ABSTRACT
Brown seaweeds are a promising source of bioactive substances, particularly oligosaccharides. This group has recently gained considerable attention due to its diverse cell wall composition, structure, and wide-spectrum bioactivities. This review article provides a comprehensive update on advances in oligosaccharides (OSs) production from brown seaweeds and their potential health applications. It focuses on advances in feedstock pretreatment, extraction, characterization, and purification prior to OS use for potential health applications. Brown seaweed oligosaccharides (BSOSs) are extracted using various methods. Among these, enzymatic hydrolysis is the most preferred, with high specificity, mild reaction conditions, and low energy consumption. However, the enzyme selection and hydrolysis conditions need to be optimized for desirable yield and oligosaccharides composition. Characterization of oligosaccharides is essential to determine their structure and properties related to bioactivities and to predict their most suitable application. This is well covered in this review. Analytical techniques such as high-performance liquid chromatography (HPLC), gas chromatography (GC), and nuclear magnetic resonance (NMR) spectroscopy are commonly applied to analyze oligosaccharides. BSOSs exhibit a range of biological properties, mainly antimicrobial, anti-inflammatory, and prebiotic properties among others. Importantly, BSOSs have been linked to possible health advantages, including metabolic syndrome management. Metabolic syndrome is a cluster of conditions, such as obesity, hypertension, and dyslipidemia, which increase the risk of cardiovascular disease and type 2 diabetes. Furthermore, oligosaccharides have potential applications in the food and pharmaceutical industries. Future research should focus on improving industrial-scale oligosaccharide extraction and purification, as well as researching their potential utility in the treatment of various health disorders.
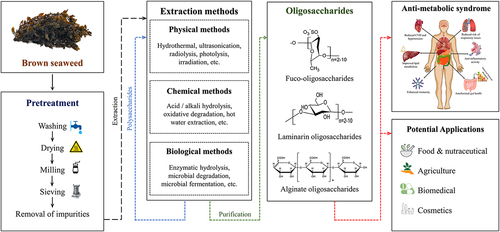
Highlights
Brown algae exhibit a great diversity to offer a wide range of OSs bioactivities.
Non-enzymatic extraction yields 30-58% OSs more than enzymatic routes.
Enzymatically derived OSs exhibit better bioactivity than non-enzymatic OSs.
Nanofiltration/activated carbon can solve bottlenecks in large-scale purification.
OSs health applications are proven at in vitro stage, clinical studies yet to be done.
1. Introduction
The marine ecosystem thrives with a plethora of different plants and animals; among these species, seaweed is one of the exceptionally important components supporting marine animals and humans as potential renewable resources. Seaweeds are a potential source of structurally diverse bioactive compounds showing great potential in food, nutraceutical, pharmacy, and other industries [Citation1]. Brown seaweeds are the second largest group, belonging to the class Phaeophyceae. This class comprises 14 orders, 265 genera, and around 2000 species. Brown seaweeds are rich in bioactive compounds such as polyphenols, peptides, polysaccharides, and carotenoids. Compared to red and green seaweeds, brown algae crude polysaccharides exhibit higher antioxidant properties with a high quantity of polyphenols and sulfates [Citation2,Citation3]. In the past two decades, the seaweed farming and production industry has grown consistently. As per Food and Agriculture Organization (FAO) data, global seaweed production has tripled from 118,000 to 358,200 tons. China holds the first position in the market, accounting for 58% of world aquaculture production [Citation4].
Brown algae are rich in sulfated polysaccharides fucoidan and non-sulfated polysaccharides alginate and laminarin, which are extracted mostly from Sargassum, Laminaria, Turbinaria, Saccharina, and Ecklonia species through various physiochemical (hydrothermal, ultrasonication, irradiation, hydrolysis with acid/alkaline and microwave), and biological methods (enzyme hydrolysis, microbial fermentation, etc.) [Citation1,Citation5,Citation6]. Oligosaccharides are potential carbohydrates that consist of 2–10 single chained or branched monosaccharide units bound together by α- and/or β-glycosidic bonding. Recent studies have explored the structure and biological activities of brown algal oligosaccharides (BSOSs). This is due to their low molecular weight, high adsorption capacity, and high water solubility. The physio-chemical characteristics indicate promising applications in food, agriculture, fishery industries, etc., including anti-diabetic, anti-inflammatory, anti-obesity, and other health-promoting properties [Citation1,Citation7,Citation8]. The methodology employed for extracting oligosaccharides shows a significant effect on yield, structure, molecular weight, viscosity, and biological activity [Citation9–12].
Metabolic syndrome is a collective condition and disorder consisting of type 2 diabetes, obesity, cardiovascular diseases, hypertension, and altered sugar levels. The increased ROS production in the body affects regular metabolism by damaging DNA, proteins, cell walls, etc. Changes in lifestyle, higher consumption of hypercaloric foods, less physical activity, etc., are the main causes of metabolic syndrome, which alters lipid metabolism and induces obesity and related risks such as diabetes, fatty liver, and hypertension [Citation13,Citation14]. According to WHO reports, CVD is becoming a threat, by 2030 the CVD-related deaths will reach 22.2 million, and 17.5 million deaths have already been recorded since 2012. In Western countries, 50% of CVD-related deaths were caused by a high-calorie diet, and it was estimated that as of 2016, 1.9 billion adults were overweight and 650 million were obese [Citation15]. Recent studies have confirmed that obesity and CVD are influenced by intestinal and gut health. On the other hand, seaweed oligosaccharides are reported to have a positive influence on gut microflora by enhancing the growth of beneficial microbes hence it has gained high attention in recent times, due to their substantial scientific and economic values and role in prebiotics [Citation16–18]. Apart from human health applications, oligosaccharides possess potential applications and commercial values in food and nutraceuticals, agriculture, forestry, animal husbandry, fishery, bio-medical, and cosmetic industries [Citation1,Citation19,Citation20].
Despite more advantages and potential applications, only a few pieces of the literature have been reported on oligosaccharides derived from brown macroalgae. Hence, this review introduces the emerging potential of brown seaweeds for bioactive oligosaccharide production. It focuses on various biosynthesis methods employed for oligosaccharides extraction, subsequent purification and physio-chemical characterization, structural variations, anti-metabolic activities, and potential commercial applications.
2. Extraction and purification of algal oligosaccharides
2.1. Source and chemical composition
In recent years, due to the possession of rich bioactive compounds and the feasibility of the process, seaweeds have gained great attention in drug and dietary research [Citation21,Citation22]. Among them, brown seaweeds, the second largest group after green seaweeds are of great importance due to their unique structural composition and range of bioactive components [Citation23]. Brown seaweeds are widely spread worldwide, and they are rich in polyphenols, proteins, pigments, and polysaccharides. Among them, the polysaccharide is a major fraction found more diverse in brown seaweeds such as fucoidan, alginate, and laminarin [Citation1,Citation2].
Oligosaccharide production from marine sources is gaining significant attention due to its high bioactivity. These bioactivities are reported to be more prevalent in oligosaccharides derived from sulfated polysaccharides [Citation22]. Therefore, polysaccharides from brown seaweeds were the major focus of oligosaccharide research. Fucoidan is a structural sulfated polysaccharide with a rich fucose content that can only be found in brown seaweeds. After depolymerization, it forms fucooligosaccharides. Fucoidan is mainly composed of sulfate esters groups and L-fucose monosaccharides, linked by α-(1→3) or alternating α-(1→3) and (1→4) glycosidic bonds, whereas the backbone of fucoidan oligosaccharides composed of α-(1→2) linked mannose and β-(1→4) linked glucuronic acid [Citation1,Citation2,Citation24]. Laminarin is used for laminarin oligosaccharide biosynthesis, the food storage polysaccharides, composed of β-(1→3) linked β-D-glucose monomers. It has a small portion of C-6 branched singular β-D-glucose [Citation2,Citation25,Citation26]. The source of alginate oligosaccharides is alginate (sodium alginate), which is composed of β-(1→4) linked mannuronic acid and α-(1→4)-guluronic acid [Citation1,Citation27,Citation28]. The seaweed polysaccharides have widespread applications in industries, yet their potential is limited due to their high molecular weight. Hence, it was aimed to be reduced in length or at lower molecular weight to increase its dissolution in water and other solvents. This was done while retaining their bioactivity. It is more likely that when solubility increases, the bioactivity is also deciphered more strongly. Therefore, the oligosaccharides synthesis has been proposed to have a better commercial and health prospect. The oligosaccharides are mainly synthesized directly from algal biomass or algal polysaccharides by adopting various pretreatment and treatment routes [Citation1,Citation20]. However, the most prevalent methods for the degradation of polysaccharides into oligosaccharides are physio-chemical and biological methods.
2.2. Pretreatment of biomass
Oligosaccharides cannot be naturally obtained from biological sources economically. Therefore, it is synthesized in vitro from biomass and polysaccharides by employing costly chemicals and enzymes. Certain ratios of chemicals and enzymes are added to the biomass. So, it is important to remove unwanted fractions and make the catalysts or biocatalysts more accessible to biomass or polysaccharides prior to the synthesis of oligosaccharide, and its pretreatment is essential. The seaweed samples were adequately washed with water and dried before milling and sieving which were further washed with alcohol and other organic solvents to remove other fractions and impurities such as pigments, lipids, and proteins [Citation1,Citation29–31]. summarizes recent works describing the different methods employed for oligosaccharide pretreatment and extraction from polysaccharides.
Table 1. Advances in enzymatic and non-enzymatic extractions of brown algal oligosaccharides.
2.3. Chemical methods
Seaweed biomass and polysaccharides are degraded into oligosaccharides using chemical treatment methods such as hydrolysis (acid and alkali), oxidative degradation, and the synthesis of organic compounds. Due to easy operation, high yield, reproducibility, and low cost, hydrolysis is considered a steadfast method [Citation1,Citation27]. During hydrolysis, the polysaccharide’s glycosidic bonds were separated in an acidic environment with H+ catalysts and produced relatively low-molecular-weight oligosaccharides. Since this method is random and has no specificity, it produces low organic acid inhibitors along with oligosaccharides [Citation27]. However, this method has some drawbacks such as high time consumption (4–12 h), post neutralization, by-product formation, and eco-hazards [Citation27,Citation28].
In one study, fucoidan was extracted from Kjellmaniella crassifolia by hot-water extraction and ethanol precipitation [Citation49] with a yield of 4.2% (w/w), Fucoidan was subsequently purified by DEAE-Cellulose column, then hydrolyzed by 0.1 M HCl (at 80°C for 30 min), and neutralized by 0.2 M NaOH solution. After the digestate was mixed with ethanol and centrifuged, the supernatant containing significant fuco-oligosaccharides was recovered after rotary evaporation and lyophilization [Citation24]. Similarly, another study has extracted crude fucoidan polysaccharides from Saccharina japonica by hot-water extraction and ethanol precipitation, followed by this oligosaccharide were synthesized by acid hydrolysis at relatively high concentration of 0.6 M HCl at 80°C for 30 min and the oligosaccharides were recovered by ethanol precipitation and centrifugation [Citation8]. The sun-dried brown seaweed Ecklonia cava subsp. stolonifera (formerly Ecklonia stolonifera) was also used for the synthesis of β-gluco oligosaccharides by treating it with mild 0.1 M HCl for 20 min at room temperature and neutralized by Na2CO3 afterward the oligosaccharides were recovered from the supernatant using Bio gel P-4 chromatography with a yield of 9.71 nmol/mL concentrate [Citation50]. At higher concentrations and longer duration, polysaccharides were completely or partially denatured. This can be overcome by maintaining an optimum temperature and a mildly acidic environment [Citation27,Citation51].
The synthesis of oligosaccharides using the alkali hydrolysis method was less reported in the literature and mostly employed along with other methods to produce oligosaccharides [Citation1]. This method is widely employed to produce oligosaccharides from plants and spent biomass [Citation52–54]. Alginic acid was treated with alkali to breakdown internal glycosidic links [Citation27]. A strong alkaline environment resulted in various products such as glucuronide, anhydrous isogluconic acid, and 2-deoxy-3-C-methyltetrahydro acid, whereas low alkaline concentration produces majorly 2,3-dideoxypentaric acid. Likewise, low-molecular-weight alginates were prepared by a series of alkaline treatments, which showed better antioxidant activities; moreover, the optimized conditions such as time, temperature, and pH resulted in a higher yield of alginate [Citation37].
Oxidative degradation is a clean, eco-friendly, and lucrative method to synthesize oligosaccharides from polysaccharides. Various chemicals are used in oxidative degradation such as H2O2, NaClO, KmNO4, and NaNO2. Among them, H2O2 is extensively used to synthesize oligosaccharides. When temperature and pressure are controlled, water is produced. Hence it is called the green process. During the degradation process, H2O2 forms hydroxyl, superoxide, and hydroperoxyl radicals, which cleave glycosidic bonds and produce oligosaccharides [Citation1,Citation27]. Unlike alkaline hydrolysis, only a few works have been reported using this technique for oligosaccharide synthesis. Zúñiga et al. [Citation32] synthesized low-molecular-weight fractions from sulfated galactan polysaccharide extracted from Schizmenia binderi (Rhodophyta) using H2O2 in the presence of Copper (II) acetate monohydrate. In brief, the polysaccharide (250 mg) was extracted with 40 mg Cu (II) acetate monohydrate in water. Subsequently, 16 ml of 9% H2O2 solution was added (at 4 mL/h) in a reaction at 60°C and pH 7.5 for 4 h. During the process, every 30 min. the contents were treated with Chelex 100 resin to remove Cu+ ions. Afterward, the fraction was freeze-dried and screened by gel chromatography.
Huang et al. [Citation25] discussed various bio- and organic-synthesis techniques to produce laminarin oligosaccharides from laminarin polysaccharides extracted from various seaweeds. Where they used 4,6-O-benzylidenated acceptors to cleave β-(1→3) bonds into homogenous oligosaccharides with α-(1→3) and α- (1→6) bonds, with a yield of up to 43%.
Chemical degradation of polysaccharides or other substances into oligosaccharides is one of the easiest and most viable methods in terms of cost, operation, and yield. Yet these conditions apply to certain samples, and the application of this method is limited due to abrasive reaction conditions such as high temperature and pressure, involvement of repeated protection and deprotection steps, the release of hazardous chemicals, and unwanted by-product formation [Citation1,Citation25].
2.4. Physical methods
Various physical methods have been widely employed to depolymerize the algal polysaccharides into oligosaccharides. Hydrothermal degradation and irradiation methods such as microwave-assisted degradation, ultraviolet radiation, and gamma irradiation were mainly applied. Moreover, photolysis and ultrasonication were also used for oligosaccharide production. There are studies that suggest it is challenging to synthesize oligosaccharides using one physical method, since the resulting fraction can only reach 50 kDa; therefore, more than one method must be used to obtain desirable sizes of fragments [Citation27].
The algal samples are treated at high temperatures, either directly or hydrothermally, to produce oligosaccharides by disrupting glycosidic bonds [Citation27]. In the advanced hydrothermal methods, supercritical liquids disrupt glycosidic bonds in polysaccharides to produce oligosaccharides [Citation1]. Alginate oligosaccharides are produced along with disaccharides, monosaccharides, lactic acid, and glycolic acid during hydrothermal treatment. It cleaves glycosidic bonds in M-M, M-G, and G-G forms [Citation55]. The fucoidan molecular weight was reduced with the increasing hydrothermal treatment pressure, whereas the total sugar content remained the same. This is due to the split-up of polymer aggregates, not covalent bond denaturation in the polymer chain [Citation56]. Similarly, Shi et al. [Citation57] synthesized low-molecular-weight fuco-oligosaccharides without desulfation from Holothuria floridana by varying the operating time, temperature (100–121°C) and pH at 5–6. Using the surface resonance method, hydrothermal operation conditions were optimized for fucoidan degradation. It was stated that at 214°C, 40 bar pressure, 60 mL/g water-to-substrate ratio, 223 rpm speed, and 5 min duration, a better and more efficient yield was obtained [Citation58].
The radiolysis method is one of the most reliable physical methods for synthesizing oligosaccharides from polysaccharides [Citation25]. Moreover, various bioactive chemicals and biomedical compounds were also produced [Citation1]. Where gamma radiation and ultraviolet light radiation were applied for the degradation of polysaccharides [Citation1,Citation25,Citation59,Citation60], among these, gamma radiation is efficient and effective than UV radiation due to its higher energy content [Citation1,Citation61] The oligomers produced by the radiation method have been widely used in agriculture as plant growth-promoting agents [Citation28]. Gamma irradiation can be applied to synthesize laminarin oligosaccharides from laminarin, which show higher antioxidant activities (Fe2+ reduction and DPPH) than laminarin, due to proper reduction of polymerization degree [Citation25]. Likewise, gamma radiation can produce alginate oligosaccharides without a special prerequisite to control additives, environment, and temperature for denaturation. The low-molecular-weight alginate (1.3–5.6×101 average molecular weight) was obtained at 20–100 kGy 60Co gamma irradiation [Citation61]. As a result of gamma radiation, fucoidan obtained from Fucus vesiculosus, does not affect the sulfated groups, but reactive carboxylic group forms, which enhanced the bioactivity of fucoidan derivatives [Citation59]. Compared to gamma radiation, UV radiation requires a shorter denaturation time because seaweed-derived polysaccharides more effectively absorb UV radiation than gamma radiation [Citation1].
Microwave-assisted extraction has significant efficiency and is suitable for extracting temperature-sensitive and active substances [Citation25]. Microwave-assisted irradiation method reduces the denaturation time by activating a dipole reaction during operation. It has also been reported that this method can be employed individually and in combination with other methods for an efficient process [Citation1]. Hair growth-promoting fuco-oligosaccharides (0.5–1 kDa) were derived from Sargassum glaucescens using microwave-assisted high-pressure water extraction. After repeated test cycles, the optimal conditions were 5 bar pressure, 130°C temperature, and 25 min. The fragments above 1 kDa induced the higher hair growth in subjected rat models, which clearly indicates the application of low-molecular-weight [Citation5]. Low-molecular-weight (5–30 kDa) fucoidans were extracted from CO2-deoiled Undaria pinnatifida by microwave-assisted extraction at 600W, 140°C, and 15–60 min duration, experiments suggest that continuous irradiation for 1 min at constant power results in better yields [Citation62].
Ultrasonic-assisted denaturation of seaweed polysaccharides is another promising physical method to synthesize oligosaccharides by applying a wide range of frequencies (16–100 kHz) and power (80–200 W). It has the potential for large-scale applications. Also, the ultrasonic-assisted technique extracts phycoerythrin, lipids, and amino acids from various algae sources [Citation1,Citation25]. One study extracted low molecular weight (<10 kDa) laminarin from Ascophyllum nodosum and Laminaria hyperborea using 60% ultrasonic power amplitude and 0.1 M HCl at 70°C for 15 min, and the obtained fragments showed efficient antioxidant and antibacterial activity [Citation63]. The sulfated polysaccharide was derived from Saccharina japonica (formerly Laminaria japonica) through a hot-water extraction and further reduced by H2O2-ultrasound induced extraction at 800W for 71 min. During denaturation, the molecular weight of laminarin was reduced from 2000 to 18.576 kDa [Citation64].
In addition to these techniques, plasma can also be used to degrade the polysaccharides into oligosaccharides. It has been mentioned that an electric discharge will be applied to the reactor containing alginate polysaccharides to produce reactive O2 species by disrupting the polymer chain bonding [Citation25]. Physical methods are employed to degrade polysaccharides into valuable oligosaccharides and other products, and this is widely accepted as green, simple, cost-effective, nontoxic, and efficient methods. Despite these advantages, this method has disadvantages, such as higher power consumption, non-control over polymerization degree, and optimization. For example, prolonged exposure or irradiation can denature the entire polysaccharide and affects its bioactivity [Citation1,Citation25,Citation61]. These disadvantages can be resolved by combining them with other green methods in series [Citation28].
2.5. Biological methods
Biological techniques have been used to produce oligosaccharides from diverse sources. Among them, enzymatic hydrolysis has gained more attention due to its potential advantages. These include specificity and the production of target oligosaccharides in large quantities by site-specific cleavage. Unlike the chemical methods, it does not produce harmful substances, is environmentally friendly, offers an efficient reaction rate, and produces fewer amount of monosaccharides [Citation20,Citation25,Citation31]. Moreover, enzyme hydrolysis improves bioactive compounds extraction yield, food applications safety, and sustainability [Citation9]. The enzymes involved in the polysaccharides degradation are obtained from various sources such as bacteria, fungi, and algae. The production of laminarin oligosaccharides can be achieved using laminarase or direct sources of laminarase such as bacteria (B. circulans, Flavobacterium johnsoniae, Paenibacillus sp., Rhodothermus marinus, and Thermotoga maritima), fungi (Candida albicans, Saccharomyces cerevisiae, and Yarrowia lipolytica), and algae (L. digitata) which were used in past studies [Citation25]. Like chemical synthesis, biosynthesis is also applied to produce oligosaccharides. It differs from chemical and physical synthesis because it employs a targeted degree of polymerization without the use of protection and deprotection steps. Generally, biosynthesis involves a variety of enzymes working together to produce desired oligosaccharides [Citation1].
Production of crude oligosaccharides obtained from Sargassum confusum using a mixture of enzymes is an example of a biosynthesis method. In one study, the polysaccharides were extracted by hot-water extraction. The obtained products were hydrolyzed using amylase, cellulose, pectinase, and xylanase in equal ratios (1:1:1:1 w/w/w/w) for 3.9 h at pH 6.2 and 51°C. The synthesized oligosaccharides (<3000 Da) were dialyzed with a molecular-weight cutoff membrane, and derivatives were lyophilized [Citation65]. Alginate oligosaccharides (1–5 kDa) were prepared by mixing 2% alginate with alginate lyase (28 U/mL) at 37°C for 6 h, and the hydrolyzates were recovered by ethanol precipitation. Similarly, agar and carrageenan oligosaccharides (0.4–1.4 and 1–7 kDa) were derived by enzymatic hydrolysis [Citation17]. Tran et al. [Citation66] cloned endo-α(1,3)-fucoidanase encoding gene Mef2 using E. coli produced Mef2 fucoidanase. It is further used to synthesize fuco-oligosacchrides from Fucus distichus subsp. evanescens (formerly Fucus evanescens) and Saccharina latissimi. The enzyme showed the maximal activity at pH 8 and 35°C, and results confirmed the enzyme could withstand up to 50°C. The obtained oligosaccharides consisted of aunique backbone of α(1,3)-linked fucosyl residues and a novel α(1,4)-linked fucosyl branches. Similarly, synthetic laminarinase-encoding genes (Relam1, Tvlam 1, and Trlam1) were cloned and native genes were obtained from S. cerevisiae strain Y294 [Citation67]. A generation of engineered S. cerevisiae Y294 was used to hydrolyze Ecklonia maxima laminarin in small (120 mL) and large (2 L) fermenters at 30°C for 7 days, where laminarin was degraded into small fragments and produced ethanol with a yield of 43%. Wang et al. [Citation68] reported that the unsaturated alginate oligosaccharide produced by alginate lyases has novel bioactivities such as antitumor, antioxidant, neuroprotective, and immune stimulation. Also, it showed better anti-obesity properties than saturated alginate oligosaccharides through activating AMPK signaling pathway in high-fat diet-fed mice. In particular, endolytic alginate lyase cleaves alginate and produces unsaturated alginate oligosaccharides (as di, tri, & tetra-saccharides), whereas the exolytic enzymes further degrade oligosaccharides into monosaccharides. Hence, endolytic enzymes are preferred for alginate oligosaccharide synthesis [Citation69]. Compared to other nonspecific methods (acid hydrolysis, alkaline hydrolysis, etc.), the enzymatic method is more precise in producing oligosaccharides and does not break their derivatives. Yet this method has drawbacks, such as credible loss of sulfate groups, difficulties in reusing enzymes, and recovery of enzymes after the reaction [Citation1,Citation28].
In order to overcome the cost of enzyme purification and product recovery after enzymatic hydrolysis, microbes capable of producing desired enzymes have been used directly to produce oligosaccharides via the fermentation [Citation28]. Different fungal species were used to produce fucose and alginate oligosaccharides from Sirophysalis trinodis (formerly Cystoseira trinodis [Citation70]). The fermentation was carried out in 40 mL bottles, using seawater as medium (10 mL medium +0.5 g biomass +0.1 mL fungal culture) under aseptic conditions for 3 days at 28°C and 120 rpm. Later, the alginate and fucoidan oligosaccharides were recovered from fermentation medium with CaCl2 and ethanol treatments, respectively. Depending on the fungal species, the MW of fucoidan was reduced from 41 to 81.5% and alginate was reduced from 28 to 75%. Alginate oligosaccharides were produced by engineered Yarrowia lipolytica while fermenting cellulase-hydrolyzed Saccharina japonica biomass for 72 h. It produced 29.9 g/L of alginate oligosaccharides with a polymerization degree of 2–3 [Citation71]. Even though biological methods cost more, due to high degree of polymerization, site-specific activity, purity, and eco-friendly attributes, biological methods are widely preferred to produce oligosaccharides under controlled reaction conditions. Extensive research has been carried out to improve biological methods for large-scale OSs production and application.
3. Purification of oligosaccharides
Oligosaccharides produced by physicochemical and biological methods may contain impurities such as protein, monosaccharides, acids, and furfurals. They must be removed since they’re used for nutraceutical and pharmaceutical purposes. In certain instances, oligosaccharides synthesized by combining different methods will have different molecular weights, G/M ratios and sequences. It is, therefore, necessary to purify them for homogenous formulation [Citation29,Citation41]. Various methods have been employed to purify the oligosaccharides, such as chromatographic techniques, electrophoresis, precipitation, and activated carbon adsorption. Still many pieces of literature have reported that chromatographic techniques are the simplest and most efficient way for the oligosaccharide’s purification [Citation28,Citation29,Citation41].
A recent study used molecularly imprinted polymers modified SPE with deep eutectic and ionic solvents to purify fucoidan and laminarin. This showed a higher efficiency of 95.5 and 87.6%, respectively [Citation72]. On the other hand, isoelectric point precipitation, trichloroacetic acid denaturation, and CaCl2 precipitation methods were applied to remove proteins and uronic acids from low-molecular-weight fucoidan. It was further purified by 14 kDa membrane separation to separate samples based on molecular weight [Citation41]. Thin-layer chromatography (TLC) helps to determine a sample’s polymerization degree. The solvent system used to detect alginate oligosaccharides was n-butanol: formic acid: water (4:6:1) followed by cerium sulfate solution for stains. This method is challenging for the separation of alginate oligosaccharides since they’re anionic which can react with stationary phase solvent and it is difficult if the sample contains more than six residues [Citation28].
A three-stage purification process purified laminarin oligosaccharides. The proteins, polyphenols, and alginic acid fractions were removed stepwise from the sample via ethanol and CaCl2 precipitation [Citation73]. The purified sample was analyzed using HPLC-RI, which confirmed t laminarin purity (1.5-fold higher than crude extract). Later, the obtained laminarin was further purified by filtration using 10 kDa molecular weight cutoff membrane. The purity of laminarin was enhanced up to 1.7 and 2.5 folds higher than stage 1 and crude extract. Finally, the fraction obtained from stage 2 was mixed with 0.1 M HCl and passed through a SPE cartridge. This further increased laminarin purity up to 1.6 and 2.3 folds, higher than stage 2 and crude extract, respectively. The method of size exclusion chromatography (SEC) is widely used to separate crude polysaccharides based on their molecular weight. This is also used to determine the molecular weight. However, for samples extracted from different species, separation is achieved by relying on size and diffusion characteristics and not only based on molecular weights [Citation25,Citation74].
Size exclusion chromatography and affinity chromatography are powerful and convenient methods for laminarin purification [Citation25]. Affinity chromatography can be used separately or combined with other purification techniques to separate the active compounds such as enzymes, proteins, saccharides, and antibodies. Low-molecular-weight alginates obtained from Bifurcaria bifurcata were characterized by high-performance-size exclusion chromatography (HPLC-SEC) and high-performance anion chromatography (HPAEC) to analyze the molecular weight and polymerization degree (DP), respectively. The resolution of oligosaccharides separated by SEC is low; thus, it needs repeated chromatograms, by using HPLC-SEC such problems can be rectified. HPAEC separates the samples based on molecular weight, saccharide composition, and glycosidic bonds. Hence, it is widely used to study the DP and to distinguish between saturated and unsaturated samples. For example, the retention time of saturated alginate oligosaccharides is shorter than unsaturated alginic oligosaccharides with the same molecular size [Citation7,Citation28]. Moreover, HPAEC-pulsed amperometric detection was used to overcome the lag caused by UV/RI detectors in the sensitive oligomers detection. Similar to SEC, ultra-high performance liquid chromatography (UHPLC) coupled with an ethylene bridge hybrid (BEH) amide column was also used to differentiate saturated and unsaturated oligosaccharides, where it distinguishes the samples based on the hydrophilic interaction chromatography principle [Citation28]. A previous study used activated carbon adsorption and gel chromatography to purify the neoagaro-oligosaccharides. Subsequently, the activated carbon adsorption effectively removed salt impurities, while gel chromatography with Sephadex columns separated oligosaccharides based on their molecular weights [Citation75].
Despite the advantages, the application of these purification methods at the industrial scale was limited due to cost, low efficiency, low product yield, etc. Some techniques are reported to be being used in industrial scale, such as activated carbon adsorption, membrane filtration, moving bed chromatography, activated fixed bed columns, etc [Citation76–78]. Nanofiltration is a reliable and feasible purification method for industrial-scale applications as compared to other listed methods [Citation79,Citation80]. However, the membranes are rapidly fouling with higher pressures, hence by optimizing the operating conditions the membrane life and product quality can be improved [Citation76,Citation81].
4. Structural characteristics of oligosaccharides
The synthesized oligosaccharides were composed of various functional groups and chemical structures. It is necessary to characterize both the structure and chemical composition since they also play a major role in bioactivity and various applications. The oligosaccharides can be characterized by various techniques such as mass spectrometry, electrospray ionization (ESI), matrix-assisted laser desorption/ionization (MALDI), nuclear magnetic resonance (NMR), and Fourier infrared spectroscopy (FTIR).
Mass spectrometry is a susceptible method to analyze oligosaccharides, which provides detailed information on molecular weight, chain length distribution, fragments, monosaccharide composition, degree of polymerization, links, and modification sites, especially ESI and MALDI are advanced tools used to examine the low and middle molecular weight of oligosaccharides [Citation29]. Zhang et al. [Citation12] and Kailemia et al. [Citation82] summarized various mass spectrum methods used for the oligosaccharides characterization, such as ESI-MS, MALDI-MS, time-of-flight coupled MALDI (TOF-MALDI-MS), infrared multiphoton dissociation mass spectroscopy (IRMPD-MS), collision-induced dissociation mass spectroscopy (CID-MS), and fast atom bombardment mass spectroscopy (FAB-MS). These spectroscopic methods can be differentiated based on time-of-flight detection and triple quadrupole. Holtan et al. [Citation83] characterized mannuronic and guluronic oligosaccharides obtained from alginic acid using ESI-MS and the results helped them to determine the uniform chain lengths of oligomers. Wu et al. [Citation24] used ESI-CID-MS to characterize the sulfated fuco-oligosaccharides. The results confirmed various ionic residues of oligo-fucose and suggest characterizing highly branched and sulfated polysaccharides. Compared to ESI-MS, MALDI-MS forms mostly single charged ions during oligosaccharide analysis; hence, the spectral complexity is lesser than ESI-MS, which results in fast and accurate results [Citation29].
Nuclear Magnetic Resonance (NMR) is another sensitive method for the structural characterization of polysaccharides and oligosaccharides. NMR consists of proton magnetic resonance (1H-NMR), carbon 13 NMR (13C-NMR), and 2-dimensional NMR (2D-NMR). Like MS, NMR provides information on monosaccharide orientation, oligosaccharide composition, presence of α and β carbohydrates, and bonds [Citation12,Citation29,Citation39]. Mrudulakumari Vasudevan et al. [Citation28] summarized NMR usage, pros, and cons. The authors mentioned that 13C-NMR has the potential to characterize saturated alginic oligosaccharides with high polymerization degrees as well as alginate lyases based on their specificity. Although this method is rapid and accurate, it is difficult to use only 1H-NMR for the characterization of larger unsaturated polysaccharides (>3 DP) since the analyte requirement is high. Unlike mass spectroscopy, NMR spectroscopy is direct and has a quantitative relationship with the concentration (molar) and intensity of NMR [Citation84]. Laminarin oligosaccharides obtained from Laminaria digitata have been characterized by 1H-NMR and 13C-NMR spectroscopy. This revealed that the repeating units of oligo-laminarin are 1→3 linked β-D-Glcp with non-reducing terminal β-D-Glcp residues at C3 [Citation83]. Thanh et al. [Citation85] studied the structural characteristics of various alginate blocks obtained from Turbinaria oranta using 1H-NMR and 13C-NMR spectroscopy. The results revealed the M/G ratio of alginate as 1.25 and gave detailed information about all the alginate blocks (MG-block, M-block, and G-block).
FTIR is a fundamental and sensitive analysis used to study the structure and functional groups by measuring the bonds in the mid-infrared frequency range [Citation86]. Various bioactive compounds such as polysaccharides, oligosaccharides, proteins, etc., are primarily characterized using FTIR. The FTIR can be used to distinguish the M and G blocks (absorption bands of M are 893 and 892 cm−1 where G are 947, 903, 813, and 780 cm−1) in alginate [Citation12]. The FTIR can be used to separate alginate oligosaccharides based on structural variation. It can produce rapid results without complicated pretreatments. Tran et al. [Citation86] determined the enzymatic activity of two structurally different fucoidans obtained from Fucus distichus subsp. evanescens and Saccharina latissima. Sellimi et al. [Citation23] characterized the fucans obtained from Gongolaria barbata (formerly Cystoseria barbata) using ATR-FTIR. It primarily confirmed the presence of sulfate group in fucans and later using NMR and mass spectroscopic methods. Likewise, Yudiati et al. [Citation37] used FTIR to characterize various functional groups including β-mannuronic acid and α-guluronic acid present in alginate oligosaccharides extracted from Sargassum latifolium.
Brown seaweeds are mainly composed of three polysaccharides such as fucoidan, alginate, and laminarin. Among them, fucoidan is the only sulfated polysaccharide [Citation5]. Each polysaccharide is chemically and structurally different from other polysaccharides and from their own derivatives. The structure of oligosaccharides derived from polysaccharides through various methods will be different from each other. This is because each extraction method has different digestion/denaturation patterns. For example, alginate oligosaccharides are extracted from alginate polysaccharides through various physio-chemical (hydrothermal, irradiation, microwave and ultrasonic-assisted extraction, oxidative degradation, acid, and alkaline hydrolysis) and biological methods (microbial fermentation, enzymatic hydrolysis, etc.). Yet acid hydrolysis, oxidative degradation, and enzymatic hydrolysis are the most preferred methods to produce alginate oligosaccharides [Citation12,Citation27,Citation28]. Based on the structure, alginate is an unbranched co-polymer of hexuronic acid epimers α-L-guluronic acid (G) and β-D-mannuronic acid (M), linked by 1→4 glycosidic bonds [Citation28,Citation31]. The chemical structure and monomer composition vary with the species or type of algal tissue [Citation20]. As shown in , structurally diverse alginate oligosaccharides were obtained with different extraction methods. When alginate oligosaccharides are synthesized by acid hydrolysis, they contain unmodified hexuronic acid. It is similar to the parent compound; however, their degradation process is random [Citation12,Citation31], whereas, in H2O2-induced oxidative degeneration, the structure is modified by a carboxyl group oxidized from the aldehyde group at the reducing end. This structural modification was induced due to the abstraction of C1 protons from M or G epimers and glycosidic bond degradation [Citation12]. In enzymatic hydrolysis, the bonds between G and M were cleaved via the β-elimination mechanism. It forms an unsaturated non-reducing end moiety due to the action of lyase double bond. The alginate oligosaccharide is formed at the end of this reducing end between C4 and C5, which is called 4-deoxy-L-erythro-hex-4 enopyranosyluronic acid [Citation12,Citation28].
Figure 1. Different structures of alginate oligosaccharide produced by acid hydrolysis, oxidative degradation, and enzymatic hydrolysis [Citation31].
![Figure 1. Different structures of alginate oligosaccharide produced by acid hydrolysis, oxidative degradation, and enzymatic hydrolysis [Citation31].](/cms/asset/d9cc4fe7-032d-4364-b15d-0800eb13686e/kbie_a_2252659_f0001_oc.jpg)
Unlike alginate, only very few literatures were reported for fucoidan and laminarin structural study. Fucoidan is a sulfated polysaccharide found predominantly in brown seaweeds, which is reduced to oligosaccharides either by direct or indirect methods. Fucoidan contains a backbone of (1→4) linked α-L-fucopyranose residues or varying (1→3) and (1→4) linked α-L-fucopyranose residues may be substituted with sulfate or acetate and/or have side-branched fucopyranose or other glycosyl units [Citation51,Citation87]. Fucoidan has a more complex structure than laminarin and alginate. It mainly composes fucose sugar and other monosaccharides such as galactose, mannose, xylose, glucose, and glucuronic acid based on seaweed species [Citation9]. During acid hydrolysis, the fucoidan composition varies with respect to time, temperatures, and concentrations. It is because, at higher concentration and temperature, the fucose in fucoidan is almost denatured, hence low/mild acid hydrolysis is preferred [Citation51]. Conventional methods such as acid hydrolysis and hot-water extraction can partially cleave esters which affect bioactivity. Hence, these methods are not preferred [Citation5]. Tran et al. [Citation66] synthesized fuco-oligosaccharide using endo-α-(1,3)-fucoidanase Mef2, which formed fucose with novel α-(1,4)-linked fucosyl branch at C4 position. The structure of fucoidan, laminarin polysaccharide, and oligosaccharide can be seen in .
Figure 2. Structural illustration of fucoidan and laminarin polysaccharides [Citation87] and their oligosaccharides [Citation88].
![Figure 2. Structural illustration of fucoidan and laminarin polysaccharides [Citation87] and their oligosaccharides [Citation88].](/cms/asset/b13b99e3-9e51-4924-bddd-6bb2ac1179c2/kbie_a_2252659_f0002_b.gif)
Laminarin is a neutral and water-soluble polysaccharide extracted from brown seaweeds which is composed of β-(1,3) and β-(1,6) glucan in the ratio of 3:1 [Citation88] and a backbone of β-(1,3)-D-glucopyranose and 6-O branching [Citation87]. Laminarin differs based on the sugar at the reducing end, which contains M chains with D-mannitol and G chains with glucose [Citation88]. Laminarinase is an endo β-glucan enzyme that reduces laminarin into glucose by cleaving either (1→3), (1→4) &; (1→6) or mixed (1→3), (1→4) &; (1→6) linkages [Citation67]. The effect of acid hydrolysis has been evaluated using laminarin obtained from L. digitata [Citation39]. This resulted in laminarin oligosaccharides with different degrees of polymerization (DP) with respect to hydrolysis time, dose, and temperature. Based on DP, laminarin oligosaccharides with different sugar compositions (laminarbinose, laminaritriose, laminaritetrose, and laminaripentose) were formed, but the main chain β-D-Glcp-(1→3)-β-D-Glcp remained same.
5. Health-promoting applications of algal oligosaccharides
5.1. Anti-metabolic syndrome activity
Metabolic syndrome is a collection of metabolic dysfunctions of fats, proteins, carbohydrates, and other substances. This causes abdominal obesity, hyperglycemia, insulin resistance, hypertension, hypertriglyceridemia, fasting plasma glucose, dyslipidemia, post-inflammatory and post-thrombotic states. Metabolic syndrome is indicated by chronic low-grade inflammation, and these metabolic dysfunctions are considered the main precursors for cardiovascular diseases (CVDs), stroke, myocardial infarction, and developing diabetes especially type 2 diabetes mellitus, which affects people’s quality of health and decreases lifespan compared to normal healthy people. Changes in lifestyle, diet such as excessive consumption of fat, carbs, and sugar, and low-physical activity result in obesity which has increased the risks of CVDs and diabetes even at a very young age [Citation14,Citation15,Citation89,Citation90]. In addition to this, increased levels of ROS in the body stimulate the overaccumulation of oxidized low-density lipoproteins and induce metabolic syndrome [Citation6]. These conditions are initially diagnosed and treated based on symptoms using synthetic drugs. However, certain drugs induce undesired side effects and the efficacy of these medications becomes adverse with chronic administration [Citation14,Citation15]. In the past decades, various studies have suggested that natural products have potential benefits in metabolism such as anti-inflammation, anti-diabetic, anti-lipogenic, glucose, and insulin sensitivity; especially seaweed-based polysaccharides and oligosaccharides have tremendous applications in treating metabolic syndrome [Citation15,Citation20,Citation31,Citation65]. shows the schematic illustration of anti-metabolic activity.
5.2. Anti-diabetic activity
Diabetes is a chronic disease caused by environment factor and from metabolic dysfunction hereditary such as hormonal and immune imbalances. It can be diagnosed by prolonged high blood sugar level or diminishing function of pancreatic β cell which secretes insulin. Changes in lifestyle, higher consumption of alcohol, smoking, fat, and less physical activity are the main reasons for developing diabetes. Especially, obesity is the primary reason for 55% of type 2 diabetic cases worldwide. Whereas obesity is caused by insulin resistance, excess oxidative stress, and increased inflammatory marker expression [Citation41,Citation65,Citation90,Citation91]. In addition, polysaccharides and oligosaccharides derived from various seaweeds have potential prebiotic effects. These bioactive compounds can be fermented by gut microbiota to alter or resume intestinal function [Citation18].
In a previous study, glucose tolerance was enhanced in diabetic mice with polyguluronate sulfate and its oligosaccharides (PGS 12 and PGS 25) induced fibroblast growth factor 19 (FBF19) activity. This approach eventually enhanced FBF1/FBFR1c and FBF19/FBFR1c signaling and resulted in reduced blood glucose levels [Citation92]. The functional fuco-oligosaccharide was extracted from S. confusum, and its efficacy was evaluated in treating diabetics in both in vitro and in vivo modes using Hep G2 cells and hamsters, respectively [Citation65]. A group of hamsters were fed a high fat/high sucrose diet, where half of them were supplemented with 150 mg/kg body weight of oligosaccharides for 60 days. At the end of the trial, better glucose tolerance, low blood glucose level, and normal weight were observed in oligosaccharide-fed hamsters than in non-fed hamsters. Also, histology of livers confirmed the protective effects of oligosaccharides by inducing anti-lipogenic properties. The mRNA expression and protein profiling of GLUT4, IRS1, PI3K, and JNK1/2 gene models revealed the high hypoglycemic effect induced by oligosaccharides in regulating the JNK-IRS1/PI3K signaling pathway. Similarly, Wang et al. [Citation68] fed a group of mice with a high fat and chow diet to evaluate the efficacy of alginate oligosaccharides in lipid metabolism and its effect on gut microbiota. The study reveals that mice fed with alginate oligosaccharides have reduced blood sugar levels, low LDL-C, and low expression of lipogenesis genes than non-supplemented mice. Also, the oligosaccharides have increased the short-fatty acid concentration, inhibited endotoxin levels, and increased specific and beneficial gut microbes. Xing et al. [Citation20] and Zhang et al. [Citation12] have reported a detailed study of alginate oligosaccharides and their health applications. The results suggest low-molecular-weight marine oligosaccharides have shown higher hypoglycemic effects and increased insulin sensitivity. Laminarin oligosaccharides derived through acid hydrolysis exhibited an efficient anti-diabetic activity by inhibiting the activity of α-amylase up to 32.2% at 30 µg concentration. It exhibited IC50 of 46.6 µg/mL and α-glucoside activity up to 58.8% at 30 µg concentration with IC50 of 25.5 µg/mL. The authors mentioned that the maximum inhibition and antioxidant activity were observed in 2 h hydrolyzed samples. In contrast, less inhibition was observed in 1, 4, and 6 h hydrolyzed samples. This was due to insufficient and excessive hydrolysis of polysaccharides [Citation26]. Zheng et al. [Citation1] mentioned that the monosaccharide composition of oligosaccharides alters the anti-diabetic effects. For example, oligosaccharides composed of the odd number of monosaccharides inhibited the colonic microbiota dysbiosis, colitis, and type 2 diabetes mellitus, whereas oligosaccharides composed of an even number of monosaccharides reduced obesity and diabetes induced by alloxan and fat-rich diets.
5.3. Anti-inflammatory activity
Immune and inflammatory mechanisms protect the body from various injuries and pathological conditions, such as tumor, tissue damage, obesity, and CVDs. However, in certain situations, immune and inflammatory reactions can cause various diseases, such as autoimmune disease, cancer, granulomatous condition, respiratory, joint, and bone diseases. It can be treated with anti-inflammatory drugs that act as mediators, suppress pro-inflammatory cytokines, and block immune cells [Citation20,Citation93]. In the last decade, many reports have suggested that seaweeds polysaccharides and oligosaccharides have potential and efficient anti-inflammatory properties [Citation20,Citation28]. Wang et al. [Citation31] reported that prolonged consumption of anticancer drugs induces various side effects, including gastrointestinal inflammation. Such pharmacotherapy-induced inflammation can be treated using probiotics, selenium, volatile oils, and prebiotics. Inflammatory response is regulated by toll-like receptors and TLR4 signaling pathway [Citation20]. It can be activated by lipopolysaccharides or endotoxins, which initiates the inflammatory process by triggering the activation of nuclear factor (NF)-kB, different kinases such as MAP, Akt, and PI3K, nitric oxide (NO), prostaglandin E2 (PGE2), reactive oxygen species (ROS), nitric oxide synthase (iNOS), cyclooxygenase (COX)-2, and pro-inflammatory cytokines [Citation20,Citation28].
It was found that the alginate oligosaccharide prevented intestinal mucositis induced by busulfan, a chemotherapeutic drug using a mice model and IPEC-J2 cells [Citation94]. During the trial, alginate oligosaccharides enriched many cell clusters, including stem cells, transit-amplifying progenitors (TA), TAG1, TA-G2, enterocyte progenitors (EP), enterocyte progenitor early (EPE), enterocyte progenitor late (EPL), enterocyte immature proximal (EIP), enterocyte immature distal (EID), enterocyte matures proximal (EMP), enterocyte matures distal (EMD), EEC, small intestinal tuft cells, goblet cells, and Paneth cells. This aided to recover enterocytes and intestinal functions. However, the above study didn’t explain the intestinal cell integrity and migration, which was later studied by Xiong et al. [Citation95] using the IPEC-J2 cell lines. The study on the successive integrity of cell monolayers and cell migration by MR signaling pathway was confirmed by TEER analysis and wound healing capacity. The width of the wound was closed for 24 h after post-scratch (oligosaccharide concentration 10 µg/mL). These data confirmed the action of alginate oligosaccharide in the previous study and how it alleviated mucositis in a murine model. Zhou et al. [Citation33] reported that the guluronate oligosaccharide derived from alginate via oxidative degeneration has suppressed the over-expression of redundant inflammatory mediators (NO, PGE2, and ROS), inflammatory proteins (iNOS and COX-2), and pro-inflammatory cytokines (TNF-α, Il-1β, and IL-6) produced by LPS induced murine macrophage RAW264.7 cells. Also, the oligosaccharides hindered NF-κB and MAP kinase signaling pathways and TL4 and CD14 expressions by inhibiting the binding of LPS to cell surface. Similarly, Wang et al. [Citation31] reported the alginate oligosaccharides hindered the expression of IL-1β and CD11c inflammation markers.
5.4. Anti-obesity activity
Hyperplasia of adipocytes causes overaccumulation of fat in the body and results in obesity [Citation14,Citation96]. This increases endothelial dysfunction, leptin production, reactive oxygen species and decreased adiponectin production, and reduced nitric oxide availability [Citation97]. Obesity is defined by the BMI of the body, if the BMI (kg/m2) value is above 30 or the weight is ≥ 95% of BMI, the person is considered obese [Citation98,Citation99]. Excess body weight or obesity can cause diabetes, hypertension, abnormal lipid metabolism, insulin resistance, sleep apnea, nonalcoholic fatty liver disease, depression, and respiratory issues [Citation97,Citation98,Citation100]. Obesity can be controlled by losing weight and medication. Widely used anti-obesity drugs act on the gastrointestinal system or on the central nervous system to regulate lipid metabolism. The gastrointestinal drugs (e.g., Orlistat) use gastric and pancreatic lipase inhibitors which reduce the adsorption of dietary fats, whereas drugs that act on the central nervous system (e.g., sibutramine, rimonabant) suppress the appetite by enhancing the sensation of satiety [Citation101]. So far, as per the literature, only a few anti-obesity drugs are available, whereas some studies suggest that targeting the adipose tissue can be an efficient way to treat the obesity [Citation28]. It is necessary to find alternate medications since the existing anti-obesity drugs have adverse side effects, such as dizziness, vomiting, nausea, headache, palpitation, constipation, dry mouth, insomnia, hand tremor, and high blood pressure [Citation102–104].
Macroalgal products as whole extracts (aqueous/alcoholic) or as derived functional compounds (fucoidans, alginates, laminarin, etc.) have been proven to have anti-hypertensive/anti-obesity activity [Citation97]. Studies reported that gut microflora regulates obesity and energy metabolism. Also, the consumption of sodium alginate has been reported to reshape the gut microflora for better intestine function and metabolism [Citation20]. Commercial fucoidan extracted from brown seaweed has shown better anti-obesity activity when fed to mice (2% fucoidan + HFD), which eventually reduced the body mass, serum TG, TC, and LDL levels. Additionally, it suppresses the epididymal adipose tissue genes (PPAR-γ, A-FABP, and ACC), which help to regulate the fat accumulation in the body [Citation105]. Unsaturated alginate oligosaccharides extracted from S. japonica showed higher anti-obesity activity in HFD-fed mice than that of saturated alginate oligosaccharides. It was facilitated by reducing the body weight, serum lipids (triacylglycerol, total cholesterol, and free fatty acids), liver mass, TG and TC, serum alanine aminotransferase, aspartate aminotransferase, adipose mass, ROS formation, and fat accumulation. Also, the oligosaccharides enhance AMPK signaling by increasing AMP-activated protein kinase α and acetyl CoA carboxylase phosphorylation in adipocytes [Citation106]. Similarly, galactooligosaccharides have been used to treat obese rats, which was induced by HFD. The oligosaccharide reduced the fat accumulation without affecting the appetite, which significantly reduced the total serum cholesterol, including HDL, LDL, and triglycerides. Also, it improved the expressions of browning proteins, including PPAR-γ, PGC-1α, and PRDM16 in both brown and white adipose tissues. Moreover, it enhanced LXR- α, PPAR- α, LDL-R, and CYP7A1 in the liver of obesity-induced mice [Citation107].
6. Other potential applications of oligosaccharides
Seaweed-derived oligosaccharides have shown better bioactivities and higher commercial values. Some of the reported bioactivities of brown algal oligosaccharides are given in . The BSOSs obtained from various brown seaweeds and their potential commercial and industrial applications have been discussed below.
Table 2. Bioactivities and health benefits of brown algal oligosaccharides.
6.1. Food and nutraceuticals
Functional foods and/or nutraceuticals are novel food options that provide better nutrition and health benefits than traditional foods. These foods exert positive effects on physical and mental health performance besides their nutritional values the following are examples of functional foods: antioxidants, dietary supplements, fortified dairy products, cereals, herbals, milk, minerals, and vitamins [Citation1,Citation127,Citation128]. Higher consumption of carbohydrates leads to hyperglycemia or high glucose levels in blood serum, which results in excess ROS in the body. At higher ROS concentrations, it induces oxidative stress and leads to chronic diseases such as diabetes, insulin resistance, β-cell dysfunction, etc. It can be treated by inhibiting α-amylase and α-glucosidase activities to lower glucose levels. Hence, the most adaptable method is delaying glucose production and metabolism [Citation129].
Seaweeds are one of the main sources of nutraceutical supplements and are used as flavoring agents in noodles, soups, meals, snacks, etc., in Japan, China, Korea, and other coastal countries. Compared to whole seaweed, seaweed-derived products, e.g., polysaccharides, proteins, and polyphenols, have more nutritional benefits. In particular, oligosaccharides have more bioactivities due to their low molecular weight, water solubility, better surface area, and functional sites as well as relatively lower production costs [Citation130]. Hence, oligosaccharides derived from marine algae are potent functional foods. It is also consumed as dry powdered forms for various prebiotic applications, such as fructo-, galacto-, xylo-, mannano-, glycol-, and isomalto-oligosaccharides in daily diet as sweeteners, fibers, humectants, etc. [Citation1,Citation131]. During metabolism, the gut microbiota produces beneficial metabolites after utilizing marine oligosaccharides [Citation132]. Oligosaccharides exert positive effects on gut microflora, neoagarooligosaccharides enhance the population of Lactobacillus and Bifidobacterium in the gut, which reduces the putrefactive bacterial population in mice feces [Citation130]. Alginate oligosaccharides reduce the pathogenic bacteria population, such as Escherichia, Shigella, and Peptoniphilus sp., and enrich the Ruminococcaceae, Coprococcus, Roseburia, and Faecalibacterium sp. population in the gut [Citation17]. Specific microbes such as Ruminococcus sp. and Bacteroides sp. in the gut ferments mucopolysaccharides into short-chain fatty acids that include acetate, propionate, and butyrate. These are essential metabolites for the gut epithelial cells [Citation87]. Strong gelling properties of alginate can regulate appetite by slowing digestion and adsorption in the intestine. Thus, the risk of obesity and hypertension can be reduced. In particular, the alginate forms gel while reacting with multivalent cations due to rich in G-blocks that form strong ionic cross-linkages [Citation1,Citation20]. In a prebiotic study, sodium alginate significantly reduced the daily energy, carbohydrate, sugar, fat, and protein intake over a 7-day trial [Citation133]. Oligosaccharides derived from marine seaweeds have nutritional impacts on humans and animals. For example, laminarin oligosaccharides have improved hatching rate, embryo development, and blastocyst formation during in vitro studies. Moreover, it has been proven to suppress pro-inflammatory cytokines secretion, pathogens in the intestine, volatile fatty acid concentration, and improved gut health in pigs [Citation25].
6.2. Agriculture, animal feed, and fishery
Widespread use of chemical fertilizers and agrochemicals has significantly increased the risk of severe ecological threats and impacts on human health. Hence, various alternative sources such as genetic modification and plant growth stimulants have been adapted to replace chemical fertilizers. These include humic acid, fulvic acids, protein hydrolyzates, N-containing compounds, seaweed extracts, beneficial microorganisms or biofertilizers, botanicals, chitosan, other biopolymeric inorganic compounds, etc [Citation134]. In recent years, seaweeds or marine algae-derived oligosaccharides have shown a potential to induce growth in plants and animals. With their low molecular size and unsaturated double bonds, algal oligosaccharides find several fascinating applications. It has exhibited plant growth-promoting activity by improving N and C absorption, basal metabolism, and cell division. Moreover, the natural anti-microbial activity of oligo-containing essential oil/PPC acts as protective guard [Citation1,Citation135]. Alginate oligosaccharides extracted by enzymatic digestion showed the better growth-promoting activity in the green microalga Chlamydomonas reinhardtii than oligosaccharides extracted by acid hydrolysis. This is due to the different degrees of polymerization and loss of functional sites during extraction. In addition, the oligosaccharide has enhanced the fatty acid concentration in C. reinhardtii [Citation122]. Similarly, alginate oligosaccharides obtained through enzymatic hydrolysis with low molecular weight (0.5–3 kDa) and G/M ratio > 1 have shown better growth-promoting activity in Hordeum vulgare L. due to enhanced absorption, and the oligosaccharides with molecular weigh 573.8 and 2370.5 Da have shown better root growth in seedlings [Citation134].
Huang et al. [Citation25] discussed the various applications of laminarin oligosaccharides in plant disease control and growth-promoting activities. The results propound that laminarin oligosaccharides can reduce leaf spot and powdery mildew incidents by 50 and 70–80%, it can also hinder the growth and aflatoxin production of Aspergillus flavus and an infection caused by pathogen Botrytis cinera up to 80% in plants. In addition, laminarin oligosaccharides enhanced the germination by inducing amylase formation, which can affect abiotic stress by regulating the DEFL mediated pathway. Alginate oligosaccharides individually and in combination with Meyerozyma guilliermondii yeast inhibited Penicillium italicum (blue mold) growth on mandarin fruit and also prevented water loss from fruit, which made it a better protectant for storing fruits post-harvest [Citation136].
The addition of marine algae-derived oligosaccharides to animal feed has increased the post-harvest shelf-life of fruits and frozen food by acting as cryoprotective agents [Citation1]. The results show that the fruits post-harvest shelf life was increased through suppression of abscisic acid signaling, gene expression, and cell wall degradation by agar-oligosaccharides. The addition of laminarin to animal feed has improved growth performance, immune response, and gut-microbiota in animals. The research outcomes denote that laminarin hindered the secretion of pro-inflammatory cytokines in pigs and improved their gut microflora. Furthermore, the pork meat quality has also been improved by adding fucoidan and laminarin as food additives. This reduced saturated fatty acids and lipid oxidation in the longissimus thoracis and lumborum muscles of pigs [Citation25]. In addition to this, laminarin was used as an additive in fish cultivation, which has the potential to regulate immune response and growth promotion in fish. Laminarin increased the hatching rate, blastocyst formation rate, and blastocyst cell numbers by reducing oxidase stress when used as a food additive in porcine early-stage embryo development [Citation137]. Zheng et al. [Citation1] reported shrimp storage stability can be increased by suppressing the degradation of myofibrillar protein by treating them with marine algae oligosaccharides prior to the frozen storage.
6.3. Bio-medical
A study reports that only 50% of the world’s population has access to essential medicines, while others cannot afford them due to high prices and low-purchasing power. It can be overcome by combining traditional medicine with modern medicine like in Canada and France. Traditional medicine uses seaweeds, plants, herbs, etc., to treat diseases and disorders. Since seaweeds possess numerous bioactivities and they are nontoxic, edible, cheap, and easy to cultivate, they can be used in biomedical applications [Citation138]. Drugs are manufactured using active compounds derived from synthetic chemicals and biological sources. Due to their high solubility and low molecular weight, oligosaccharides are preferred for drug production, since they can easily mix with the bloodstream and ooze curative effects [Citation139]. Zheng et al. [Citation1] have explored the potential of marine-derived algae in biomedical and pharmaceutical applications. According to a recent study, oligosaccharides-based nasal spray has potential to suppress common cold. Likewise, the oligosaccharides helped regulate mucus formation due to cystic fibrosis. Also, it has been noted that the regulating efficiency was higher than marine algae-derived polysaccharides. This is because of the low-molecular-weight and absence of intermolecular crosslinks. The use of alginate oligosaccharide pretreatment in mice prevented cardiotoxicity (includes oxidative stress and activated endoplasmic reticulum mediated apoptosis) caused by doxorubicin, a potent chemotherapeutic agent. During treatment, the alginate oligosaccharide suppressed phox and 4-HNE gene expression (related to oxidative stress) as well as CHOP and bax (endoplasmic reticulum mediated apoptosis) gene expression. It also improved the gene expression of anti-apoptotic protein Bcl-2 [Citation121]. Similarly, the growth performance of weaned pigs was studied with alginate oligosaccharides produced by enzymatic hydrolysis, which improved intestinal morphology and barrier function as well as hindered enterocyte death via reducing apoptosis by modulating mitochondria-dependent apoptosis [Citation123].
Dental implants and removable dental dentures are prone to bacterial and fungal infection, which requires extra care to prevent such microbe colonization. An in vitro study has been conducted with alginate oligomers and triclosan, individually and in combination. From the study, the oligomer and triclosan combination showed potential anti-microbial activity against Streptococcus mutants and Porphyromonas gingivalis. Moreover, the pre-treatment with oligomer and triclosan hindered P. gingivalis from attaching to the titanium brace. This proves that the alginate oligomers can be used for dental care, and if combined with triclosan, it could reduce the concentration of triclosan in oral care products [Citation124]. Oligo-fucoidan reduced the occurrence of spontaneous DNA lesions in human colorectal cancer HCT116 cells as well as Oligo-fucoidan supplementation increased cell mortality and decreased pro-inflammatory cytokine IL-6 and chemokine CCL2/MCP-1, plus it inhibited the tumor-promoting macrophages, which shows the ability of oligo-fucoidans in suppressing chemotherapy side effects [Citation140]. Likewise, low-molecular-weight fucoidan (>2 kDa) extracted from Undaria pinnatifida has shown better anti-tumor activity against breast cancer cells MCF-7 and MDA-MB-231. The study proves that anti-tumor activity increases with decreasing molecular weight. During trials, fucoidan induced NO expression in MDA-MB-231 cells, which suppressed apoptosis [Citation141]. Zheng et al. [Citation1]. Furthermore, they explained the benefits of oligosaccharides combined with other drugs and their therapeutical applications. As reported by the authors, fucoidan combined with gelatin served as a more effective chemo-targeting agent, while alginate gel mixed with gelatin produced a 3D-porous matrix, which was stronger in terms of biocompatibility and wound healing properties.
6.4. Cosmetics
The cosmetics sector offers an evergreen global business, as people use a wide range of cosmetic products daily [Citation142]. In the past decade, the demand for safe and high-quality cosmetics production has dramatically increased. According to recent reports, seaweed-derived oligosaccharides have gained remarkable attention because of their biocompatible property, antioxidant, anti-melanogenic, and UV-protection properties [Citation1]. These properties help get rid of acne, skin darkening, wound healing, hair growth, and cosmetic stabilization [Citation1,Citation5,Citation125]. Anti-melanogenesis activity of fucoidans extracted from Undaria pinnatifida with different molecular weights was evaluated using B16BL6 Melanoma cells. The study showed that melanin content decreased up to 50% with 6–17 kDa fucoidans. In addition, 65–75% tyrosine inhibition was also higher at this molecular-weight range. Furthermore, the radical scavenging activity increased with decreasing molecular-weight [Citation126]. Similarly, Low-molecular-weight fucoidans (5–10 kDa) derived from Saccharina japonica showed better skin whitening activity by efficient antioxidant activity, anti-melanogenic activity, and inhibition of tyrosinase, using B16 mouse melanoma [Citation143]. The research indicates that for oligosaccharide biosynthesis, enzymatic degradation of biomass is safer than other methods, such as hydrothermal degradation, acid hydrolysis, and oxidative degradation, since they might have undesired residues which are unsafe for dermal applications. Alginate oligosaccharides synthesized by 6, 12, and 24 h of enzymatic hydrolysis have been effective for collagen expression in HS27 human dermal fibroblasts, and oligosaccharides obtained by 24 h hydrolysis have shown better matrix metalloproteinase-1 (MMP-1) inhibition at higher concentration and increased expression of tissue inhibitor metalloproteinase-1 (TIMP-1) with increasing dose concentration. As a result, it promoted the synthesis of Col-I and Col-III. The results suggest that the collagen synthesis by inhibiting MMP-1 delays the aging and prevents skin wrinkles [Citation125]. Fucoidan oligosaccharides obtained from Sargassum glaucescens have shown hair growth activity in hair follicles and dermal papilla cells in mice models. It enhanced the expression of IGF-1, LEF-1, and FGF-1 gene expression which resulted in better hair growth [Citation5].
7. Current status and prospects on microalgal oligosaccharide
Macroalgae are a major source of marine polysaccharides and oligosaccharides and find major applications in food industries, pharmaceuticals, nutraceuticals, cosmeceuticals, etc. It has been apparent in recent years that microalgae are an emerging platform to produce algal polysaccharides and oligosaccharides [Citation144]. The major advantage of this platform is its scale-up potential and attractive productivity, especially in mixotrophic conditions [Citation145,Citation146]. This has become more promising with the merging of new technologies such as nanobubbles and nanoparticles [Citation147,Citation148]. In addition to providing bioactive polysaccharides and oligosaccharides, their biomass also accumulates other high-value products such as omega fatty acids, carotenoids, lipids, etc. [Citation149–154]. Moreover, the microalgae platform’s advantages are not limited to many products. They can offset production and harvesting costs [Citation22,Citation96,Citation148,Citation150,Citation152–155]. It also offers other advantages such as CO2 and other greenhouse gas mitigation, bioremediation, bio-desalination, carbon footprint improvement, etc. [Citation155–160]. By focusing on polysaccharide and oligosaccharide production as the main products, all these advantages can be exploited through a biorefinery approach.
8. Challenges and perspectives of seaweed oligosaccharides
Despite various advantages, there are some issues to resolve in the future. The diversity and complexity of macroalgal biomass and polysaccharides have been highlighted as the main obstacles to oligosaccharide research. As a result, there is no uniform chemo/enzymatic or enzymatic method for the feasible production of OSs from all types of algal biomass. Additionally, research has not yet developed sufficiently to offer an adequate link between molecular size, functional group, and bioactivity. This link may be used to manufacture products with specific health uses. To understand the process for feasible or efficient oligosaccharide production and thorough characterization before designing the precise application in the future, further developments are necessary to have a sufficient database. One of the major drawbacks is industrial-scale production and purification, which is limited for various reasons. It includes seaweed availability [Citation161], extraction efficiency [Citation162], purification efficiency [Citation73], cost, etc. For example, industrial fructo-oligosaccharide production could have been improved due to process optimization, enzyme selection, expensive purification methods, and low yield [Citation76].
9. Conclusions
This review brings research advances in brown seaweed oligosaccharides (BSOSs) extraction, purification, characterization, and potential applications. Despite the higher extraction yield (30–58%), physical and chemical extraction methods produced less bioactive OSs due to less specific cleavage, harsh chemicals, and abrasive conditions, whereas enzymatic extractions offered relatively less OS yields, but yielded highly bioactive OSs with higher DP, site-specificity, and feasibility. However, using pure enzymes for hydrolysis is not economically feasible. Therefore, microbial fermentation using various fungi has been used to produce BSOSs with 41–81.5% reduced MW from native fucoidan. The purity of BSOSs is one of the major limiting factors in their industrial applications; however, using nanofiltration and activated carbon adsorption methods, the BSOS can be utilized at a large scale, yet further research is required to avoid membrane fouling and repeatability. BSOSs possess a variety of bioactivities in nutraceuticals, agriculture, biomedical, and cosmetics. In particular, BSOSs controlled the anti-metabolic syndrome, in both in vivo and in vitro conditions by inducing anti-lipogenic, anti-diabetic, and anti-inflammatory properties. However, further research is required for industrial-scale production, purification, and characterization before demonstrating their potential applications.
CRediT authorship contribution statement
Pitchurajan Krishna Perumal: Writing original draft, literature review; Chun-Yung Huang: Supervision, draft preparation; Chiu-Wen Chen: Supervision, draft preparation; Grace Sathyanesan Anisha: Writing – review, and editing, draft preparation; Reeta Rani Singhania: Supervision, writing review, and editing.; Cheng-Di Dong: Supervision, writing review and editing; supervision; Anil Kumar Patel: Supervision, validation, conceptualization, writing review and editing.
Disclosure statement
No potential conflict of interest was reported by the author(s).
Additional information
Funding
References
- Zheng L-X, Liu Y, Tang S, et al. Preparation methods, biological activities, and potential applications of marine algae oligosaccharides: a review. Food Sci Hum Wellness. 2023;12(2):359–27. doi: 10.1016/j.fshw.2022.07.038
- Mohd Fauziee NA, Chang LS, Wan Mustapha WA, et al. Functional polysaccharides of fucoidan, laminaran and alginate from Malaysian brown seaweeds (Sargassum polycystum, Turbinaria ornata and Padina boryana). Int J Biol Macromol. 2021;167:1135–1145. PMID 33188815. doi: 10.1016/j.ijbiomac.2020.11.067
- Myklestad SM, Granum E. Chapter 4.2. Biology of (1,3)-β-glucans and related glucans in protozoans and chromistans. In: Bacic A, Fincher G Stone B, editors. Chemistry, biochemistry, and biology of 1-3 beta glucans and related polysaccharides. San Diego: Academic Press; 2009. pp. 353–385. doi: 10.1016/B978-0-12-373971-1.00010-8
- Zhang L, Liao W, Huang Y, et al. Global seaweed farming and processing in the past 20 years. Food Prod Process And Nutr. 2022;4(1):23. doi: 10.1186/s43014-022-00103-2
- Huang C-Y, Huang C-Y, Yang C-C, et al. Hair growth-promoting effects of Sargassum glaucescens oligosaccharides extracts. J Taiwan Inst Chem Eng. 2022;134:104307. doi: 10.1016/j.jtice.2022.104307
- Zaitseva OO, Sergushkina MI, Khudyakov AN, et al. Seaweed sulfated polysaccharides and their medicinal properties. Algal Res. 2022;68:102885. doi: 10.1016/j.algal.2022.102885
- Soukaina B, Zainab EA, Guillaume P, et al. Radical depolymerization of alginate extracted from Moroccan brown seaweed Bifurcaria bifurcata. Appl Sci. 2020;10(12):4166. doi: 10.3390/app10124166
- Zhang X, Liu Y, Chen X-Q, et al. Catabolism of Saccharina japonica polysaccharides and oligosaccharides by human fecal microbiota. LWT. 2020;130:109635. doi: 10.1016/j.lwt.2020.109635
- Gurpilhares D, Gurpilhares DB, Cinelli LP, et al. Marine prebiotics: polysaccharides and oligosaccharides obtained by using microbial enzymes. Food Chem. 2019;280:175–186. PMID 30642484. doi: 10.1016/j.foodchem.2018.12.023
- Nordgård CT, Rao SV, Draget KI. The potential of marine oligosaccharides in pharmacy. Bioact Carbohydr Diet Fibre. 2019;18:100178. doi: 10.1016/j.bcdf.2019.100178
- Suprunchuk VE. Low-molecular-weight fucoidan: chemical modification, synthesis of its oligomeric fragments and mimetics. Carbohydr Res. 2019;485:107806. PMID 31526929. doi: 10.1016/j.carres.2019.107806
- Zhang C, Wang W, Zhao X, et al. Preparation of alginate oligosaccharides and their biological activities in plants: a review. Carbohydr Res. 2020;494:108056. PMID 32559511. doi: 10.1016/j.carres.2020.108056
- Gabbia D, Saponaro M, Sarcognato S, et al. Fucus vesiculosus and Ascophyllum nodosum ameliorate liver function by reducing diet-induced steatosis in rats. Mar Drugs. 2020;18(1):62. PMID 31963560. doi: 10.3390/md18010062
- Shih M-K, Hou C-Y, Dong C-D, et al. Production and characterization of Durvillaea Antarctica enzyme extract for antioxidant and anti-metabolic syndrome effects. Catalysts. 2022;12(10):1284. doi: 10.3390/catal12101284
- Gabbia D, De Martin S. Brown seaweeds for the management of metabolic syndrome and associated diseases. Molecules. 2020;25(18):4182. PMID 32932674. doi: 10.3390/molecules25184182
- Charoensiddhi S, Conlon MA, Vuaran MS, et al. Impact of extraction processes on prebiotic potential of the brown seaweed Ecklonia radiata by in vitro human gut bacteria fermentation. J Funct Foods. 2016;24:221–230. doi: 10.1016/j.jff.2016.04.016
- Han ZL, Yang M, Fu XD, et al. Evaluation of prebiotic potential of three marine algae oligosaccharides from enzymatic hydrolysis. Mar Drugs. 2019;17(3):173. PMID 30889794. doi: 10.3390/md17030173
- Shang Q, Jiang H, Cai C, et al. Gut microbiota fermentation of marine polysaccharides and its effects on intestinal ecology: an overview. Carbohydr Polym. 2018;179:173–185. PMID 29111040. doi: 10.1016/j.carbpol.2017.09.059
- Huang W, Tan H, Nie S. Beneficial effects of seaweed-derived dietary fiber: highlights of the sulfated polysaccharides. Food Chem. 2022;373(B):131608. PMID 34815114. doi: 10.1016/j.foodchem.2021.131608
- Xing M, Cao Q, Wang Y, et al. Advances in research on the bioactivity of alginate oligosaccharides. Mar Drugs. 2020;18(3):144. PMID 32121067. doi: 10.3390/md18030144
- Patel AK, Singhania RR, Awasthi M, et al. Emerging role of macro- and microalgae as prebiotic. Microb Cell Factories. 2021c;20(1):112. doi: 10.1186/s12934-021-01601-7
- Patel AK, Vadrale AP, Singhania RR, et al. Algal polysaccharide: current status and future perspectives. Phytochem Rev. 2022a;22(4):1167–1196. doi: 10.1007/s11101-021-09799-5
- Sellimi S, Kadri N, Barragan-Montero V, et al. Fucans from a Tunisian brown seaweed Cystoseira barbata: structural characteristics and antioxidant activity. Int j biol macromol. 2014;66:281–288. PMID 24582933. doi: 10.1016/j.ijbiomac.2014.02.041
- Wu SJ. Preparation and antioxidant activity of the oligosaccharides derived from Laminaria japonica. Carbohydr Polym. 2014;106:22–24. PMID 24721046. doi: 10.1016/j.carbpol.2014.01.098
- Huang Y, Jiang H, Mao X, et al. Laminarin and laminarin oligosaccharides originating from brown algae: preparation, biological activities, and potential applications. J Ocean Univ China. 2021;20(3):641–653. doi: 10.1007/s11802-021-4584-8
- Jayapala N, Toragall V, GK BS, et al. Preparation, characterization, radical scavenging property and antidiabetic potential of laminarioligosaccharides derived from laminarin. Algal Res. 2022;63:102642. doi: 10.1016/j.algal.2022.102642
- Liu M, Liu L, Zhang H-F, et al. Alginate oligosaccharides preparation, biological activities and their application in livestock and poultry. Journal Of Integrative Agriculture. 2021;20(1):24–34. doi: 10.1016/S2095-3119(20)63195-1
- Mrudulakumari Vasudevan U, Lee OK, Lee EY. Alginate derived functional oligosaccharides: recent developments, barriers, and future outlooks. Carbohydr Polym. 2021;267:118158. PMID 34119132. doi: 10.1016/j.carbpol.2021.118158
- Cheong KL, Qiu HM, Du H, et al. Oligosaccharides derived from red seaweed: production, properties, and potential health and cosmetic applications. Molecules. 2018;23(10):2451. PMID 30257445. doi: 10.3390/molecules23102451
- Nazarudin MF, Alias NH, Balakrishnan S, et al. Chemical, nutrient and physicochemical properties of brown seaweed, Sargassum polycystum C. Agardh (Phaeophyceae) collected from Port Dickson, Peninsular Malaysia. Molecules. 2021;26(17):5216. PMID 34500650. doi: 10.3390/molecules26175216
- Wang M, Chen L, Zhang Z. Potential applications of alginate oligosaccharides for biomedicine – a mini review. Carbohydr Polym. 2021;271:118408. PMID 34364551. doi: 10.1016/j.carbpol.2021.118408
- Zúñiga EA, Matsuhiro B, Mejías E. Preparation of a low-molecular weight fraction by free radical depolymerization of the sulfated galactan from Schizymenia binderi (Gigartinales, Rhodophyta) and its anticoagulant activity. Carbohydr Polym. 2006;66(2):208–215. doi: 10.1016/j.carbpol.2006.03.007
- Zhou R, Shi X, Gao Y, et al. Anti-inflammatory activity of guluronate oligosaccharides obtained by oxidative degradation from alginate in lipopolysaccharide-activated murine macrophage RAW 264.7 cells. J Agric Food Chem. 2015;63(1):160–168. PMID 25483391. doi: 10.1021/jf503548a
- Yang Z, Li J-P, Guan H-S. Preparation and characterization of oligomannuronates from alginate degraded by hydrogen peroxide. Carbohydrate Polymers. 2004;58(2):115–121. doi: 10.1016/j.carbpol.2004.04.022
- Okolie CL, Mason B, Mohan A, et al. The comparative influence of novel extraction technologies on in vitro prebiotic-inducing chemical properties of fucoidan extracts from Ascophyllum nodosum. Food Hydrocoll. 2019;90:462–471. doi: 10.1016/j.foodhyd.2018.12.053
- Li X, Xu A, Xie H, et al. Preparation of low molecular weight alginate by hydrogen peroxide depolymerization for tissue engineering. Carbohydr Polym. 2010;79(3):660–664. doi: 10.1016/j.carbpol.2009.09.020
- Yudiati E, Santosa GW, Tontowi MR, et al. Optimization of alginate alkaline extraction technology from Sargassum polycystum and its antioxidant properties. IOP Conf S Earth Environ Sci. 2018;139(1):012052. doi: 10.1088/1755-1315/139/1/012052
- Ermakova S, Men’shova R, Vishchuk O, et al. Water-soluble polysaccharides from the brown alga Eisenia bicyclis: Structural characteristics and antitumor activity. Algal Res. 2013;2(1):51–58. doi: 10.1016/j.algal.2012.10.002
- Cheong KL, Li JK, Zhong S. Preparation and structure characterization of high-value laminaria digitata oligosaccharides. Front Nutr. 2022;9:945804. PMID 35873409. doi: 10.3389/fnut.2022.945804
- Hu T, Li C, Zhao X, et al. Preparation and characterization of guluronic acid oligosaccharides degraded by a rapid microwave irradiation method. Carbohydr Res. 2013;373:53–58. PMID 23584235. doi: 10.1016/j.carres.2013.03.014
- Wang S-H, Huang C-Y, Chen C-Y, et al. Isolation and purification of brown algae fucoidan from Sargassum siliquosum and the analysis of anti-lipogenesis activity. Biochem Eng J. 2021;165:107798. doi: 10.1016/j.bej.2020.107798
- Aida TM, Yamagata T, Watanabe M, et al. Depolymerization of sodium alginate under hydrothermal conditions. Carbohydr Polym. 2010;80(1):296–302. doi: 10.1016/j.carbpol.2009.11.032
- Choi JI, Kim HJ. Preparation of low molecular weight fucoidan by gamma-irradiation and its anticancer activity. Carbohydr Polym. 2013;97(2):358–362. PMID 23911457. doi: 10.1016/j.carbpol.2013.05.002
- Sun M, Sun C, Xie H, et al. A simple method to calculate the degree of polymerization of alginate oligosaccharides and low molecular weight alginates. Carbohydr Res. 2019;486:107856. PMID 31689577. doi: 10.1016/j.carres.2019.107856
- Becker S, Scheffel A, Polz MF, et al. Accurate quantification of laminarin in marine organic matter with enzymes from marine microbes. Appl Environ Microbiol. 2017;83(9): PMID 28213541. doi: 10.1128/AEM.03389-16
- Li L, Jiang X, Guan H, et al. Preparation, purification and characterization of alginate oligosaccharides degraded by alginate lyase from Pseudomonas sp. HZJ 216. Carbohydr Res. 2011;346(6):794–800. PMID 21371694. doi: 10.1016/j.carres.2011.01.023
- Wang D, Kim DH, Seo N, et al. A novel glycoside hydrolase family 5 β-1,3-1,6-Endoglucanase from saccharophagus degradans 2-40T and its transglycosylase activity. Appl Environ Microbiol. 2016;82(14):4340–4349. PMID 27208098. doi: 10.1128/AEM.00635-16
- Mitsuya D, Sugiyama T, Zhang S, et al. Enzymatic properties and the gene structure of a cold-adapted laminarinase from Pseudoalteromonas species LA. J Biosci Bioeng. 2018;126(2):169–175. PMID 29627318. doi: 10.1016/j.jbiosc.2018.02.018
- Shiao W-C, Kuo C-H, Tsai Y-H, et al. In vitro evaluation of anti-colon cancer potential of crude extracts of fucoidan obtained from Sargassum glaucescens pretreated by compressional-puffing. Appl Sci. 2020;10(9):3058. doi: 10.3390/app10093058
- Sumiyoshi W, Miyanishi N, Nakakita S-I, et al. An alternative strategy for structural glucanomics using β-gluco-oligosaccharides from the brown algae Ecklonia stolonifera as models. Bioact Carbohydr Diet Fibre. 2015;5(2):137–145. doi: 10.1016/j.bcdf.2015.03.002
- Ale MT, Meyer AS. Fucoidans from brown seaweeds: an update on structures, extraction techniques and use of enzymes as tools for structural elucidation. RSC Adv. 2013;3(22):8131–8141. doi: 10.1039/C3RA23373A
- Coelho E, Rocha MA, Saraiva JA, et al. Microwave superheated water and dilute alkali extraction of brewers’ spent grain arabinoxylans and arabinoxylo-oligosaccharides. Carbohydr Polym. 2014;99:415–422. PMID 24274525. doi: 10.1016/j.carbpol.2013.09.003
- Hakala TK, Liitiä T, Suurnäkki A. Enzyme-aided alkaline extraction of oligosaccharides and polymeric xylan from hardwood kraft pulp. Carbohydr Polym. 2013;93(1):102–108. PMID 23465907. doi: 10.1016/j.carbpol.2012.05.013
- Spadoni Andreani E, Karboune S. Comparison of enzymatic and microwave-assisted alkaline extraction approaches for the generation of oligosaccharides from American Cranberry (Vaccinium macrocarpon) Pomace. J Food Sci. 2020;85(8):2443–2451. PMID 32691432. doi: 10.1111/1750-3841.15352
- Liu J, Yang S, Li X, et al. Alginate oligosaccharides: production, biological activities, and potential applications. Compr Rev Food Sci Food Saf. 2019;18(6):1859–1881. PMID 33336967. doi: 10.1111/1541-4337.12494
- Li G-Y, Luo Z-C, Yuan F, et al. Combined process of high-pressure homogenization and hydrothermal extraction for the extraction of fucoidan with good antioxidant properties from Nemacystus decipients. Food And Bioproducts Processing. 2017;106:35–42. doi: 10.1016/j.fbp.2017.08.002
- Shi D, Qi J, Zhang H, et al. Comparison of hydrothermal depolymerization and oligosaccharide profile of fucoidan and fucosylated chondroitin sulfate from Holothuria floridana. Int j biol macromol. 2019;132:738–747. PMID 30904529. doi: 10.1016/j.ijbiomac.2019.03.12
- Saravana PS, Cho YN, Patil MP, et al. Hydrothermal degradation of seaweed polysaccharide: characterization and biological activities. Food Chem. 2018;268:179–187. PMID 30064746. doi: 10.1016/j.foodchem.2018.06.077
- Stefaniak Vidarsson M, Gudjonsdottir M, Sigurjonsson O, et al. Fucoidan oligosaccharides – bioactive and therapeutic potential. Encycl Mar Biotechnol. 2020;2:1133–1139. doi: 10.1002/9781119143802.ch46
- Zhang C, Li M, Rauf A, et al. Process and applications of alginate oligosaccharides with emphasis on health beneficial perspectives. Crit Rev Food Sci Nutr. 2021;63:1–19.
- El-Mohdy HLA. Radiation-induced degradation of sodium alginate and its plant growth promotion effect. Arab J Chem. 2017;10:S431–8. doi: 10.1016/j.arabjc.2012.10.003
- Quitain AT, Kai T, Sasaki M, et al. Microwave–hydrothermal extraction and degradation of fucoidan from supercritical carbon dioxide deoiled Undaria pinnatifida. Ind Eng Chem Res. 2013;52(23):7940–7946. doi: 10.1021/ie400527b
- Kadam SU, O’Donnell CP, Rai DK, et al. Laminarin from Irish brown seaweeds Ascophyllum nodosum and laminaria Hyperborea: ultrasound assisted extraction, characterization and bioactivity. Mar Drugs. 2015;13(7):4270–4280. PMID 26184235. doi: 10.3390/md13074270
- Zha S, Zhao Q, Zhao B, et al. Molecular weight controllable degradation of Laminaria japonica polysaccharides and its antioxidant properties. J Ocean Univ China. 2016;15(4):637–642. doi: 10.1007/s11802-016-2943-7
- Yang CF, Lai SS, Chen YH, et al. Anti-diabetic effect of oligosaccharides from seaweed Sargassum confusum via JNK-IRS1/PI3K signalling pathways and regulation of gut microbiota. Food Chem Toxicol. 2019;131:110562. PMID 31181236. doi: 10.1016/j.fct.2019.110562
- Tran VHN, Nguyen TT, Meier S, et al. The endo-α(1,3)-fucoidanase Mef2 releases uniquely branched oligosaccharides from Saccharina latissima fucoidans. Mar Drugs. 2022;20(5):305. PMID 35621956. doi: 10.3390/md20050305
- Rocher DF, Cripwell RA, Viljoen-Bloom M. Engineered yeast for enzymatic hydrolysis of laminarin from brown macroalgae. Algal Res. 2021;54:102233. doi: 10.1016/j.algal.2021.102233
- Wang Y, Li L, Ye C, et al. Alginate oligosaccharide improves lipid metabolism and inflammation by modulating gut microbiota in high-fat diet fed mice. Appl Microbiol Biotechnol. 2020;104(8):3541–3554. PMID 32103315. doi: 10.1007/s00253-020-10449-7
- Zhu B, Ni F, Xiong Q, et al. Marine oligosaccharides originated from seaweeds: source, preparation, structure, physiological activity and applications. Crit Rev Food Sci Nutr. 2021;61(1):60–74. PMID 31968996. doi: 10.1080/10408398.2020.1716207
- Hifney AF, Fawzy MA, Abdel-Gawad KM, et al. Upgrading the antioxidant properties of fucoidan and alginate from Cystoseira trinodis by fungal fermentation or enzymatic pretreatment of the seaweed biomass. Food Chem. 2018;269:387–395. PMID 30100450. doi: 10.1016/j.foodchem.2018.07.026
- Li SY, Wang ZP, Wang LN, et al. Combined enzymatic hydrolysis and selective fermentation for green production of alginate oligosaccharides from Laminaria japonica. Bioresour Technol. 2019;281:84–89. PMID 30802819. doi: 10.1016/j.biortech.2019.02.056
- Li G, Dai Y, Wang X, et al. Molecularly imprinted polymers modified by deep eutectic solvents and ionic liquids with two templates for the simultaneous solid-phase extraction of fucoidan and laminarin from marine kelp. Anal Lett. 2019;52(3):511–525. doi: 10.1080/00032719.2018.1471697
- Rajauria G, Ravindran R, Garcia-Vaquero M, et al. Molecular characteristics and antioxidant activity of laminarin extracted from the seaweed species Laminaria Hyperborea, using hydrothermal-assisted extraction and a multi-step purification procedure. Food Hydrocoll. 2021;112:106332. doi: 10.1016/j.foodhyd.2020.106332
- Uliyanchenko E. Size-exclusion chromatography—from high-performance to ultra-performance. Anal Bioanal Chem. 2014;406(25):6087–6094. PMID 25116601. doi: 10.1007/s00216-014-8041-z
- Mei J, Shao J, Wang Q, et al. Separation and quantification of neoagaro-oligosaccharides. J Food Sci Technol. 2013;50(6):1217–1221. PMID 24426038. doi: 10.1007/s13197-011-0448-3
- Nobre C, Teixeira JA, Rodrigues LR. New trends and technological challenges in the industrial production and purification of fructo-oligosaccharides. Compr Rev Food Sci Food Saf. 2015;55(10):1444–1455. doi: 10.1080/10408398.2012.697082
- Kuhn RC, Filho FM. Purification of fructooligosaccharides in an activated charcoal fixed bed column. N Biotechnol. 2010;27(6):862–869. doi: 10.1016/j.nbt.2010.05.008
- Kuhn RC, Palacio L, Prádanos P, et al. Selection of membranes for purification of fructooligosaccharides. Desalin Water Treat. 2011;27(1–3):18–24. doi: 10.5004/dwt.2011.2038
- Goulas AK, Kapasakalidis PG, Sinclair HR, et al. Purification of oligosaccharides by nanofiltration. J Membr Sci. 2002;209(1):321–335, ISSN 0376–7388. doi: 10.1016/S0376-7388(02)00362-9
- Pruksasri S, Nguyen TH, Novalin S, et al. Purification of heterooligosaccharides by microbial treatment and nanofiltration. Int J Food Sci Technol. 2023;58(5):2618–2624. doi: 10.1111/ijfs.16414
- Feng YM, Chang XL, Wang WH, et al. Separation of galacto-oligosaccharides mixture by nanofiltration. J Taiwan Inst Chem Eng. 2009;40(3):326–332. ISSN 1876-1070. doi: 10.1016/j.jtice.2008.12.003
- Kailemia MJ, Ruhaak LR, Lebrilla CB, et al. Oligosaccharide analysis by mass spectrometry: a review of recent developments. Anal Chem. 2014;86(1):196–212. PMID 24313268. doi: 10.1021/ac403969n
- Holtan S, Zhang Q, Strand WI, et al. Characterization of the hydrolysis mechanism of polyalternating alginate in weak acid and assignment of the resulting MG-oligosaccharides by NMR spectroscopy and ESI−mass spectrometry. Biomacromolecules. 2006;7(7):2108–2121. PMID 16827577. doi: 10.1021/bm050984q
- Veena N, Neelam U, Battula Surendra N, et al. Advances in fractionation and analysis of milk carbohydrates. In: Nurcan K, editor. Technological approaches for novel applications in dairy processing. Rijeka: IntechOpen; 2018. p. Ch. 7.
- Thanh TTT, Quach TTM, Tran VTT, et al. Structural characteristics and biological activity of different alginate blocks extracted from brown seaweed Turbinaria ornata. J Carbohydr Chem. 2021;40(1–3):97–114. doi: 10.1080/07328303.2021.1928155
- Tran VHN, Perna V, Mikkelsen MD, et al. A new FTIR assay for quantitative measurement of endo-fucoidanase activity. Enzyme Microb Technol. 2022;158:110035. PMID 35489196. doi: 10.1016/j.enzmictec.2022.110035
- Xie XT, Cheong KL. Recent advances in marine algae oligosaccharides: structure, analysis, and potential prebiotic activities. Crit Rev Food Sci Nutr. 2022;62(28):7703–7717. PMID 33939558. doi: 10.1080/10408398.2021.1916736
- Dobrinčić A, Balbino S, Zorić Z, et al. Advanced technologies for the extraction of marine brown algal polysaccharides. Mar Drugs. 2020;18(3):168. PMID 32197494. doi: 10.3390/md18030168
- Krishnamoorthy Y, Rajaa S, Murali S, et al. Association between anthropometric risk factors and metabolic syndrome among adults in India: A systematic review and meta-analysis of observational studies. Prev Chronic Dis. 2022;19:E24. PMID 35512304. doi: 10.5888/pcd19.210231
- Lee MK, Han K, Kim MK, et al. Changes in metabolic syndrome and its components and the risk of type 2 diabetes: a nationwide cohort study. Sci Rep. 2020;10(1):2313. PMID 32047219. doi: 10.1038/s41598-020-59203-z
- Kawser Hossain M, Abdal Dayem A, Han J, et al. Molecular mechanisms of the anti-obesity and anti-diabetic properties of flavonoids. Int J Mol Sci. 2016;17(4):569. PMID 27092490. doi: 10.3390/ijms17040569
- Lan Y, Zeng X, Guo Z, et al. Polyguluronate sulfate and its oligosaccharides but not heparin promotes FGF19/FGFR1c signaling. J Ocean Univ China. 2017;16(3):532–536. doi: 10.1007/s11802-017-3195-x
- Feuerstein GZ, Ruffolo RR, Coughlin C, et al. Inflammation. In: Fink G, editor. Encyclopedia of stress. 2nd. New York: Academic Press; 2007. pp. 530–535. doi: 10.1016/B978-012373947-6/00530-4
- Zhao Y, Feng Y, Liu M, et al. Single-cell RNA sequencing analysis reveals alginate oligosaccharides preventing chemotherapy-induced mucositis. Mucosal Immunol. 2020;13(3):437–448. PMID 31900405. doi: 10.1038/s41385-019-0248-z
- Xiong B, Liu M, Zhang C, et al. Alginate oligosaccharides enhance small intestine cell integrity and migration ability. Life Sci. 2020;258:118085. PMID 32663578. doi: 10.1016/j.lfs.2020.118085
- Rodríguez-Guzmán R, Miranda EMC, Guzmán-Díaz P. Endothelial dysfunction and cardiovascular diseases through oxidative stress pathways. In: Chatterjee S, editor. Endothelial signaling in vascular dysfunction and disease. Academic Press; 2021. pp. 213–219. doi: 10.1016/B978-0-12-816196-8.00012-6
- Seca AML, Pinto DCGA. Overview on the antihypertensive and anti-obesity effects of secondary metabolites from seaweeds. Mar Drugs. 2018;16(7):237. PMID 30011911. doi: 10.3390/md16070237
- Bolling CF, Daniels SR. Obesity. In: Haith M Benson J, editors Encyclopedia of infant and early childhood development. San Diego: Academic Press; 2008. pp. 461–468.
- Mobbs CV. Obesity. In: Aminoff M Daroff R, editors. Encyclopedia of the neurological sciences. 2nd ed. Oxford: Academic Press; 2014. pp. 621–622. doi: 10.1016/B978-0-12-385157-4.01212-4
- Sanders MH, Givelber R. Obesity. In: Laurent G Shapiro S, editors. Encyclopedia of respiratory medicine. Oxford: Academic Press; 2006. pp. 181–185. doi: 10.1016/B0-12-370879-6/00268-4
- Uchegbu EC, Kopelman PG. Obesity | treatment. In: Caballero B, editor. Encyclopedia of human nutrition. 2nd ed. Oxford: Elsevier; 2005. pp. 421–431. doi: 10.1016/B0-12-226694-3/00237-4
- Cheung BM, Cheung TT, Samaranayake NR. Safety of antiobesity drugs. Ther Adv Drug Saf. 2013;4(4):171–181. PMID 25114779. doi: 10.1177/2042098613489721
- Kang JG, Park CY. Anti-obesity drugs: a review about their effects and safety. Diabetes Metab J. 2012;36(1):13–25. PMID 22363917. doi: 10.4093/dmj.2012.36.1.13
- Tak YJ, Lee SY. Long-term efficacy and safety of anti-obesity treatment: where do we stand? Curr Obes Rep. 2021;10(1):14–30. PMID 33410104. doi: 10.1007/s13679-020-00422-w
- Kim MJ, Jeon J, Lee JS. Fucoidan prevents high-fat diet-induced obesity in animals by suppression of fat accumulation. Phytother Res. 2014;28(1):137–143. PMID 23580241. doi: 10.1002/ptr.4965
- Li S, Wang L, Liu B, et al. Unsaturated alginate oligosaccharides attenuated obesity-related metabolic abnormalities by modulating gut microbiota in high-fat-diet mice. Food Funct. 2020;11(5):4773–4784. PMID 32420551. doi: 10.1039/C9FO02857A
- Kong S, Huang X, Cao H, et al. Anti-obesity effects of galacto-oligosaccharides in obese rats. Eur J Pharmacol. 2022;917:174728. PMID 34965390. doi: 10.1016/j.ejphar.2021.174728
- Robert L, Molinari J, Ravelojaona V, et al. Age- and passage-dependent upregulation of fibroblast elastase-type endopeptidase activity. Role of advanced glycation endproducts, inhibition by fucose- and rhamnose-rich oligosaccharides. Arch Gerontol Geriatr. 2010;50(3):327–331. PMID 19560218. doi: 10.1016/j.archger.2009.05.006
- Zhao J, Han Y, Wang Z, et al. Alginate oligosaccharide protects endothelial cells against oxidative stress injury via integrin-α/FAK/PI3K signaling. Biotechnol Lett. 2020;42(12):2749–2758. PMID 32986180. doi: 10.1007/s10529-020-03010-z
- Kim KH, Kim YW, Kim HB, et al. Anti-apoptotic activity of laminarin polysaccharides and their enzymatically hydrolyzed oligosaccharides from Laminaria japonica. Biotechnol Lett. 2006;28(6):439–446. PMID 16614911. doi: 10.1007/s10529-005-6177-9
- Yamamoto Y, Kurachi M, Yamaguchi K, et al. Stimulation of multiple cytokine production in mice by alginate oligosaccharides following intraperitoneal administration. Carbohydr Res. 2007;342(8):1133–1137. PMID 17336950. doi: 10.1016/j.carres.2007.02.015
- Xu X, Wu X, Wang Q, et al. Immunomodulatory effects of alginate oligosaccharides on murine macrophage RAW264.7 cells and their structure-activity relationships. J Agric Food Chem. 2014;62(14):3168–3176. PMID 24628671. doi: 10.1021/jf405633n
- Xu X, Bi D, Wu X, et al. Unsaturated guluronate oligosaccharide enhances the antibacterial activities of macrophages. FASEB J. 2014;28(6):2645–2654. PMID 24599964. doi: 10.1096/fj.13-247791
- Fang W, Bi D, Zheng R, et al. Identification and activation of TLR4-mediated signalling pathways by alginate-derived guluronate oligosaccharide in RAW264.7 macrophages. Sci Rep. 2017;7(1):1663. PMID 28490734. doi: 10.1038/s41598-017-01868-0
- Miao Y, Sun T, Sun G. Application of alginate oligosaccharides and sodium alginate in breast abscess incision and drainage. IOP Conf S Mater Sci Eng. 2019;562(1):012135. doi: 10.1088/1757-899X/562/1/012135
- Pritchard MF, Jack AA, Powell LC, et al. Alginate oligosaccharides modify hyphal infiltration of Candida albicans in an in vitro model of invasive human candidosis. J Appl Microbiol. 2017;123(3):625–636. PMID 28635170. doi: 10.1111/jam.13516
- Chen LM, Liu PY, Chen YA, et al. Oligo-fucoidan prevents IL-6 and CCL2 production and cooperates with p53 to suppress ATM signaling and tumor progression. Sci Rep. 2017;7(1):11864. PMID 28928376. doi: 10.1038/s41598-017-12111-1
- Szekalska M, Wróblewska M, Trofimiuk M, et al. Alginate oligosaccharides affect mechanical properties and antifungal activity of alginate buccal films with posaconazole. Mar Drugs. 2019;17(12): PMID 31835313. doi: 10.3390/md17120692
- Li S, He N, Wang L. Efficiently anti-obesity effects of unsaturated alginate oligosaccharides (UAOS) in high-fat diet (HFD)-fed mice. Mar Drugs. 2019;17(9):540. PMID 31533255. doi: 10.3390/md17090540
- Bi D, Lai Q, Han Q, et al. Seleno-polymannuronate attenuates neuroinflammation by suppressing microglial and astrocytic activation. J Funct Foods. 2018;51:113–120. doi: 10.1016/j.jff.2018.10.010
- Guo JJ, Ma LL, Shi HT, et al. Alginate oligosaccharide prevents acute doxorubicin cardiotoxicity by suppressing oxidative stress and endoplasmic reticulum-mediated apoptosis. Mar Drugs. 2016;14(12):231. PMID 27999379. doi: 10.3390/md14120231
- Yamasaki Y, Yokose T, Nishikawa T, et al. Effects of alginate oligosaccharide mixtures on the growth and fatty acid composition of the green alga Chlamydomonas reinhardtii. J Biosci Bioeng. 2012;113(1):112–116. PMID 22018736. doi: 10.1016/j.jbiosc.2011.09.009
- Wan J, Zhang J, Chen D, et al. Alginate oligosaccharide-induced intestinal morphology, barrier function and epithelium apoptosis modifications have beneficial effects on the growth performance of weaned pigs. J Anim Sci Biotechnol. 2018;9(1):58. PMID 30128148. doi: 10.1186/s40104-018-0273-x
- Roberts JL, Khan S, Emanuel C, et al. An in vitro study of alginate oligomer therapies on oral biofilms. J Dent. 2013;41(10):892–899. PMID 23907083. doi: 10.1016/j.jdent.2013.07.011
- Park R-M, Ahn J-Y, Kim SY, et al. Effect of alginate oligosaccharides on collagen expression in HS 27 human dermal fibroblasts. Toxicol Environ Health Sci. 2019;11(4):327–334. doi: 10.1007/s13530-019-0421-5
- Park E-J, Choi J-I. Melanogenesis inhibitory effect of low molecular weight fucoidan from Undaria pinnatifida. J Appl Phycol. 2017;29(5):2213–2217. doi: 10.1007/s10811-016-1048-4
- Lee S. Chapter 4. Strategic design of delivery systems for nutraceuticals. In: Oprea A Grumezescu A, editors. Nanotechnology applications in food. Academic Press; 2017. pp. 65–86. doi: 10.1016/B978-0-12-811942-6.00004-2
- Rincón-León F. Functional foods. In: Caballero B, editor. Encyclopedia of food science and nutrition. 2nd ed. Oxford: Academic Press; 2003. pp. 2827–2832.
- Lin F, Yang D, Huang Y, et al. The potential of neoagaro-oligosaccharides as a treatment of type II diabetes in mice. Mar Drugs. 2019;17(10):541. PMID 31547097. doi: 10.3390/md17100541
- Charoensiddhi S, Conlon MA, Franco CMM, et al. The development of seaweed-derived bioactive compounds for use as prebiotics and nutraceuticals using enzyme technologies. Trends Food Sci Technol. 2017;70:20–33. doi: 10.1016/j.tifs.2017.10.002
- Jutur PP, Nesamma AA, Shaikh KM. Algae-derived marine oligosaccharides and their biological applications. Front Mar Sci. 2016;3:83. doi: 10.3389/fmars.2016.00083
- Hu J, Lin S, Zheng B, et al. Short-chain fatty acids in control of energy metabolism. Crit Rev Food Sci Nutr. 2018;58(8):1243–1249. PMID 27786539. doi: 10.1080/10408398.2016.1245650
- Paxman JR, Richardson JC, Dettmar PW, et al. Daily ingestion of alginate reduces energy intake in free-living subjects. Appetite. 2008;51(3):713–719. PMID 18655817. doi: 10.1016/j.appet.2008.06.013
- Yang J, Shen Z, Sun Z, et al. Growth stimulation activity of alginate-derived oligosaccharides with different molecular weights and mannuronate/guluronate ratio on Hordeum vulgare L. J Plant Growth Regul. 2021;40(1):91–100. doi: 10.1007/s00344-020-10078-4
- González A, Castro J, Vera J, et al. Seaweed oligosaccharides stimulate plant growth by enhancing carbon and nitrogen assimilation, basal metabolism, and cell division. J Plant Growth Regul. 2013;32(2):443–448. doi: 10.1007/s00344-012-9309-1
- Wang Z, Li J, Liu J, et al. Management of blue mold (Penicillium italicum) on mandarin fruit with a combination of the yeast, Meyerozyma guilliermondii and an alginate oligosaccharide. Biol Control. 2021;152:104451. doi: 10.1016/j.biocontrol.2020.104451
- Jiang H, Liang S, Yao XR, et al. Laminarin improves developmental competence of porcine early stage embryos by inhibiting oxidative stress. Theriogenology. 2018;115:38–44. PMID 29705658. doi: 10.1016/j.theriogenology.2018.04.019
- Lomartire S, Gonçalves AMM. An overview of potential seaweed-derived bioactive compounds for pharmaceutical applications. Mar Drugs. 2022;20(2):141. PMID 35200670. doi: 10.3390/md20020141
- Manigandan V, Karthik R, Ramachandran S, et al. Chapter 15. Chitosan applications in food industry. In: Grumezescu A Holban A, editors. Biopolymers for food design. Academic Press; 2018. pp. 469–491. doi: 10.1016/B978-0-12-811449-0.00015-3
- Chen J, Hu Y, Zhang L, et al. Alginate oligosaccharide DP5 exhibits antitumor effects in osteosarcoma patients following surgery. Front Pharmacol. 2017;8:623. PMID 28955228. doi: 10.3389/fphar.2017.00623
- Lu J, Shi KK, Chen S, et al. Fucoidan extracted from the New Zealand Undaria pinnatifida—physicochemical comparison against five other fucoidans: unique low molecular weight fraction bioactivity in breast cancer cell lines. Mar Drugs. 2018;16(12):461. PMID 30469516. doi: 10.3390/md16120461
- Rodrigues F, Cádiz-Gurrea MDL, Nunes L. Cosmetics. In: Galanakis C, editor. Polyphenols: Properties, recovery, and applications. Pinto: MA, D. Vinha. Linares: AF, I. B., … Carretero, A. S. Woodhead Publishing. 12 - Cosmetics; 2018. pp. 393–427.
- Chen Q, Kou L, Wang F, et al. Size-dependent whitening activity of enzyme-degraded fucoidan from Laminaria japonica. Carbohydr Polym. 2019;225:115211. PMID 31521267. doi: 10.1016/j.carbpol.2019.115211
- Gouda M, Tadda MA, Zhao Y, et al. Microalgae bioactive carbohydrates as a novel sustainable and eco-friendly source of prebiotics: Emerging health functionality and recent technologies for extraction and detection. Front Nutr. 2022;9:806692. doi: 10.3389/fnut.2022.806692
- Sim SJ, Joun J, Hong ME, et al. Split mixotrophy: A novel cultivation strategy to enhance the mixotrophic biomass and lipid yield of Chlorella protothecoides. Bioresour Technol. 2019;291:121820. PMID 31344639. doi: 10.1016/j.biortech.2019.121820
- Patel AK, Joun JM, Hong ME, et al. Effect of light conditions on mixotrophic cultivation of green microalgae. Bioresour Technol. 2019;282:245–253. PMID 30870690. doi: 10.1016/j.biortech.2019.03.024
- Patel AK, Singhania RR, Chen CW, et al. Advances in micro-and Nano bubbles technology for application in biochemical processes. Environ Technol Innov. 2021d;23:101729. doi: 10.1016/j.eti.2021.101729
- Patel AK, Kumar P, Chen CW, et al. Nano magnetite assisted flocculation for efficient harvesting of lutein and lipid producing microalgae biomass. Bioresour Technol. 2022;363:128009. PMID 36162780. doi: 10.1016/j.biortech.2022.128009
- Patel AK, Singhania RR, Sim SJ, et al. Recent advancements in mixotrophic bioprocessing for production of high value microalgal products. Bioresour Technol. 2021;320(B):124421. PMID 33246239. doi: 10.1016/j.biortech.2020.124421
- Patel AK, Singhania RR, Dong CD, et al. Mixotrophic biorefinery: A promising algal platform for sustainable biofuels and high value coproducts. Renew Sustain Energ Rev. 2021e;152:111669. doi: 10.1016/j.rser.2021.111669
- Patel AK, Albarico FPJB, Perumal PK, et al. Algae as an emerging source of bioactive pigments. Bioresour Technol. 2022;351:126910. PMID 35231601. doi: 10.1016/j.biortech.2022.126910
- Patel AK, Vadrale AP, Tseng YS, et al. Bioprospecting of marine microalgae from Kaohsiung seacoast for lutein and lipid production. Bioresour Technol. 2022;351:126928. PMID 35257880. doi: 10.1016/j.biortech.2022.126928
- Patel AK, Chauhan AS, Kumar P, et al. Emerging prospects of microbial production of omega fatty acids: recent updates. Bioresour Technol. 2022;360:127534. PMID 35777644. doi: 10.1016/j.biortech.2022.127534
- Patel AK, Tambat VS, Chen CW, et al. Recent advancements in astaxanthin production from microalgae: a review. Bioresour Technol. 2022;364:128030. PMID 36174899. doi: 10.1016/j.biortech.2022.128030
- Patel AK, Katiyar R, Chen CW, et al. Antibiotic bioremediation by new generation biochar: recent updates. Bioresour Technol. 2022;358:127384. PMID 35644454. doi: 10.1016/j.biortech.2022.127384
- Patel AK, Choi YY, Sim SJ. Emerging prospects of mixotrophic microalgae: way forward to bioprocess sustainability, environmental remediation and cost-effective biofuels. Bioresour Technol. 2020a;300:122741. PMID 31956058. doi: 10.1016/j.biortech.2020.122741
- Patel AK, John J, Sim SJ. A sustainable mixotrophic microalgae cultivation from dairy wastes for carbon credit, bioremediation and lucrative biofuels. Bioresour Technol. 2020b;313:123681. PMID 32562971. doi: 10.1016/j.biortech.2020.123681
- Patel AK, Tseng YS, Rani Singhania R, et al. Novel application of microalgae platform for biodesalination process: a review. Bioresour Technol. 2021;337:125343. PMID 34120057. doi: 10.1016/j.biortech.2021.125343
- Patel AK, Singhania RR, Pal A, et al. Advances on tailored biochars for bioremediation of antibiotics, pesticides and polycyclic aromatic hydrocarbon pollutants from aqueous and solid phases. Sci Total Environ. 2022b;817:153054. PMID 35026237. doi: 10.1016/j.scitotenv.2022.153054
- Patel AK, Singhania RR, Albarico FPJB, et al. Organic wastes bioremediation and its changing prospects. Science Of The Total Environment. 2022c;824:153889. PMID 35181362. doi: 10.1016/j.scitotenv.2022.153889
- Bixler HJ, Porse H. A decade of change in the seaweed hydrocolloids industry. J Appl Phycol. 2011;23(3):321–335. doi: 10.1007/s10811-010-9529-3
- Alboofetileh M, Rezaei M, Tabarsa M, et al. Ultrasound-assisted extraction of sulfated polysaccharide from Nizamuddinia zanardinii: Process optimization, structural characterization, and biological properties. J Food Process Eng. 2019;42(2):e12979. doi: 10.1111/jfpe.12979