ABSTRACT
Endothelial dysfunction is an important mechanism involved in myocardial ischemia-reperfusion (I/R) injury. We aimed to explore the effects of Oxycodone on myocardial I/R injury in vivo and in vitro to reveal its mechanisms related to Sigma-1 Receptor (SIGMAR1). A rat model of I/R-induced myocardial injury was developed. The ischemic area and myocardial histopathological changes after oxycodone addition were evaluated by TTC staining and H&E staining. LDH, CK-MB and cTnI levels were used to assess myocardial function. Then, the endothelial integrity was reflected by the expressions of ZO-1, Claudin-1 and Occludin. Afterward, ELISA, RT-qPCR, western blot and immunofluorescence assays were adopted for the detection of inflammation-related genes. SIGMAR1 expression in myocardial tissues induced by I/R and cardiac microvascular endothelial cells (CMECs) under hypoxic/reoxygenation (H/R) was determined using RT-qPCR and western blotting. Subsequently, after SIGMAR1 silencing or BD1047 addition (a SIGMAR1 antagonist), cell apoptosis and endothelial integrity were analyzed in the presence of Oxycodone in H/R-stimulated CMECs. Results indicated that Oxycodone decreased the ischemic area and improved myocardial function in myocardial I/R injury rat. Oxycodone improved myocardial histopathological injury and elevated endothelial integrity, evidenced by upregulated ZO-1, Claudin-1 and Occludin expressions. Moreover, inflammatory response was alleviated after Oxycodone administration. Molecular docking suggested that SIGMAR1 could directly bind to Oxycodone. Oxycodone elevated SIGMAR1 expression and SIGMAR1 deletion or BD1047 addition attenuated the impacts of Oxycodone on apoptosis and endothelial integrity of CMECs induced by H/R. Collectively, Oxycodone alleviates myocardial I/R injury in vivo and in vitro by binding to SIGMAR1.
Graphical Abstract
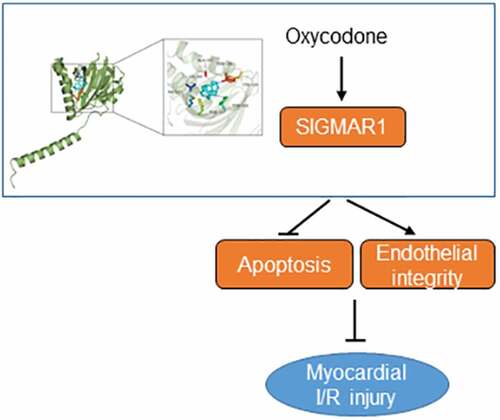
Introduction
Myocardial ischemia/reperfusion (I/R) injury is a cardiovascular disease accompanied by high morbidity and mortality globally from acute myocardial infarction and coronary artery disease [Citation1]. Timely restoration of blood flow (reperfusion) to the ischemic myocardium is the standard treatment for patients with myocardial ischemia [Citation2]. However, the recovery of perfusion and concomitant reoxygenation exacerbates the dysfunction of myocardium, accelerates cardiomyocyte death and results in structural damage. Despite paramount clinical interests, there is no effective therapy for preventing and treating I/R-induced cardiac injury [Citation3]. Thus, management of I/R injury is important for improving the outcome of these patients.
An increasing number of researches have validated that microvascular disorder is an important mechanism of myocardial I/R injury, and the mechanisms involved include inflammation, calcium overload and pH changes [Citation4,Citation5]. Cardiac microvascular endothelial cells (CMECs) located in the capillaries are the basic components of myocardial microcirculation, which can secrete cytokines related to cardiac growth, contractility and heart rhythm [Citation6]. CMECs participate in up to one-third of the cardiac microvascular system and function to match myocardial perfusion and oxygen consumption [Citation7]. As one of the major cell types damaged immediately after myocardial I/R injury, CMECs dysfunction is considered to be an important pathophysiological event of myocardial I/R injury. Therefore, maintaining microvascular endothelial integrity is a new potential therapeutic approach to improving myocardial I/R injury.
Oxycodone (6-deoxy-7,8-dehydro-14-hydroxy-3-O-methyl-6-oxomorphine) is a multiple-opioid receptor agonist first synthesized in 1917 that commonly serves as a sedative in clinic practice. Accumulating studies have confirmed that oxycodone possesses excellent anti-inflammatory and anti-apoptotic properties. For instance, oxycodone attenuates the acute lung injury-induced rat lung histological changes and reduces pulmonary microvascular permeability via inhibiting inflammation and apoptosis [Citation8]. By downregulating nuclear factor-κB (NF-κB) expression and decreasing tumor necrosis factor-α (TNF-α), interleukin-6 (IL-6) and IL-1β levels in hippocampal astrocytes, Oxycodone protects against lipopolysaccharide-induced neuroinflammatory damage [Citation9]. Oxycodone inhibits oxygen glucose deprivation/recovery-induced hippocampal neuronal apoptosis [Citation10]. In addition, studies have also shown the cardioprotective effects of oxycodone, that is, it can inhibit myocardial I/R injury by hampering apoptosis of cardiomyocytes [Citation11,Citation12]. However, whether oxycodone can reduce the damage of CMECs is still unknown. The swisstarget database (http://www.swisstargetprediction.ch/) showed that oxycodone could target Sigma-1 Receptor (SIGMAR1), a member of a relatively novel receptor group that is a unique binding site commonly expressed in the central nervous system and other peripheral tissues [Citation13]. Emerging evidence supports the notion that activation of SIGMAR1 relieves myocardial apoptosis in rats suffering from myocardial I/R injury [Citation14]. Importantly, compelling evidence indicates that chronic SIGMAR1 activation can improve the ventricular remodeling after myocardial infarction in rats and decrease the susceptibility to ventricular arrhythmia [Citation15], suggesting that SIGMAR1 might relieve myocardial I/R injury. By employing the human protein expression database (https://www.proteinatlas.org/), we observed that SIGMAR1 is widely expressed in myocardial tissues and expressed in CMECs. For this reason, we speculated that Oxycodone could target SIGMAR1 to impart protective effects against CMECs damage during myocardial I/R.
In the present study, the myocardial I/R injury rat model was established to investigate the effects of Oxycodone on the inflammation and microvascular damage. The further experiments were performed to explore the regulation of Oxycodone on SIGMAR1 in CMECs under hypoxic/reoxygenation (H/R) condition. Our findings may open novel avenues for future therapies of myocardial I/R damage.
Materials and methods
Animal
A total of 24 male Sprague-Dawley (SD) rats weighing 200–300 g (6–8 weeks age) provided by the Model Animal Research Center of Nanjing University (Nanjing, China) were housed in air-conditioned rooms at 23 ± 2°C with a 12-h light/dark cycle. Rats were given a standard diet and allowed to drink water freely. Before the experiment, the rats were adaptively reared for 1 week. Animal experiment got the permission from the Animal Research Ethics Committee of Nanjing First Hospital, Nanjing Medical University.
Induction of myocardial I/R injury
Myocardial I/R surgery was conducted according to previous description [Citation16]. To simulate myocardial I/R injury, rats were anesthetized intraperitoneally with 40 mg/kg sodium pentobarbital. After the pericardium being dissected open, the left anterior descending was exposed and occluded by ligation with a 5–0 silk suture. The slipknot was released following 30 min of ischemia and rats received 2 h of reperfusion [Citation17]. The ischemia was confirmed by observing the color change of the ischemic area of myocardial tissue, and reperfusion was achieved by loosening the ligature. Animals were sacrificed through intraperitoneal injection of 200 mg/kg sodium pentobarbital after receiving 2 h of reperfusion. The blood samples and heart tissues were harvested with the aim of performing follow-up experiments.
Grouping and drug administration
The SD rats were randomly distributed into groups of control, sham, I/R, I/R + Oxycodone (n = 6 in each group). Rats in the Oxycodone treated group were subjected to an intravenous injection of 0.5 mg/kg Oxycodone for 30 min prior to surgery. Rats in the other groups underwent intravenous injection with normal saline at the same time.
Evaluation of myocardial infarct area
The infarct area of the myocardium was tested by 2,3,5-triphenyltetrazolium chloride (TTC). Following the rinse with normal saline for three times, cardiac tissues were sliced into 2-mm-thick transverse sections for five slices. Then, 1% TTC solution (cat. no. T8877; Sigma-Aldrich) was used to stain cardiac tissues at 37°C for 15 min. The infarct size was identified with the application of Image J software (Version 1.52 r; National Institutes of Health, Bethesda, MA, USA).
Determination of release of lactate dehydrogenase (LDH), creatine kinase- myocardial band (CK-MB) and cardiac troponin I (cTnI) into serum
To reflect the myocardial cellular damage, blood samples were centrifuged at 3000 rpm for 20 min at 4°C to obtain serum. The levels of LDH (cat. no. A020-1-2), CK-MB (cat. no. H197-1-1) and cTnI (cat. no. H149-2) in serum were evaluated by the corresponding commercial kits supplied by Nanjing Jiancheng Bioengineering Institute (Nanjing, China).
Hematoxylin and Eosin (H&E) staining
Following the rinse with normal saline for three times, heart tissues were immersed in 4% paraformaldehyde for 24 h and embedded with conventional paraffin wax. 5-μm-thick sections were obtained. Deparaffinization of the sections was performed and the sections were then stained with hematoxylin and eosin, prior to dehydration in a graded ethanol series and xylene. The sections were imaged with the aid of a light microscope (Olympus Corporation).
Measurement of inflammatory cytokines
The secretion of TNF-α (cat. no. F16960), IL-1β (cat. no. F15810) and IL-6 (cat. no. F15870) in serum were determined with the enzyme-linked immunosorbent assay (ELISA) kits provided by Shanghai Xitang Biotechnology (Shanghai, China) as per the standard procedures of the supplier. The optical density (OD) value was evaluated at 450 nm with the adoption of a microplate reader (Molecular Devices, USA).
Cell culture
Rat CMECs were obtained from iCell Bioscience (Shanghai, China) and cultured in the medium filling with a mixture of Dulbecco’s Modified Eagle’s Medium (DMEM; HyClone, Logan, UT) and 10% fetal bovine serum (FBS; HyClone, Logan, UT). Cells were kept in culture under 5% CO2 at 37°C.
Hypoxic/reoxygenation (H/R) induction and treatment
To construct the H/R models, CMECs were placed in an anaerobic chamber with the anoxic atmosphere of 94% N2, 5% CO2 and 1% O2 for 30 min to simulate the hypoxic condition. After establishing hypoxia, cells were transferred to a normal cell incubator with a mixture of 95% air and 5% CO2 for 2 h [Citation18]. The control group cells without hypoxia treatment were kept in normoxic conditions. 0.5, 1 and 1.5 ng/mL Oxycodone were used to treat CMECs [Citation11]. CMECs were treated in advance with 10 μM BD1047, a SIGMAR1 antagonists, for 30 min before Oxycodone addition [Citation19].
Cell viability assay
CMECs (3000 cells/well) were inoculated into 96-well plates at 37°C for 24 h. Then, 10 μl cell counting kit-8 (CCK-8) reagent was applied to incubate the cells in each well for another 4 h, and the absorbance at 450 nm was determined by means of a microplate reader (Molecular Devices, USA).
Cell transfection
To knockdown SIGMAR1, the specific short hairpin RNAs (shRNAs) targeting SIGMAR1 (shRNA-SIGMAR1-1 or shRNA-SIGMAR1-2) which regarded shRNA-NC as the negative control were designed and synthesized by Genechem (Shanghai, China). Later, these vectors were transfected into cells with the application of Lipofectamine 2000 (cat. no. 11,668,030; Invitrogen, Carlsbad, CA, USA). After 48 h of transfection, the transfection efficiency was evaluated by reverse transcription-quantitative (RT-q) PCR and western blotting.
Immunofluorescence
After different treatment, CMECs were fixed with 4% paraformaldehyde for 30 min, washed with phosphate buffer solution (PBS) for three times, and then treated with 0.1% Triton X-100 (Solarbio, Beijing) at room temperature for 20 min. After being washed, the inhibition of cells by 5% bovine serum albumin (BSA; Solarbio, Beijing) was performed for 30 min at room temperature. Subsequently, cells were carried on incubating overnight with primary antibodies against Zona occludens 1 (ZO-1; cat. no. 20,742-1-AP; Proteintech, Chicago, IL, USA) and Gr-1 (cat. no. bs-23,111 R; Bioss, Beijing China) at 4°C. After that, samples were interacted with anti-rabbit secondary antibody (cat. no. 4412S; Cell Signaling Technology, Boston, MA, USA) at 37°C for 1 h. And the cells were subjected to a light-proof incubation with DAPI for 5 min.
Terminal-deoxynucleoitidyl transferase mediated nick end labeling (TUNEL) staining
The apoptosis of CMECs was determined by means of a TUNEL staining kit (cat. no. C1088; Beyotime, Shanghai, China) following manufacturer’s recommendations. Briefly, cells were first immobilized in 4% paraformaldehyde and subsequently treated with 0.5% Triton X-100. 50 μl TUNEL was employed to incubate the cells for 1 h at 37°C. Nuclei was stained with the adoption of 4’,6-diamidino-2-phenylindole (DAPI) solution. The apoptotic-positive cells were indicated by green staining. Cells from five randomly selected fields of view were imaged under a fluorescence microscope (Olympus Corporation).
RT-qPCR assay
After the isolation of total RNA from hearts tissues and cells with the aid of TRIzol Reagent (Invitrogen, Carlsbad, CA, USA), complementary DNA (cDNA) was synthesized by reverse transcription of RNA using a RevertAid RT Kit (cat. no. K1691; Invitrogen, Carlsbad, CA, USA). PCR was undertaken with the use of PrimeScript™ RT-PCR Kit (cat. no. RR014B; TaKaRa) on an Applied Biosystems (ABI) PCR System 7500 (ABI; Foster City, CA, USA). Primers used in this experiments were as follow: ZO-1, forward 5’-ACAGTACAGCCAGCCAGTTC-3’, reverse 5’-GGCTCAGCAGAGTTTCACCT-3’; Claudin-1, forward 5’-TGGGGCTGATCGCAATCTTT-3’, reverse 5’-ACTTAAGGAGCACCCTTCGC-3’; Occludin, forward 5’-ATTGAGCCCGAGTGGAAAGG-3’, reverse 5’-GAGGTAGCACCACGTTGGAA-3’; TNF-α, forward 5’-AACACACGGACGCTGAAGT-3’, reverse 5’-TCCAGTGAGTTCCGAAAGCC-3’; IL-1β, forward 5’-CCTTGTCGAGAATGGGCAGT-3’, reverse 5’-CAGGGAGGGAAACACACGTT-3’; IL-6, forward 5’-CACTTCACAAGTCGGAGGCT-3’, reverse 5’-AGCACACTAGGTTTGCCGAG-3’; monocyte chemoattractant protein 1 (MCP-1), forward 5’-TAGCATCCACGTGCTGTCTC-3’, reverse 5’-CAGCCGACTCATTGGGATCA-3’; SIGMAR1, forward 5’-ATGGCCATTCGGGACGATAC-3’, reverse 5’-TCATTGCTCCCCAAGAGCTG-3’; Glyceraldehyde-phosphate dehydrogenase (GAPDH), forward 5’-TGTGAACGGATTTGGCCGTA-3’, reverse 5’-GATGGTGATGGGTTTCCCGT-3’. Quantification was undertaken in accordance with a comparative Ct (2−ΔΔCT) method [Citation20]. GAPDH was designated as a standard internal control for relative gene expression.
Western blot analysis
Total proteins were isolated from cardiac tissues and cultured cells utilizing ice-cold RIPA buffer (Beyotime, Shanghai, China), followed by the identification of protein concentrations with the bicinchoninic acid (BCA) Protein Assay kit (cat. no. P0012S; Beyotime, Shanghai, China) in line with the instructions of the supplier. Following protein electrophoresis separation applying sodium dodecyl sulfate-polyacrylamide gel electrophoresis (SDS-PAGE) gels, these proteins were moved onto polyvinylidene fluoride (PVDF) membranes which were blocked for 2 h. Subsequently, appropriate primary antibodies were employed to incubate the membranes at 4°C overnight. The primary antibodies used in this study included anti-ZO-1 (cat. no. 20,742-1-AP; Proteintech, Chicago, IL, USA), anti-Claudin-1 (cat. no. 28,674-1-AP; Proteintech, Chicago, IL, USA), anti-Occludin (cat. no. 91131S; Cell Signaling Technology, Boston, MA, USA), anti-TNF-α (cat. no. 17,590-1-AP; Proteintech, Chicago, IL, USA), anti-IL-1β (cat. no. ab254360; Abcam, Cambridge, UK), anti-IL-6 (cat. no. sc-57,315; Santa Cruz Biotechnology, CA, USA), anti-phospho (p)-NF-κB p65 (cat. no. 3033 T; Cell Signaling Technology, Boston, MA, USA), NF-κB p65 (cat. no. 8242 T; Cell Signaling Technology, Boston, MA, USA), p-inhibitor of kappa B-α (IκB-α) (cat. no. 2859 T; Cell Signaling Technology, Boston, MA, USA), anti-IκB-α (cat. no. 4812S; Cell Signaling Technology, Boston, MA, USA), anti-MCP-1 (cat. no. 25,542-1-AP; Proteintech, Chicago, IL, USA), anti-SIGMAR1 (cat. no. 61994S; Cell Signaling Technology, Boston, MA, USA), anti-B-cell lymphoma 2 (Bcl-2) (cat. no. 60,178-1-Ig; Proteintech, Chicago, IL, USA), anti-Bcl-2-associated X protein (Bax) (cat. no. 2772 T; Cell Signaling Technology, Boston, MA, USA), anti-cleaved caspase3 (cat. no. 9661 T; Cell Signaling Technology, Boston, MA, USA), anti-caspase3 (cat. no. 9662S; Cell Signaling Technology, Boston, MA, USA) and anti-GAPDH (5174 T, Cell Signaling Technology, Boston, MA, USA). Then, these blots were incubated by horseradish peroxidase (HRP)-conjugated secondary antibodies (cat. no. 7074S and cat. no. 7076S; Cell Signaling Technology, Boston, MA, USA) at room temperature for 1 h. After washing with PBS, an Ultra High Sensitivity Enhanced Chemiluminescence kit (cat. no. HY-K1005; MedChemExpress) was prepared and adopted to detect protein bands. Finally, the blotting film was placed in a luminescent imager for photography with Image J software (Version 1.52 r; National Institutes of Health, Bethesda, MA, USA).
Molecular docking
The interaction of Oxycodone and SIGMAR1 was predicted by molecular docking method. The structures of Oxycodone and SIGMAR1 were acquired from the PDB database (https://www.rcsb.org/) and constructed by the Molecular Operating Environment software. Subsequently, the 3D structures of Oxycodone and SIGMAR1 were matched. And the 32-bit command program CMD.exe was used to calculate the affinity between Oxycodone and SIGMAR1.
Statistical analysis
The measured data were presented in the form of mean ± standard deviation. Comparisons among multiple groups were done by One-way analysis of variance (ANOVA) as well as the corresponding Tukey post-hoc test. All data processing was done on GraphPad Prism software 8.0 (La Jolla, CA, USA). The variability among groups was regarded as statistically significant at P-value <0.05.
Results
Oxycodone decreases the ischemic area and improves myocardial function in rat with myocardial I/R injury
Several previous studies have demonstrated the cardioprotective effects of oxycodone on cardiomyocytes under I/R condition by hampering apoptosis [Citation11,Citation12]. However, whether oxycodone could reduce the damage of CMECs during I/R was still unknown. Firstly, the effects of Oxycodone on the myocardial ischemic area were detected by TTC staining. As presented in , the myocardial infarct size in the sham group had no significant difference (vs Control). A significant increase in ischemic area was observed after I/R induction as comparison to the sham group, which was prominently reduced following the further addition of Oxycodone. Additionally, to reflect the myocardial cellular damage, the levels of LDH, CK-MB and cTnl in serum of rats in each group were tested using commercial kits. It could be found that I/R exposure led to notably elevated LDH, CK-MB and cTnl levels when compared to the sham group (). However, Oxycodone treatment inhibited the increase in LDH, CK-MB and cTnl levels induced by I/R. These results implied a protective role of Oxycodone in myocardial I/R injury.
Figure 1. Oxycodone decreased the ischemic area and improved myocardial function in rat with myocardial I/R injury. (a) The myocardial ischemic area was identified through TTC staining. (b) Percentage of myocardial infarct volume. The levels of (c) LDH, (d) CK-MB and (e) cTnl in serum were evaluated with commercial kits. ***P < 0.001.
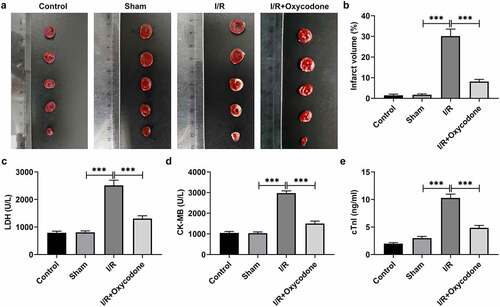
Oxycodone ameliorates myocardial histopathological injury and enhances endothelial integrity in rat with myocardial I/R injury
Then, H&E staining was performed to evaluate the effects of Oxycodone on the pathological changes of myocardial tissues in each group. As displayed in , the myocardia in the sham group were arranged regularly and showed no significant changes relative to the control group. By contrast, in the I/R group, the myocardia were arranged disorganized and ruptured, with inflammatory cells in the interstitial tissues. Particularly, Oxycodone administration distinctly ameliorated the myocardial histopathological injury induced by I/R stimulation. Results from revealed that I/R stimulation caused conspicuously downregulated protein and mRNA expressions of tight junction proteins such as ZO-1, Claudin-1 and Occludin, in myocardial tissues in comparison with the sham group, which were partially reversed by Oxycodone treatment. Meanwhile, immunofluorescence results indicated that I/R-induced decrease in ZO-1 level was markedly elevated by Oxycodone (). These findings suggested that Oxycodone could attenuate myocardial histopathological injury and enhance endothelial integrity in rat with myocardial I/R injury.
Figure 2. Oxycodone ameliorated myocardial histopathological injury and enhanced endothelial integrity in rat with myocardial I/R injury. (a) The myocardial histopathological changes were measured by H&E staining. The (b) protein and (c) mRNA expressions of ZO-1, Claudin-1 and Occludin in myocardial tissues were examined by western blot analysis and RT-qPCR, respectively. (d) Immunofluorescent staining was applied for the determination of ZO-1 expression. ***P < 0.001.
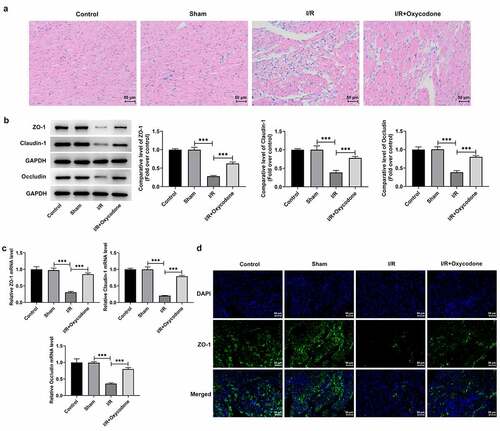
Oxycodone attenuates inflammatory response in myocardial tissues of rat with myocardial I/R injury
To study the effects of Oxycodone on the inflammation of myocardial tissues during myocardial I/R injury, the levels of TNF-α, IL-1β and IL-6, in serum and heart tissues were detected with ELISA and RT-qPCR. As what is observable from , no significant difference was found in the levels of TNF-α, IL-1β and IL-6 between sham and control groups. On the contrary, I/R exposure dramatically increased the secretion of these inflammatory factors as comparison to the sham group, whereas the addition of Oxycodone alleviated the impacts of I/R induction on TNF-α, IL-1β and IL-6 levels. Concurrently, the protein levels of TNF-α, IL-1β and IL-6 tested by western blot analysis presented the same results as ELISA and RT-qPCR assay (). Besides, it could be found in that the expression levels of p/t-NF-κB p65 and p/t-IκB-α were obviously elevated in the I/R group compared with the sham group, which were restored by Oxycodone addition. Moreover, the expressions of Gr-1 and MCP-1 was evaluated. As exhibited in /R stimulation notably enhanced Gr-1 and MCP-1 expressions, which was partially counteracted by Oxycodone. These observations revealed that Oxycodone ameliorated inflammatory response in myocardial tissues of rat with myocardial I/R injury.
Figure 3. Oxycodone attenuated inflammatory response in myocardial tissues of rat with myocardial I/R injury. (a) The releases of TNF-α, IL-1β and IL-6 in rat serum were detected utilizing ELISA. The (b) mRNA and (c) protein expressions of TNF-α, IL-1β and IL-6 were examined with RT-qPCR and western blotting. (d) Analysis of p-NF-κB p65 and p-IκB-α proteins was conducted using western blot assay. **P < 0.01, ***P < 0.001.
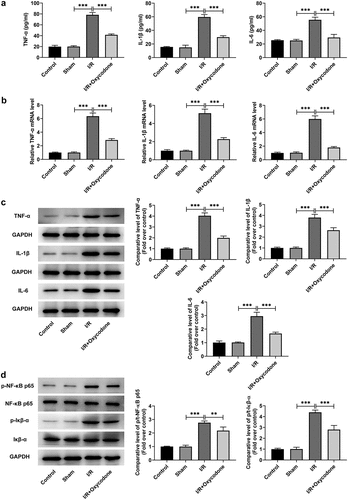
Figure 4. Oxycodone mitigated inflammatory response in myocardial tissues of rat with myocardial I/R injury. (a) Detection of Gr-1 expression was carried out through immunofluorescent staining. The (b) mRNA and (c) protein expressions of MCP-1 were assessed with RT-qPCR and western blot analysis. **P < 0.01, ***P < 0.001.
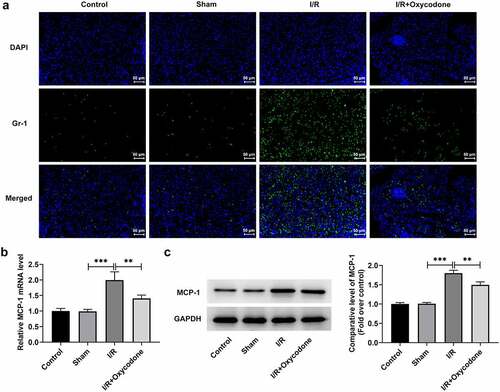
Oxycodone activates SIGMAR1 expression in I/R-induced rat myocardial tissues and H/R-induced CMECs
To explore the potential mechanisms of Oxycodone in the protective effects of myocardial I/R injury, Swisstarget database was used to predict the molecule that could be targeted by Oxycodone. SIGMAR1 was found to be a potential protein that could be combined with Oxycodone, which was confirmed by the result of molecular docking experiments (). Then, it was illustrated in that SIGMAR1 expression was notably decreased in the I/R group relative to the sham group. On the contrary, the further Oxycodone addition increased SIGMAR1 level when compared to the I/R group. In vitro, H/R treatment also upregulated SIGMAR1 expression when compared to the control group (). Afterward, it was found that 0.5, 1 and 1.5 ng/ml Oxycodone had no obvious impacts on the viability of CMECs (). Furthermore, under the H/R condition, CMECs exhibited low SIGMAR1 expression as comparison to the control group (). However, Oxycodone dose-dependently elevated SIGMAR1 expression in H/R-induced CMECs. Through the above findings, we proved that Oxycodone could target and upregulate SIGMAR1 expression in I/R-induced rat myocardial tissues and H/R-induced CMECs.
Figure 5. Oxycodone could target and upregulate SIGMAR1 expression in I/R-induced rat myocardial tissues and H/R-induced CMECs. (a) The molecular docking result between Oxycodone and SIGMAR1. The (b) mRNA and (c) protein expressions of SIGMAR1 in I/R-induced rat myocardial tissues were assessed using RT-qPCR and western blotting. The (d) mRNA and (e) protein expression levels of SIGMAR1 were detected by RT-qPCR and western blotting. (f) Cell viability was tested by a CCK-8 assay after CMECs being treated with different concentrations of Oxycodone. (g) SIGMAR1 expression was tested with the application of western blot analysis in H/R-induced CMECs with Oxycodone treatment. ***P < 0.001.
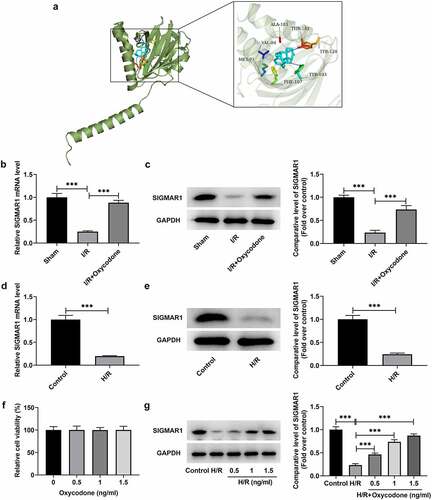
Oxycodone reduces apoptosis of H/R-induced CMECs by upregulating SIGMAR1 expression
To further explore whether the impacts of Oxycodone on I/R-induced myocardial damage were realized by regulating SIGMAR1 expression, SIGMAR1 was silenced in CMECs by transfection with shRNAs targeting SIGMAR1. As displayed in , SIGMAR1 expression was conspicuously downregulated in the shRNA-SIGMAR1-1 and shRNA-SIGMAR1-2 groups compared with the shRNA-NC group. CMECs transfected with shRNA-SIGMAR1-1 were selected to conduct subsequent experiments due to its better transfection efficiency. Then, as what is observable from /R stimulation-induced loss of CMECs viability, which was then increased after Oxycodone addition. By contrast, the further SIGMAR1 deletion reduced cell viability when compared to the H/R + 1.5 ng/ml Oxycodone+shRNA-NC group. The addition of BD1047, a SIGMAR1 antagonist, exhibited the same effects on cell viability as shRNA-SIGMAR1 transfection. Afterward, results obtained from indicated that H/R-induced increase in apoptosis of CMECs was significantly decreased by Oxycodone treatment. On the contrary, SIGMAR1 silencing and BD1047 intervention markedly elevated cell apoptosis. Meanwhile, a drop in the expression of Bcl-2 was observed in H/R-stimulated CMECs, accompanied by upregulated Bax and cleaved caspase3 expression, which was reversed by Oxycodone treatment (). However, SIGMAR1 silencing and BD1047 intervention attenuated the impacts of Oxycodone on the expression of aforementioned apoptosis-related proteins. These data evidenced that Oxycodone inhibited apoptosis of H/R-induced CMECs by upregulating SIGMAR1 expression.
Figure 6. Oxycodone reduced apoptosis of H/R-induced CMECs by upregulating SIGMAR1 expression. The (a) mRNA and (b) protein expressions of SIGMAR1 were examined with the use of RT-qPCR and western blotting. (c) Assessment of cell viability employed CCK-8. (d-e) Cell apoptosis estimation was undertaken utilizing TUNEL. (f) Western blot analysis was adopted for the evaluation of apoptosis-related proteins. *P < 0.05, **P < 0.01, ***P < 0.001.
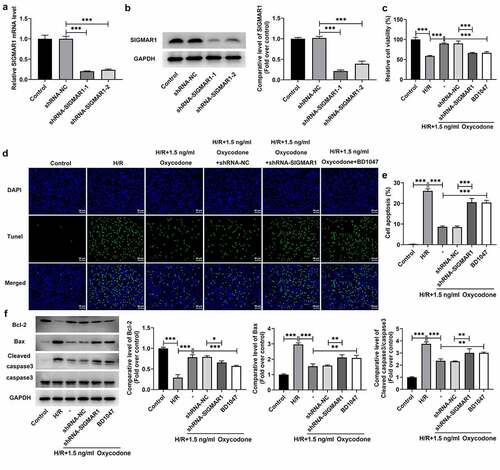
Oxycodone enhances endothelial integrity of H/R-induced CMECs by upregulating SIGMAR1 expression
Disruption of endothelial integrity is a common pathological process following myocardial I/R, which promotes neutrophil infiltration, inflammation and ultimately impairs myocardial function [Citation21]. Then, endothelial integrity was evaluated in H/R-induced CMECs with Oxycodone treatment. It was found that H/R stimulation downregulated ZO-1, Claudin-1 and Occludin expressions at transcriptional level and post-transcriptional level relative to the control group, which were restored by Oxycodone addition (). However, SIGMAR1 knockdown and BD1047 application alleviated the impacts of Oxycodone on ZO-1, Claudin-1 and Occludin expressions in H/R-induced CMECs. Consistently, immunofluorescent staining showed the same results on ZO-1 as RT-qPCR and western blotting (). Together, these findings suggested that Oxycodone could enhance endothelial integrity of H/R-induced CMECs by upregulating SIGMAR1 expression.
Figure 7. Oxycodone could enhance endothelial integrity of H/R-induced CMECs by upregulating SIGMAR1 expression. The (a) mRNA and (b) protein expression levels of ZO-1, Claudin-1 and Occludin were examined employing RT-qPCR and western blot analysis, respectively. (d) Immunofluorescent staining was applied for the identification of ZO-1 expression. *P < 0.05, **P < 0.01, ***P < 0.001.
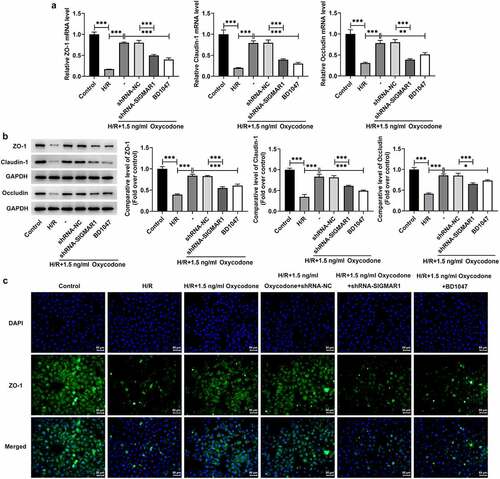
Discussion
Myocardial infarction is a common and serious clinical disease and a threat to public health worldwide in the future and tissue damage caused by myocardial ischemia is an important cause of fatal diseases [Citation22,Citation23]. In the present study, the protective effects of Oxycodone on myocardial I/R injury were investigated in vivo and in vitro. Results demonstrated that Oxycodone decreased the ischemic area, improved myocardial function, ameliorated myocardial histopathological injury and enhanced endothelial integrity in rat with myocardial I/R injury. The further in vitro experiments suggested that Oxycodone alleviated myocardial I/R injury by binding to SIGMAR1.
Multiple mechanisms contribute to the progression of myocardial I/R injury. Among them, endothelial damage is a key mediator of myocardial I/R injury. Endothelial cells make up the endocardial epithelium and the inner layer of myocardial capillaries, which are much more sensitive to I/R in the heart than cardiomyocytes [Citation24,Citation25]. Baars et al. have highlighted that amelioration of endothelial injury can improve long-term outcomes in patients and animals suffering from myocardial infarction [Citation26]. A previous study has shown that I/R induction can upregulate the expression of surface adhesion molecules on endothelial cells and recruit neutrophils, which further damages the endothelial cells [Citation21]. Disruption of endothelial integrity is a common pathological process following myocardial I/R, which promotes neutrophil infiltration, inflammation and ultimately impairs myocardial function. Endothelial integrity depends on the intercellular junction complex between adjacent endothelial cells, and ZO-1 has been shown to be one of the key components in this complex [Citation27]. Occludin and Claudin-1 are two important transmembrane tight junction proteins [Citation28]. Although distributed mainly in the nervous system, opioid receptors have also been proven to be expressed in endothelial cells [Citation29]. Oxycodone has been demonstrated to ameliorate permeability damage of pulmonary microvascular endothelial cells in acute lung injury-induced rat model via inhibiting inflammation and apoptosis [Citation8]. Shao et al. held the opinion that oxycodone treatment notably improved the permeability damage by elevating the expression of ZO-1 and occludin in oxygen-glucose deprivation/reoxygenation-induced brain microvascular endothelial cells [Citation30]. It is noteworthy that the cardioprotective effects of Oxycodone are reflected in its ability to prevent myocardial damage from I/R by suppressing apoptosis of cardiomyocytes [Citation11,Citation12]. This study was the first to explore the effects of Oxycodone on endothelial integrity in rat with myocardial I/R injury. We found that Oxycodone greatly upregulated ZO-1, Occludin and Claudin-1 expressions in rat myocardial tissues during myocardial I/R injury, suggesting that Oxycodone could effectively improve myocardial endothelial cell integrity.
Myocardial inflammation has been recognized as a key hallmark of myocardial I/R injury [Citation31–33]. Compelling evidence indicate that a large number of inflammatory mediators and chemokines are produced during myocardial I/R injury, and leukocytes, platelets and vascular endothelial cells are activated to express a large number of adhesion molecules, which promote the adhesion of leukocytes to vascular endothelial cells [Citation34]. Furthermore, I/R increases endothelial permeability by disrupting endothelial barrier function, which will exacerbate myocardial inflammation after I/R stimulation [Citation35]. TNF-α, IL-1β, and IL-6 are important inflammatory factors that play crucial roles in cell damage during myocardial I/R [Citation36,Citation37]. Additionally, the accumulation of neutrophils in the myocardium is a key event in myocardial I/R injury. In the early inflammatory response after acute myocardial injury, neutrophils dominate the infiltrating immune cell population and cause the greatest tissue damage [Citation38]. Gr-1 and MCP-1 are important markers to reflect neutrophil infiltration in myocardial I/R injury [Citation39]. Myocardial I/R damage exhibits a distinct relationship with cell apoptosis [Citation40]. A previous study has suggested that in isolated perfused hearts subjected to I/R, endothelial apoptosis occurs earlier than it does in cardiomyocytes [Citation41]. Therefore, strategies aimed at suppressing the inflammatory response and apoptosis favor amelioration of myocardial I/R injury. In recent years, an increasing number of researches have focused on the anti-inflammatory and anti-apoptotic effects of Oxycodone. For instance, by inhibiting NF-κB signaling, Oxycodone inhibited lipopolysaccharide-induced neuroinflammation in hippocampal astrocytes [Citation9]. Kong et al. demonstrated that Oxycodone hampered oxygen-glucose deprivation/recovery-induced hippocampal neurons apoptosis in rats [Citation10]. Oxycodone attenuated pulmonary microvascular permeability via inhibiting inflammation and apoptosis [Citation8]. Similarly, the present study revealed that Oxycodone treatment relieved the inflammation and apoptosis, evidenced by the decreased levels of TNF-α, IL-1β, IL-6, p-NF-κB p65, p-IκB-α, Gr-1, MCP-1, Bax, cleaved caspase3 and increased level of Bcl-2. These results demonstrated the inhibitory effects of Oxycodone on inflammation and apoptosis in I/R-challenged rat myocardial tissues and H/R-induced CMECs.
To study the mechanism of Oxycodone action on myocardial I/R injury, the possible targets were predicated by using swisstarget database and SIGMAR1 was noticed as a potential target of Oxycodone, which was further confirmed by the result of molecular docking analysis. As a molecular chaperone, SIGMAR1 possesses multiple cellular functions and is implicated in various diseases. For example, the activation of SIGMAR1 alleviated the release of inflammatory cytokines by retinal Müller glial cells [Citation42]. Accumulating study suggested that the induction of SIGMAR1 expression exhibited outstanding neuroprotective effects in Parkinson’s disease and Alzheimer’s disease [Citation43–45]. Additionally, overexpression of SIGMAR1 has been demonstrated to inhibit cell apoptosis previous in vivo and in vitro studies [Citation46,Citation47]. Importantly, SIGMAR1, a molecular chaperone protein commonly expressed in the body, is also widely expressed in myocardial tissues and in CMECs [Citation48]. SIGMAR1 loss of function results in the impairment of mitochondrial function and adverse cardiac remodeling, ultimately leading to cardiac contractile dysfunction [Citation49]. It has been well reported that activation of SIGMAR1 relieved myocardial apoptosis in rats suffering from myocardial I/R injury [Citation14]. Particularly, chronic SIGMAR1 activation can improve the ventricular remodeling after myocardial infarction in rats and decrease the susceptibility to ventricular arrhythmia [Citation15], suggesting that SIGMAR1 might help to alleviate myocardial I/R injury. In this study, the in vivo and in vitro experiments supported that Oxycodone conspicuously upregulated SIGMAR1 expression after I/R or H/R stimulation. The rescue experiments indicated that SIGMAR1 silencing or SIGMAR1 antagonists BD1047 intervention alleviated the protective effects of Oxycodone on H/R-induced damage of CMECs, suggesting that Oxycodone attenuated myocardial I/R injury by binding to SIGMAR1.
Of course, our experiment has a limitation. In this study, we only discussed the regulatory effect of Oxycodone on SIGMAR1 by silencing SIGMAR1 or adding SIGMAR1 antagonist in H/R-induced CMECs. The further in vivo experiments involved in transgenic animals will be performed in the future investigation to support the conclusion obtained in this study.
Conclusion
Collectively, this study is the first evidence confirming the protective effects of Oxycodone on myocardial I/R injury from the perspective of cardiac microvascular endothelial cells. We demonstrated that Oxycodone can alleviate myocardial I/R damage in both I/R-exposed rats and H/R-induced CMECs by binding to SIGMAR1 and upregulating SIGMAR1 expression. Our results might provide a rationale to use Oxycodone, a multiple-opioid receptor agonist, as a new therapy to treat myocardial I/R injury via targeting SIGMAR1, suggesting that Oxycodone may be a promising complementary agent for clinically treating ischemic heart disease.
Ethics approval and consent to participate
All animal studies were approved by the Animal Research Ethics Committee of Nanjing First Hospital, Nanjing Medical University.
Consent for publication
Not applicable
Availability of data and materials
All data present in the paper is available for reasonable request to the corresponding author.
Supplemental Material
Download Zip (183.4 MB)Acknowledgements
Not applicable.
Disclosure statement
No potential conflict of interest was reported by the author(s).
Supplementary material
Supplemental data for this article can be accessed here
Additional information
Funding
References
- Li W, Li Y, Chu Y, et al. PLCE1 promotes myocardial ischemia-reperfusion injury in H/R H9c2 cells and I/R rats by promoting inflammation. Biosci Rep. 2019;39:BSR20181613.
- Anderson JL, Morrow DA. Acute myocardial infarction. N Engl J Med. 2017;376:2053–2064.
- Davidson SM, Ferdinandy P, Andreadou I, et al. Multitarget strategies to reduce myocardial ischemia/reperfusion injury: JACC review topic of the week. J Am Coll Cardiol. 2019;73:89–99.
- Bai J, Wang Q, Qi J, et al. Promoting effect of baicalin on nitric oxide production in CMECs via activating the PI3K-AKT-eNOS pathway attenuates myocardial ischemia-reperfusion injury. Phytomedicine. 2019;63:153035.
- Shang J, Gao ZY, Zhang LY, et al. Over-expression of JAZF1 promotes cardiac microvascular endothelial cell proliferation and angiogenesis via activation of the Akt signaling pathway in rats with myocardial ischemia-reperfusion. Cell Cycle. 2019;18:1619–1634.
- Cui H, Li X, Li N, et al. Induction of autophagy by Tongxinluo through the MEK/ERK pathway protects human cardiac microvascular endothelial cells from hypoxia/reoxygenation injury. J Cardiovasc Pharmacol. 2014;64:180–190.
- Sodha NR, Boodhwani M, Clements RT, et al. Coronary microvascular dysfunction in the setting of chronic ischemia is independent of arginase activity. Microvasc Res. 2008;75:238–246.
- Li X, Li R, Fang Q, et al. Oxycodone attenuates vascular leak and lung inflammation in a clinically relevant two-hit rat model of acute lung injury. Cytokine. 2021;138:155346.
- Zhou L, Fan L, Kong C, et al. Oxycodone suppresses the lipopolysaccharide-induced neuroinflammation by downregulating nuclear factor-kappaB in hippocampal astrocytes of Sprague-Dawley rats. Neuroreport. 2020;31:99–108.
- Kong C, Miao F, Wu Y, et al. Oxycodone suppresses the apoptosis of hippocampal neurons induced by oxygen-glucose deprivation/recovery through caspase-dependent and caspase-independent pathways via kappa- and delta-opioid receptors in rats. Brain Res. 2019;1721:146319.
- Zhao S, Zhang C, Pi Z, et al. Oxycodone protects cardiomyocytes from ischemia-reperfusion-induced apoptosis via PI3K/Akt pathway. Pharmazie. 2020;75:430–435.
- Xie Y, Ge CL, Zhang ZY, et al. Oxycodone inhibits myocardial cell apoptosis after myocardial ischemia-reperfusion injury in rats via RhoA/ROCK1 signaling pathway. Eur Rev Med Pharmacol Sci. 2020;24:6371–6379.
- Rousseaux CG, Greene SF. Sigma receptors [sigmaRs]: biology in normal and diseased states. J Recept Signal Transduct Res. 2016;36:327–388.
- Gao QJ, Yang B, Chen J, et al. Sigma-1 receptor stimulation with PRE-084 ameliorates myocardial ischemia-reperfusion injury in rats. Chin Med J (Engl). 2018;131:539–543.
- Fo Y, Zhang C, Chen X, et al. Chronic sigma-1 receptor activation ameliorates ventricular remodeling and decreases susceptibility to ventricular arrhythmias after myocardial infarction in rats. Eur J Pharmacol. 2020;889:173614.
- Guo J, Wang SB, Yuan TY, et al. Coptisine protects rat heart against myocardial ischemia/reperfusion injury by suppressing myocardial apoptosis and inflammation. Atherosclerosis. 2013;231:384–391.
- Zhou H, Zhang Y, Hu S, et al. Melatonin protects cardiac microvasculature against ischemia/reperfusion injury via suppression of mitochondrial fission-VDAC1-HK2-mPTP-mitophagy axis. J Pineal Res. 2017 ;63:e12413
- Zhang Y, Zhou H, Wu W, et al. Liraglutide protects cardiac microvascular endothelial cells against hypoxia/reoxygenation injury through the suppression of the SR-Ca(2+)-XO-ROS axis via activation of the GLP-1R/PI3K/Akt/survivin pathways. Free Radic Biol Med. 2016;95:278–292.
- Wu Z, Li L, Zheng LT, et al. Allosteric modulation of sigma-1 receptors by SKF83959 inhibits microglia-mediated inflammation. J Neurochem. 2015;134:904–914.
- Livak KJ, Schmittgen TD. Analysis of relative gene expression data using real-time quantitative PCR and the 2(-Delta Delta C(T)) method. methods (san diego. Calif.). 2001;25:402–408.
- Kong Q, Dai L, Wang Y, et al. HSPA12B attenuated acute myocardial ischemia/reperfusion injury via maintaining endothelial integrity in a PI3K/Akt/mTOR-dependent mechanism. Sci Rep. 2016;6:33636.
- Liebelt JJ, Yang Y, DeRose JJ, et al. Ventricular septal rupture complicating acute myocardial infarction in the modern era with mechanical circulatory support: a single center observational study. Am J Cardiovasc Dis. 2016;6:10–16.
- Xiao SH, Wang Y, Cao X, et al. Long non-coding RNA LUCAT1 inhibits myocardial oxidative stress and apoptosis after myocardial infarction via targeting microRNA-181a-5p. Bioengineered. 2021;12:4546–4555.
- Singhal AK, Symons JD, Boudina S, et al. Role of endothelial cells in myocardial ischemia-reperfusion injury. Vasc Dis Prev. 2010;7:1–14.
- Tuuminen R, Syrjala S, Krebs R, et al. Donor simvastatin treatment abolishes rat cardiac allograft ischemia/reperfusion injury and chronic rejection through microvascular protection. Circulation. 2011;124:1138–1150.
- Baars T, Konorza T, Kahlert P, et al. Coronary aspirate TNFalpha reflects saphenous vein bypass graft restenosis risk in diabetic patients. Cardiovasc Diabetol. 2013;12:12.
- Schlegel N, Waschke J. Vasodilator-stimulated phosphoprotein: crucial for activation of Rac1 in endothelial barrier maintenance. Cardiovasc Res. 2010;87:1–3.
- Tong N, Zhang Z, Zhang W, et al. Diosmin alleviates retinal edema by protecting the blood-retinal barrier and reducing retinal vascular permeability during ischemia/reperfusion injury. PLoS One. 2013;8:e61794.
- Grace PM, Ramos KM, Rodgers KM, et al. Activation of adult rat CNS endothelial cells by opioid-induced toll-like receptor 4 (TLR4) signaling induces proinflammatory, biochemical, morphological, and behavioral sequelae. Neuroscience. 2014;280:299–317.
- Shao F, Han D, Shen Y, et al. Oxycodone relieves permeability damage and apoptosis of oxygen-glucose deprivation/reoxygenation-induced brain microvascular endothelial cells through ras homolog family member A (RhoA)/ Rho-associated coiled-coil containing kinases (ROCK)/ myosin light chain 2 (MLC2) signal. Bioengineered. 2022;13:5205–5215.
- Ge X, Meng Q, Wei L, et al. Myocardial ischemia-reperfusion induced cardiac extracellular vesicles harbour proinflammatory features and aggravate heart injury. J Extracell Vesicles. 2021;10:e12072.
- Wallert M, Ziegler M, Wang X, et al. alpha-Tocopherol preserves cardiac function by reducing oxidative stress and inflammation in ischemia/reperfusion injury. Redox Biol. 2019;26:101292.
- Ke WL, Huang ZW, Peng CL, et al. m(6)A demethylase FTO regulates the apoptosis and inflammation of cardiomyocytes via YAP1 in ischemia-reperfusion injury. Bioengineered. 2022;13:5443–5452.
- Slegtenhorst BR, Dor FJ, Rodriguez H, et al. Ischemia/reperfusion injury and its consequences on immunity and inflammation. Curr Transplant Rep. 2014;1:147–154.
- Eltzschig HK, Eckle T. Ischemia and reperfusion–from mechanism to translation. Nat Med. 2011;17:1391–1401.
- Pudil R, Pidrman V, Krejsek J, et al. Cytokines and adhesion molecules in the course of acute myocardial infarction. Clin Chim Acta. 1999;280:127–134.
- Yuvaraj S, Ramprasath T, Saravanan B, et al. Chrysin attenuates high-fat-diet-induced myocardial oxidative stress via upregulating eNOS and Nrf2 target genes in rats. Mol Cell Biochem. 2021;476:2719–2727.
- Schofield ZV, Woodruff TM, Halai R, et al. Neutrophils–a key component of ischemia-reperfusion injury. Shock. 2013;40:463–470.
- Qin X, Peterson MR, Haller SE, et al. Caspase recruitment domain-containing protein 9 (CARD9) knockout reduces regional ischemia/reperfusion injury through an attenuated inflammatory response. PLoS One. 2018;13:e0199711.
- Zhao Q, Cui Z, Zheng Y, et al. Fenofibrate protects against acute myocardial I/R injury in rat by suppressing mitochondrial apoptosis as decreasing cleaved caspase-9 activation. Cancer Biomark. 2017;19:455–463.
- Scarabelli T, Stephanou A, Rayment N, et al. Apoptosis of endothelial cells precedes myocyte cell apoptosis in ischemia/reperfusion injury. Circulation. 2001;104:253–256.
- Shanmugam A, Wang J, Markand S, et al. Sigma receptor 1 activation attenuates release of inflammatory cytokines MIP1gamma, MIP2, MIP3alpha, and IL12 (p40/p70) by retinal Muller glial cells. J Neurochem. 2015;132:546–558.
- Behensky AA, Yasny IE, Shuster AM, et al. Stimulation of sigma receptors with afobazole blocks activation of microglia and reduces toxicity caused by amyloid-beta25-35. J Pharmacol Exp Ther. 2013;347:458–467.
- Francardo V, Bez F, Wieloch T, et al. Pharmacological stimulation of sigma-1 receptors has neurorestorative effects in experimental parkinsonism. Brain. 2014;137:1998–2014.
- Nguyen L, Kaushal N, Robson MJ, et al. Sigma receptors as potential therapeutic targets for neuroprotection. Eur J Pharmacol. 2014;743:42–47.
- Wang L, Eldred JA, Sidaway P, et al. Sigma 1 receptor stimulation protects against oxidative damage through suppression of the ER stress responses in the human lens. Mech Ageing Dev. 2012;133:665–674.
- Zhang Y, Shi Y, Qiao L, et al. Sigma-1 receptor agonists provide neuroprotection against gp120 via a change in bcl-2 expression in mouse neuronal cultures. Brain Res. 2012;1431:13–22.
- Bhuiyan MS, Fukunaga K. Targeting sigma-1 receptor signaling by endogenous ligands for cardioprotection. Expert Opin Ther Targets. 2011;15:145–155.
- Abdullah CS, Alam S, Aishwarya R, et al. Cardiac dysfunction in the sigma 1 receptor knockout mouse associated with impaired mitochondrial dynamics and bioenergetics. J Am Heart Assoc. 2018;7:e009775.