ABSTRACT
Mounting evidence supports that angiotensin-converting enzyme 2 (ACE2) may exert a vital function in multiple complications induced by diabetes. The aim of this research was to verify the function of ACE2 in diabetic angiopathy (DA). In our study, it was revealed that high glucose (HG) treatment impeded cell proliferation and induced cell apoptosis. Moreover, ACE2 level was reduced in HG-stimulated HMEC-1 cells. Functional assays demonstrated that ACE2 addition promoted cell viability, suppressed apoptosis, oxidative stress, ROS generation, and inflammation in HG-stimulated HMEC-1 cells. Furthermore, the activation of the JAK2/STAT3 pathway induced by HG was impeded by overexpression of ACE2. Besides, JAK2/STAT3 pathway inhibitor AG490 reversed the changes of cell viability, apoptosis, oxidative stress, and inflammation caused by ACE2 deletion in HG-treated HMEC-1 cells. In sum, our findings highlighted that ACE2 promoted the viability and restrained the oxidative stress, inflammation, and apoptosis in HG-induced microvascular endothelial cells (VECs) injury via regulating the JAK2/STAT3 pathway, suggesting ACE2 might be a potential therapeutic target for DA treatment.
Graphical Abstract
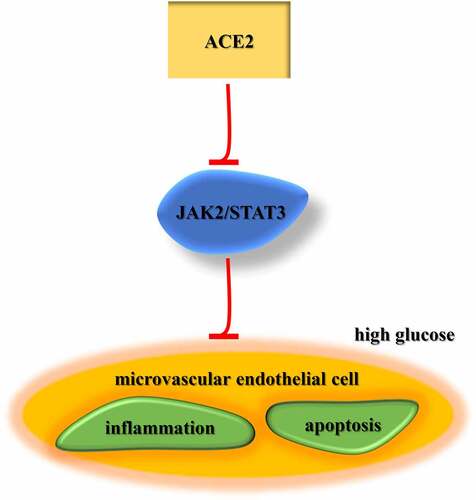
Highlights
ACE2 expression is reduced in DA model in vitro.
ACE2 inhibits apoptosis, oxidative stress, and inflammation in HG-treated HMEC- 1 cells.
ACE2 protects HMEC-1 against HG-triggered injury via the JAK2/STAT3 signaling.
Introduction
Diabetes is a kind of lifelong metabolic disease caused by multiple etiologies, which is featured by a high level of blood sugar over a prolonged period [Citation1]. Diabetic angiopathy (DA) is the main cause of morbidity and mortality in patients with diabetes mellitus [Citation2]. Vascular endothelial cells (VECs) are a monolayer of epithelial cells, which play an essential role in maintaining vascular function [Citation3]. Increasing evidence has indicated that vascular endothelial dysfunction is involved in the development of DA [Citation4]. Hyperglycemia can damage the function of VECs through various mechanisms, such as proliferation, apoptosis, and inflammation [Citation5]. The increased VECs apoptosis can result in various chain reactions, such as the release of pro-inflammatory cytokines, cytochrome c and other substances, which activate the inflammatory response and exacerbate vascular injury [Citation6,Citation7]. However, the molecular mechanism underlying DA pathogenesis remain to be elucidated.
Angiotensin-converting enzyme 2 (ACE2) is a negative regulator of the renin-angiotensin system and participates in the modulation of vascular function by converting angiotensin II (Ang II) to Ang- [Citation1–9]. Previous studies have reported that ACE2 is associated with multiple complications induced by diabetes. For instance, Wong et al elaborated that reduced ACE2 expression expedited diabetic kidney injury [Citation10]. Additionally, Duan et al exhibited that deletion of ACE2 exacerbated diabetic retinopathy through facilitating bone marrow dysfunction [Citation11]. Nonetheless, the role and function of ACE2 in the pathogenesis of DA are unclear.
In the current study, we hypothesized that ACE2 might be involved in the progression of DA. This study aimed to investigate the role of ACE2 in the pathogenesis of DA using an in vitro DA model. Our findings may help to throw light upon the complex mechanism of DA and provide a new approach for DA treatment.
Materials and methods
Cell culture
Human microvascular endothelial cells (HMEC-1) were provided by ATCC and cultured in an endothelial cell medium containing 10% FBS (Gibco), 1 mg/ml hydrocortisone (Sigma), and 1% penicillin/streptomycin (Gibco) at 37°C with 5% CO2. Cells cultured with 5.5 mM glucose (NG) for 48 h were used as controls and cells treated with HG (25 mM) for 48 h were used to establish in vitro DA model according to the previous report [Citation12].
Cell transfection
pcDNA3.1/ACE2 and its control (pcDNA3.1), and small interfering RNA targeting ACE2 (si-ACE2) and its control (si-NC) were synthesized by Sangon Biotech (Shanghai, China) and transfected into HMEC-1 by Lipofectamine 2000 (Invitrogen). Subsequent experiments were performed 48 h after transfection.
MTT assay
Cell viability under HG conditions was determined using the MTT assay. HMEC-1 (1 × 104 cells/well) cells were plated in a 96-well plate. After treatment under HG for 48 h, MTT (5 mg/ml) solution was added to each well for 4 h. DMSO was then added to dissolve the formazan crystals, and the absorption at 450 nm was detected using a microplate reader (Bio-Rad).
Cell apoptosis
Cell apoptosis was analyzed by flow cytometry using the FITC conjugated Annexin V Apoptosis Detection Kit (BD Biosciences). Cells seeded on 6-well plate were digested by 0.25% trypsin. After centrifugation, cells were stained with annexin V-FITC and PI, and then analyzed by flow cytometer (BD Biosciences, USA).
Reverse transcription-quantitative PCR (RT-qPCR)
TRIzol (Invitrogen, USA) was used to extract total RNA, and reverse transcription was carried out using cDNA Reverse Transcription Kit (Takara, China). Then, qPCR was conducted with SYBR Premix Ex Taq™ (Takara). ACE2 level was calculated by 2−ΔΔCT method normalized to GAPDH. The primer sequences are as follows: ACE2 forward, 5ʹ-TCCATTGGTCTTCTGTCACCCG-3ʹ, reverse, 5ʹ-AGACCATCCACCTCCACTTCTC-3ʹ; GAPDH forward, 5ʹ-GTCTCCTCTGACTTCAACAGCG-3ʹ, reverse, 5ʹ-ACCACCCTGTTGCTGTAGCCAA-3ʹ.
Western blot
Total protein was extracted using radioimmunoprecipitation lysis buffer (Beyotime). The proteins (20 μg) were separated using 10% SDS-PAGE and transferred to PVDF membranes. Following blocking with 5% skimmed milk for 1 h at room temperature, the membranes were incubated overnight at 4°C with primary antibodies against: ACE2 (1:1000), Bax (1:1000), cleaved caspase-3 (1:1000), phosphorylated JAK2 (p-JAK2; 1:500), JAK2 (1:1000), p-STAT3 (1:1000), STAT3 (1:1000) and GAPDH (1:1000) overnight at 4°C, followed by incubation with a horseradish peroxidase-conjugated secondary antibody for additional 1 h. The protein bands were visualized with an ECL kit, and protein expression was quantified using Image-Pro® Plus software (version 6.0; Media Cybernetics)
Enzyme-linked immunosorbent assay (ELISA)
The concentrations of inflammatory cytokines IL-6, IL-1β, and TNF-a using the ELISA kit (Abcam) follow the manufacturer’s instructions.
Reactive oxygen species (ROS) detection
The ROS generation of cells was detected via an active oxygen detection kit (Beijing Solarbio Science & Technology). After treatment, HMEC-1 cells were washed with PBS and incubated with 10 µM dichlorodihydrofluorescein diacetate (DCFH-DA). The cells were washed twice with PBS, and fluorescence was detected using flow cytometry at an excitation wavelength of 480 nm and emission wavelength of 530 nm.
Measurement of antioxidant enzyme activity
The levels of superoxide dismutase (SOD) and malondialdehyde (MDA) in cell culture fluid were detected by different kits purchased from Jiancheng Bioengineering (Nanjing, China).
Statistical analysis
All experiments were performed at least three times and data are presented as mean ± SD. SPSS 22.0 was employed for statistical analysis. The differences between groups were evaluated via Student’s t-test or one-way ANOVA. P < 0.05 indicated significant in statistics.
Results
ACE2 expression is reduced in DA model in vitro
HMEC-1 cells possess similar characteristics to their in vivo counterparts [Citation13]. In addition, accumulating studies used HMEC-1 cells to investigate the pathogenesis of DA in vitro [Citation14–16]. Therefore, HG-induced HMEC-1 cells were employed to establish in vitro DA model. The results from MTT assay exhibited that HG impeded cell viability in a time dependence (). Then, flow cytometry assay elucidated that HG treatment elevated the apoptosis rate of HMEC-1 cells (). Moreover, western blotting implied that HG treatment elevated Bax and cleaved caspase-3 levels (). Besides, the mRNA and protein levels of ACE2 in HMEC-1 cells were detected following HG treatment. As displayed in dande, ACE2 expression was lower in HG group than that in NG group. These data implied that ACE2 might exert a crucial role in modulating the progression of DA.
Figure 1. ACE2 expression is reduced in DA model in vitro.(a) Cell viability was detected by MTT assay in HG-induced HMEC-1 cells at different time points. (b) Cell apoptosis was assessed by flow cytometry in HG-induced HMEC-1 cells. (c) Bax and cleaved caspase-3 protein expression was detected by western blotting in HG-induced HMEC-1 cells. (d and e) The expression level of ACE2 was determined by RT-qPCR and western blotting. *P < 0.05; **P < 0.01.
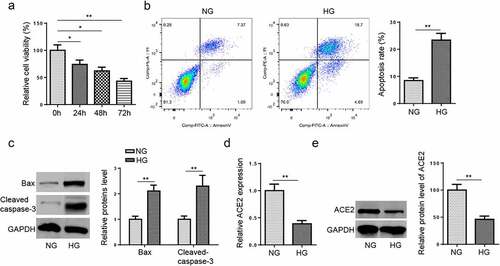
Overexpression of ACE2 attenuates apoptosis and oxidative stress in HG-treated HMEC-1 cells
To study the effect of ACE2 on HG-stimulated HMEC-1 cells damage, cells were transfected with pcDNA3.1/ACE2 or pcDNA3.1. RT-qPCR and western blot determined that ACE2 was overexpressed by ACE2 addition (aandb). Moreover, MTT assay exhibited that ACE2 addition expedited cell viability (), while reduced apoptosis in HG-stimulated HMEC-1 cells (). Consistently, the expression of cleaved caspase-3 and Bax were decreased in HMEC-1 cells after transfection of ACE2 overexpression plasmid (). Furthermore, the levels of ROS, MDA, and SOD were detected to reflect the oxidative stress [Citation17]. The results indicated that ACE2 overexpression mitigated HG-caused oxidative stress by downregulating ROS and MDA and promoting SOD activity (). These data indicated that ACE2 addition weakened HG-induced apoptosis and oxidative stress.
Figure 2. Overexpression of ACE2 attenuates apoptosis and oxidative stress in HG-treated HMEC-1 cells.(a and b) RT-qPCR and western blot showed ACE2 expression level in HMEC-1 cells treated with NG, HG, HG+pcDNA3.1 and HG+pcDNA3.1/ACE2. (c) Cell viability was detected by MTT assay in HMEC-1 cells treated with NG, HG, HG+pcDNA3.1 and HG+pcDNA3.1/ACE2. (d) Cell apoptosis was assessed by flow cytometry. (e) The protein levels of Bax and cleaved caspase-3 were detected by western blotting. (f-h) The ROS generation, MDA, and SOD expression levels were detected in treated HMEC-1 cells. *P < 0.05; **P < 0.01.
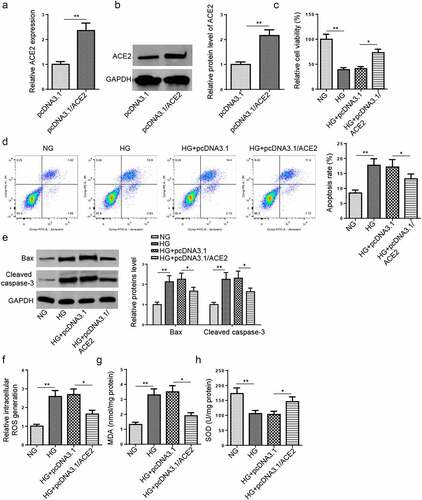
The addition of ACE2 inhibits the release of inflammatory factors in HG‑stimulated HMEC-1 cells
Hyperglycemia can cause functional damage to VECs through a variety of mechanisms, including inflammation [5]. Therefore, we assessed the effect of ACE2 on HG-induced inflammation. ELISA showed that HG treatment elevated the levels of inflammatory cytokines IL-6, IL-1β, and TNF-a, while overexpression of ACE2 reversed the above effects (). Collectively, all these results implied that ACE2 supplementation alleviated inflammation in HG-induced HMEC-1 cells.
ACE2 blocks HG-induced activation of JAK2/STAT3 pathway
Extensive evidence has elucidated that the JAK2/STAT3 pathway regulates multiple biological effects, including apoptosis, reticulum stress, ROS, and inflammation [Citation18–20]. Previous studies have displayed that HG contributes to the activation of the JAK2/STAT3 pathway [Citation21]. Besides, the pathway was reported to promote the development of type 2 diabetes mellitus by mediating inflammation associated with VECs [Citation22]. To explore whether ACE2 treatment could regulate the JAK2/STAT3 signaling pathway in HG-stimulated HMEC-1 cells, the effects of ACE2 addition on phosphorylation levels of JAK2 and STAT3 were assessed. As depicted in aandb, the results determined that HG distinctly upregulated p-JAK2 and p-STAT3 levels in HMEC-1 cells, while overexpression of ACE2 attenuated these changes. Taken together, the results elaborated that ACE2 inhibited HG-triggered activation of the JAK2/STAT3 pathway in HMEC-1 cells.
Deletion of ACE2 expedites the apoptosis and oxidative stress via the JAK2/STAT3 pathway in HG-treated HMEC-1 cells
To further analyze whether ACE2 regulated VECs injury via the JAK2/STAT3 signaling, AG490, a specific JAK2/STAT3 signaling inhibitor, was employed to impede the JAK2/STAT3 pathway. Then, si-ACE2 transfected HG-cultured HMEC-1 cells were treated with AG490. Western blotting indicated that ACE2 deficiency increased the protein levels of phosphorylated JAK2 and STAT3 in HG-treated HMEC-1 cells, while AG490 treatment abrogated this impact (). Meanwhile, AG490 abrogated the impacts of ACE2 knockdown on the proliferation and apoptosis of HG-cultured HMEC-1 cells (). Besides, AG490 restored the ACE2 knockdown-caused changes in ROS generation, and the levels of MDA, SOD, and inflammatory cytokines (). In sum, the above data elucidated ACE2 protected HMEC-1 against HG-triggered damage and inflammation via restraining the JAK2/STAT3 signaling.
Figure 5. Deletion of ACE2 expedites apoptosis and oxidative stress via the JAK2/STAT3 pathway in HG-treated HMEC-1 cells.(a) JAK2 and STAT3 phosphorylation levels in HG-treated HMEC-1 cells transfected with si-NC, si-ACE2 and si-ACE2+ AG490 were evaluated by western blotting.(b-d) Cell viability and apoptosis were assessed by MTT, flow cytometry, and western blot in HG-treated HMEC-1 cells. (e-j) The ROS generation, levels of MDA and SOD, and inflammatory cytokines (IL-6, IL-1β, and TNF-a) were measured in HG-treated HMEC-1 cells. *P < 0.05; **P < 0.01.
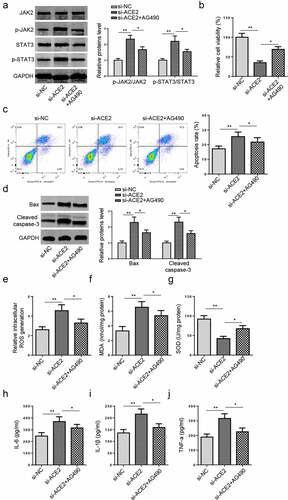
Discussion
DA includes micro-and macroangiopathy and is associated with high morbidity and mortality rate in patients with long-term diabetes [Citation23]. Previous researches elucidated that microvascular endothelial damage occurs in diabetic patients and animal models [Citation24]. Herein, cell proliferation, apoptosis, oxidative stress, and inflammation-associated factors were measured to evaluate the damage in HG-treated HMEC-1 cells. Our results suggested that ACE2 addition could accelerate cell viability, attenuate apoptosis, oxidative stress, and inflammatory response of HG-induced HMEC-1 cells through inactivating JAK2/STAT3 pathway.
It was reported that ACE2 was widely expressed in epithelial cells of multiple organs, such as lungs, heart, kidney, and pancreas. Moreover, ACE2 was found to act as essential regulars in various diseases, such as heart failure, hypertension, renal diseases, and diabetes [Citation25,Citation26]. For instance, ACE2 inhibited cardiomyocyte autophagy and alleviated doxorubicin-induced heart failure via the miR-30E/Beclin-1 signaling [Citation27]. ACE2 protected against hypoxic pulmonary hypertension by restraining the viability of pulmonary artery smooth muscle cells [Citation28]. Furthermore, ACE2 and Ang- [Citation1–7] have protective effects against diabetic retinopathy [Citation29]. Besides, ACE2 was downregulated in the hippocampus of diabetes and lncRNA OIP5-AS1 improved microangiopathy via modulating miR-200b/ACE2 axis [Citation30]. In the present study, ACE2 expression was reduced in HG-cultured HMEC-1 cells, and overexpression of ACE2 enhanced SOD expression and reduced MDA and ROS expression. Additionally, ACE2 addition expedited cell viability and reduced apoptosis and levels of inflammatory mediators in HMEC-1 cells following HG stimulation. To sum up, our data demonstrated that ACE2 could relieve HG-stimulated HMEC-1 cell inflammation and oxidative stress.
Previous studies have indicated that the JAK2/STAT3 pathway is associated with cell viability, migration, and inflammation [Citation31,Citation32], and executes essential functions in the progression of diabetes complications-related diseases. Sun et al implied that suppression of the JAK2/STAT3 signaling improved diabetic renal dysfunction and declined the inflammatory factor levels [Citation33]. Xiao et al determined that miR-19b inhibited SOCS6 to regulate apoptosis and inflammation in HG-treated human retinal VECs of diabetic retinopathy via the JAK2/STAT3 signaling [Citation34]. In this research, HG significantly elevated the phosphorylation levels of JAK2 and STAT3 in HMEC-1 cells, while the addition of ACE2 abrogated these impacts. Moreover, JAK2/STAT3 pathway inhibitor (AG490) reversed the changes of the viability, apoptosis, oxidation stress and inflammation caused by ACE2 deletion in HG-cultured HMEC-1 cells. These results manifested that ACE2 protected HMEC-1 against HG-induced injury through restraining the JAK2/STAT3 signaling.
Conclusions
Our discoveries illustrated that ACE2 restrained apoptosis, inflammation, and oxidative stress to protect against HG-triggered microvascular endothelial cell damage via inactivating the JAK2/STAT3 pathway. These findings might enrich the pathogenesis of DA and provide a theoretical basis for the further development of innovative therapeutic strategies for DA. In the future study, other inflammatory pathways, such as NF-κB and PI3K/AKT, should be further investigated in DA.
Disclosure statement
No potential conflict of interest was reported by the author(s).
Additional information
Funding
References
- Li Y, Yao Z, Yue W, et al. Reusable, non-invasive, and ultrafast radio frequency biosensor based on optimized integrated passive device fabrication process for quantitative detection of glucose levels. Sensors. 2020;20(6):1565.
- Hurlow JJ, Humphreys GJ, Bowling FL, et al. Diabetic foot infection: a critical complication. Int Wound J. 2018;15(5):814–821.
- Qiu Y, Zhang C, Zhang G, et al. Endothelial progenitor cells in cardiovascular diseases. Aging medicine. 2018;1(2):204–208
- Knapp M, Tu X, Wu R. Vascular endothelial dysfunction, a major mediator in diabetic cardiomyopathy. Acta Pharmacol Sin. 2019;40(1):1–8.
- Ding X, Yao W, Zhu J, et al. Resveratrol attenuates high glucose-induced vascular endothelial cell injury by activating the e2f3 pathway. Biomed Res Int. 2020;2020:6173618.
- Nakagami H, Kaneda Y, Ogihara T, et al. Endothelial dysfunction in hyperglycemia as a trigger of atherosclerosis. Curr Diabetes Rev. 2005;1(1):59–63.
- Eelen G, de Zeeuw P, Simons M, et al. Endothelial cell metabolism in normal and diseased vasculature. Circ Res. 2015;116(7):1231–1244.
- Pena Silva RA, Chu Y, Miller JD, et al. Impact of ACE2 deficiency and oxidative stress on cerebrovascular function with aging. Stroke. 2012;43(12):3358–3363.
- Lin Z, Chen Y, Zhang W, et al. RNA interference shows interactions between mouse brainstem angiotensin AT 1 receptors and angiotensin-converting enzyme 2. Exp Physiol. 2008;93(5):676–684.
- Wong DW, Oudit GY, Reich H, et al. Loss of angiotensin-converting enzyme-2 (Ace2) accelerates diabetic kidney injury. Am J Pathol. 2007;171:438–451.
- Duan Y, Beli E, Li Calzi S, et al. Loss of angiotensin-converting enzyme 2 exacerbates diabetic retinopathy by promoting bone marrow dysfunction. Stem Cells. 2018;36(9):1430–1440.
- Ye EA, Steinle JJ. miR-146a attenuates inflammatory pathways mediated by TLR4/NF-kappaB and TNFalpha to protect primary human retinal microvascular endothelial cells grown in high glucose. Mediators Inflamm. 2016;2016:3958453.
- Kamal K, Du W, Mills I, et al. Antiproliferative effect of elevated glucose in human microvascular endothelial cells. J Cell Biochem. 1998;71(4):491–501.
- Chen K, Zhao XL, Li LB, et al. miR-503/Apelin-12 mediates high glucose-induced microvascular endothelial cells injury via JNK and p38MAPK signaling pathway. Regener Ther. 2020;14:111–118.
- Xu F, Liu Y, Zhu X, et al. Protective effects and mechanisms of vaccarin on vascular endothelial dysfunction in diabetic angiopathy. Int J Mol Sci. 2019;20(18):4587.
- Lee CH, Shieh YS, Hsiao FC, et al. High glucose induces human endothelial dysfunction through an Axl-dependent mechanism. Cardiovasc Diabetol. 2014;13(1):53.
- Amin MM, Rafiei N, Poursafa P, et al. Association of benzene exposure with insulin resistance, SOD, and MDA as markers of oxidative stress in children and adolescents. Environ. Sci. Pollut. Res. Int. 2018;25(34):34046–34052
- Cui ZT, Liu JP, Wei WL. The effects of tanshinone IIA on hypoxia/reoxygenation-induced myocardial microvascular endothelial cell apoptosis in rats via the JAK2/STAT3 signaling pathway. Biomed Pharmacother. 2016;83:1116–1126.
- Wu L, Tan JL, Wang ZH, et al. ROS generated during early reperfusion contribute to intermittent hypobaric hypoxia-afforded cardioprotection against postischemia-induced Ca(2+) overload and contractile dysfunction via the JAK2/STAT3 pathway. J Mol Cell Cardiol. 2015;81:150–161.
- Li L, Li M, Li Y, et al. Exogenous H2S contributes to recovery of ischemic post-conditioning-induced cardioprotection by decrease of ROS level via down-regulation of NF-kappaB and JAK2-STAT3 pathways in the aging cardiomyocytes. Cell Biosci. 2016;6(1):26.
- Hu TY, Li LM, Pan YZ. CTRP3 inhibits high glucose-induced human glomerular mesangial cell dysfunction. J Cell Biochem. 2019;120(4):5729–5736.
- Yang M, Tian M, Zhang X, et al. Role of the JAK2/STAT3 signaling pathway in the pathogenesis of type 2 diabetes mellitus with macrovascular complications. Oncotarget. 2017;8(57):96958–96969.
- Cosentino F, Eto M, De Paolis P, et al. High glucose causes upregulation of cyclooxygenase-2 and alters prostanoid profile in human endothelial cells: role of protein kinase C and reactive oxygen species. Circulation. 2003;107(7):1017–1023.
- Lotfy M, Adeghate J, Kalasz H, et al. Chronic complications of diabetes mellitus: a mini review. Curr Diabetes Rev. 2017;13(1):3–10.
- Yan R, Zhang Y, Li Y, et al. Structural basis for the recognition of SARS-CoV-2 by full-length human ACE2. Science. 2020;367(6485):1444–1448.
- Patel VB, Zhong JC, Grant MB, et al. Role of the ACE2/Angiotensin 1-7 axis of the renin-angiotensin system in heart failure. Circ Res. 2016;118(8):1313–1326.
- Lai L, Chen J, Wang N, et al. MiRNA-30e mediated cardioprotection of ACE2 in rats with Doxorubicin-induced heart failure through inhibiting cardiomyocytes autophagy. Life Sci. 2017;169:69–75.
- Zhang R, Su H, Ma X, et al. MiRNA let-7b promotes the development of hypoxic pulmonary hypertension by targeting ACE2. Am J Physiol Lung Cell Mol Physiol. 2019;316(3):L547–L557.
- Verma A, Shan Z, Lei B, et al. ACE2 and Ang-(1-7) confer protection against development of diabetic retinopathy. Mol Ther. 2012;20(1):28–36.
- Xie W, Wu D, Ren Y, et al. OIP5-AS1 attenuates microangiopathy in diabetic mouse by regulating miR-200b/ACE2. World Neurosurg. 2020;139:e52–e60.
- Duan W, Yang Y, Yan J, et al. The effects of curcumin post-treatment against myocardial ischemia and reperfusion by activation of the JAK2/STAT3 signaling pathway. Basic Res Cardiol. 2012;107(3):263.
- Qi QR, Yang ZM. Regulation and function of signal transducer and activator of transcription 3. World J. Biol. Chem. 2014;5(2):231–239
- Sun M, Bu W, Li Y, et al. Danzhi Jiangtang Capsule ameliorates kidney injury via inhibition of the JAK-STAT signaling pathway and increased antioxidant capacity in STZ-induced diabetic nephropathy rats. Biosci Trends. 2019;12(6):595–604.
- Xiao F, Li L, Fu JS, et al. Regulation of the miR-19b-mediated SOCS6-JAK2/STAT3 pathway by lncRNA MEG3 is involved in high glucose-induced apoptosis in hRMECs. Biosci Rep. 2020;40(7). doi: 10.1042/BSR20194370.