ABSTRACT
The aim of this study was to investigate whether HUCMSCsWnt10b could promote long bone fracture healing. Commercially-available HUCMSCsEmp (human umbilical cord mesenchymal stem cells transfected with empty vector) in hydrogel, HUCMSCsWnt10b in hydrogel and HUCMSCsWnt10b with the Wnt signaling pathway inhibitor IWR-1 were transplanted into the fracture site in a rat model of femoral fracture. We found that transplantation of HUCMSCsWnt10b significantly accelerated bone healing in a rat model of femoral fracture. Meanwhile, three-point bending test proved that the mechanical properties of the bone at the fracture site in the HUCMSCWnt10b treatment group were significantly better than those of the other treatment groups. To understand the cellular mechanism, we explored the viability of periosteal stem cells (PSCs), as they contribute the greatest number of osteoblast lineage cells to the callus. In line with in vivo data, we found that conditioned medium from HUCMSCsWnt10b enhanced the migration and osteogenic differentiation of PSCs. Furthermore, conditioned medium from HUCMSCsWnt10b also induced endothelial cells to form capillary-like structures in a tube formation assay, which was blocked by SU5416, an angiogenesis inhibitor, suggesting that enhanced vessel formation and growth also contribute to accelerated hard callus formation. In summary, our study demonstrates that HUCMSCsWnt10b promote fracture healing via accelerated hard callus formation, possibly due to enhanced osteogenic differentiation of PSCs and vessel growth. Therefore, HUCMSCsWnt10b may be a promising treatment for long bone fractures.
Grapical abstract
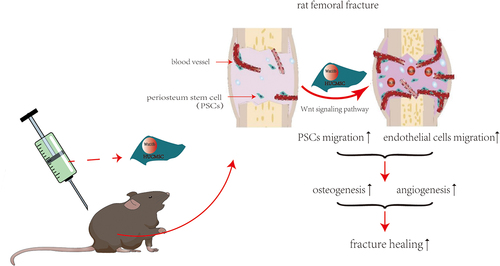
Research highlights
• We constructed novel transgenic HUCMSCs for bone regeneration
• HUCMSCsWnt10b significantly promoted healing in a rat femoral fracture model
• HUCMSCsWnt10b accelerated turnover of cartilage callus to hard callus
• HUCMSCsWnt10b showed strong osteogenic and angiogenic effects.
1 Introduction
Approximately five million patients experience a fracture each year in China, of whom 3–7% suffer delayed healing or nonunion fractures [Citation1]. These failures are a substantial physical, medical and financial burden for both individuals and healthcare systems [Citation2]. Consequently, further clarification of the pathological mechanism of delayed union or nonunion of fractures in the fracture microenvironment under pathological conditions is a significant issue for both surgeons and basic scientists.
Long bone fracture healing is a complex physiological process known as endochondral ossification, which is divided into three stages: the inflammatory hematoma formation period, the callus formation period and the bone remodeling period [Citation3]. This sequence of events is totally different from that of flat bones such as the calvaria, were new bone is formed via intramembranous ossification. At each stage, this continuous change in cellular function is precisely regulated by a series of signal transduction pathways, such as Wingless/Integrated (Wnt)/β-catenin, bone morphogenetic protein (BMP)/transforming growth factor beta (TGF-β), etc [Citation4]. During fracture healing, the callus provides a microenvironment that promotes bone formation and maintains temporary biomechanical stability [Citation5]. Consequently, callus formation disorder is an important cause of atrophic fracture nonunion [Citation6,Citation7]. The osteogenic lineage cells in the callus are mainly derived from periosteal stem cells (PSCs), so impairment of PSC function is an important cause of bone regeneration disorders [Citation8–10]. The Wnt signaling pathway has important biological functions during bone regeneration [Citation11,Citation12], and changes in serum Wnt protein levels are highly correlated with fracture healing progress [Citation13]. We previously found that Wnt10b-overexpressing human umbilical cord mesenchymal stem cells (HUCMSCsWnt [Citation10]b) significantly promoted calvarial defect healing through enhanced osteogenesis and angiogenesis. However, due to the totally different mechanism of long bone fracture healing, whether Wnt10b-overexpressing HUCMSCs are a suitable treatment for fracture healing in long bones is still unknown.
We hypothesized that HUCMSCsWnt [Citation10]b could promote fracture healing by enhancing the function of both PSCs and vascular endothelial cells. Therefore, in this study, we generated HUCMSCsWnt [Citation10]b to explore their effects on femoral fracture healing in a rat model. Our study may provide a promising cell source for fracture healing.
2 Materials and methods
2.1 Construction of HUCMSCsWnt [Citation10]b
HUCMSCs (human umbilical cord mesenchymal stem cells) were purchased from Wuhan Hamilton Biotechnology Co., LTD, and inoculated into 6-well plates at 4 × 106 cells/well. When cells reached 90% confluence in each well, the culture medium (Huxuc-03061; Cyagen Biosciences Inc., Guangzhou, China) was changed and lentiviral vector was used to introduce the Wnt10b gene to construct transgenic umbilical cord mesenchymal stem cells (HUCMSCsWnt [Citation10]b); umbilical cord mesenchymal stem cells transfected with empty vector (HUCMSCsEmp) were used as controls. Both were provided by the technical department of Wuhan Hamilton Biotechnology Co., Ltd [Citation14].
2.2 Rat femoral fracture model
All animal experiments in this study followed the guiding principles of the Animal Welfare Law and were approved by the Animal Care and Use Committee of the Union Hospital of Huazhong University of Science and Technology (Ethical approval number: 2644). Fractures were surgically created in 8 weeks-old Sprague-Dawley (SD) rats at different time points (1, 2, 4 and 6 weeks, n = 8 in each group at each time point), and the following treatments are introduced separately: (A) saline (veh) (B) hydrogel (gel) (C) hydrogel with HUCMSCsEmp (D) hydrogel with HUCMSCWnt [Citation10]b (E) hydrogel with HUCMSCWnt [Citation10]b + IWR-1. Rats were anesthetized by injection with Amobarbital Sodium (10%, 0.5 mL/100 g). A Kirschner wire was inserted into the upper end of the femur for internal fixation, then the midshaft of the femur was cut to create a femoral fracture model. The wound was disinfected and sutured, and antibiotics were given for the first three days after operation to combat infection. Hydrogel was used to support the cells locally and prevent cells leakage; cells (1 × 105) were mixed with an equivalent volume of commercially-available hydrogel (BD™, Puramatrix™ catalog number 354,250; BD Biosciences, Franklin Lakes, NJ, USA) and placed at the fracture site [Citation14,Citation15].
2.3 Micro-CT analysis
Femurs were collected at 1, 2, 4 and 6 weeks after surgery, and fixed with 4% paraformaldehyde for 48 hours. Then a SkyScan 1176 high-resolution micro-CT imaging system (micro-CT) was used to scan the femurs at 18 μm resolution, with a 1 mm aluminum filter, 90 kV voltage and 273 μA current. The raw data obtained were processed using SkyScan NRecon reconstruction software and CT-VOX three-dimensional reconstruction analysis software for volume reconstruction and three-dimensional image generation. Bone volume fraction (BT/TV) was determined by three-dimensional standard microstructure analysis. Based on micro-CT images, fracture healing scores were calculated from 4-week fracture samples based on an improved five-point X-ray scoring system [Citation10].
2.4 Three-point bending test
The stored bone specimens were equilibrated at room temperature then placed across two fulcrums 5 mm apart. An axial load was then applied to the fractured part between the two fulcrums. The load was applied at a rate of 5 mm/min until the bone broke. This test was performed with a universal three-point bending machine. The load-failure curve was recorded and the max-load, max-stress and elastic modulus were quantified [Citation16].
2.5 Histology and immunohistochemistry
The rat femurs were collected and fixed with 4% paraformaldehyde for 24 hours, and then decalcified at room temperature with 10% EDTA for 30 days. After that, the specimens were dehydrated with a graded series of ethanol concentrations and embedded in paraffin. After embedding, the paraffin-embedded specimens were cut into sagittal sections at a thickness of 6 μm, and stained with safranin O-fast green.
Paraffin sections were also used for immunohistochemistry after appropriate antigenic repair and slides were incubated overnight at 4°C with antibodies against CD31(1:100, BD, 555025), and osteocalcin (OCN; 1:100, SC-365797). Next day, the secondary antibody was applied, and the results was visualized with DAB color solution. After color development was complete, the slides were counterstained with hematoxylin for 2 minutes, then dehydrated with 100% anhydrous ethanol, coverslipped and sealed. This experiment was conducted by Hubei Baos Biotechnology Co., Ltd., which provided all the reagents except the primary antibodies.
2.6 Conditioned medium collection
HUCMSCsEmp and HUCMSCWnt [Citation10]b were seeded into 6-well plates and cultured until they reached 80%–90% confluence, then washed three times with phosphate-buffered saline and changed to serum-free α-MEM. Next day the conditioned medium was collected and centrifuged at 2,000 rpm for 5 minutes to remove cell debris before the medium was used in experiments [Citation17].
2.7 Cell migration experiment
Periosteal stem cells were plated into the upper chamber of a 24-well Transwell™ plate at a density of 20,000 cells/well. HUCMSCsEmp -conditioned medium and HUCMSCWnt [Citation10]b-conditioned medium were added to the lower chamber. After culture for 24 hours, the cells on the bottom surface of each Transwell™ were fixed with 4% paraformaldehyde and stained with crystal violet, then the number of cells that had migrated was observed under a microscope.
2.8 Alkaline phosphatase (ALP) and alizarin red (ARS) staining
Periosteal stem cells were seeded into 6-well plates, and when the cell density reached 80–90%, they were changed to culture in osteogenic differentiation medium containing different treatments (control group and HUCMSCWnt [Citation10]b group). After 14 days, the cells were fixed and an ALP staining kit was used to detect ALP activity. ARS staining was performed after 28 days of treatment to detect mineralization, and the staining was observed and analyzed using Image J (NIH, Bethesda, MD, USA).
2.9 Real-time polymerase chain reaction (RT-PCR)
Total RNA was extracted by the TRIzol method, and cDNA was synthesized by reverse transcription using a high-capacity cDNA reverse transcription kit (Applied Biosystems, Foster City, CA, USA). A PCR machine was used for RT-PCR detection. Expression levels were normalized to GAPDH which was used as the internal reference. The 2−ΔΔCT method was used to analyze the relative expression levels of each target gene. The primer sequences used in this study were as follows: ALP (Forward: 5ʹ-GGGGACATGCAGTATGAATT-3ʹ, Reverse: 5ʹ- GGCCTGGTAGTTGTTGTGAG-3ʹ); RUNX2 (Forward: 5ʹ- CAGACCAGCAGCACTCCATA-3ʹ, Reverse: 5ʹ- AGACTCATCCATTCTGCCGC-3ʹ); Osx (Forward: 5ʹ- CCCACCCTTCCCTCACTCAT-3ʹ, Reverse: 5ʹ-CCTTGTACCACGAGCCATAGG-3ʹ), GAPDH (Forward: 5ʹ- ACCACGAGAAATATGACAACTCCC-3ʹ, Reverse: 5ʹ- CCAAAGTTGTCATGGATGACC-3ʹ).
2.10 Western blot assay
For total protein extraction, cells were lysed in RIPA buffer (Thermo Fisher Scientific, Waltham, MA, USA) supplemented with 1% Protease inhibitor on ice. Protein concentration was determined by the BCA assay (Sigma-Aldrich, St Louis, MO, USA). Next, proteins were subjected to sodium dodecyl sulfate polyacrylamide gel electrophoresis (SDS-PAGE) and transferred to a 0.2 μm polyvinylidene difluoride membrane. The membranes were blocked with 5% skimmed milk and incubated with the primary antibodies anti-RUNX2 (Abcam, Cambridge, MA, USA ab236639) and anti-OSTERIX (Abcam, ab209484) overnight at 4°C. Glyceraldehyde 3-phosphate dehydrogenase (GAPDH, Abcam, ab8245) was used as an internal reference. Next day, secondary antibodies against the species of primary antibodies were added and incubated for 1 hour at room temperature. Signals on the membrane were detected by electrochemiluminescence (ECL, Pierce, Rockford, IL, USA), and the protein band density of immune complexes was analyzed using AlphaEaseFC (Alpha Innotech Corporation, San Leandro, CA, USA) software.
2.11 Angiogenesis experiment
Vascular matrix gel was added to a 96-well plate on ice at a volume of 50 μL per well, then the 96-well plate was placed at 37°C for 1 hour to allow gelation. Then human umbilical vein endothelial cells (HUVECs) were added at a density of 20,000 cells/well, and the plate was placed in a 37°C, 5% CO2 tissue culture incubator. After 4 hours, angiogenesis was observed under a light microscope, and the number of lumens and branch points formed was counted.
2.12 Statistical analysis
Descriptive statistical results are expressed in the form of mean ± SEM. All statistical analysis and mapping were performed with GraphPad Prism 8.0. Groups were compared using t-test (paired or unpaired as appropriate), or one-way or two-way analysis of variance (repeated measurements as appropriate) and Bonferroni correction was used for analysis to adjust for multiplicity. P values of less than 0.05 were considered statistically significant.
3 RESULTS
The objective of this study was to evaluate the effect of HUCMSCsWnt [Citation10]b on fracture healing and investigate the underlying mechanism, we first established a rat model of femoral fracture then treated the fracture with saline, hydrogel, hydrogel with HUCMSCsEmp, hydrogel with HUCMSCsWnt [Citation10]b or hydrogel with HUCMSCsWnt [Citation10]b + IWR-1. Subsequently, micro-CT analysis, three-point bending tests and histological analysis were performed to evaluate the therapeutic effect of HUCMSCsWnt [Citation10]b. Lastly, we verified the effect of HUCMSCsWnt [Citation10]b conditioned medium on the PSCs and HUVECs in vitro and concluded that HUCMSCsWnt [Citation10]b significantly promoted fracture healing by accelerating the turnover of cartilage to bone.
3.1 HUCMSCsWnt [Citation10]b accelerated fracture healing in a femoral fracture rat model
We incorporated HUCMSCsEmp and HUCMSCsWnt [Citation10]b into a commercially-available hydrogel to provide a physically steady environment for the cells. Next, we injected the cell–hydrogel complex into the fracture site, and then micro-CT scanning was performed at 1, 2, 4 and 6 weeks post-surgery. The results showed that the calluses at the fracture site were still obvious after 4 weeks in the veh- and gel-treated groups. However, in the HUCMSCsEmp treatment group, the callus was smaller than in the former two groups, but the fracture site was still clearly visible. Notably, in the HUCMSCsWnt [Citation10]b treatment group, the callus had almost disappeared, leaving an almost nearly normal femur and a significantly better fracture healing score (). The effects were significantly reduced by the addition of IWR-1, a Wnt signaling pathway inhibitor (). Regarding the bone volume fraction, the veh- and gel-treated groups had similar bone volume fraction at the 4 w time-point: 42 ± 0.26% and 40 ± 0.33%, respectively; both lower than the HUCMSCsEmp treatment group (49 ± 0.28%). However, the bone volume in the HUCMSCsWnt [Citation10]b treatment group was 17 ± 0.24% and 10 ± 0.17% higher than in the veh and HUCMSCsEmp groups, respectively, and these effects were abolished by the treatment with IWR-1 (). These results suggested that HUCMSCsWnt [Citation10]b promoted fracture healing via enhanced Wnt signaling.
Figure 1. HUCMSCsWnt10b promoted the healing of a femoral fracture in a rat model. (a) Rat femoral fracture was treated with veh, hydrogel, hydrogel with HUCMSCsEmp, hydrogel with HUCMSCsWnt10b or hydrogel with HUCMSCsWnt10b + IWR-1 (the Wnt signaling pathway inhibitor), then femurs were harvested at 1, 2, 4 and 6 weeks after surgery. Three-dimensional reconstruction showed the healing of the fracture. (b) Fracture healing scores were quantified based on micro-CT images at 4 weeks after surgery. (c) Micro-CT analysis of the BV/TV of the fracture area at 1, 2, 4 and 6 weeks after surgery (n = 8, mean ± SEM, *: P < 0.05, **: P < 0.01, ***: P < 0.001, Student’s t test was used for statistical analysis).
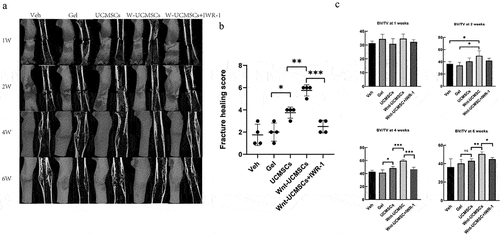
3.2 HUCMSCsWnt [Citation10]b improved the mechanical properties of the repaired femur
As mechanical properties are a key indicator of long bone fracture healing, the femurs were collected at 4 weeks after surgery and subjected to a three-point bending test. As can be seen from the results, max-load, max-stress and elastic modulus in the femurs did not differ significantly between the hydrogel-treated groups and the HUCMSCsEmp-treated group, while in the HUCMSCsWnt [Citation10]b-treated group, the max-load, max-stress and elastic modulus increased by 70%, 93% and 50% respectively, compared with the two groups, which proved that the mechanical properties of the healed femoral fracture in the HUCMSCsWnt [Citation10]b treatment group were significantly better than those in the other groups ().
Figure 2. The UCMSCWnt10b treatment group exhibited better mechanical properties of the healing femoral fracture. (A, B and C) The maximum load, maximum stress and elastic modulus at failure of the repaired fracture were calculated from the load and displacement curves (n = 8, mean ± SEM, *: P < 0.05, **: P < 0.01, Student’s t test was used for statistical analysis).
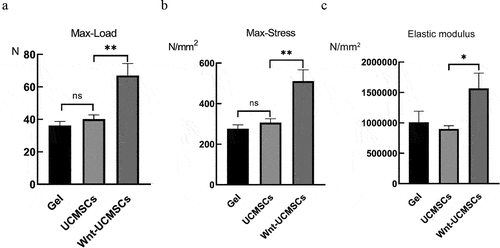
3.3 HUCMSCsWnt [Citation10]b induced accelerated turnover of cartilage callus to hard callus
In order to clarify the possible mechanism of action of HUCMSCsWnt [Citation10]b on fracture healing, we examined the callus by histology. Interestingly, we found similar percentages of cartilage callus at 2 weeks in the HUCMSCsWnt [Citation10]b-treated rats and the controls (), but significantly more bone formation in HUCMSCsWnt [Citation10]b treated callus at 4 weeks, indicating an accelerated turnover of cartilage callus to hard callus (). This could be due to enhancement of the osteogenic differentiation, as much stronger OCN staining was also seen in the HUCMSCsWnt [Citation10]b treatment group (). Furthermore, these effects were largely abolished by IWR-1 (). In summary, the effects of HUCMSCsWnt [Citation10]b on fracture healing may be due to accelerated cartilage remodeling and hard callus formation.
Figure 3. Histological analysis of femurs during fracture healing. (a) Sections were stained with safranin O–fast green and labeled for cartilage (red) and mineralized tissue (green) at 2 and 4 weeks after surgery. (b) Based on histology, cartilage callus volume/tissue volume was assessed using Image J at 2 weeks after surgery. (c) Immunohistochemistry was used to identify the expression of OCN in the callus of the fracture site at 2 weeks after surgery. On the right is an enlarged image of the area indicated by the arrow, which is the interface between the bone and the callus. (d) The area of positive OCN staining was analyzed using Image J. (n = 3, mean ± SEM, ns: not significant, *: P < 0.05, **: P < 0.01, ***: P < 0.001, Student’s t test, results are representative of at least three independent experiments, scale bar = 1 mm (left picture), scale bar = 200 μm (zoom in image on the right picture)).
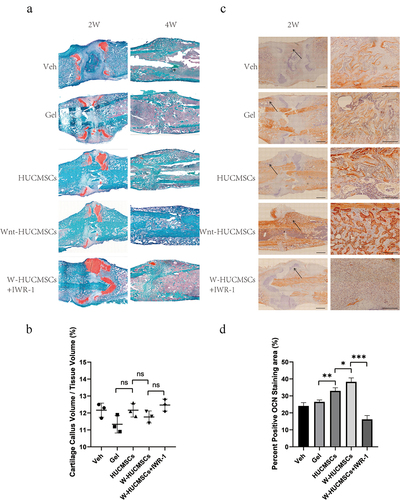
3.4 HUCMSCsWnt [Citation10]b promoted the migration and osteogenic differentiation of PSCs in vitro
As PSCs constitute most of the osteoblast lineage cells in the fracture callus, we next explored the modulating effects of HUCMSCsWnt [Citation10]b on PSCs so as to understand the cellular mechanism. We performed Transwell™ assays, and found that compared with the HUCMSCsEmp-treated group, the HUCMSCsWnt [Citation10]b-treated group significantly promoted the migration of PSCs when conditioned medium from HUCMSCsWnt [Citation10]b was added to the lower chambers of a Transwell™ plate (). Next, ALP staining and APS staining were performed to study whether HUCMSCsWnt [Citation10]b conditioned medium modulated the osteogenic differentiation ability of PSCs. As can be seen from the results, HUCMSCsWnt [Citation10]b conditioned medium significantly enhanced both ALP activity () and ARS staining (). Moreover, RT-PCR revealed a significant increase in ALP, Runx2 and Osterix gene expression in HUCMSCsWnt [Citation10]b-treated PSCs (). To further confirm this change at the protein level, total protein was collected and western blot analysis was performed. The results showed that, compared with the control group, the expression of Runx2 and Osterix protein in HUCMSCsWnt [Citation10]b-treated PSCs were significantly increased (). In summary, HUCMSCsWnt [Citation10]b enhanced both migration and osteogenic differentiation ability of PSCs, which is a possible mechanism underlying the accelerated hard callus formation and thus promoting fracture healing.
Figure 4. HUCMSCsWnt10b enhanced the migration and osteogenic differentiation of periosteal stem cells (PSCs). (a) The migration ability of PSCs was assessed by Transwell™ assay. PSCs were seeded into the upper chambers of Transwell™ at 2,000 cells/mL, then HUCMSCsEmp-conditioned medium or HUCMSCsWnt10b-conditioned medium was added to the lower chamber. After 24 hours, cells on the lower surface of the Transwell™ membrane were stained with crystal violet. The number of colonies was quantified using Image J (n = 3, mean ± SEM, **: P < 0.01, by Student’s t test, results are representative of at least three independent experiments, scale bar = 200 μm). (b) PSCs were treated with HUCMSCsEmp-conditioned medium or HUCMSCsWnt10b-conditioned medium and stained for alkaline phosphatase (ALP) at 7 days. The area of ALP staining was analyzed using ImageJ. (scale bar = 0.5 μm). (c) PSCs were treated with HUCMSCsEmp-conditioned medium or HUCMSCsWnt10b-conditioned medium, then used for alizarin red (ARS) staining at 28 days. Plate view (top) and microscopic view (bottom) of ARS staining are shown. In addition, the area of mineralization was measured using ImageJ. (scale bar = 0.5 μm (top picture), scale bar = 200 μm (bottom picture)). (d) Expression of the osteogenic genes ALP, RUNX2 and Osterix was assessed by RT-PCR. (e) The expression of osteogenic-related proteins (RUNX2 and Osterix) in PSCs was detected by western blotting. (n = 3, mean ± SEM, *: P < 0.05, **: P < 0.01, by Student’s t test, results are representative of at least three independent experiments).
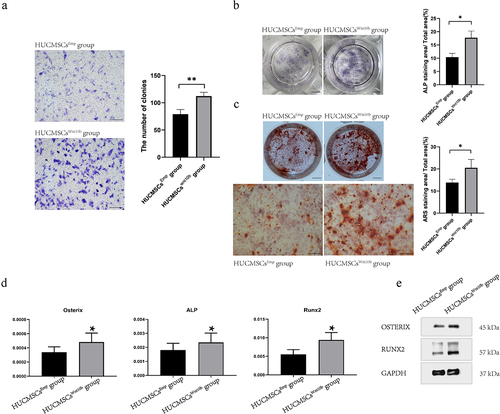
3.5 HUCMSCsWnt [Citation10]b promoted angiogenesis and migration of HUVECs
Vessel growth plays a vital role in the turnover of cartilage to bone both in the growth plate during development and in fracture healing. We previously found that HUCMSCsWnt [Citation10]b enhanced angiogenesis, so to evaluate the involvement of this activity in fracture healing we performed CD31 immunostaining for blood vessels at 2 weeks after surgery. The results showed that CD31-positive areas were significantly increased in the callus of HUCMSCsWnt [Citation10]b-treated rats compared with the other groups (). In addition, the number of CD31+ vessels in the total area was obviously higher in the HUCMSCsWnt [Citation10]b treatment group (0.18 ± 0.03) compared to the HUCMSCsEmp treatment group (0.14 ± 0.08) (). Additionally, these effects were significantly inhibited by IWR-1 (). Next, we verified the effect of HUCMSCsWnt [Citation10]b on the migration of HUVECs using the Transwell™ assay as described above and found that again, HUCMSCsWnt [Citation10]b promoted the migration of HUVECs (). Furthermore, tube formation assay of HUVECs showed that HUCMSCsWnt [Citation10]b-conditioned medium significantly improved the angiogenic ability of HUVECs (). The number of tubes (41.33 ± 1.85) and branch points (76.00 ± 2.44) in the HUCMSCsWnt [Citation10]b group were both obviously higher than in the HUCMSCsEmp group (29.33 ± 2.74 tubes and 21.00 ± 2.94 branch points) or any other groups (). These effects were abolished after the addition of SU5416, an angiogenesis inhibitor (). Combining those in vivo and in vitro data, we hypothesized that HUCMSCsWnt [Citation10]b enhance vessel growth to promote the turnover of cartilage to bone, which is one important mechanism for accelerated fracture healing.
Figure 5. HUCMSCsWnt10b promoted the migration and angiogenesis of HUVECs. (a) Immunohistochemistry was used to identify the expression of CD31 in the callus of the fracture site at 2 weeks after surgery. (b) The number of CD31+ vessels in the total area was analyzed using Image J (n = 8, the red arrows indicate the CD31+ blood vessels, scale bar = 200 μm). (c) Transwell™ assay was performed to analyze the migration ability of the HUVECs. HUVECs were seeded into the upper chambers at 2,000 cells/mL, and HUCMSCsEmp-conditioned medium or HUCMSCsWnt10b-conditioned medium was added to the lower chambers. After 24 hours, cells on the lower surface of the Transwell™membrane were stained with crystal violet. The number of colonies was quantified using Image J (n = 3, mean ± SEM, ***: P < 0.001, by Student’s t test, results are representative of at least three independent experiments, scale bar = 200 μm). (d) HUVECs were seeded into a 96-well plate and treated with growth medium, HUCMSCs-CM, Wnt-HUCMSCs-CM or Wnt-HUCMSCs+SU5416 (an angiogenesis inhibitor). Four hours later, pictures were taken under a microscope. (e, f) The number of tubes (e) and branch points (f) were counted (n = 3, mean ± SEM, **: P < 0.01, ****: P < 0.0001, by Student’s t test, results are representative of at least three independent experiments, scale bar = 100 μm).
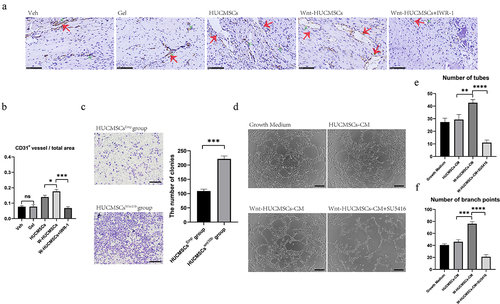
4 Discussion
Implantation of stem cells at the injury site is considered to be an effective method of promoting tissue regeneration [Citation18,Citation19]. They can not only directly differentiate and replace the damaged tissue [Citation20], but also regulate endogenous tissue repair, such as by secreting bioactive factors to activate local stem cells, thereby indirectly promoting tissue regeneration [Citation21,Citation22]. Because Wnt is a factor that promotes osteogenesis and angiogenesis, we generated HUCMSCsWnt [Citation10]b, hoping to achieve dual effects by both the secretion of Wnt and the provision of a source of stem cells to promote fracture healing.
In this study, we found that, compared with HUCMSCsEmp, HUCMSCsWnt [Citation10]b significantly promoted fracture healing, especially by increasing the bone mass in the callus of the fractured site. The known effects of Wnt on osteogenesis and the marked difference between HUCMSCsEmp and HUCMSCsWnt [Citation10]b suggest that these therapeutic effects are caused by the Wnt10b protein secreted by HUCMSCsWnt [Citation10]b acting on the endogenous stem cells of the femur, such as PSCs, rather than the osteogenic differentiation of HUCMSCsWnt [Citation10]b themselves [Citation23]. The healing of long bone fractures is a complex physiological process, which can be divided into three main stages: (1) formation of inflammatory hematoma after fracture injury; (2) activation and differentiation of bone stem/progenitor cells in the periosteum into chondrocytes to form cartilage callus, and with the growth of blood vessels, reconstructed the cartilage callus into a hard callus rich in osteoblast lineage cells; (3) activation of osteoclasts to reconstruct the callus and repair the fracture [Citation3]. During fracture healing, the callus provides initial stability for the fractured site, and instability at the fracture site seems to be a major risk for development of nonunion [Citation24]. Through the three-point bending tests, we found that HUCMSCsWnt [Citation10]b significantly enhanced the mechanical properties of the fracture callus, possibly because they enhanced the migration and osteogenic differentiation of PSCs, and accelerated callus formation. Therefore, HUCMSCsWnt [Citation10]b may be a precise target for the treatment of fracture nonunion.
Blood vessels allow the transport of cells, oxygen, nutrients, and waste, but also provide so-called vascular secretion signals that control organ growth and homeostasis [Citation25]. During fracture healing, blood vessels play an important role in the formation of inflammatory hematoma and the transformation of cartilage callus into hard callus [Citation26,Citation27]. Consequently, angiogenesis disorder is one of the important causes of delayed healing or fracture nonunion [Citation28]. Recently, it has been reported that activation of the Wnt/β-catenin pathway in vascular endothelial cells promotes the expression of vascular endothelial cell adhesion protein VE-cadherin, thereby promoting neovascularization [Citation29]. In our study, we observed an increase of CD31+ blood vessels in the HUCMSCsWnt [Citation10]b treatment group, and cytological experiments also showed that HUCMSCsWnt [Citation10]b conditioned medium had a stronger effect in promoting vascular endothelial growth factor (VEGF)-mediated angiogenesis of HUVECs. These results indicated that HUCMSCsWnt [Citation10]b promoted endothelial cell regeneration and angiogenesis, and thus promoted fracture healing. However, although HUCMSCsWnt [Citation10]b can promote VEGF-mediated angiogenesis, exactly how they activate and regulate the VEGF pathway needs to be further elucidated.
5 Conclusion
In summary, our study demonstrates that HUCMSCsWnt [Citation10]b promote fracture healing by accelerating hard callus formation, possibly by enhancing the osteogenic differentiation of PSCs and increasing blood vessel growth. These results suggest that implantation of HUCMSCsWnt [Citation10]b may be a promising treatment for fractures.
Supplemental Material
Download TIFF Image (4.9 MB)Disclosure statement
No potential conflict of interest was reported by the author(s).
Supplementary material
Supplemental data for this article can be accessed here
Correction Statement
This article has been republished with minor changes. These changes do not impact the academic content of the article.
Additional information
Funding
References
- CHEN W, LV H, S LIU, et al. National incidence of traumatic fractures in China: a retrospective survey of 512 187 individuals [J]. The Lancet. Global Health. 2017;5(8):e807–e17.
- Kostenuik P, M MIRZAF. Fracture healing physiology and the quest for therapies for delayed healing and nonunion [J]. J Orthop Res. 2017;35(2):213–223.
- A EINHORNT, C GERSTENFELDL. Fracture healing: mechanisms and interventions [J]. Nat Rev Rheumatol. 2015;11(1):45–54.
- D HANKENSONK, Gagne K, Shaughnessy M. Extracellular signaling molecules to promote fracture healing and bone regeneration [J]. Adv Drug Deliv Rev. 2015;94(3):3–12.
- Lanske B, Chandler H, PIERCE A, et al. Abaloparatide, a PTH receptor agonist with homology to PTHrP, enhances callus bridging and biomechanical properties in rats with femoral fracture [J]. J Orthop Res. 2019;37(4):812–820.
- R HIXONK, A MCKENZIEJ, W SYKESDA, et al. Ablation of proliferating osteoblast lineage cells after fracture leads to atrophic nonunion in a mouse model [J]. J Bone Miner Res. 2021;36(11):2243–2257.
- WANG C, YING J, X NIE, et al. Targeting angiogenesis for fracture nonunion treatment in inflammatory disease [J]. Bone Res. 2021;9(1):29.
- WANG T, ZHANG X, D BIKLED. Osteogenic differentiation of periosteal cells during fracture healing [J]. J Cell Physiol. 2017;232(5):913–921.
- Debnath S, R YALLOWITZA, Mccormick J, et al. Discovery of a periosteal stem cell mediating intramembranous bone formation [J]. Nature. 2018;562(7725):133–139.
- WANG L, J TOWERR, Chandra A, et al. Periosteal mesenchymal progenitor dysfunction and extraskeletally-derived fibrosis contribute to atrophic fracture nonunion [J]. J Bone Miner Res. 2019;34(3):520–532.
- Majidinia M, Sadeghpour A, Yousefi B. The roles of signaling pathways in bone repair and regeneration [J]. J Cell Physiol. 2018;233(4):2937–2948.
- HUANG P, R YAN, ZHANG X, et al. Activating Wnt/beta-catenin signaling pathway for disease therapy: challenges and opportunities [J]. Pharmacol Ther. 2019;196(79):79–90.
- Sarahrudi K, Thomas A, Albrecht C, et al. Strongly enhanced levels of sclerostin during human fracture healing [J]. J Orthop Res. 2012;30(10):1549–1555.
- Y LIU, FANG J, ZHANG Q, et al. Wnt10b-overexpressing umbilical cord mesenchymal stem cells promote critical size rat calvarial defect healing by enhanced osteogenesis and VEGF-mediated angiogenesis [J]. J Orthop Translat. 2020;23:29–37.
- HUANG Q, Y ZOU, C ARNOM, et al. Hydrogel scaffolds for differentiation of adipose-derived stem cells [J]. Chem Soc Rev. 2017;46(20):6255–6275.
- Leppanen O, Sievanen H, Jokihaara J, et al. Three-point bending of rat femur in the mediolateral direction: introduction and validation of a novel biomechanical testing protocol [J]. J Bone Miner Res. 2006;21(8):520–532.
- CHEN W, Y SUN, GU X, et al. Conditioned medium of human bone marrow-derived stem cells promotes tendon-bone healing of the rotator cuff in a rat model [J]. Biomaterials. 2021;271(120714):120714.
- P WONGS, E ROWLEYJ, N REDPATHA, et al. Pericytes, mesenchymal stem cells and their contributions to tissue repair [J]. Pharmacol Ther. 2015;151:107–120.
- ZHANG Y, Z HAO, WANG P, et al. Exosomes from human umbilical cord mesenchymal stem cells enhance fracture healing through HIF-1alpha-mediated promotion of angiogenesis in a rat model of stabilized fracture [J]. Cell Prolif. 2019;52(2):e12570.
- G WALMSLEYG, C RANSOMR, R ZIELINSE, et al. Stem Cells in Bone Regeneration [J]. Stem Cell Rev Rep. 2016;12(5):524–529.
- Bagno L, E HATZISTERGOSK, Balkan W, et al. Mesenchymal stem cell-based therapy for cardiovascular disease: progress and challenges [J]. Mol Ther. 2018;26(7):524–529.
- G PHINNEYD, F PITTENGERM. Concise review: MSC-derived exosomes for cell-free therapy [J]. Stem Cells. 2017;35(4):851–858.
- Wilk K, A YEHS, J MORTENSENL, et al. Postnatal calvarial skeletal stem cells expressing PRX1 reside exclusively in the calvarial sutures and are required for bone regeneration [J]. Stem Cell Reports. 2017;8(4):933–946.
- Rupp M, Biehl C, Budak M, et al. Diaphyseal long bone nonunions - types, aetiology, economics, and treatment recommendations [J]. Int Orthop. 2018;42(2):247–258.
- P KUSUMBEA, K RAMASAMYS, H ADAMSR. Coupling of angiogenesis and osteogenesis by a specific vessel subtype in bone [J]. Nature. 2014;507(7492):323–328.
- Peng Y, WU S, LI Y, et al. Type H blood vessels in bone modeling and remodeling [J]. Theranostics. 2020;10(1):426–436.
- H LIUJ, Yue T, W LUOZ, et al. Akkermansia muciniphila promotes type H vessel formation and bone fracture healing by reducing gut permeability and inflammation [J]. Dis Model Mech. 2020;13(11). DOI:10.1242/dmm.043620.
- C LIMJ, I KOK, Mattos M, et al. TNFalpha contributes to diabetes impaired angiogenesis in fracture healing [J]. Bone. 2017;99(26):26–38.
- Hubner K, Cabochette P, Dieguez-hurtado R, et al. Wnt/beta-catenin signaling regulates VE-cadherin-mediated anastomosis of brain capillaries by counteracting S1pr1 signaling [J]. Nat Commun. 2018;9(1):4860.