urol. 2014;192(4):1229–1237.
- Pencheva N, Tavazoie SF. Control of metastatic progression by microRNA regulatory networks. Nat Cell Biol. 2013;15(6):546–554.