ABSTRACT
This study aimed to determine the amount of expression of the ATP-binding cassette subfamily B member 1 (ABCB1) gene chip as a prospective diagnostic marker for acute myocardial infarction (AMI) in a wide population . In the AMI and control groups, 113 patients with AMI and 83 persons with non-coronary artery disease were selected for peripheral venous leukocyte collection. Western blot and real-time polymerase chain reaction (RT-PCR) were employed to detect relative ABCB1 expression in both groups. The results showed that the ABCB1 transcription and protein levels in the AMI group were higher than in the control. The relative mRNA expression of ABCB1 was 0.26 (0.03–0.79) in the AMI group and 0.13 (0.01–0.52) in the control group (P < 0.05). The expression of the ABCB1 gene at the protein level in the AMI group was 1.65 times that in the control (P < 0.05). Further, the subjects in the AMI group were older (P < 0.001), had lower levels of high-density lipoprotein cholesterol (P = 0.038), and had higher incidence of type II diabetes mellitus (P = 0.003) compared with the control. Logistic regression analysis showed that the expression of ABCB1 in peripheral blood was correlated with the occurrence of AMI (P = 0.003). High ABCB1 expression, type II diabetes, and advanced age were found to serve as potential independent risk factors for AMI, with a 4.88-fold, 2.99-fold, and 2.63-fold increased risk of AMI. Overall, the high expression of ABCB1 in peripheral blood might be related to the occurrence of AMI.
Graphical Abstract
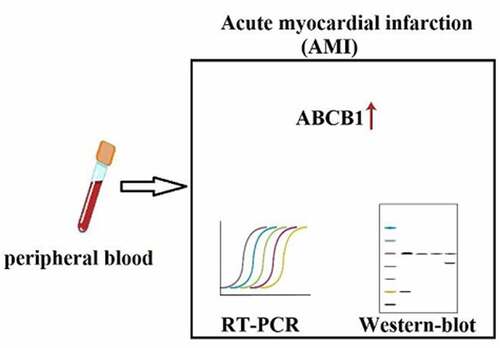
Highlights
The level of ABCB1 gene expression in patients with AMI was higher.
High expression of ABCB1 gene was an independent risk factor of AMI.
High expression of ABCB1 gene could serve as a potential biomarker of AMI.
1. Introduction
Coronary heart disease is a major reason contributing to increased morbidity and mortality around the globe [Citation1]. Most coronary heart disease cases can be attributed to the occurrence of acute myocardial infarction (AMI). Although AMI-related mortality has declined, its prevalence has increased dramatically, posing a significant public health burden around the world [Citation1]. AMI is myocardial ischemic necrosis caused by an acute interruption of myocardial blood flow. The pathogenesis of this phenomenon mostly involves the formation of acute thrombi on the ruptured atherosclerotic plaques. Myocardial infarction must be diagnosed with relevant clinical evidence of AMI, such as symptoms, changes in electrocardiography (ECG), examination of traditional cardiac markers, abnormal wall motion, and angiographic results. Acute chest pain needs to be diagnosed early for individuals observed under emergency conditions to save lives. However, AMI does not always have an association with chest pain, and ECG performance is not specific in up to 50% of cases, challenging AMI’s diagnosis [Citation2]. Thus, the development of accurate biomarkers for risk prediction is needed to manage the outbreak of AMI [Citation3]. The multi-biomarker approach can significantly improve AMI’s diagnostic accuracy and provide more relevant information for early risk stratification of AMI [Citation4].
Leukocytes in the peripheral blood can be potentially used as an evaluation window for cardiovascular diseases [Citation5]. In the leukocytes of individuals with AMI, dozens of genes have shown an altered expression during or after myocardial infarction [Citation6]. A few new biomarkers have been discovered for the diagnosis and risk assessment of AMI. For instance, reports indicated that formate might be a biomarker of acute coronary syndrome [Citation7]. For the development of stable coronary heart disease to AMI, high expression of suppressor of cytokine signaling 3 in the peripheral blood can serve as an independent risk factor [Citation8]. Another molecular level marker for early diagnosis of AMI might be the low expression of free fatty acid receptor 2 in the peripheral blood leukocytes [Citation9].
Our previous gene chip study suggested that the ABCB1 gene was more highly expressed in the AMI than in the control group. The latest study found that ABCB1 was highly expressed in the vascular endothelium of the neocortex in patients with drug-resistant epilepsy, and the expression of ABCB1 was regulated by epigenetic marker [Citation10].Nevertheless, few studies have investigated the role of ABCB1 in cardiovascular disease, especially in patients with AMI. ABCB1 may regulate coronary heart disease, and the expression of ABCB1 is closely related to inflammatory response and glucose metabolism. We hypothesized that patients with high ABCB1 expression in peripheral blood were more likely to develop AMI than those with low ABCB1 expression in a larger population. This study aims to expand the sample size, double judge whether ABCB1 expression is up-regulated in patients with AMI in a larger population at the gene and protein levels, and determine whether the relatively high expression of ABCB1 is related to the occurrence of AMI. This could help identify biomarkers for AMI early diagnosis.
2. Methods and subjects
2.1. Research subjects
This study was retrospective in nature. This study complied with the ethical principles of medical research on human subjects, elaborated in the Helsinki Declaration. The Ethics Committee of China-Japan Union Hospital of Jilin University approved the study. The test samples and sample information collection of all the research subjects were carried out after obtaining informed consent from all the participants. 113 individuals suffering from AMI were admitted to the hospital in the Department of Cardiology, China-Japan Union Hospital of Jilin University, China, and were selected as the AMI group. The AMI diagnosis follows the globally recognized definition of myocardial infarction elaborated by the European Society of Cardiology in 2017 [Citation11], thereby fulfilling the requirements of coronary angiography and confirming clear vascular disease. Severe stenosis and occlusion of the primary coronary arteries (the left main trunk and right main trunk) and the major branches (the anterior descending and circumflex arteries) were observed. For the AMI group, the exclusion criteria included were as follows: (1) myocardial infarction secondary to ischemic imbalance; (2) myocardial infarction when the serum biochemical markers, myoglobin, and troponin were not at hand; (3) myocardial infarction related to stent thrombosis and percutaneous coronary intervention; (4) myocardial infarction related to coronary artery bypass grafting.
In addition, 83 individuals with non-coronary heart diseases were selected as the control group. The control group inclusion criteria were hospitalization due to chest pain and angiography indicating <50% extent of coronary artery stenosis, whereas ECG revealed no pathological Q wave, T wave, and ST segments secondary changes. Individuals with intercostal neuritis, acute pneumonia, pleuritis, and other diseases were excluded from the control group.
The detailed clinical data, including age, history of hypertension, smoking, and diabetes; total cholesterol (TC), serum triglycerides (TG), low-density lipoprotein cholesterol (LDL-C), and high-density lipoprotein cholesterol (HDL-C) of all the research subjects were recorded.
2.2. Research methods
2.2.1. The overall process of the experiment
The patients were divided into two groups (experimental and control) according to the inclusion criteria. Part of the blood of each group was collected for RNA extraction, and then an real-time polymerase chain reaction (RT-PCR) experiment was performed, which was used for the validation at the gene level. Part of the blood was used for protein extraction, and a western-blot experiment was performed. Finally, the results of the gene and protein levels have been summarized.
2.2.2. Acquiring peripheral blood lymphocytes
Six milliliters of fasting peripheral blood from each research subject were extracted in the morning in an EDTA anticoagulation tube and stored at 4°C. AMI patients were subjected to blood collection before percutaneous coronary intervention in the experimental group. The patients in the control group were newly hospitalized for a physical examination to ensure that there was no prescription for injection and oral medicine on the first day. The extracted lymphocytes were separated within 4 h after collection using the reagent for peripheral blood lymphocyte separation(Stemcell Technologies, Vancouver, Canada). The detailed procedures used were as follows: The fresh anticoagulant blood sample was taken and mixed evenly, having an equal volume of 0.9% sodium chloride injection. The resultant solution was methodically added to an equivalent volume of peripheral blood lymphocyte separation medium and centrifuged for 20 min at 1600 g to obtain four different layers: plasma, lymphocyte, separation medium, and red blood cell layer [Citation12]. For the subsequent experiments, the lymphocyte layer was after that carefully aspirated.
2.2.3. cDNA synthesis of lymphocytes isolated from the peripheral blood samples
The total RNA of the isolated lymphocytes was extracted using the Blood Total RNA Kit purchased from Simgen Biological Reagent Development (Hangzhou, Zhejiang Province, China) by strictly following the manufacturer’s instructions to circumvent the possible contamination RNA degradation during the extraction process. The quality of the total obtained RNA was tested using polyacrylamide gel electrophoresis(1% agarose). The 18S and 28S rRNA bands were observed in the gel. 28S rRNA band was approximately two times as intense as the 18S rRNA. The concentrations and absorbance of the total RNA samples that met the standards were measured by a microplate reader. The reverse transcription was performed subsequently using the reverse transcription kit (a cDNA first-strand synthesis premix that uses the FastKing one-step method to remove genomic DNA, Tiangen Biochemical Technology, Beijing, China) as per the manufacturer’s instructions. The total RNA added to each sample was consistent with each other. The cDNA samples thus procured were stored for subsequent RT-PCR detection at −80°C
2.2.4. RT-PCR
Upon dilution of the obtained cDNA sample 20 times, PCR amplification was performed using the SYBR fluorescence quantification kit and a Taq qPCR synthesis premix purchased from Sangon Biotech (Shanghai, China) [Citation13]. The internal reference gene glyceraldehyde 3-phosphate dehydrogenase (GAPDH) was used, while ABCB1 was the target gene. All the samples were taken in triplicates, the experiment was repeated 3 times, and the average value was taken. The reaction conditions used were as follows: pre-denaturation at 95°C for 5 minutes; 40 cycles: denaturation at 95°C for 3 seconds, annealing at 60°C for 30 seconds, extension at 72°C for 20 seconds; dissolution curve and amplification curve 95°C for 15 seconds, 60°C for 1 minute, 95°C for 15 seconds. The specific amplification conditions were determined according to the melting curve of the software of the ABI-FAST7500 system. The RT-PCR primer sequences(5’-3’) used are as follows, the upstream primer sequence of ABCB1 is GGGAGCTTAACACCCGACTTA, and the downstream primer sequence is GCCAAAATCACAAGGGTTAGCTT. The upstream primer sequence of GAPDH is TGTGGGCATCAATGGATTTGG, and the downstream primer sequence is ACACCATGTATTCCGGGTCAAT.
2.2.5. Western blot
The peripheral blood leukocytes collected were collected with the lymphocyte separation solution after centrifugation (Sigma Centrifuges, Germany). The total protein samples of the lymphocytes were extracted and quantified. The protein extraction process follows Step-1; 100 UL of lysate was added to the cell precipitation (protease and phosphatase inhibitor were added 1 min before the experiment, lysate: protease inhibitor = 200:1) mixed well and placed on ice for 30 min. Step-2; centrifugated at 12,000 rpm for 20 minutes at 4°C. Step-3; the supernatant was transferred into a 1.5 ml centrifuge tube, and a part was separated to determine protein concentration. Step-4; the rest samples were added with loading buffer, and the drying bath was boiled for 10 minutes to denature. Step-5; the denatured sample is cooled on ice. Step-6; protein concentration determination is carried out according to BCA kit instructions. Twenty micrograms of total protein were added with the buffer and loaded onto the stacking gel. Additionally, they were separated using a 60 V voltage. When the protein bands reached the separating gel-stacking gel boundary, the gel electrophoresis voltage was maintained at 110 V until completion. The proteins thus separated were subjected to incubation with primary antibodies (Omnimabs, Inc., Upland, CA, USA) at 4°C overnight following their transfer to a polyvinylidene fluoride membrane. Following washing, the membrane was incubated with a secondary antibody (Omnimabs, Inc., Upland, CA, USA) at room temperature for 1 h. The membrane was after that developed in the chemiluminescence imaging system. Each sample was subjected to a western blot three times individually.
2.3. Statistical analysis
All the data was subjected to statistical analysis using SPSS 25.0 software (IBM, Armonk, NY). The normality test was employed for measurement data, presented as mean ± standard deviation if that fulfilled the normal distribution (P > 0.1). The difference between the groups was compared by using an independent sample t-test. The non-normally distributed data (P ≤ 0.1) was presented as interquartile range and median. The differences between the groups were compared using a nonparametric rank-sum test of two independent samples. The count data were presented as frequency, and a comparison of the groups’ differences was conducted using the chi-square test. Binary logistic regression analysis was employed for AMI-related risk factors. The comparisons were considered statistically significant with a 2-sided P < 0.05.
3. Results
In this study, we expanded the sample size based on the previous gene chip results to further verify the relationship between the expression of ABCB1 gene and AMI. It is assumed that the relative expression level of ABCB1 gene in peripheral blood of patients with AMI increased, which associated with the disease. Further, the ABCB1 gene expression was detected at the transcriptional and protein levels, and the ABCB1 gene may be used as a molecular marker for the early diagnosis of AMI.
3.1 Baseline data analysis
The clinical data in this report showed no significant differences in smoking or hypertension history, TG, TC, and LDL-C among the control and AMI groups (P > 0.05). However, compared to the control group, the AMI group had significantly older patients (P < 0.01). The total count of patients having diabetes in the AMI group was also significantly higher (P < 0.01), and the AMI group also had a lower level of HDL-C (P = 0.04) (see for details).
Table 1. Baseline data of the research subjects in this study
3.2. Analysis of ABCB1
3.2.1. RT-PCR product identification
Significantly smooth S-shaped amplification curves were obtained for the internal reference gene and ABCB1. In contrast, all the single peaks without any multiple peaks were obtained as the dissolution curves, indicating that RT-PCR’s reaction conditions were appropriate. The amplification primer had very strong specificity, and no nonspecific amplification occurred.
3.2.2. ABCB1 expression level
Each RT-PCR experiment was performed in triplicate. The standard deviation met the requirement of RT-PCR. The quantification of the gene expression in the experimental group and the control group was carried out by subtracting internal reference gene values from the target gene. The results showed a value of 0.26 (0.03–0.79) as the relative expression of ABCB1 within the AMI group (i.e., with the 2−ΔCt value quantitatively measured by RT-PCR reaction) and the relative expression of ABCB1 in the control group was 0.13 (0.01–0.52), thus showing that the two groups were significantly different (P < 0.05, ). The relative mRNA expression of ABCB1 in the peripheral blood in the AMI group was 2.00-fold as high as the patients in the control group, which was significantly different. Furthermore, during western blot, β-actin was employed as the internal reference protein in this study. 6 patients who met the inclusion criteria were selected, including 3 cases in the experimental group and 3 cases in the control group. Each protein test group was repeated three times to detect ABCB1 protein levels in the peripheral blood. The outcome of western blot demonstrated that in the AMI group, the degree of expression of the ABCB1 protein was 1.65 times that in the control group (p < 0.05). See for details.
Figure 1. Relative expression of ABCB1. The figure is the comparison of relative expression of ABCB1 at gene level between the AMI group and the control. AMI: acute myocardial infarction.
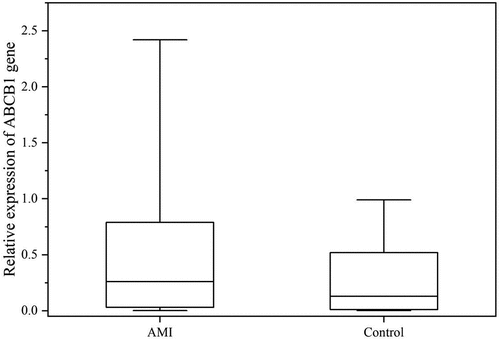
Figure 2. Relative expression level of the ABCB1 protein. In Figure, 1,3,5 represent the AMI group, 2,4,6 represent the control. In Figure 2b, the abscissa represents the sample number, the ordinate is the relative expression of ABCB1.Each sample repeated three times.AMI:acute myocardial infarction.
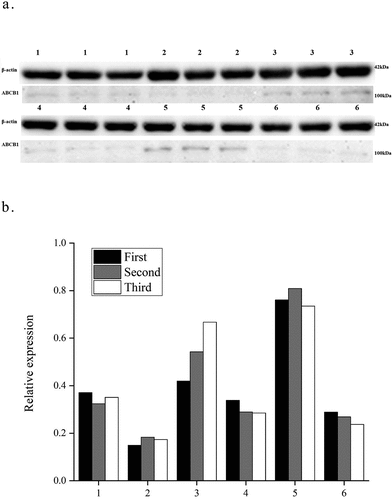
3.3. Correlation analysis
Concerning age, history of type II diabetes, and HDL-C, the baseline data obtained in this study manifested significant differences among the AMI and control groups. Thus, it was necessary to analyze further whether the relative expression of ABCB1 was related to the above factors.
We divided subjects into advanced age (≥65 years old) and young age (<65) groups; type II diabetic group and non-type II diabetic group; elevated-HDL-C group (≥1.04 mmol/L) and the low-HDL-C group (<1.04 mmol/L). 2−ΔCt was employed to present the relative expression of the ABCB1 gene level of the individual research subject. The comparisons of the relative expression of ABCB1 between the advanced age and young-age groups, between the low-HDL-C group and the elevated-HDL-C group, and between the type II diabetic group and the non-type II diabetic group were performed.
Interestingly, no significant associations were found between the relative expression of ABCB1 and patient age (P = 0.388), between the relative expression of ABCB1 and type II diabetes (P = 0.380), and between the relative expression of ABCB1 and HDL-C level (P = 0.091) (see for details).
Table 2. Correlation analysis between ABCB1 and age, type II diabetes, and HDL-C level
3.4. Logistic regression analysis
Individual research subjects were categorized into a high-expression group (2−ΔCt ≥0.907) and a low-expression group (2−ΔCt <0.907) according to the cutoff value (The maximum value corresponding to the Youden index, Youden index = sensitivity + specificity – 1) of the relative expression of ABCB1. According to the clinical data, individual subjects were also divided into the advanced-age group, the young-age group, the non-type II diabetic group, and the type II diabetic group. A binary logistic regression analysis was employed for further investigation of the results.
The results showed that the high expression of ABCB1 was related to the occurrence of AMI (P = 0.003). Compared with the low ABCB1 expression group, the risk of AMI in the high ABCB1 expression group was increased to 4.88-fold. In addition, for AMI, advanced age was also found to serve as an independent risk factor (P = 0.003) that could increase AMI’s risk to 2.63-fold. Type II diabetes was also an independent risk factor for AMI (P = 0.003) that increased AMI’s risk to 2.99-fold ().
Table 3. Logistic regression analysis results of independent risk factors for AMI
4. Discussion
This work demonstrated that compared to the control group, the expression levels of ABCB1 and ABCB1 protein in the leukocytes of peripheral blood were higher in the patients in the AMI group.
ATP-binding cassette (ABC) transporter is one of the largest families of translocation promoters widely distributed from prokaryotes to humans [Citation14]. Forty-nine different genes can encode human ABC transporters [Citation15]. Each of these 49 genes encodes one protein and can also encrypt multiple variants of ABC proteins because some of these genes are alternately spliced during the transcription process. Some members of the ABC family can also encode transporters involved in transporting the various substrates. ABCB1 is the first cloned member of the ABC family and is highly conserved in phylogeny [Citation16,Citation17]. ABCB1’s promoter region contains multiple transcription factor binding sequences, including specific protein 1, activator protein 1, nuclear factor interleukin (IL) 6, and forehead transcription factor or T cell factor/lymphoid enhancer factor, suggesting the presence of a complex regulatory mechanism [Citation18]. A previous study has shown that certain specific polymorphisms in the promoter region of ABCB1 may play an important regulatory role in coronary heart disease occurrence and development [Citation19].
The mechanism of AMI is that the formation of atherosclerotic plaque is rather complex. It has been established that from the formation of atherosclerotic plaque to rupture or erosion of plaque leading to partial or occlusion of coronary arteries, inflammation plays a key part in coronary artery disease [Citation20]. Atherosclerosis is a chronic inflammatory disease in which the various active immune cells in the lesion mainly produce pro-inflammatory cytokines [Citation21]. The activation of inflammatory cells plays a key role in acute coronary syndrome [Citation22]. Additionally, prothrombin is exposed to the coagulation system due to the significant influence of pro-inflammatory cytokines and chemokines on the fibrous cap damage plaques are destroyed and ruptured the blood flow and inducing acute cardiovascular events [Citation21]. Moreover, the severity of inflammation is an important indicator of the poor prognosis of AMI [Citation23]. In this study, a high ABCB1 expression might be an independent risk factor for AMI because it can promote inflammation.
AMI can have multiple etiology and complex physiological and pathological mechanisms, with the Framingham Heart Study identifying risk factors [Citation24]. It has been reported that important risk factors of individuals with AMI include diabetes, inflammation, and aging, but age growth might not be controlled by external factors [Citation25]. ABCB1 is involved in at least two risk factors of coronary heart disease: glucose metabolism and inflammatory response.
ABCB1 has been reported to mediate the process of systemic inflammation [Citation19]. On the one hand, ABCB1 can secrete endogenous inflammatory mediators, such as prostaglandins and leukotrienes. Moreover, ABCB1 can effectively aggravate the inflammation and destruction of ischemic tissues of various sizes from an initial stage [Citation26]. Alternatively, ABCB1 may also be involved in the transmembrane transport of IL-2 and interferon-gamma (INF-γ) and other pro-inflammatory cytokines. However, how ABCB1 can directly regulate the release of the various cytokines remains to be further confirmed [Citation18]. A study has shown that compared with ABCB1-negative T helper (Th)17 cells, ABCB1-positive Th17 cells are characterized by the massive production of pro-inflammatory Th1 (i.e., INF-γ) and Th17 cytokines (i.e., IL-17A, IL-17 F, and IL-22), but generate low levels of anti-inflammatory cytokines, such as IL-10 [Citation27]. ABCB1 thus can act as a highly sensitive indicator of inflammation. The increase in serum ABCB1 levels can be related to the future dangers of coronary heart disease [Citation19].
One important risk factor identified for coronary heart disease is diabetes, and it has been positively correlated with atherosclerosis onset and progression [Citation24]. As a systemic metabolic disease, various vascular complications often occur during diabetes. Atherosclerosis, coronary artery disease, and peripheral vascular disease are only a few of the many macrovascular complications resulting from diabetes [Citation17]. Thus, by identifying the different risk factors, be possible to plan better the various preventive measures to reduce AMI and prevent potentially fatal consequences. The ABC transporter family, including P-glycoprotein (P-GP) encoded by ABCB1, is widely expressed in the lumen membrane of the microvascular endothelium and the apical membrane of the choroid plexus epithelium. However, these transporters are easily altered in certain diseases, and diabetes can cause changes in the functions and expression of P-GP in the blood-brain barrier and can lead to the development of many macrovascular and microvascular complications related to endothelial dysfunction [Citation17]. Chronic hyperglycemia can change P-GP activity in patients with diabetes [Citation28]. In addition to insulin deficiency, diabetes has also been associated with an increased level of systemic inflammatory mediators (e.g., cytokines), regulating the function and expression of P-GP [Citation29]. In the diabetic state, protein kinase C (PKC) signal transduction has been enhanced, and the PKC activator, phorbol ester, can increase the expression of human ABCB1. Hyperglycemia can also increase the protein expression encoded by ABCB1 by activating PKC-alpha and nuclear factor kappa B [Citation30]. In this study, it was observed that there was a statistically significant difference between the AMI group and the control group for diabetes (P = 0.003). However, the correlation analysis found no significant correlation between the high ABCB1 expression and type II diabetes (P = 0.380).
Advanced age has been related to the prevalence and intensity of coronary artery disease [Citation31]. More than 60% of AMI occurs in individuals 65 years of age or older in the United States. Around a third of AMI, cases have been observed in people above 75 years of age [Citation32]. The mortality of AMI increases exponentially with age. Approximately 60% of myocardial infarction deaths in the United States occur in people 75 years of age or older, accounting for about 6% of the population [Citation32]. With the rapid population growth and aging of the world population, along with an increase in the pervasiveness of long-term survivors of AMI, the burden of AMI has increased significantly.
The expression of ABCB1 in CD8(+) and CD4(+) T lymphocytes can also increase with age. The P-GP encoded by ABCB1 may have possible involvement in activating the growth factors, secretion of cytokines, and cytotoxic molecules. Moreover, due to the slow response of T cells in the elderly, the ability of immunodeficiency has been significantly reduced, thus suggesting that ABCB1 plays a role in the age-related immune process [Citation33]. The high expression of ABCB1 may be related to the slow response of T cells. For instance, it has been found that the AMI group and the control group were statistically different concerning age distribution. The age of individuals in the AMI group was significantly high compared to that of the control group. Being an independent risk factor for AMI, advanced age increased the AMI risk 2.63-fold, which was consistent with the theoretical expectation [Citation33].
Additionally, in the literature, we found an intriguing fact that high expression of the ABCB1 increases drug efflux, particularly antiplatelet drugs such as clopidogrel (CLP) [Citation34–36]. The response to CLP may be affected by genetic and non-genetic factors. Among the genetic factors, the common polymorphism of the gene encoding P-GP is considered to be a potential determinant factor of the therapeutic effect of CLP. However, the presence of the 3435c > T allele affected the pharmacokinetics of CLP but did not affect pharmacodynamics. 3435c > T polymorphism had no significant effect on long-term results and adverse reactions during treatment [Citation37]. MicroRNA mediated genes regulate the drug efflux pump of ABC transporter family. Epigenetic mechanisms, such as DNA methylation and tissue modification, alter the expression of ABC transporters and contribute to increased drug efflux [Citation38]. MicroRNA plays an important role not only in the change of drug distribution but also in drug resistance. It should be noted that few patients do directly progress to AMI from a healthy state. At the vascular level, they show a step by step formation of thrombosis and the gradual narrowing of the cardiovascular system. During this process, oral antiplatelet drugs are necessary, and patients with high expression of ABCB1 might reduce the effectiveness of these oral drugs, thereby accelerating thrombosis and vascular stenosis and then possibly progressing to AMI [Citation36]. Moreover, microRNA can target multiple genes and control the molecular and cellular mechanism of the occurrence and development of atherosclerosis by acting on different vascular cells in atherosclerosis. In all stages of atherosclerosis, more than 190 microRNAs are involved in regulating 160 different genes. MicroRNA is involved in atherosclerosis-related molecular pathways, including endothelial dysfunction, cell adhesion, proliferation, lipid uptake, and outflow, production of inflammatory mediators, activation of platelets, and plaque formation [Citation39].
The results showed that the high expression of ABCB1 in peripheral blood leukocytes was related to the occurrence of AMI. A 4.88-fold risk of AMI was found when the expression of ABCB1 was significantly high. Additionally, advanced age and type 2 diabetes were found to act as independent risk factors for AMI, increasing AMI risk by 2.63-fold and 2.99-fold, respectively ().
5. Conclusions
The ABCB1 gene and protein levels in the leukocytes of the peripheral blood within the AMI group were significantly higher than those in the control group. This high expression of ABCB1 in peripheral blood leukocytes was associated with AMI, and its increased levels may raise the risk of AMI by promoting inflammation. The high ABCB1 expression in peripheral blood leukocytes might serve as a novel molecular marker for an early diagnosis of AMI.
Authors’ contributions
JR, HM and FM designed the research study. JR, HM and FM performed the research.
FM, XL, YC and ZY conducted the work and were involved in data collection. XL, YC and ZY analyzed the data. All authors contributed to editorial changes in the manuscript. All authors read and approved the final manuscript.
Ethics approval and consent to participate
All subjects gave their informed consent for inclusion before they participated in the study. The study was conducted in accordance with the Declaration of Helsinki, and the protocol was approved by the Ethics Committee of The Third Hospital of Jilin University (approval number: 2016WJW013)
Consent for publication
This manuscript does not contain any individual data of any subject.
Supplemental Material
Download Zip (2.4 MB)Acknowledgements
We would like to express our gratitude to all those who helped us during the writing of this manuscript. We thank Shenyang Huibai Company for its support of the western blot
experiments in this study, and all the peer reviewers for their opinions and suggestions.
Disclosure statement
No potential conflict of interest was reported by the author(s).
Data availability statement
The datasets used and/or analyzed during the current study are available from the corresponding author on reasonable request.
Supplementary material
Supplemental data for this article can be accessed here
Additional information
Funding
References
- Bajaj A, Sethi A, Rathor P, et al. Acute complications of myocardial infarction in the current era: diagnosis and management. J Investig Med. 2015 Oct;63(7):844–855.
- Tiwari RP, Jain A, Khan Z, et al. Cardiac troponins I and T: molecular markers for early diagnosis, prognosis, and accurate triaging of patients with acute myocardial infarction. Mol Diagn Ther. 2012 Dec;16(6):371–381.
- Xue S, Zhu W, Liu D, et al. Circulating miR-26a-1, miR-146a and miR-199a-1 are potential candidate biomarkers for acute myocardial infarction.Mol Med. 2019 May 15;25(1):18.
- Chen Y, Tao Y, Zhang L, et al. Diagnostic and prognostic value of biomarkers in acute myocardial infarction. Postgrad Med J. 2019 Apr;95(1122):210–216.
- Aziz H, Zaas A, Ginsburg GS. Peripheral blood gene expression profiling for cardiovascular disease assessment. Genomic Med. 2007;1(3–4):105–112.
- Kiliszek M, Burzynska B, Michalak M, et al. Altered gene expression pattern in peripheral blood mononuclear cells in patients with acute myocardial infarction. PLoS One. 2012;7(11):e50054.
- Vignoli A, Tenori L, Giusti B, et al. NMR-based metabolomics identifies patients at high risk of death within two years after acute myocardial infarction in the AMI-Florence II cohort. BMC Med. 2019 Jan 7;17(1):3.
- Meng H, Wang X, Ruan J, et al. High expression levels of the socs3 gene are associated with acute myocardial infarction. Genet Test Mol Biomarkers. 2020 Jul;24(7):443–450.
- Ruan J, Meng H, Wang X, et al. Low expression of FFAR2 in peripheral white blood cells may be a genetic marker for early diagnosis of acute myocardial infarction. Cardiol Res Pract. 2020 Jan 25;2020:3108124.
- Kong FC, Lang LQ, Hu J, et al. A novel epigenetic marker, Ten-eleven translocation family member 2 (TET2), is identified in the intractable epileptic brain and regulates ATP binding cassette subfamily B member 1 (ABCB1) in the blood-brain barrier. Bioengineered. 2022 Mar;13(3):6638–6649.
- Ibanez B, James S, Agewall S, et al. Héctor Bueno, et al.2017 ESC guidelines for the management of acute myocardial infarction in patients presenting with ST-segment elevation: the task force for the management of acute myocardial infarction in patients presenting with ST-segment elevation of the European Society of Cardiology (ESC). Eur Heart J. 2018 Jan 7;39(2):119–177.
- Wang X, Meng H, Ruan J, et al. Low G0S2 gene expression levels in peripheral blood may be a genetic marker of acute myocardial infarction in patients with stable coronary atherosclerotic disease: a retrospective clinical study. Medicine (Baltimore). 2021;100(3):e23468.
- Tan B, Liu L, Yang Y, et al. Low CPNE3 expression is associated with risk of acute myocardial infarction: a feasible genetic marker of acute myocardial infarction in patients with stable coronary artery disease. Cardiol J. 2019;26(2):186–193.
- José Ferreira M, Sá-Nogueira ID. A multitask ATPase serving different ABC-type sugar importers in Bacillus subtilis. J Bacteriol. 2010 Oct;192(20):5312–5318.
- Schumacher T, Benndorf RA. ABC transport proteins in cardiovascular disease-A brief summary. Molecules. 2017 Apr 6;22(4):589.
- Vasiliou V, Vasiliou K, Nebert DW. Human ATP-binding cassette (ABC) transporter family. Hum Genom. 2009;3:281–290.
- Liu L, Liu X-D. Alterations in function and expression of ABC transporters at blood-brain barrier under diabetes and the clinical significances. Front Pharmacol. 2014 Dec 10;5:273.
- Elke Cario. P-glycoprotein multidrug transporter in inflammatory bowel diseases: more questions than answers. Editorial World J Gastroenterol. 2017 Mar 7;23(9):1513–1520.
- Jin Y, Wang Q, Wang G, et al. ABCB1 C3435T polymorphism and the risk of coronary heart disease: a meta-analysis. Genet Test Mol Biomarkers. 2014 Mar;18(3):164–173.
- Libby P. Inflammation in atherosclerosis. Nature. 2002 Dec 19-26;420(6917):868–874.
- Frostegård J. Immunity, atherosclerosis and cardiovascular disease. BMC Med. 2013 May 1;11:117.
- Chen X-M, Zhang T, Qiu D, et al. Gene expression pattern of TCR repertoire and alteration expression of IL-17A gene of γδ T cells in patients with acute myocardial infarction. J Transl Med. 2018 Jul 5;16(1):189.
- Wang R, Wen X, Huang C, et al. Association between inflammation-based prognostic scores and in-hospital outcomes in elderly patients with acute myocardial infarction. Clin Interv Aging. 2019 Jul 4;14:1199–1206.
- Dzubur A, Gacic E. Mevludin mekic. Comparison of patients with acute myocardial infarction according to age. Med Arch. 2019 Feb;73(1):23–27.
- Johansson S, Rosengren A, Young K, et al. Mortality and morbidity trends after the first year in survivors of acute myocardial infarction: a systematic review. BMC Cardiovasc Disord. 2017 Feb 7;17(1):53.
- Kim Y-O, Kim S-Y, Hwan Yun D, et al. Association between ABCB1 polymorphisms and ischemic stroke in Korean population. Exp Neurobiol. 2012 Dec;21(4):164–171.
- Andersen V, Svenningsen K, Almind Knudsen L, et al. Novel understanding of ABC transporters ABCB1/MDR/P-glycoprotein, ABCC2/MRP2, and ABCG2/BCRP in colorectal pathophysiology. World J Gastroenterol. 2015 Nov 7;21(41):11862–11876.
- Chitnis SD, Ogasawara K, Schniedewind B, et al. Concentration of tacrolimus and major metabolites in kidney transplant recipients as a function of diabetes mellitus and cytochrome P450 3A gene polymorphism. Xenobiotica. 2013 Jul;43(7):641–649.
- Zhang L-L, Liang L, Jin S, et al. Tissue-specific alterations in expression and function of P-glycoprotein in streptozotocin-induced diabetic rats. Acta Pharmacol Sin. 2011 Jul;32(7):956–966.
- Kameyama N, Arisawa S, Ueyama J, et al. Increase in P-glycoprotein accompanied by activation of protein kinase Calpha and NF-kappaB p65 in the livers of rats with streptozotocin-induced diabetes. Biochim Biophys Acta. 2008 May;1782(5):355–360.
- Jing H, Yang Y, Zhang G, et al. Clinical risk factors for new-onset atrial fibrillation in acute myocardial infarction: a systematic review and meta-analysis. Medicine (Baltimore). 2019 Jun;98(26):e15960.
- Rich MW. Epidemiology, clinical features, and prognosis of acute myocardial infarction in the elderly. quiz 12. Am J Geriatr Cardiol. 2006 Jan-Feb;15(1):7–11.
- Efferth T. Adenosine triphosphate-binding cassette transporter genes in ageing and age-related diseases. Ageing Res Rev. 2003 Jan;2(1):11–24.
- Taubert D, von Beckerath N, Grimberg G, et al. Impact of P-glycoprotein on clopidogrel absorption. Clin Pharmacol Ther. 2006;80(5):486–501.
- Ma L, Yuan Y, Li J, et al. Distribution of CYP2C19, ABCB1 and PON1 polymorphisms in Chinese Han, Hui, Uygur and Kazak patients with coronary atherosclerotic heart disease. Int J Immunogenet. 2020;47(6):539–545.
- Wang XQ, Shen CL, Wang BN, et al. Genetic polymorphisms of CYP2C19 2 and ABCB1 C3435T affect the pharmacokinetic and pharmacodynamic responses to clopidogrel in 401 patients with acute coronary syndrome. Gene. 2015;558(2):200–207.
- Karaźniewicz-Łada M, Danielak D, Rubiś B, et al. Impact of common ABCB1 polymorphism on pharmacokinetics and pharmacodynamics of clopidogrel and its metabolites. J Clin Pharm Ther. 2015 Apr;40(2):226–231.
- Haenisch S, Werk AN, Cascorbi I. MicroRNAs and their relevance to ABC transporters. Br J Clin Pharmacol. 2014;77(4):587–596.
- Chatzopoulou F, Kyritsis KA, Papagiannopoulos CI, et al. Dissecting miRNA-Gene networks to map clinical utility roads of pharmacogenomics-guided therapeutic decisions in cardiovascular precision medicine. Cells. 2022 Feb 10;11(4):607.