ABSTRACT
This study aims to investigate the immunoglobulin superfamily containing leucine-rich repeat (ISLR) expression in gastric cancer (GC) and ISLR’s underlying mechanisms regulation of GC progression. Through The Cancer Genome Atlas (TCGA) cohort datasets, we analyzed the ISLR expression in GC tumor tissues and normal tissues. ISLR expression in GC tissues and cells was determined using quantitative real-time polymerase chain reaction. Cell viability, proliferation, migration, and invasion assays were performed in GC cells transfected with sh-ISLR, ISLR plasmids, or controls. TCGA results showed that ISLR expression was higher in GC tumor tissues compared to normal tissues, and its expression levels were related to lymph node metastasis, tumor size, and clinical stage. ISLR was highly expressed in tumor cells. ISLR knockdown suppressed cell viability, proliferation, migration, and invasion in HGC-27 cells, whereas ISLR overexpression led to opposite effects in AGS cells. Gene Set Enrichment Analysis showed that ISLR could activate the epithelial–mesenchymal transition (EMT) signaling pathway. Silencing of ISLR suppressed EMT in HGC-27 cells and overexpression of ISLR promoted EMT in AGS cells. ISLR was overexpressed in both GC cell lines and tumor tissues, and our study first showed that silencing of ISLR inhibited GC cell growth and metastasis by reversing EMT.
GRAPHICAL ABSTRACT
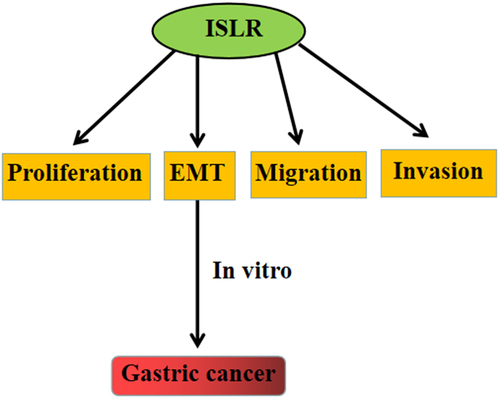
Highlights
ISLR expression is up-regulated in gastric cancer tissues and cells.
ISLR promotes gastric cancer cell proliferation, migration, and invasion.
ISLR promotes the activation of epithelial–mesenchymal transition.
Introduction
Gastric cancer (GC) is one of the most common primary malignant tumors, and it is the third principal cause of cancer death, accounting for 9% of fatality in world-wide [Citation1,Citation2]. The incidence and prevalence rates of GC vary by geographical location and are highest in Central and East Asia, Latin America, and Eastern Europe, where 87% of new cases occur, and are much lower in Africa and North America [Citation3,Citation4]. GC is a highly heterogeneous disease with molecular and phenotypic characteristics, and endoscopic resection is the most effective treatment for GC [Citation5,Citation6]. In the last 10 years, the incidence of GC has remarkably decreased, but the incidence rates are still high in many developing countries [Citation7]. Although great progress has been made in the early detection, diagnosis, and treatment of GC, the overall prognosis of GC patients remains poor, and the 5-year survival rate is still about 20.25%, which is mainly because of postoperative recurrence and metastasis of tumor [Citation8,Citation9]. Tumor metastasis and invasiveness are complex and multifactorial process. Therefore, a comprehensive study of the molecular mechanisms that facilitate the evolvement of GC may contribute to improve prevention, diagnosis, and therapy of GC.
The human immunoglobulin superfamily containing leucine-rich repeat (ISLR), a glycosylphosphatidylinositol (GPI)-anchored membrane protein, is a newly discovered family of proteins that covers a leucine-rich repeat (LRR) sequence and immunoglobulin-like domain [Citation10,Citation11]. The ISLR gene with 2.4-kb transcript situates to human chromosome 15q23-q24, and multiple genetic disorders involving this gene have been identified through link analysis [Citation12]. Genome sequencing showed that there were two transcripts of ISLR gene, namely ISLR-1 and ISLR-2, which were stemmed from different first exons 1b or 1a, the expression of ISLR-1 in spinal cord was higher than that of ISLR-2 [Citation10]. Some studies have reported ISLR protein participates in many kinds of biological processes [Citation13–15]. Recently, Kobayashi et al. concluded that ISLR expression was increased in the stroma of patients with colorectal cancer involving colorectal carcinogenesis [Citation13]. In addition, a recent study has reported that ISLR gene takes part in GC occurrence, in which latent molecular mechanism may induce epithelial–mesenchymal transition (EMT) [Citation16]. EMT is a good embryological process and is considered to play a key role in tumor development, including metastasis and invasiveness; thus, cancer cells can obtain more aggressive characteristics [Citation17–19]. However, no study has explored the potential and detailed functional relevance of ISLR and EMT in GC.
In our study, we hypothesized that ISLR may be an important player in GC. Therefore, this work aimed to investigate the biological impact of ISLR on GC cells and its potential mechanisms.
Materials and methods
Clinical samples
Tissue samples were collected from 27 GC patients (12 cases stage I/II, 15 cases stage III/IV) from our hospital between May 2017 and December 2019. None of the patients underwent preoperative radiation or chemotherapy. Tissue samples were directly frozen and preserved in liquid nitrogen (−80°C). The study was approved by the Ethics and Research Committees of Yantaishan Hospital (approval number: 201705011), and each patient completed the written informed consent.
Cell culture
Human GC cell lines (HGC-27, MKN-45, MGC-803, and AGS), normal gastric mucosa epithelial cells (GES-1), and embryonic renal epithelial cell line (293 T) were offered by China Infrastructure of Cell Line Resources (Beijing, China), and were hatched using RPMI‑1640 medium (Thermo Fisher Scientific, Waltham, USA) with 10% fetal bovine serum (FBS) in a 37°C 5% CO2 of incubator (Thermo Fisher Scientific). The medium was renewed every 2–3 days [Citation20].
Cell transfection
Short hairpin RNA against ISLR (sh-ISLR), negative control shRNA (shRNA-NC), and ISLR plasmids were designed and packaged into lentiviral vector by GenePharma (Shanghai, China). According to the manufacturers’ instructions of Lipofectamine 2000 (Invitrogen, Thermo, USA), the 293 T cells were transfected with lentiviral vector and packaging plasmids. After transfection for 48 h, HGC-27 and AGS cells were inoculated and cultivated in 24-well plates for 24 h. Once to 70–80% confluency, 1 × 105 HGC-27 cells were transfected with lentivirus that carries shISLR-1, shISLR-2, or shRNA-NC to establish ISLR downregulated cell model; meanwhile, 1 × 105 AGS cells were transfected with lentivirus carrying ISLR plasmids or vector to construct ISLR-upregulated cells model. Cells with >80% transfection efficiency were used for the subsequent experiments [Citation21].
Quantitative real-time polymerase chain reaction (qRT-PCR)
RNA was extracted by an RNA kit (Takara, Japan), and it reversed into cDNA via a reverse transcription kit (Takara, Japan). Afterward, cDNA was amplified in a reaction mixture (Applied Biosystems, USA) and 10 μL SYBR-Green qPCR Mix. RT-PCR was conducted on Applied Biosystems 7500 Sequence Detection system (ABI, USA) as follows: predenaturation at 96°C for 3 min; denaturation at 96°C for 15 s; and annealing at 58°C for 30 s, 40 cycles. The primer sequences were as follows: ISLR-forward (F), 5’-GAAGAGGGCCTATTTCCCAT-3’, ISLR-reverse (R), 5’-GCCTTCCATCTGTTGCTGCG-3’; glyceraldehyde-3-phosphate dehydrogenase (GAPDH)-F, 5’-TCCTCTGACTTCAACAGCGACAC-3’; GAPDH-R, 5’-CACCCTGTTGCTGTAGCCAAATTC-3’ [Citation22].
Cell viability
The cell viability was tested by Cell Counting kit-8 (CCK-8) assay (Beyotime Biotechnology Co., Ltd., Shanghai, China). Approximately 1 × 104 HGC-27 and AGS cells were inoculated and cultured in 96-well plates (100 μL medium/well) at 37°C. Next, the CCK-8 solution (10 μL) was supplemented into per well. The absorbance (450 nm) was detected by Thermomax microplate reader (Thermo Fisher Scientific) at 0, 24, 48, and 72 h to plot the growth curves [Citation23].
Plate cloning experiment
After 48 h of transfection, according to the concentration of 200 HGC-27 and AGS cells per well, 5 ml cell suspension was inoculated into Petri dishes (diameter 60 mm) and cultured at 37°C and 5% CO2 for 2 weeks. The culture medium was replaced with a fresh culture medium every 2–3 days. Once visible colonies appeared in per well, cells were carefully washed twice with PBS, fixed with 4% formaldehyde for 15 min, and stained with 0.05% crystal violet (Beyotime Biotechnology Co., Ltd) for 15 min. After the plates were flushed 3 times with PBS, the colonies with ≥50 cells were directly photographed by cameras and counted by Image J software (Bethesda, MD, USA) [Citation24].
Wound-healing assay
Firstly, we digested the transfected HGC-27 and AGS cells by trypsin, then 50 μL of cell suspension (5 × 105 cells/ml) was added into per well of a 6-well plate. Then, once the fusion degree of cells achieved 80%, a 200 µL pipette tip was used to draw a horizontal line in per well. Finally, the cell movement was observed and photographed by a microscope (Olympus Corp.) at 48 h after scratching to evaluate healing rate [Citation25].
Transwell assay
The cell invasiveness and migration capabilities were assessed by 8 μm porous membranes of transwell chambers coated with or without Matrigel (BD Biosciences, NJ, USA). 5 × 105 cells/mL of HGC-27 or AGS cells cultured in RPMI-1640 medium was seeded onto the upper chamber of the Transwell insert. In the lower chamber, RPMI-1640 medium containing 10% FBS was supplemented. 48 h later, cells were flushed with PBS for 3 times, fixed with 4% paraformaldehyde, and stained with 0.2% crystal violet (Beyotime Biotechnology Co., Ltd). Finally, the images were captured by an inverted microscope (Nikon, Tokyo, Japan) [Citation26].
Western blotting
Cell and tissue samples were lysed using RIPA lysis buffer (Beyotime Biotechnology Co., Ltd) for 30 min. The proteins were separated by 10% sodium dodecyl sulfate-polyacrylamide gel electrophoresis. The specific protein was transferred to a polyvinylidene fluoride membrane. After blocking with 5% nonfat dry milk for 30 min, specific proteins were incubated with primary antibodies [ISLR, GAPDH, Snail, Twist, E-cadherin, Vimentin, N-cadherin, biglycan (BGN), matrix metalloproteinase (MMP)-2, and secreted frizzled related protein 4 (SFRP4); 1:1000, Sigma, USA] overnight at 4°C. After three washes with Tris-buffered saline with 0.1% Tween 20 detergent, the membranes were incubated with horseradish peroxidase conjugated secondary antibodies for 1 h. Finally, enhanced chemiluminescence reagents (GE Healthcare, WI, USA) were used to visualize the protein signals [Citation27].
The Cancer Genome Atlas (TCGA) data for human GC
The relation between ISLR expression and cancer stages was retrieved from UALCAN (http://ualcan.path.uab.edu/) by analyzing the clinicopathological data available from the TCGA project for human GC. The Kaplan–Meier method was performed to analyze the underlying function of ISLR on overall survival (OS) rates of GC patients [Citation28].
Gene set enrichment analysis (GSEA)
GSEA was performed to analyze the gene set associated with ISLR in GC. According to the median expression value, the expression of ISLR was set to high and low. The related genes and pathways of ISLR were researched in c2.cp.kegg.v7.0.symbols.gmt dataset through GSEA v3 software. Falseovery rate less than 0.25 was used to identify significantly enriched genes [Citation29].
Statistical analysis
All data were analyzed using GraphPad Prism 7 (USA). All tests were made in triplicate. Data are presented as the mean ± standard deviation. Differences between the two groups and among multiple groups were assessed using Student’s t-test and analysis of variance, respectively. The relationship between the ISLR expression and the clinicopathological characteristics of GC patients was estimated by chi-square test. Statistical significance was set at P < 0.05.
Results
In this study, we investigated the influence of ISLR on the biological behavior of GC cells and its potential mechanisms. Our findings showed that ISLR promoted GC cell proliferation, migration, and invasion by regulating the EMT pathway.
ISLR is upregulated in GC patients
To evaluate the ISLR expression in GC, we analyzed the TCGA stomach adenocarcinoma (STAD) cohort datasets. Our results indicated that the ISLR expression in GC tumor tissues was higher compared with that in normal tissues (). In addition, ISLR expression in patients with stage 1 was decreased when confronted with that in normal tissues, while ISLR expression in patients with stage 2/3/4 significantly increased (). Additionally, according to the expression level of the ISLR in GC cases in the TCGA-STAD cohort, OS analysis was performed using the Kaplan–Meier method. A group cutoff of ‘quartile’ was used to divide samples as high- or low-expression group. Low-expression group includes 39 stage I patients, 64 stage II patients, 92 stage III patients, 25 stage IV patients, and 6 unknown stage. High-expression group includes 8 stage I patients, 42 stage II patients, 46 stage III patients, 10 stage IV patients, and 9 unknown stage. As shown in , GC patients with high ISLR expression had poorer OS than that of GC patients with low ISLR expression (hazard ratio = 1.1, 95% CI: 1–1.2, p = 0.027). Then, we detected ISLR expression in 27 GC patients through qRT-PCR and found that GC tissues showed significantly higher ISLR expression than that in normal tissues (). The protein level of ISLR was higher in tumor tissues than in normal tissues of five GC patients, as detected by western blotting (). To explore the clinical value of ISLR expression in GC, we analyzed the correlations between the mRNA expression of ISLR and the clinicopathological characteristics of GC patients. Based on the median value of ISLR expression, we divided GC patients into high ISLR expression group (n = 13) and low ISLR expression group (n = 14). As shown in , ISLR expression was correlated with tumor size, lymph node metastasis, and clinical stage, while ISLR expression had no relation to age or gender.
Table 1. Correlations between ISLR expression and clinicopathological characteristics of gastric cancer
Figure 1. ISLR was up-regulated expression in gastric cancer. (a) The TCGA-STAD datasets indicated ISLR expression in GC tumor tissues was higher compared with that in normal tissues. (b) The ISLR expression in normal tissues and stage 1/2/3/4 of STAD. (c) As Kaplan–Meier survival plots shown, the higher ISLR abundance had relation to a poorer overall survival. (d) The mRNA expression of ISLR in tumor tissues and adjacent normal tissues of 27 patients with gastric cancer was detected using qRT-PCR. (e) Western blotting was used to detect the protein expression of ISLR in tumor tissues and adjacent normal tissues from 5 patients with gastric cancer. **P < 0.01, compared with normal tissues. T, gastric cancer tissue; N, adjacent normal tissues.
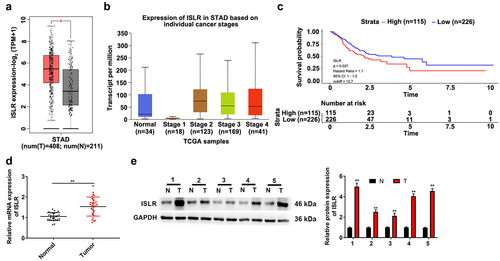
ISLR silencing suppresses the proliferation of GC cells
The mRNA expression of ISLR in different GC cell lines was determined by qRT-PCR. The results suggested that ISLR was highly expressed in tumor cell lines (HGC-27, MKN-45, MGC-803, and AGC cells), with the expression in HGC-27 cells being the most pronounced and that in AGS cells being the lowest (). Afterward, ISLR was silenced in HGC-27 cells and overexpressed in AGS cells, which was certified by qRT-PCR (). The CKK-8 and colony formation assays clearly manifested that ISLR silencing prohibited the viability and proliferation of HGC-27 cells, nevertheless ISLR overexpression facilitated the viability and proliferation of AGS cells ().
Figure 2. ISLR silencing suppressed gastric cancer cell proliferation. (a) The mRNA expression of ISLR in human gastric cancer cell lines (HGC-27, MKN-45, MGC-803, and AGS) and normal human gastric mucosa epithelial cells (GES-1) was tested using qRT-PCR. (b) HGC-27 cells were transfected with ShISLR-1, ShISLR-2, or ShRNA-NC and AGS cells were transfected with ISLR plasmids or vector, then the mRNA expression of ISLR was examined in HGC-27 and AGS cells using qRT-PCR. (c) Cell viability of HGC-27 and AGS cells was evaluated using CKK8 assay. (d) Cell proliferation of HGC-27 and AGS cells was evaluated using colony formation assay. **P < 0.01, compared with GES-1, shRNA-NC, or Vector group.
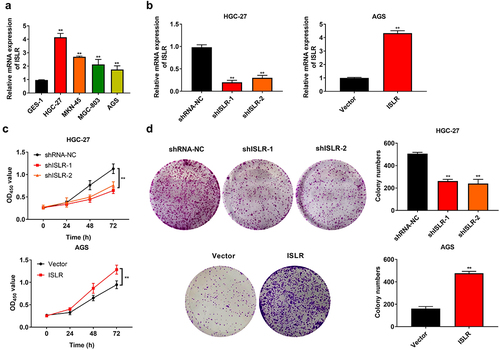
ISLR silencing suppresses the migration and invasion of GC cells
Wound healing and transwell assay was carried out to determine the role of ISLR in GC cell metastasis and invasiveness. ISLR silencing evidently weakened the adhesion ability of HGC-27 cells, whereas ISLR overexpression had the opposite effect on AGS cells (). ISLR downregulation obviously restrained cell migration and invasion in HGC-27 cells, while the opposite effect was confirmed in AGS cells with ISLR overexpression ().
Figure 3. ISLR silencing suppressed migration and invasion in gastric cancer cells. (a) Wound healing was used to detect cell migration in HGC-27 and AGS cells. (b) Transwell assay was used to detect cell migration in HGC-27 and AGS cells. (c) Transwell assay was performed to detect cell invasion in HGC-27 and AGS cells. **P < 0.01, compared with shRNA-NC or Vector group.
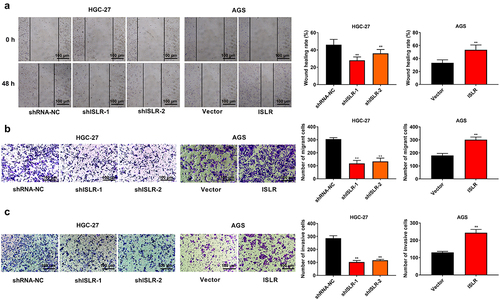
ISLR promotes EMT activation
To probe the latent mechanisms of ISLR in GC progression, the KEGG downstream pathway of ISLR was evaluated by GSEA. The findings showed that ISLR expression could activate the EMT signaling pathway (). Furthermore, many EMT downstream enrichment genes were obtained (). Based on TCGA database, the expression correlation between ISLR gene and EMT markers was analyzed. We found that the ISLR expression was positively correlated with the expression of Snail, Twist, Vimentin, and N-cadherin (also known as CDH2) (), while ISLR expression was inversely correlated with E-cadherin (also known as CDH1) expression ().
Figure 4. ISLR promotes EMT activation. (a) GESA analysis showed that ISLR regulated HALLMARK_EPITHELIAL_MESENCHYMAL_TRANSITION signaling pathway. (b) EMT downstream enrichment genes. (c-g) According to TCGA database, the expression correlation between ISLR gene and EMT markers [Snail, Twist, Vimentin, N-cadherin (also known as CDH2), and E-cadherin (also known as CDH1)] was analyzed.
![Figure 4. ISLR promotes EMT activation. (a) GESA analysis showed that ISLR regulated HALLMARK_EPITHELIAL_MESENCHYMAL_TRANSITION signaling pathway. (b) EMT downstream enrichment genes. (c-g) According to TCGA database, the expression correlation between ISLR gene and EMT markers [Snail, Twist, Vimentin, N-cadherin (also known as CDH2), and E-cadherin (also known as CDH1)] was analyzed.](/cms/asset/77f2654a-364e-4bc2-a2ab-1407907050d1/kbie_a_2079303_f0004_oc.jpg)
ISLR silencing suppresses EMT
The changes of expression level of proteins related to EMT signaling pathway and some EMT downstream enrichment genes were evaluated by western blotting. Just as expected, ISLR silencing led to a statistically remarkable increase in E-cadherin protein expression and resulted in a dramatic decrease in the expression of Snail, Twist, Vimentin, and N-cadherin, and BGN, MMP-2, and SFRP4 in HGC-27 cells. Rather, the overexpressed ISLR had a counter influence on the expression of those proteins in AGS cells (). Thus, ISLR silencing suppresses EMT in GC.
Figure 5. ISLR silencing suppressed EMT signaling pathway. (a) Western blot assay was applied to detect the expression of EMT-related proteins (Snail, Twist, Vimentin, N-cadherin, and E-cadherin) in HGC-27 and AGS cells. (b) Western blot assay was used to detect the protein expression of EMT pathway downstream enrichment genes (BGN, MMP-2, and SFRP4) in HGC-27 cells and AGS cells. **P < 0.01, compared with shRNA-NC or Vector group.
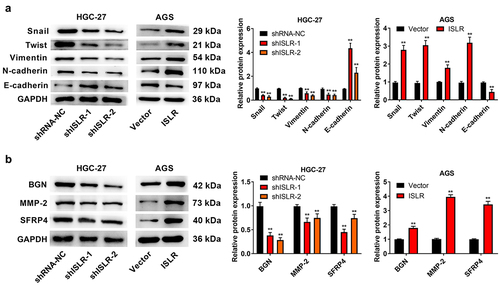
Discussion
GC is usually diagnosed at advanced stage, and recurrence and metastasis are the greatest challenges in the treatment process [Citation30]. Although environmental factors, such as Helicobacter pylori infection, smoking, and diet, have been proven to be risk factors, host genetics also play a key role in GC occurrence [Citation31,Citation32]. The multiformity and role of host genetics in the transcriptional active part of the genome and abnormalities in a number of cancers, including GC, have been confirmed in many studies, prompting research to identify molecular candidates for diagnosis and treatment [Citation33]. In this research, we found that ISLR expression in GC tissues was higher than that in normal tissues. In addition, ISLR expression was correlated with tumor size, lymph node metastasis, and clinical stage. Collectively, these findings suggest the potential impact of ISLR on the etiology of GC.
ISLR is detected in muscle tissues and satellite cells, and regulates the muscle regeneration and muscle atrophy [Citation34,Citation35]. Cui et al. have reported that ISLR plays a significant role in the differentiation of myoblasts, alleviating skeletal muscle atrophy and preventing myocyte apoptosis through IGF/PI3K/AKT-FOXO signaling pathway [Citation34]. Xu et al. and Kobayashi et al. have verified that ISLR is crucial for regulating the growth of epithelial cells during intestinal tumourigenesis, particularly in colorectal carcinoma [Citation36,Citation37]. In our study, the results showed that ISLR silencing inhibited cell proliferation, migration, and invasion in HGC-27 cells, whereas ISLR overexpression in AGS cells had the opposite effects.
In recent years, numerous molecular markers, including miRNAs, long noncoding RNAs, and oncogenic ncRNAs, involved in EMT have been shown to manage most cellular processes, leading to the development of many cancers, including GC [Citation3,Citation38–42]. In clinical practice, EMT is associated with a poor prognosis in cancer patients [Citation43]. Invasion and metastasis of tumor cells are the primary causes of death in patients with GC. Accumulating evidences indicates that EMT plays an important role in intercellular adhesion, metastasis, and invasiveness, and is closely related to the adverse clinical outcomes of GC patients [Citation44]. In 2020, Shu et al. reported that ISLR expression was relevant to the activation of the EMT pathway, according to GSCAlite pathway score analysis [Citation16]. In our study, GSEA showed that ISLR could activate the EMT signaling pathway. The expression of collagen, vimentin, fibronectin, and N-cadherin was increased, and expression of E-cadherin was reduced in EMT, leading to cell metastasis and invasiveness [Citation44]. The transcription regulators such as Slug, β-catenin, Snail, and Twist were up-regulated in EMT [Citation45]. MMPs are a class of zinc containing enzymes that can decrease extracellular matrix and promote cell invasion. BGN, as a constituent of ECM, is considered a new mesenchymal marker for the EMT, and is overexpressed in GC tissues and expedited metastasis [Citation46]. SFRP4 can drive GC invasion and is an early predictor of GC recrudescence [Citation47]. Our findings indicated that ISLR silencing led to a remarkable upregulation of E-cadherin and downregulation of Snail, Twist, Vimentin, and N-cadherin, as well as EMT downstream enrichment genes (BGN, MMP-2, and SFRP4). However, the overexpressed ISLR had contrary influence on the expression of those proteins.
Conclusion
We observed that ISLR expression was increased in both GC cells and tumor tissues, and our study, for the first time, indicated that ISLR silencing inhibited cell growth and metastasis by regulating the EMT pathway. Thus, SLR silencing expression may have important implications in GC therapy. Our results provided evidence that ISLR induces EMT and promotes invasion and metastasis in GC cells.
Availability of data and material
The datasets used and analyzed during the current study are available from the corresponding author on reasonable request.
Consent for publication
The authors have read the manuscript and agreed to publish
Ethics approval and consent to participate
The protocol of this research has been approved by the Ethics Committee of Yantaishan Hospital (approval number: 201705011). All patients have signed written informed consent.
Author contributions
Aitao Sun designed the study; Aitao Sun and JinBo Li performed the research; Weijing Kong and Xiaodong Jiang analyzed data; Aitao Sun wrote the paper. All authors have read and approved the manuscript
Supplemental Material
Download Zip (19.7 KB)Disclosure statement
No potential conflict of interest was reported by the author(s).
Supplementary material
Supplemental data for this article can be accessed online at https://doi.org/10.1080/21655979.2022.2079303
Additional information
Funding
References
- Smyth EC, Nilsson M, Grabsch HI, et al. Gastric cancer. Lancet. 2020;396(10251):635–648.
- Petryszyn P, Chapelle N, Matysiak-Budnik T. Gastric cancer: where are we heading? Dig Dis. 2020;38(4):280–285.
- Bure IV, Nemtsova MV, Zaletaev DV. Roles of E-cadherin and noncoding RNAs in the epithelial-mesenchymal transition and progression in gastric cancer. Int J Mol Sci. 2019;20(12):2870.
- Wang J, Zhang M, Hu X, et al. miRNA-194 predicts favorable prognosis in gastric cancer and inhibits gastric cancer cell growth by targeting CCND1. FEBS Open Bio. 2021;11(7):1814–1826.
- Rawla P, Barsouk A. Epidemiology of gastric cancer: global trends, risk factors and prevention. Prz Gastroenterol. 2019;14(1):26–38.
- Johnston FM, Beckman M. Updates on management of gastric cancer. Curr Oncol Rep. 2019;21(8):67.
- Camargo MC, Figueiredo C, Machado JC. Review: gastric malignancies: basic aspects. Helicobacter. 2019;24(S1):e12642.
- Suzuki H, Oda I, Abe S, et al. High rate of 5-year survival among patients with early gastric cancer undergoing curative endoscopic submucosal dissection. Gastric Cancer. 2016;19(1):198–205.
- Li D, Cheng P, Wang J, et al. IRF6 is directly regulated by ZEB1 and ELF3, and predicts a favorable prognosis in gastric cancer. Front Oncol. 2019;9:220.
- Nagasawa A, Kudoh J, Noda S, et al. Human and mouse ISLR (immunoglobulin superfamily containing leucine-rich repeat) genes: genomic structure and tissue expression. Genomics. 1999;61(1):37–43.
- Nagasawa A, Kubota R, Imamura Y, et al. Cloning of the cDNA for a new member of the immunoglobulin superfamily (ISLR) containing leucine-rich repeat (LRR). Genomics. 1997;44(3):273–279.
- Lind L, Sandstrom H, Wahlin A, et al. Localization of the gene for congenital dyserythropoietic anemia type III, CDAN3, to chromosome 15q21-q25. Hum Mol Genet. 1995;4(1):109–112.
- Homma S, Shimada T, Hikake T, et al. Expression pattern of LRR and Ig domain-containing protein (LRRIG protein) in the early mouse embryo. Gene Expression Patterns GEP. 2009;9(1):1–26.
- Yoon IK, Kim HK, Kim YK, et al. Exploration of replicative senescence-associated genes in human dermal fibroblasts by cDNA microarray technology. Exp Gerontol. 2004;39(9):1369–1378.
- Lugowska A, Hetmanczyk-Sawicka K, Iwanicka-Nowicka R, et al. Gene expression profile in patients with gaucher disease indicates activation of inflammatory processes. Sci Rep. 2019;9(1):6060.
- Li S, Zhao W, Sun M. An analysis regarding the association between the ISLR gene and gastric carcinogenesis. Front Genet. 2020;11:620.
- Yue B, Song C, Yang L, et al. METTL3-mediated N6-methyladenosine modification is critical for epithelial-mesenchymal transition and metastasis of gastric cancer. Mol Cancer. 2019;18(1):142.
- Huang X, Chen C, Xu Y, et al. Infiltrating T-cell abundance combined with EMT-related gene expression as a prognostic factor of colon cancer. Bioengineered. 2021;12(1):2688–2701.
- Cui X, Shan T, Qiao L. Collagen type IV alpha 1 (COL4A1) silence hampers the invasion, migration and epithelial–mesenchymal transition (EMT) of gastric cancer cells through blocking Hedgehog signaling pathway. Bioengineered. 2022;13(4):8972–8981.
- Wang X, Li Y, Fang Z, et al. Elevated expression of NFE2L3 promotes the development of gastric cancer through epithelial-mesenchymal transformation. Bioengineered. 2021;12(2):12204–12214.
- Dalby B, Cates S, Harris A, et al. Advanced transfection with Lipofectamine 2000 reagent: primary neurons, siRNA, and high-throughput applications. Methods. 2004;33(2):95–103.
- Livak KJ, Schmittgen TD. Analysis of relative gene expression data using real-time quantitative PCR and the 2−ΔΔCT method. Methods. 2001;25(4):402–408.
- Yang J, Fan L, Liao X, et al. CRTAC1 (Cartilage acidic protein 1) inhibits cell proliferation, migration, invasion and epithelial-mesenchymal transition (EMT) process in bladder cancer by downregulating Yin Yang 1 (YY1) to inactivate the TGF-β pathway. Bioengineered. 2021;12(2):9377–9389.
- Zhao N, Wang C, Guo P, et al. CCDC106 promotes the proliferation and invasion of ovarian cancer cells by suppressing p21 transcription through a p53-independent pathway. Bioengineered. 2022;13(4):10957–10973.
- Yu Y, Wang W, Lu W, et al. Inhibin β-A (INHBA) induces epithelial-mesenchymal transition and accelerates the motility of breast cancer cells by activating the TGF-β signaling pathway. Bioengineered. 2021;12(1):4681–4696.
- Zhong X, Cai Y. Long non-coding RNA (lncRNA) HOXD-AS2 promotes glioblastoma cell proliferation, migration and invasion by regulating the miR-3681-5p/MALT1 signaling pathway. Bioengineered. 2021;12(2):9113–9127.
- Wang J-S, Wang M-J, Lu X, et al. Artesunate inhibits epithelial-mesenchymal transition in non-small-cell lung cancer (NSCLC) cells by down-regulating the expression of BTBD7. Bioengineered. 2020;11(1):1197–1207.
- Therneau TM, Lumley T. Package ‘survival’. R Top Doc. 2015;128:28–33.
- Aea S. Gene set enrichment analysis: a knowledge-based approach for interpreting genome-wide expression profiles. Proc Natl Acad Sci U S A. 2005;102(43):15545–15550.
- Wu CE, Xue WW, Zhuang YW, et al. A clinical study on the efficacy of Yiqi Huayu Jiedu decoction for reducing the risk of postoperative recurrence and metastasis of gastric cancer: protocol for a multicenter, randomized, double-blind, placebo-controlled trial. Medicine (Baltimore). 2020;99(48):e23417.
- Sandoval-Borquez A, Saavedra K, Carrasco-Avino G, et al. Noncoding genomics in gastric cancer and the gastric precancerous cascade: pathogenesis and biomarkers. Dis Markers. 2015;2015:503762.
- Jia ZF, Zhang SL, Cao XY, et al. Interaction between Helicobacter pylori and host genetic variants in gastric carcinogenesis. Future Oncol. 2016;12(18):2127–2134.
- Tanikawa C, Kamatani Y, Toyoshima O, et al. Genome-wide association study identifies gastric cancer susceptibility loci at 12q24.11-12 and 20q11.21. Cancer Sci. 2018;109(12):4015–4024.
- Cui C, Han S, Shen X, et al. ISLR regulates skeletal muscle atrophy via IGF1-PI3K/Akt-Foxo signaling pathway. Cell Tissue Res. 2020;381(3):479–492.
- Zhang K, Zhang Y, Gu L, et al. Islr regulates canonical Wnt signaling-mediated skeletal muscle regeneration by stabilizing dishevelled-2 and preventing autophagy. Nat Commun. 2018;9(1):5129.
- Xu J, Tang Y, Sheng X, et al. Secreted stromal protein ISLR promotes intestinal regeneration by suppressing epithelial Hippo signaling. EMBO J. 2020;39(7):e103255.
- Kobayashi H, Gieniec KA, Wright JA, et al. The balance of stromal BMP signaling mediated by GREM1 and ISLR drives colorectal carcinogenesis. Gastroenterology. 2020;160(4): 1224–1239.
- Choi RS, Lai WYX, Lee LTC, et al. Current and future molecular diagnostics of gastric cancer. Expert Rev Mol Diagn. 2019;19(10):863–874.
- Tam C, Wong JH, Tsui SKW, et al. LncRNAs with miRNAs in regulation of gastric, liver, and colorectal cancers: updates in recent years. Appl Microbiol Biotechnol. 2019;103(12):4649–4677.
- Li D, Wang J, Zhang M, et al. LncRNA MAGI2-AS3, regulated by BRD4, is an independent prognostic marker and promotes gastric cancer progression via maintaining ZEB1 overexpression. Mol Ther Nucleic Acids. 2019;19:109–123.
- Li D, She J, Hu X, et al. The ELF3-regulated lncRNA UBE2CP3 is over-stabilized by RNA–RNA interactions and drives gastric cancer metastasis via miR-138-5p/ITGA2 axis. Oncogene. 2021;40(35):1–13.
- Li D, Xu M, Wang Z, et al. The EMT-induced lncRNA NR2F1-AS1 positively modulates NR2F1 expression and drives gastric cancer via miR-29a-3p/VAMP7 axis. Cell Death Dis. 2022;13(1): 022–04540.
- Li G, Su Q, Liu H, et al. Frizzled7 promotes epithelial-to-mesenchymal transition and stemness via activating canonical Wnt/beta-catenin pathway in gastric cancer. Int J Biol Sci. 2018;14(3):280–293.
- Xu G, Meng L, Yuan D, et al. MEG3/miR21 axis affects cell mobility by suppressing epithelial mesenchymal transition in gastric cancer. Oncol Rep. 2018;40(1):39–48.
- Fioroni I, Dell’Aquila E, Pantano F, et al. Role of c-mesenchymal-epithelial transition pathway in gastric cancer. Expert Opin Pharmacother. 2015;16(8):1195–1207.
- Hu L, Zang MD, Wang HX, et al. Biglycan stimulates VEGF expression in endothelial cells by activating the TLR signaling pathway. Mol Oncol. 2016;10(9):1473–1484.
- Busuttil RA, George J, House CM, et al. SFRP4 drives invasion in gastric cancer and is an early predictor of recurrence. Gastric Cancer. 2020;24(3):589–601.