ABSTRACT
LncRNAs play a regulatory role in osteoarthritis (OA); however, the detailed mechanism remains to be elucidated. This study aimed to investigate the role of lncRNA zinc finger NFX1-type containing 1 (ZNFX1) antisense 1 (ZFAS1) in OA progression and explore its possible mechanismsagainst oxidative stress. Human cartilage specimens were obtained from 10 patients without OA who underwent traumatic amputation and 25 patients with OA who underwent total knee replacement surgery. Chondrocytes were prepared from harvested articular cartilage. ZFAS1, nuclear factor erythroid 2-related factor 2 (Nrf2), and heme oxygenase 1 (HO-1) expression levels were analyzed using quantitative reverse transcription PCR and WB. The chondrocyte growth was indicated by MTT and colony formation assays. Chondrocyte apoptosis, reactive oxygen species generation, and anti-oxidative enzymes activities were also measured. ZFAS1 expression was reduced in OA samples and lipopolysaccharide (LPS)-treated chondrocytes used as an OA cell model mimic. ZFAS1 overexpression facilitated proliferation and repressed oxidative stress, inflammation, and apoptosis in LPS-induced chondrocytes. ZFAS1 also activated the anti-oxidative Nrf2-HO-1 pathway. ZFAS1 directly targeted miR-1323, which partially reversed the effects of ZFAS1 on chondrocyte proliferation, oxidative stress, inflammation, and apoptosis. Furthermore, Nrf2 was negatively regulated by miR-1323. The effect of miR-1323 inhibition was partly abrogated by the administration of brusatol, an Nrf2 inhibitor. Collectively, the results showed that ZFAS1 promoted chondrocyte proliferation and repressed oxidative stress, possibly by regulating the novel miR-1323-Nrf2 axis of the inflammation and apoptosis triggered by LPS, indicating that ZFAS1 is a promising therapeutic target for OA.
Graphical abstract
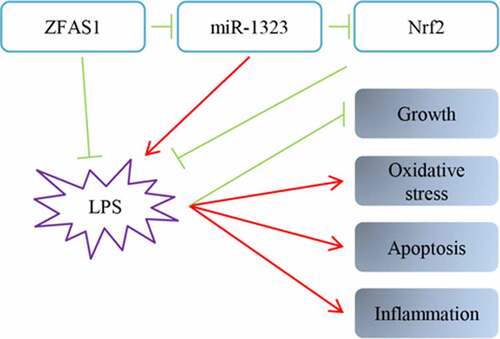
Highlights
ZFAS1 was downregulated in OA samples compared with non-OA samples;
ZFAS1 activated the Nrf2-HO-1 pathway in LPS-treated chondrocytes;
ZFAS1 acted as competing endogenous RNA of miR-1323;
miR-1323 partially rescued the ZFAS1 89 repression of chondrocyte oxidative 90 disorders.
Introduction
Osteoarthritis (OA) is a complicated disease with an unclear etiology that can negatively influence various joints and hampers daily life activities. It is characterized by molecular, biomechanical, biochemical, and morphological alterations in the extracellular matrix (ECM) and cells. This results in softening, ulceration, fibrillation, synovial inflammation, articular cartilage loss, subchondral bone sclerosis, cysts, and osteophyte formation. OA is a polygenic and multifactorial disease. Its pathogenesis is influenced by environmental and genetic factors related to the activation of molecular pathways relevant to articular injury development [Citation1]. In order to discover novel approaches for OA prevention and treatment, it is necessary to further investigate these pathways and their corresponding phenotypes in joint tissues.
Previous studies have demonstrated that OA development is closely associated with reactive oxygen species (ROS) and oxidative stress [Citation2,Citation3]. Cellular processes that lead to OA can be initiated by pro-inflammatory mediators such as cytokines, ROS, and lipid mediators [Citation4,Citation5]. Oxidative stress is a disorder in the pro-oxidant/antioxidant balance, which is conducive to OA [Citation6], indicating that adding appropriate antioxidants can correct this disturbance. Redox mechanisms can affect intracellular signaling, and cells appear to be sensitive to the deletion of the redox control and regulatory systems [Citation7].
Several studies have shown the essential role of long non-coding RNAs (lncRNAs), transcripts over 200 nucleotides in length, in the inflammatory response and their relation to tumors and other diseases [Citation8–10]. The therapeutic value of the lncRNA zinc finger NFX1-type containing 1 antisense 1 (ZFAS1) has been shown in many diseases. ZFAS1 is transcribed from the antisense strand near the 5’-terminus of the protein-encoding gene zinc finger NFX1-type containing 1 (ZNFX1) [Citation11]. Low ZFAS1 expression in breast cancer suggests the tumor suppressor role of this transcript [Citation11]. However, other studies have revealed an oncogenic role of ZFAS1 in cancer progression [Citation12–14]. Apart from its role in neoplasia pathogenesis, ZFAS1 participates in molecular cascades resulting in a series of disorders, such as OA [Citation10,Citation15], epilepsy [Citation16], rheumatoid arthritis [Citation17,Citation18], and atherosclerosis [Citation19]. MicroRNAs (miRs) are conservative small RNAs consisting of 20–22 nucleotides, capable of regulating gene expression in a post-transcriptional manner by combining with mRNA 3’-UTR, which then leads to mRNA degradation and translation suppression [Citation20–22]. Mounting evidence has confirmed that miRs might impact different biological disorders, including osteoporosis [Citation23]. miR-1323 has been associated with various diseases, such as lung cancer, breast cancer, adenocarcinoma, hepatocellular cancer, and diabetes [Citation24–28]. However, the molecular mechanisms of action of ZFAS1 and miR-1323 in oxidative stress regulation, including the antioxidant nuclear factor-erythroid 2-related factor 2 (Nrf2) and response to OA progression, remained unclear.
In this study, we explored lipopolysaccharide (LPS) treatment on chondrocytes to mimic an OA cell model, according to previous reports [Citation29–31]. We hypothesized that ZFAS1 might play a role in OA progression. Therefore, we attempted to verify the function of ZFAS1 in oxidative response, inflammation, and apoptosis during OA development and explore the interaction between ZFAS1, miR-1323, and Nrf2 using bioinformatics analysis and mechanistic experiments. Our findings revealed that low ZFAS1 expression alleviates oxidative stress in chondrocytes via regulation of the miR-1323-Nrf2 axis, thus making it a promising new therapeutic target for OA.
Material and methods
Patient samples
We obtained human cartilage specimens from 10 patients without OA who underwent traumatic amputation and 25 patients with OA who underwent total knee replacement surgery. Using the American College of Rheumatology criteria, we diagnosed the OA cases. The samples were obtained from the Affiliated Wuxi No. 2 People’s Hospital from the department of Orthopedics of the Nanjing Medical University. All patients provided informed consent, and the Ethics Committee of the Affiliated Wuxi No. 2 People’s Hospital of the Nanjing Medical University approved the study.
Cell culture
Chondrocytes were prepared from harvested articular cartilage using Dulbecco’s modified Eagle’s medium (DMEM; Gibco, Grand Island, NY, USA), supplemented with 0.2% collagenase II (Solarbio, Beijing, China). The obtained chondrocytes were incubated in DMEM with 10% fetal bovine serum (FBS; Invitrogen, Carlsbad, CA, USA) for one day at 37°C. After filtering with a cell strainer (0.075 mm), the chondrocytes were washed using phosphate-buffered saline (PBS; Invitrogen), and treated chondrocytes were cultured for another two weeks. Next, cultured chondrocytes (experimental materials) were kept damp with 5% CO2 at 37°C. For the LPS stimulation group, the chondrocytes were treated with 0.5 μM LPS for 24 h. For the brusatol treatment group, chondrocytes were treated with 1 μM brusatol for 48 h.
Transfections
For overexpression of ZFAS1, full-length ZFAS1 sequences were cloned into a pcDNA3.1 vector (pcDNA, Invitrogen), named pcDNA3.1-ZFAS1. Ribobio (Guangzhou, China) provided the miR-1323 mimic (5’- UCA AAA CUG AGG GGC AUU UUC U-3’), miR-1323 inhibitor (5’-AGA AAA UGC CCC UCA GUU UUG A-3’), and the negative controls (NC) mimic and inhibitor (5’-UUC UCC GAA CGU GUC ACG U-3’ and 5’-CAG UAC UUU UGU GUA CAA-3’). Chondrocytes were transfected with all plasmids and oligonucleotides using the Lipofectamine 2000 (Invitrogen), as per the manual.
Dual-luciferase reporter assay (DLRA)
Sequences of ZFAS1 and Nrf2 3’-UTR, including mutant-type (Mut) or wild-type (WT) putative miR-1323 targeting sites, were amplified and cloned into the luciferase reporter pisCHECK-2 vector, constituting the constructs ZFAS1 WT, ZFAS1 Mut, Nrf2 WT 3’-UTR, and Nrf2 Mut 3’-UTR. Subsequently, the constructed reporter plasmids were treated with NC mimic or miR-1323 mimic co-transfection using the Lipofectamine 2000 reagents (Invitrogen) and incubated for 48 h. Luciferase activities were determined in chondrocyte lysates using a dual-luciferase reporter assay kit (Promega), as per the manual.
Quantitative reverse transcription PCR
We extracted total RNA from tissues (50 mg) and cells using TRIzol, and assessed its concentration using a NanoDrop2000 (OD260). The complementary DNA (cDNA) was obtained for quantitative (q)PCR via reverse transcription (RT) using the MMLV First-Strand Kit and Oligo (dT) 20 primer. We detected the mRNAs by qRT-PCR using the relevant kits, following the manufacturer’s instructions. The qRT-PCR cycles followed an initial denaturation at 95°C for 10 min; 40 denaturation cycles at 95°C for 15s; and extension at 60°C for 40s. We quantified the target mRNA expression using the glyceraldehyde-3-phosphate dehydrogenase (GAPDH) gene as the internal reference and the 2−ΔΔCT method. We performed the steps above in triplicate.
Western blot (WB)
We lysed the cells using a protease inhibitor cocktail and radioimmunoprecipitation assay buffer (pH 8.0). Protein concentrations were determined using a bicinchoninic acid assay (BCA) kit. The proteins were subjected to SDS-PAGE and subsequently transferred to a polyvinylidene fluoride membrane (Millipore, MA, USA). The vacant sites were blocked by overnight incubation with the primary antibodies at 4°C; this step was followed by a rinse with Tris-buffered saline with 0.1% Tween® 20 detergent (TBST). Next, we observed the immunoblots by incubation with the secondary antibodies at room temperature for 60 min. After rinsing with TBST a few times, we observed the bands using the maximum sensitivity substrate kit (Thermo, MA, USA).
Enzyme-linked immunosorbent assay (ELISA)
Chondrocytes were homogenized and kept on ice. The levels of inflammatory factors were determined using specific ELISA kits (Thermo Fisher Scientific, USA), following the manufacturer’s instructions.
MTT assay
The MTT (Sigma-Aldrich Corp, St. Louis, MO, USA) assay was used to detect cell proliferation. Generally, transfected chondrocytes (7 × 103 cells/well) were inoculated into 96-well plates at different time points (0, 1, 2, and 3 days) at 37°C. Chondrocytes were then added to 20 μL of 5 mg/mL MTT solution (Sigma-Aldrich) and incubated for 4 h. After removing the supernatant, 150 μL of DMSO (Sigma-Aldrich) was added to dissolve the formazan precipitate. Finally, absorbance values at 490 nm were obtained using a microplate reader.
Colony formation assay (CFA)
After cultivation in 6-well plates for seven days, chondrocytes were fixed in 4% formaldehyde for 20 min and stained with 1% crystal violet.
Flow cytometry (FC)
We evaluated apoptosis using an apoptosis detection kit. After being suspended in 20 µL of binding buffer, 10 µL of cell suspension was treated with 5 µL of Annexin V-FITC/PI. The apoptosis rate was measured using a flow cytometer.
Measurement of ROS levels
ROS generation was determined using a dichloro-dihydro-fluorescein diacetate (DCFH-DA) fluorescence probe. Cells were incubated with 10 μM DCFH-DA in the dark for 30 min at 37°C. The fluorescence intensity (excitation at 488 nm and emission at 525 nm) [Citation32,Citation33] was determined using a fluorescence microscope (Tokyo, Japan).
Anti-oxidative enzymes activities measurement
The protein concentration in the cell lysates was measured using a standardized BCA protein assay kit (Beyotime Institute of Biotechnology, Shanghai, China). Activities of the anti-oxidative enzymes superoxide dismutase (SOD) and catalase were determined using the relevant assay kits and displayed as U/mg [Ye Citation34].
Data analysis
All data are shown as the mean ± standard deviation (SD). Moreover, we used the one-way analysis of variance (ANOVA) to compare different groups; Student’s t-test was used to assess differences between two groups. Statistical significance was set at P < 0.05.
Results
ZFAS1 expression is downregulated in OA cartilage and LPS-treated chondrocytes
In this study, we hypothesized that ZFAS1 might play a role in OA progression. Therefore, we first evaluated the ZFAS1 expression in OA samples and LPS-treated chondrocytes. We analyzed OA samples (n = 25) and non-OA samples (n = 10) using qRT-PCR to elucidate the role of ZFAS1 in OA progression. The results revealed that ZFAS1 expression in OA cartilage samples was dramatically downregulated relative to that in the health samples used as control ()). To confirm this, we examined the levels of ZFAS1 in LPS-treated chondrocytes. Chondrocytes were exposed to 0.5 μM LPS for 1 day, after which ZFAS1 levels were detected. Compared to its levels in the untreated control group, LPS dramatically decreased ZFAS1 expression ()). These data suggest that ZFAS1 expression is downregulated during OA progression.
ZFAS1 overexpression increases viability and represses oxidative stress, inflammation, and apoptosis of LPS-treated chondrocytes
We exposed the chondrocytes to the pcDNA3.1-ZFAS1 vector for 24 h to analyze the influence of ZFAS1 on the LPS-treated chondrocytes’ properties. qRT-PCR data showed that transfection of the ZFAS1 overexpression vector increased ZFAS1 transcript levels ()). The MTT assay indicated that the chondrocyte growth rate was repressed on 1, 2, and 3 days after LPS stimulation, while ZFAS1 overexpression recovered the growth rate of LPS-treated cells to control values ()). Furthermore, ZFAS1 treatment led to a noticeable increase in colony numbers formed by LPS-treated chondrocytes, based on the data from the soft agar CFA ()). These results suggest a cytoprotective role of ZFAS1 in LPS-treated chondrocytes.
Figure 2. Influence of ZFAS1 overexpression on growth, oxidative stress, inflammation, and apoptosis of LPS-treated chondrocytes. Chondrocytes were exposed to pcDNA3.1-empty (NC) or pcDNA3.1-ZFAS1 (ZFAS1) transfection for one day and then exposed to 0.5 μM LPS for another day. (a) ZFAS1 level was analyzed through qRT-PCR. (b) The cell growth rate on days 1, 2, and 3 was determined after transfection through MTT assay. (c) Soft agar CFA of LPS-treated chondrocytes. The colony number is displayed in the right panel. (d) DCFH-DA staining was conducted to evaluate ROS production. (e, f) Activity levels of the anti-oxidative enzymes SOD and catalase were determined via corresponding assay kits. (g) WB was used to detect HO-1 and Nrf2 protein levels in the chondrocytes. (h) FC was used for evaluating the number of apoptotic cells. The apoptosis rate of LPS-treated chondrocytes is shown in the lower right panel. (i) ELISA was used for analyzing the production of pro-inflammatory cytokines in LPS-treated chondrocytes vs. control group (* P < 0.05, ** P < 0.01, *** P < 0.001), and LPS-treated chondrocytes vs. LPS+OE-NC group ($ P < 0.05, $$ P < 0.01).
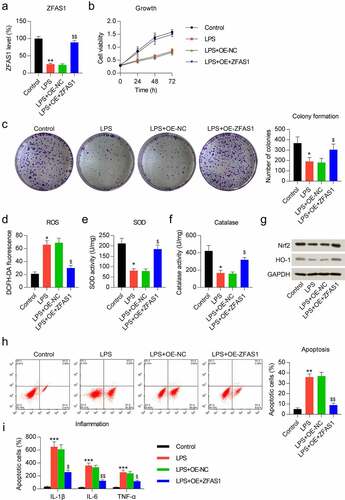
To evaluate the effect of ZFAS1 on the oxidative response of LPS-treated chondrocytes, we examined ROS production, SOD and catalase activities, and Nrf2-heme oxygenase 1 (HO-1) pathway activation in these cells. We found that ROS generation was increased in the cells treated with LPS, and ZFAS1 overexpression rescued the ROS production to control levels ()). We next assessed the activity of two anti-oxidative enzymes, SOD and catalase. The data indicated a significant reduction of activity following LPS stimulation. The downregulation of SOD and catalase activities was restored by ZFAS1 overexpression (). The Nrf2-HO-1 pathway is a well-documented anti-oxidative signal transduction pathway. LPS treatment contributed to reduce Nrf2 and HO-1 protein levels. However, following ZFAS1 overexpression, Nrf2 and HO-1 expression was increased ()). These data demonstrated that LPS stimulation induced oxidative stress in chondrocytes, which was alleviated by ZFAS1.
Considering the reports that oxidative disorders generally result in inflammation and apoptosis [Citation35–37], we next assessed the inflammation and apoptosis of LPS-treated chondrocytes. First, Annexin V-FITC/PI FC showed evident cell apoptosis in chondrocytes treated with LPS, while the apoptotic cell number was significantly reduced after transfection with the ZFAS1 overexpressing vector ()). Next, ELISA was used to detect the release of pro-inflammatory cytokines, including IL-1β, IL-6, and TNF-α, in the chondrocytes. The data showed that LPS induced these three cytokines, but this increment was abolished by ZFAS1 ()).
ZFAS1 acts as competing endogenous RNA of miR-1323
Our bioinformatic prediction results suggested that ZFAS1 might target miR-1323 ()). A direct interaction between miR-1323 and ZFAS1 was detected using DLRA. The results revealed that luciferase function was inhibited following transfection with the miR-1323 mimic, which interacted with 50% of the ZFAS1 molecules at its binding motif compared to the control groups ()). We also analyzed the effect of LPS induction and ZFAS1 overexpression on miR-1323 expression in chondrocytes using qRT-PCR. The miR-1323 expression was upregulated in response to LPS stimulation, while ZFAS1 overexpression reduced the miR-1323 levels ()). These results demonstrated that miR-1323 expression decreased following the ZFAS1 overexpression and that ZFAS1 acts as competing endogenous RNA (ceRNA) of miR-1323.
Figure 3. ZFAS1 acts as a ceRNA of miR-1323. (a) Graphical representation of the conserved ZFAS1 binding motif at miR-1323. (b) DLRA was conducted using the luciferase reporter constructs that contained either the ZFAS1 Mut or WT sequences after miR-1323/NC mimic transfection. The firefly luciferase activity was standardized to Renilla luciferase activity. (c) Chondrocytes were exposed to pcDNA3.1-empty (NC) or pcDNA3.1-ZFAS1 (ZFAS1) transfection for one day and then treated with 0.5 μM LPS for another day. The miR-1323 level was assessed using qRT-PCR. LPS-treated chondrocytes vs. indicated or control group (*, P < 0.05, **, P < 0.01); LPS-treated chondrocytes vs. LPS+OE-NC group ($, P < 0.05).
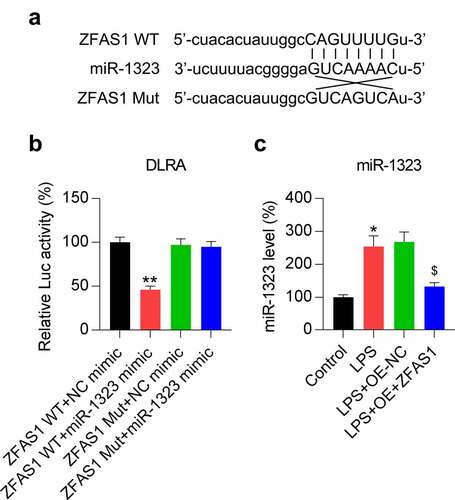
MiR-1323 upregulation counteracted the effect of ZFAS1 overexpression on cell viability and repressed oxidative stress, inflammation, and apoptosis of LPS-treated chondrocytes
To determine whether miR-1323 may reverse the influence of ZFAS1 on the viability of LPS-treated chondrocytes, the miR-1323 mimic was transfected into chondrocytes that were also co-transfected with ZFAS1 overexpression vector. qRT-PCR analyses confirmed that the miR-1323 level was upregulated in these cells ()). MTT and colony formation assays showed that miR-1323 upregulation impaired cell viability and growth rate of chondrocytes ()).
Figure 4. Influence of miR-1323 upregulation on cell growth, oxidative stress, inflammation, and apoptosis of ZFAS1-overexpressing LPS-treated chondrocytes. Chondrocytes were exposed to pcDNA3.1-ZFAS1 (ZFAS1), NC/miR-1323 mimic co-transfection for one day and then exposed to 0.5 μM LPS for another day. (a) The miR-1323 level was analyzed through qRT-PCR. (b) The cell growth rate on days 1, 2, and 3 was determined after transfection through MTT assay. (c) Soft agar CFA of LPS-treated chondrocytes. The colony number is displayed in the right panel. (d) DCFH-DA staining was conducted to evaluate ROS production. (e, f) Activity levels of the anti-oxidative enzymes SOD and catalase were determined via the corresponding assay kits. (g) WB was used to detect the HO-1 and Nrf2 protein levels in the chondrocytes. (h) FC was used for evaluating the apoptotic cell number. The apoptosis rate of LPS-treated chondrocytes is shown in the lower right panel. (i) ELISA was used to analyze the production of pro-inflammatory cytokines in chondrocytes. * P < 0.05.
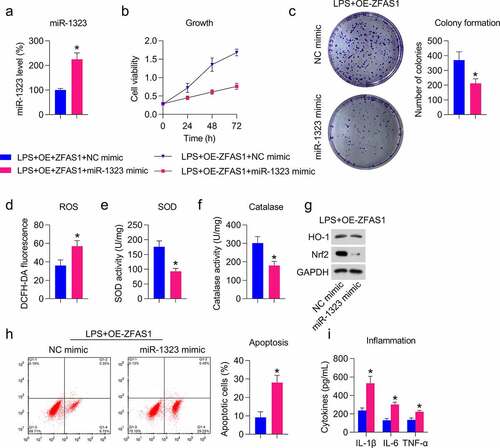
Next, we assessed the effect of miR-1323 on the oxidative stress in H2O2-treated cells overexpressing ZFAS1, in which ROS production, SOD and catalase activities, and Nrf2-HO-1 pathways were analyzed. We found that miR-1323 induced ROS production ()) but reduced SOD and catalase activities in cells with LPS stimulation and ZFAS1 overexpression ()). We also observed that Nrf2 and HO-1 protein levels were reduced after transfection with the miR-1323 mimic ()). These data suggest that miR-1323 abolished the inhibitory role of ZFAS1 on oxidative stress in LPS-treated chondrocytes.
To evaluate the role of miR-1323 mimic in cell inflammation and apoptosis of LPS-treated chondrocyte, Annexin V-FITC/PI FC and ELISA assays were performed. Noticeable apoptotic cell recovery was found in miR-1323 inhibitor transfected cells ()). Data from ELISA displayed that the accumulation of cytokines, which were reduced by ZFAS1, was promoted by miR-1323 mimic transfection ()). These data suggest that miR-1323 reverses the inhibitory role of ZFAS1 on apoptosis and inflammation in LPS-treated chondrocytes.
Nrf2 is a target of miR-1323
Considering that miR-1323 could negatively regulate the expression of Nrf2, we evaluated whether Nrf2 could be targeted by miR-1323 at the transcriptional level. First, bioinformatic analysis was performed to predict the targets of miR-1323. We found that miR-1323 targeted the 3’-UTR of Nrf2 ()). We then performed a DLRA to investigate the mechanistic connections following miR-1323-Nrf2 interaction ()). In cells transfected with the miR-1323 mimic fused to the WT Nrf2 3’-UTR, the luciferase activity was inhibited by 65% compared with control cells, confirming that miR-1323 interacts with Nrf2 ()). We next evaluated the expression of Nrf2 in LPS-treated chondrocytes transfected with the ZFAS1 overexpression vector and/or miR-1323 mimic using qRT-PCR. The results confirmed that the Nrf2 mRNA level was initially downregulated in the LPS-treated chondrocytes and then upregulated after ZFAS1 overexpression ()). However, following miR-1323 upregulation, the Nrf2 mRNA level was reduced ()). These data suggest that ZFAS1 modulates Nrf2 expression via controlling miR-1323 expression.
Figure 5. miR-1323 targets the 3′-UTR of Nrf2. (a) Graphical depiction of the conserved miR-1323 binding motif at the Nrf2 3′-UTR. (b) DLRA was conducted using the luciferase reporter constructs, which contained either the Mut or WT of Nrf2 after miR-1323/NC mimic transfection. The firefly luciferase activity was standardized to Renilla luciferase activity. (c) Chondrocytes were exposed to pcDNA3.1-empty (NC) or pcDNA3.1-ZFAS1 (ZFAS1) transfection for one day and then treated with 0.5 μM LPS for another day. qRT-PCR was conducted to assess the Nrf2 mRNA level. (d) Chondrocytes were exposed to pcDNA3.1-ZFAS1 (ZFAS1), NC/miR-1323 mimic co-transfection for one day and then treated with 0.5 μM LPS for another day. qRT-PCR was conducted to evaluate the level of Nrf2 mRNA. LPS-treated chondrocytes vs. indicated or control or LPS+OE-ZFAS1+ NC mimic group (* P < 0.05, ** P < 0.01); LPS-treated chondrocytes vs. LPS+OE-ZFAS1 + 1323 mimic group ($$ P < 0.01).

Role of miR-1323-Nrf2 crosstalk on LPS-induced oxidative stress, inflammation, and apoptosis of chondrocytes
To elucidate the effect of miR-1323-Nrf2 crosstalk on LPS-induced oxidative stress, inflammation, and apoptosis of chondrocytes, LPS-treated cells were transfected with the miR-1323 inhibitor and/or challenged with the Nrf2 inhibitor brusatol. Transfection with the miR-1323 inhibitor caused a prominent miR-1323 downregulation and upregulation of Nrf2 in LPS-exposed cells, while brusatol administration markedly repressed the Nrf2 upregulation ()). Results from the MTT assay and CFA indicated that cell growth rate and viability were promoted by miR-1323 inhibition; however, brusatol treatment depleted the beneficial effect of the miR-1323 inhibitor on cell growth ()).
Figure 6. Effect of miR-1323 downregulation and Nrf2 inhibition on growth, oxidative stress, inflammation, and apoptosis of LPS-treated chondrocytes. Chondrocytes were exposed to NC/miR-1323 inhibitor transfection for 24 h, followed by 0.5 μM LPS treatment and 1 μM brusatol treatment for 24 h. (a) The level of miR-1323 and Nrf2 mRNA was analyzed through qRT-PCR. (b) The cell growth rate on days 1, 2, and 3 was determined after transfection through MTT assay. (c) Soft agar CFA of LPS-treated chondrocytes. The colony number is displayed in the right panel. (d) DCFH-DA staining was conducted to evaluate ROS production. (e, f) Activities of the anti-oxidative enzymes SOD and catalase were determined via the corresponding assay kits. (g) WB was used to detect the HO-1 and Nrf2 protein levels in chondrocytes. (h) FC was used for evaluating the apoptotic cell number. The apoptosis rate of LPS-treated chondrocytes is shown in the lower right panel. (i) ELISA was used to analyze the production of pro-inflammatory cytokines in chondrocytes. LPS-treated chondrocytes vs. control group (* P < 0.05, ** P < 0.01, *** P < 0.001); LPS-treated chondrocytes vs. LPS+NC inhibitor group ($ P < 0.05, $$ P < 0.01); LPS-treated chondrocytes vs. LPS+miR-1323 inhibitor group (& P < 0.05, && P < 0.01, &&& P < 0.001).
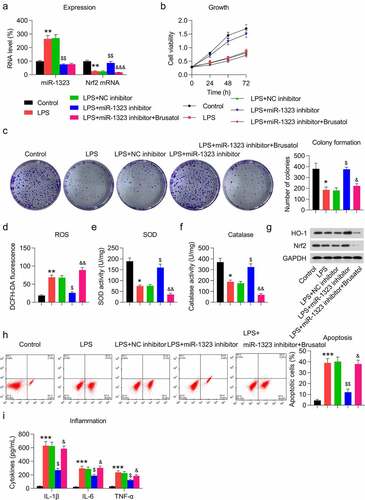
The next step was to probe the cellular oxidative stress following the miR-1323 inhibition and brusatol treatment. Similar to ZFAS1 overexpression, inhibition of miR-1323 resulted in alleviated ROS levels ()), upregulated SOD and catalase activities ()), and activated the Nrf2-HO-1 pathway ()), as expected. Notably, brusatol administration abolished the effect of miR-1323 inhibition on oxidative response to LPS stimulation ()).
We further evaluated the role of miR-1323-Nrf2 crosstalk in cell apoptosis and inflammation of LPS-treated chondrocytes. Annexin V-FITC/PI FC indicated that miR-1323 inhibition led to a noticeable reduction in apoptotic cells, while brusatol administration reversed this reduction and caused a high rate of apoptosis ()). Moreover, the expression of LPS-induced pro-inflammatory cytokines was found to be downregulated by miR-1323 inhibition. Nevertheless, in miR-1323-inhibited and LPS-treated cells, brusatol incubation contributed to cytokine accumulation ()).
Collectively, these data indicate that miR-1323 is required for LPS-induced oxidative stress, apoptosis, and inflammation in chondrocytes by mediating Nrf2 expression.
Discussion
This study explored the role of the lncRNA ZFAS1 in regulating oxidative stress-associated inflammation and apoptosis during OA progression by using LPS-induced chondrocytes. Previous studies have shown that LPS is an initial factor for inflammatory response regulation during OA pathogenesis; therefore, we used LPS to build a well-established cell-based model for in vitro investigation of OA [Citation29,Citation38]. Our study confirmed that ZFAS1 expression levels were reduced during OA and LPS-induced chondrocytes compared with non-OA and non-treated chondrocytes. ZFAS1 overexpression ameliorated LPS-induced oxidative stress. This was confirmed by reduced cellular ROS levels, elevated SOD and catalase activities, and activation of the Nrf2-HO-1 pathway. ELISA and FC assays indicated that inflammation and apoptosis triggered by LPS were also alleviated through overexpression of ZFAS1, which showed that ZFAS1 might play a regulatory role in LPS-induced chondrocyte disorders. Further experiments demonstrated that ZFAS1 exerted its effect by activating the miR-1323-Nrf2 pathway. The role of ZFAS1 in miR-1323-Nrf2 pathway activation is a novel regulatory axis of the inflammation and apoptosis triggered by LPS.
Recently, Ye et al. [focused on the influence of ZFAS1 on chondrocyte apoptosis during OA. ZFAS1 levels were decreased in OA chondrocytes relative to that in healthy chondrocytes. ZFAS1 upregulation increased the chondrocyte viability, proliferation, and migration potential and repressed apoptosis and matrix generation. Previously, ZFAS1 upregulation was shown to decrease WNT family member 3a (Wnt3a) levels. The protective effect of ZFAS1 against OA was indicated by this modulation of the Wnt3a signaling [Citation10]. Li et al. also found that ZFAS1 expression was lower, proliferation was expedited, and apoptosis was repressed in chondrocytes. These effects were found to be mediated by regulation of the miR-302d-3p-SMAD2 axis [Citation15]. Both studies did not use LPS to stimulate the generation of the OA cell model; therefore, other properties of OA, including oxidative stress and inflammation, were not investigated. Here, we confirmed the inhibitory role of ZFAS1 in OA-related oxidative stress, inflammation, and apoptosis.
LncRNAs can be utilized as ceRNAs of miRNAs [Citation39]. Hence, we further investigated whether the mechanism of ZFAS1 was driven by interacting with miR-1323. miR-1323 upregulation has been detected in radiochemotherapy-resistant esophageal squamous cell carcinoma and radiation-resistant lung cancer cells and serves as a prognostic marker for pre-radiochemotherapy patient selection [Citation24,Citation40]. miR-1323 overexpression in hepatocellular carcinoma has also been also detected and was found to be related to unfavorable disease-free and overall survival in patients with hepatocellular carcinoma [Citation41]. However, its biological roles in other diseases (i.e., OA) have not been explored. First, bioinformatic analysis and dual-luciferase reporter results showed that miR-1323 was a possible target of ZFAS1. Meanwhile, miR-1323 expression was upregulated and negatively correlated with ZFAS1 expression in LPS-induced chondrocytes. Moreover, the upregulation of miR-1323 expression could suppress the repressive function of ZFAS1 on oxidative stress, inflammation, and apoptosis of chondrocytes. This implies that miR-1323 acts as a positive modulator in OA, indicating that ZFAS1 plays a cytoprotective effect on LPS-treated chondrocytes by targeting miR-1323.
We performed loss and gain of function study to investigate whether miR-1323 affects proliferation, oxidative stress, inflammation, and apoptosis in chondrocytes. As expected, miR-1323 inhibition promoted proliferation and reduced oxidative disorder, inflammation, and apoptosis of LPS-treated chondrocytes. These effects were attenuated after administering the Nrf2 inhibitor brusatol, agreeing with our hypothesis.
Significant evidence has suggested that lncRNAs function as sponges of miRNAs to affect mRNA expression [Citation42,Citation43]. In the current study, we confirmed binding between the ZFAS1, miR-1323, and Nrf2, forming a regulatory axis. Furthermore, the OA cell model confirmed inverse correlations between the ZFAS1–miR-1323 pair and the miR-1323-Nrf2 pair. Hence, we observed a positive correlation between ZFAS1 and Nrf2. Importantly, ZFAS1 upregulated Nrf2 expression, and miR-1323 reintroduction partly mitigated the action of ZFAS1 in chondrocytes. In other words, ZFAS1 positively regulates Nrf2 by interacting with miR-1323.
Conclusion
In summary, this is the first report demonstrating that ZFAS1 could facilitate LPS-exposed chondrocyte proliferation and reduce oxidative disorder, inflammation, and apoptosis during OA, possibly by targeting the miR-1323-Nrf2 signaling axis. Thus, both ZFAS1 and miR-1323 may play a pivotal role in OA pathogenesis, providing a promising target for OA treatment. In future investigations, it would be interesting to use an OA animal model to confirm the effect of ZFAS1 on OA pathogenesis.
Abbreviation
ANOVA | = | Analysis of variance |
BCA | = | Bicinchoninic acid assay |
CFA | = | Colony formation assay |
ceRNA | = | Competing endogenous RNA |
DCFH-DA | = | Dichloro-dihydro-fluorescein diacetate |
DLRA | = | Dual-luciferase reporter assay |
DMEM | = | Dulbecco’s modified Eagle’s medium |
ELISA | = | Enzyme-linked immunosorbent assay |
ECM | = | Extracellular matrix, |
FBS | = | Fetal bovine serum |
FC | = | Flow cytometry |
GAPDH | = | Glyceraldehyde-3-phosphate dehydrogenase |
HO-1 | = | Heme oxygenase 1 |
LPS | = | Lipopolysaccharide |
lncRNA | = | Long non-coding RNA |
ZFAS1 | = | LncRNA zinc finger NFX1-type containing 1 antisense 1 |
miR | = | MicroRNA |
Mut | = | Mutant-type |
Nrf2 | = | Nuclear factor erythroid 2-related factor 2 |
OA | = | Osteoarthritis |
PBS | = | Phosphate-buffered saline |
qPCR | = | Quantitative PCR |
ROS | = | Reactive oxygen species |
RT | = | Reverse transcription |
RA | = | Rheumatoid arthritis |
SD | = | Standard deviation |
SOD | = | Superoxide dismutase |
TBST | = | Tris-buffered saline with 0.1% tween® 20 detergent |
WB | = | Western blot |
WT | = | Wild-type |
Wnt3a | = | WNT family member 3a |
ZNFX1 | = | Zinc finger NFX1-type containing 1 |
Supplemental Material
Download Zip (33.7 MB)Disclosure statement
No potential conflict of interest was reported by the author(s).
Data availability statement
The datasets generated during and/or analysed during the current study are available from the corresponding author on reasonable request.
Supplementary material
Supplemental data for this article can be accessed online at https://doi.org/10.1080/21655979.2022.2074770.
Additional information
Funding
References
- Xia B, Chen D, Zhang J, et al. Osteoarthritis pathogenesis: a review of molecular mechanisms. Calcif Tissue Int. 2014;95(6):495–505.
- Lepetsos P, Papavassiliou AG. ROS/oxidative stress signaling in osteoarthritis. Biochimica et Biophysica Acta (BBA)-Molecular Basis of Disease. Biochimica et biophysica acta. 2016;1862(4):576–591.
- Li D, Wang W, Xie G. Reactive oxygen species: the 2-edged sword of osteoarthritis. Am J Med Sci. 2012;344(6):486–490.
- Bijlsma JW, Berenbaum F, Lafeber FP. Osteoarthritis: an update with relevance for clinical practice. Lancet. 2011;377(9783):2115–2126.
- Kapoor M, Martel-Pelletier J, Lajeunesse D, et al. Role of proinflammatory cytokines in the pathophysiology of osteoarthritis. Nat Rev Rheumatol. 2011;7(1):33–42.
- Sies H. Oxidative eustress and oxidative distress: introductory remarks. Oxidative Stress: Elsevier; 2020. p. 3–12.
- Forman HJ, Fukuto JM, Torres M. Redox signaling: thiol chemistry defines which reactive oxygen and nitrogen species can act as second messengers. Am J Physiol Cell Physiol. 2004;287(2):C246–C256.
- Cen X, Huang XQ, Sun WT, et al. Long non-coding RNAs: a new regulatory code in osteoarthritis. Am J Transl Res. 2017;9(11):4747–4755. eng.
- Jiang MC, Ni JJ, Cui WY, et al. Emerging roles of lncRNA in cancer and therapeutic opportunities. Am J Cancer Res. 2019;9(7):1354–1366. eng.
- Sallam T, Sandhu J, Tontonoz P. Long Noncoding RNA Discovery in Cardiovascular Disease: decoding Form to Function. Circ Res. 2018;122(1):155–166. eng.
- Askarian-Amiri ME, Crawford J, French JD, et al. SNORD-host RNA Zfas1 is a regulator of mammary development and a potential marker for breast cancer. Rna. 2011;17(5):878–891.
- Duan R, Li C, Wang F, et al. The long non-coding RNA ZFAS1 potentiates the development of hepatocellular carcinoma via the microRNA-624/MDK/ERK/JNK/P38 signaling pathway. Onco Targets Ther. 2020;13:4431.
- Tian F, Meng F, Wang X. Overexpression of long-noncoding RNA ZFAS1 decreases survival in human NSCLC patients. Eur Rev Med Pharmacol Sci. 2016;20(24):5126–5131.
- Wang X, Hao R, Wang F, et al. ZFAS1 Promotes Cisplatin Resistance via Suppressing miR-421 Expression in Oral Squamous Cell Carcinoma. Cancer Manag Res. 2020;12:7251.
- Li J, Liu M, Li X, et al. Long non-coding RNA ZFAS1 suppresses chondrocytes apoptosis via miR-302d-3p/SMAD2 in osteoarthritis. Biosci Biotechnol Biochem. 2021;85(4):842–850.
- Hu F, Shao L, Zhang J, et al. Knockdown of ZFAS1 inhibits hippocampal neurons apoptosis and autophagy by activating the PI3K/AKT pathway via up-regulating miR-421 in epilepsy. Neurochem Res. 2020;45(10):2433–2441.
- Yang S, Yin W, Ding Y, et al. Lnc RNA ZFAS1 regulates the proliferation, apoptosis, inflammatory response and autophagy of fibroblast-like synoviocytes via miR-2682-5p/ADAMTS9 axis in rheumatoid arthritis. Biosci Rep. 2020;40(8):BSR20201273.
- Ye D, Jian W, Feng J, et al. Role of long non-coding RNA ZFAS1 in proliferation, apoptosis and migration of chondrocytes in osteoarthritis. Biomed Pharmacother. 2018;104:825–831.
- Tang X, Yin R, Shi H, et al. LncRNA ZFAS1 confers inflammatory responses and reduces cholesterol efflux in atherosclerosis through regulating miR-654-3p-ADAM10/RAB22A axis. Int J Cardiol. 2020;315:72–80.
- Aziz NB, Mahmudunnabi RG, Umer M, et al. MicroRNAs in ovarian cancer and recent advances in the development of microRNA-based biosensors. Analyst. 2020;145(6):2038–2057.
- Bartel DP. MicroRNAs: genomics, biogenesis, mechanism, and function. Cell. 2004;116(2):281–297.
- Low SS, Pan Y, Ji D, et al. Smartphone-based portable electrochemical biosensing system for detection of circulating microRNA-21 in saliva as a proof-of-concept. Sens Actuators B Chem. 2020;308:127718.
- Wang J, Liu S, Li J, et al. Roles for miRNAs in osteogenic differentiation of bone marrow mesenchymal stem cells. Stem Cell Res Ther. 2019;10(1):197.
- Li Y, Han W, Ni -T-T, et al. Knockdown of microRNA-1323 restores sensitivity to radiation by suppression of PRKDC activity in radiation-resistant lung cancer cells. Oncol Rep. 2015;33(6):2821–2828.
- Liu L, Zhang J, Liu Y. MicroRNA‑1323 serves as a biomarker in gestational diabetes mellitus and aggravates high glucose‑induced inhibition of trophoblast cell viability by suppressing TP53INP1. Exp Ther Med. 2021;21(3):1.
- Xu Y, Liu M. MicroRNA-1323 downregulation promotes migration and invasion of breast cancer cells by targeting tumour protein D52. J Biochem. 2020;168(1):83–91.
- Zhang F, Yang C, Xing Z, et al. LncRNA GAS5-mediated miR-1323 promotes tumor progression by targeting TP53INP1 in hepatocellular carcinoma. Onco Targets Ther. 2019;12:4013.
- Zhao H, Zheng C, Wang Y, et al. miR-1323 promotes cell migration in lung adenocarcinoma by targeting Cbl-b and is an early prognostic biomarker. Front Oncol. 2020;10:181.
- Scotece M, Conde J, Abella V, et al. Oleocanthal inhibits catabolic and inflammatory mediators in LPS-activated human primary osteoarthritis (OA) chondrocytes through MAPKs/NF-κB pathways. Cell Physiol Biochem. 2018;49(6):2414–2426.
- Zhang H, Ge J, Lu X. CircFADS2 is downregulated in osteoarthritis and suppresses LPS-induced apoptosis of chondrocytes by regulating miR-195-5p methylation. Arch Gerontol Geriatr. 2021;96:104477.
- Zhou Y, Chen X, Qu N, et al. Chondroprotection of PPARα activation by WY14643 via autophagy involving Akt and ERK in LPS‐treated mouse chondrocytes and osteoarthritis model. J Cell Mol Med. 2019;23(4):2782–2793.
- Eruslanov E, Kusmartsev S Identification of ROS using oxidized DCFDA and flow-cytometry. In: Advanced protocols in oxidative stress II. Methods Mol Biol: Springer; 2010. p. 57–72.
- Zhou T, Li Z, Chen H. Melatonin alleviates lipopolysaccharide (LPS)/adenosine triphosphate (ATP)-induced pyroptosis in rat alveolar Type II cells (RLE-6TN) through nuclear factor erythroid 2-related factor 2 (Nrf2)-driven reactive oxygen species (ROS) downregulation. Bioengineered. 2022;13(1):1880–1892.
- M-j Y, Meng N. Resveratrol acts via the mitogen-activated protein kinase (MAPK) pathway to protect retinal ganglion cells from apoptosis induced by hydrogen peroxide. Bioengineered. 2021;12(1):4878–4886.
- Jian Z, Guo H, Liu H, et al. Oxidative stress, apoptosis and inflammatory responses involved in copper-induced pulmonary toxicity in mice. Aging (Albany NY). 2020;12(17):16867–16886. eng.
- Redza-Dutordoir M, Averill-Bates DA. Activation of apoptosis signalling pathways by reactive oxygen species. Biochimica et Biophysica Acta (BBA)-Molecular Cell Research. Biochimica et biophysica acta. 2016;1863(12):2977–2992.
- Zuo L, Prather ER, Stetskiv M, et al. Inflammaging and oxidative stress in human diseases: from molecular mechanisms to novel treatments. Int J Mol Sci. 2019;20(18):4472.
- Sun T, Li X, Song H, et al. MiR-146a aggravates LPS-induced inflammatory injury by targeting CXCR4 in the articular chondrocytes. Cell Physiol Biochem. 2017;44(4):1282–1294.
- Jalali S, Bhartiya D, Lalwani MK, et al. Systematic transcriptome wide analysis of lncRNA-miRNA interactions. PloS one. 2013;8(2):e53823.
- Slotta-Huspenina J, Drecoll E, Feith M, et al. MicroRNA expression profiling for the prediction of resistance to neoadjuvant radiochemotherapy in squamous cell carcinoma of the esophagus. J Transl Med. 2018;16(1):1–9.
- Law PT-Y, Qin H, Ching A-K-K, et al. Deep sequencing of small RNA transcriptome reveals novel non-coding RNAs in hepatocellular carcinoma. J Hepatol. 2013;58(6):1165–1173.
- Wang P, Li J, Zhao W, et al. A novel LncRNA-miRNA-mRNA triple network identifies LncRNA RP11-363E7. 4 as an important regulator of miRNA and gene expression in gastric Cancer. Cell Physiol Biochem. 2018;47(3):1025–1041.
- Yue B, Li H, Liu M, et al. Characterization of lncRNA–miRNA–mRNA network to reveal potential functional ceRNAs in bovine skeletal muscle. Front Genet. 2019;10:91.