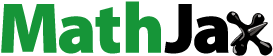
Abstract
Photochromic materials have attracted considerable interest because of their ability to undergo reversible color switching. We report highly conductive and flexible spiropyran-embedded cellulose films with multiple optical and electrical functionalities, showing negative photo- and thermochromism. Combining silver nanowires and conductive polymer with cellulose/spiropyran films achieves a very low sheet resistance of 6.7 ohm/sq. The optimized films show outstanding mechanical stability with negligible electrical loss (resistance change < 2%) after 1000 bending cycles. The photo- and thermal-responsive conductive cellulose/spiropyran films can be used to directly visualize information and as flexible heating devices, pressure-responsive sensors, and colorimetric thermometers with high device performance.
GRAPHICAL ABSTRACT
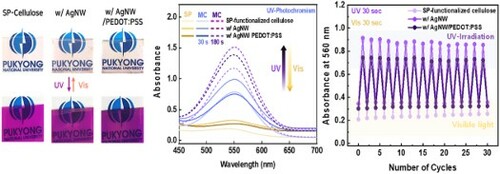
IMPACT STATEMENT
We demonstrate highly conductive, flexible, transparent, and reversibly color-switchable films based on a spiropyran-incorporated cellulose acetate biopolymer. The films can realize high performance photoelectronics that include reversibly tunable color switching.
1. Introduction
In recent decades, photochromic materials have attracted significant attention owing to their potential applications in smart colorimetric electronics [Citation1,Citation2]. Various photochromic materials, including spiropyran (SP), azobenzenes, stilbenes, stilbenes, and dithienylethenes, exhibit photoswitching effects that are responsive to external stimuli [Citation2–4]. In particular, SP has been extensively investigated because of its wide range of responsivity to light, temperature, redox changes, metal ions, pH, and mechanical force [Citation5]. The high responsiveness of SP to external stimuli results in efficient molecular conversion from the closed-ring SP isomer to the open-ring merocyanine (MC) isomer. The MC isomer strongly absorbs light at its maximum absorption wavelength of approximately 560 nm and exhibits an intense purple color, while the SP isomer exhibits a colorless behavior. Photochromic conversion due to molecular switching changes the absorption spectra, geometric structures, redox potentials, and dielectric constants of molecules [Citation2]. In addition to photoisomerization, SP-based dynamic materials can also be isomerized at elevated temperatures, at which molecular switching causes the conversion from colorless SP to colored MC.
SP-functionalized polymers have great potential for applications in electronic devices such as chemo/biosensors, optical memory, smart devices, and photoswitching devices [Citation6–8]. Nam et al. demonstrated the gas sensors based on the photochromic SP-embedded PDMS composite [Citation9]. Photoswitchable DNA-binding characteristics of SPs were reported by Andersson [Citation10]. Frolova et al. reported the non-volatile optical memory elements with photochromic SP-based salts [Citation11]. SPs have been incorporated into various polymers, including polystyrene, poly(methyl methacrylate), polyurethanes, polyacrylates, and poly(dimethylsiloxane) [Citation1,Citation12–14]. The SP moiety can serve a variety of functions in polymer systems and take on roles such as being an initiator, monomer, or cross-linker. Among various organic systems incorporating the SP moiety, cellulose acetate, which is produced from diverse natural sources such as wood, agricultural waste, and renewable resources, has unique merits in terms of its biodegradability, human and environmental friendliness, and high biocompatibility, and is regarded as an ideal substitute for plastics [Citation15,Citation16].
These properties of cellulose acetate-based biopolymers enable the production of low-cost, optically transparent, and biocompatible substrates for next-generation flexible electronics. To realize the diverse functionalities of cellulose-based materials, researchers have focused on various approaches to enhance the structural, mechanical, and electrochemical properties of cellulose systems [Citation15,Citation17–19]. However, the functionalization of cellulose-based materials for photochemical applications such as colorimetric sensors has not been widely investigated [Citation20,Citation21]. Khattab et al. reported organic-inorganic nanocomposite photoluminescent cellulose acetate with a lanthanide-doped pigment [Citation22]. Shi et al. demonstrated the thermochromic luminescent fiber based on cellulose acetate, rare earth luminescent materials, and thermochromic pigments [Citation23]. The cellulose acetate-nanostructured membrane with thermos- and photo-sensitive chromic pigments was reported by Mancipe et al [Citation24]. To the best of our knowledge, the combination of flexible and conductive cellulose-based functional materials with negative photo- and thermochromic SP has not been reported.
Here, we demonstrate highly conductive, flexible, and transparent SP-embedded cellulose acetate films that exhibit efficient negative photo- and thermochromic effects which facilitate the visualization of photopatterns. The cellulose/SP films are combined with silver nanowires (AgNWs) and conductive poly(3,4-ethylenedioxythiophene):poly(styrenesulfonate) (PEDOT:PSS) polymer, which provide high electrical conductivity. Overcoating the conductive cellulose/SP films with PEDOT:PSS significantly reduces the resistance of the films and improves their mechanical durability under cyclic bending, physical adhesion, and chemical degradation. A limited resistance change (< 2%) under 1000 bending cycles is achieved by introducing conductive PEDOT:PSS overcoat layer by preventing the disconnection of AgNWs. The reversibly switchable flexible conductive cellulose/SP films are capable of information visualization in the form of arbitrary patterns. The flexible transparent heating device based on the film allows for heat generation up to 70.8 °C. As colorimetric thermometers, the film can directly visualize uniform thermal information when applied on a high temperature object. The pressure-responsive sensor based on the AgNW/PEDOT:PSS-based cellulose film exhibits fast response time and high sensitivity over multiple cycles. Our approach paves the way for the realization of functional photo- and thermal-responsive electronic devices through the use of the flexible conductive cellulose/SP films.
2. Experimental section
2.1. Preparation of cellulose/SP films
To prepare the cellulose solution, 0.8 g of cellulose acetate (average Mn of approximately 30,000, Sigma Aldrich) was dissolved in a mixed solution of dichloromethane (9 ml, Sigma Aldrich) and ethanol (1 ml). The cellulose solution was stirred on a hot plate at room temperature at the spin speed of 1000 rpm for 10 min. Subsequently, 10 mg of 1, 3-dihydro-1, 3 ′, 3-trimethyl-6-nitrospiro[2H-1-benzopyran-2,2-(2H)-indole] (SP, Sigma Aldrich) was dissolved into the cellulose solution and stirred until its color changed to purple completely. The polarity of materials and stirring process may induce the color change to purple due to chemical- and mechano-responsiveness of SP with negative photochromism. The solution was irradiated with visible light until completely colorless (30 s) and poured onto a petri dish and cured for 16 h at room temperature. The thicknesses of cellulose/SP films measured by a surface profilometer (Dektak XT, Bruker) were about 240–250 µm.
2.2. Fabrication of conductive cellulose/SP films
To improve the bonding strength between the cellulose/SP substrates and silver nanowires (AgNWs, Flexio Co., Ltd., approximately 25 nm in diameter and approximately 25 µm in length) dispersed in isopropanol, the substrate was pre-treated with oxygen plasma for 15 min. Ethanolamine was subsequently spin-coated onto the substrate as a surface modifier. The AgNW solution was spin-coated at 1500 rpm three times and then annealed at 100 °C for 10 min. 10 ml of poly(3,4-ethylenedioxythiophene):poly(styrene sulfonate) (PEDOT:PSS, Clevios PH1000, Heraeus) was homogenized by tip sonication for 8 min. 0.6 ml of ethylene glycol was mixed into the PEDOT:PSS solution as a conductivity enhancer together with 10 µL of fluorosurfactant (Capstone FS-31). The PEDOT:PSS solution was spun onto the AgNW-coated cellulose/SP at 3000 rpm and annealed at 120 °C. Hardness values for the non-conductive, AgNW-based, and AgNW/PEDOT:PSS-based films were 0.40, 0.54, and 0.45 GPa, respectively.
2.3. Characterization
The UV light illumination was performed using a 395 nm-UV source (0.5 W) with an irradiation distance of 5 cm. The visible light irradiation was carried out using PEC-L01 portable solar simulator. Hardness was measured by a nanoindenter (TI-950, Bruker) with a contact depth of ∼ 33 nm. The transmittance and absorbance were measured using a UV-vis spectrophotometer (UV-2600, Shimadzu). The Commission Internationale de L’éclairage (CIE) coordinates were measured by a spectroradiometer (HS-1000, Otsuka Electronics). The sheet resistance was measured by a source-meter instrument (Keithley 2401) using the van der Pauw method. DC voltages were applied to the conductive films to induce the Joule heating effect, and the resulting thermal images were recorded using an infrared camera (TiS45, Fluke). The electric current response of the pressure sensing films was measured using the Keithley 2401 sourcemeter at the DC voltages of 0.1, 0.5, 1.0, and 3.0 V.
3. Results and discussion
Figure (a) shows the molecular structures of spiropyran (SP) that enables reversible photo and thermal switching. To utilize its photochromic and thermochromic properties, SP was incorporated into a cellulose substrate. The pure cellulose film used in our study is highly transparent and has an average transmittance of 90.3% at visible wavelengths (400–800 nm), as shown in Figure (b). Furthermore, AgNW networks and PEDOT:PSS overcoat layers were combined with the cellulose/SP films to realize multifunctional composite films that are highly conductive and photo/thermal-switchable (Figure (c)). The application of ultraviolet (UV) illumination or elevated temperatures initiates a [cis-trans] conversion of the SP from the SP state (transparent light pink) to the merocyanine (MC) state (transparent dark purple). The photo- and thermochromism can be divided into normal and negative chromism depending on the level of the environment polarity. In normal chromism, UV irradiation or mechanical activation cleave the C-O bond of SP that results in the ring-opening of SP. Our SP incorporating cellulose acetate films reveal negative chromism properties owing to high polar surroundings. In this case, the elevated temperature and dark condition induce the isomerization of the SP form to the colored MC form [Citation13,Citation20,Citation25,Citation26]. In addition, the reverse isomerization from the MC form to the SP form occurs by irradiation of visible light or cooling temperature. Since the negative photochromism can have a negative impact on anti-counterfeiting applications of photochromic materials, several studies to reduce the negative photochromism by introducing hydrophobic polymers have been conducted [Citation13,Citation27–29].
Figure 1. (a) Digram of reversible photo- and thermochromism switching of SP-incorporated functional films. (b) Transmittance spectra of the cellulose and glass substrates, and a photograph of the cellulose film. (c) Schematic of the flexible conductive cellulose/SP film.
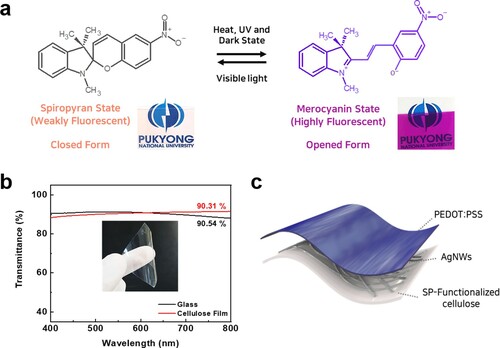
Photopatterned on-demand UV-induced photo-switching cellulose/SP films were defined by shadow masks with the designed pattern. Various patterns such as letters, characters, and arbitrary patterns can be prepared. The regions illuminated by UV light were clearly visualized by their color change, which is attributed to localized photoisomerization (Figure (a)). Figure (b-c) shows the reversible colorimetric transition of non-conductive and conductive cellulose/SP films upon photoirradiation. When exposed to UV light, the molecular conformation of the SP in the films switches from the colorless SP state to the colored MC state. The color of the films becomes darker with increasing exposure time, as can be seen from Figure S1. In the reverse process, the films return to their original colorless state upon exposure to visible light. The light absorbance of the films at the wavelengths of 450–650 nm can be reversely changed by UV or visible light irradiation because the SP-based materials transform their molecular structures after absorbing light of a particular wavelength (Figure (c)). The color conversion process leads to changes in the color coordinates of the films (Figure S1 in the Supplemental Material).
Figure 2. (a) On-demand UV-induced photopatterning of cellulose/SP films with various designed patterns. (b) Photographs, (c) absorbance spectra, and (d) absorbance changes for non-conductive, AgNW-based, and AgNW/PEDOT:PSS-based films, showing reversible photo switching. (e) Photographs, (f) absorbance spectra, and (g) absorbance changes for non-conductive, AgNW-based, and AgNW/PEDOT:PSS-based films, showing reversible thermal switching.
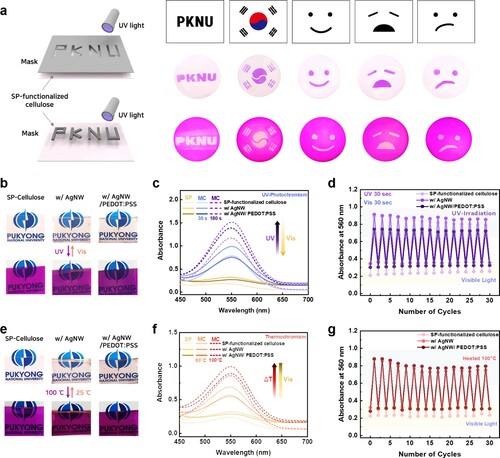
Reversible color changes in the films are also induced by heat (Figure (e,f)). The MC state possesses the more thermodynamically stable isomer in negative chromism, in which thermal treatment isomerizes the cisoid SP state to the transoid MC state. The negative thermochromism occurs when the temperature is elevated to near 40 °C, and dark purple films are obtained when the films are heated up to 100 °C (for 2 min). The heated films are decolorized (SP state) when they are exposed to visible light (for 1 min) or cooled to room temperature. The changes in the color coordinates of the cellulose/SP films with thermochromism are shown in Figure S2 in the Supplemental Material. In addition to light and temperature, mechanical forces such as pressure or strain also can induce the reversible isomerization of SP and color changes, which is the subject of further study [Citation14,Citation30,Citation31].
We performed cyclic photo- and thermochromism switching to investigate the long-term stability of the films against repetitive light and temperature stimuli (Figure (d,g), respectively). The cyclic SP-MC transitions resulting from repeated illumination of UV and white light (for 30 s each) were identified by the distinct changes in the light absorbance at the wavelength of 560 nm. A reduction in the absorbance difference between the closed SP form and opened MC form was exhibited in the cyclic photochromism behavior. The change in the relative absorbance is given by the following equation:
where η denotes the relative absorbance and A is the absorbance of the films. The absorbance changes in the optical molecular switching over 30 cycles for the non-conductive, AgNW-based, and AgNW/PEDOT:PSS-based films were approximately 20.5%, 12.7%, and 9.8%, respectively. The optical change was limited in the PEDOT:PSS-functionalized film. This demonstrates the enhancement of the film photoreversibility by the PEDOT:PSS overcoat, which keeps the state conversion constant. The rate of color conversion for photochromism is shown in Figures S3 and S4 in the Supplemental Material.
The thermochromism switching induced by heating at 100 °C (for 2 min) and illumination of visible light (for 1 min) exhibited similar behavior to the cyclic photochromism switching (Figure (f)). After 30 thermal switching cycles, the changes in the absorbance of the films were approximately 20.4%, 20.1%, and 18.6% in the non-conductive, AgNW-based, and AgNW/PEDOT:PSS-based films, respectively. These results suggest that the introduction of PEDOT:PSS enhances the switching stability of the films against repeated thermal switching cycles. The rate of color changes for thermochromism is shown in Figures S5-S10 in the Supplemental Material.
In addition to the ability to exhibit reversible photo- and thermochromism, our conductive cellulose films can be applied as transparent electrodes because of the combination of high-conductivity materials, which include conductive polymers and AgNW networks, with the films. The AgNW-based and AgNW/PEDOT:PSS-based films exhibited the low sheet resistance values of 10.3 and 6.7 ohm/sq, respectively. We performed cyclic bending tests to investigate the mechanical stability of the films (Figure (a)). Under 1000 bending cycles at the bending radii of 9.0, 7.5, and 6.0 mm, both films showed limited resistance changes, indicating that the introduction of the surface modifier and oxygen-plasma treatment caused strong interfacial bonding that effectively coupled the cellulose film and the conductive layer. The AgNW/PEDOT:PSS-based film exhibited superior stability and durability compared to the AgNW-based film. The AgNW/PEDOT:PSS-based cellulose film exhibited a 1.02-fold increase in resistance (6.87 ohm/sq) after 1000 bending cycles at the bending radius of 6.0 mm. In comparison, the resistance of the AgNW-based film increased by 1.06-fold (10.98 ohm/sq) under the same conditions. In addition, the AgNW/PEDOT:PSS-based film exhibited outstanding mechanical robustness with insignificant conductivity losses in the tape attach-detach test (Figure (b)). The enhanced mechanical properties of the PEDOT:PSS-overcoated film demonstrate that the PEDOT:PSS layer effectively suppresses the disconnection of the nanowires and improves the adhesion properties under mechanical deformation. To investigate the chemical stability of the films, the films were dipped in ethanol and toluene (Figure (c,d)). The resistance of the AgNW/PEDOT:PSS-based film increased by 1.05- and 1.15-folds after the film was dipped in ethanol and toluene for 1 h, respectively. The film therefore exhibited outstanding chemical stability. In comparison, the AgNW-based film without PEDOT:PSS overcoating exhibited a greater increase in resistance. The results indicate that the PEDOT:PSS layer has an excellent ability to reduce the electrical loss by preventing the penetration of chemicals.
Figure 3. (a) Relative resistance changes of the AgNW-based and AgNW/PEDOT:PSS-based films as a function of the number of bending cycles at different bending radii. (b) Relative resistance changes of the films with respect to the number of tape attach-detach tests. Relative resistance changes of the films dipped in (c) ethanol and (d) toluene.
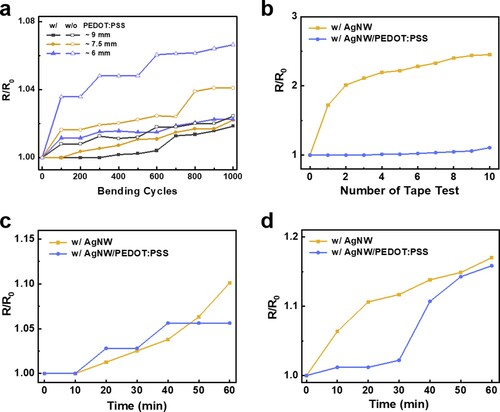
As an example of a practical application, we designed flexible transparent heating devices utilizing the conductive cellulose films. The operation of the devices is based on Joule heating at a DC voltage of 3 V (1 A current). The temperature increase of the heating devices upon voltage was accompanied by a coloration change due to thermochromic switching (Figure (a,b)). A temperature of up to 70.8 °C was achieved in the AgNW/PEDOT:PSS-based film. In comparison, the temperature reached up to only 55.6 °C without the PEDOT:PSS overcoating layer. These results suggest that the PEDOT:PSS overcoating layer enhances the conductivity as well as the uniformity of the conduction paths, which improves the heating performance of the heating devices. Figure (c,d) displays the real-time sheet resistance and temperature changes of the heating devices over three on-off cycles. Both heaters exhibited stable resistance and temperature changes following the heating and cooling processes.
Figure 4. Photographs and infrared images of the flexible heaters based on (a) AgNW and (b) AgNW/PEDOT:PSS films. (c) Sheet resistance and (d) temperature changes of the AgNW and AgNW/PEDOT:PSS-based films with repeated on/off switching as functions of time. (e) Photographs and infrared images of the cellulose/SP film applied to a cup filled with boiling water.
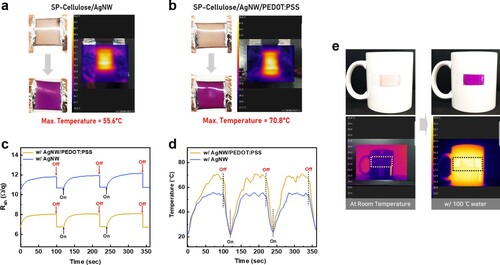
To serve as a colorimetric thermometer, the cellulose/SP film was applied to a cup filled with boiling water (Figure (e)). Applying sufficient heat to the cellulose/SP film induces a transition to the ring-opened form (MC state) and a color change to the intense purple of the MC state. The excellent colorimetric response can be used for visualizing the local heat sources in objects. The temperature distribution in this experiment was analyzed via Fourier's law of heat conduction using the finite element method (Figure S11). The temperature induced at the cellulose/SP film was approximately 76.8 °C, which corresponded to the heat transfer coefficient of 462.9 W/m °C. The thermal conductivity and temperature gradient on the solid wall resulted in a temperature drop to approximately 23 °C. The thermal stabilities of the conductive and non-conductive cellulose/SP films were investigated by thermogravimetric analysis (Figure S12).
The pressure-sensing capabilities of the conductive cellulose/SP films are shown in Figure . The pressure sensors were composed of two parallel conductive cellulose/SP films with an air gap in between. The sensors were operated at various voltages and exhibited outstanding pressure-responsive properties as their surfaces were repeatedly touched, as well as stable recoverability, high sensitivity, and rapid signal response over multiple cycles. The higher conductivity of the AgNW/PEDOT:PSS-based film resulted in larger current signals from the sensor constructed using the film compared to the sensor constructed from the film without the PEDOT:PSS overcoating layer.
4. Conclusions
In summary, we presented highly conductive, flexible, transparent, and reversibly color-switchable films based on a SP-incorporated cellulose acetate biopolymer with negative photo- and thermalchromism. The multiple optical and electrical functionalities exhibited by the cellulose/SP films include excellent reversible color switching when photo and thermal energies are applied. Photopatterns can be directly visualized using the films. High conductivity was realized in the cellulose/SP film by employing AgNW networks and conductive PEDOT:PSS polymers. The conductive color-switchable films exhibited low sheet resistances of 10.3 and 6.7 ohm/sq in the AgNW-based and AgNW/PEDOT:PSS-based structures, respectively. In addition to significantly reducing the film resistance, the functionalization by overcoating with PEDOT:PSS effectively improves the stability and durability of films in the cyclic bending, adhesion, and chemical degradation tests. The AgNW/PEDOT:PSS-based cellulose film exhibited a negligible resistance change (< 2%) after 1000 bending cycles, indicating that overcoated PEDOT:PSS capably prevents the disconnection or fracture of AgNW under mechanical deformation. The optimized conductive cellulose/SP films not only offer a direct visualization of photopatterns but also allow for realizing flexible transparent heating devices, colorimetric thermometers, and pressure sensors. The AgNW/PEDOT:PSS-based film achieves a high temperature of up to 70.8 °C when applied as a transparent heater. The dynamic colorimetric thermometer based on the film showed outstanding and uniform thermochromic performances. Furthermore, high sensitivity and rapid signal response over multiple cycles were observed for the AgNW/PEDOT:PSS-based pressure sensor. These highly robust and flexible conductive cellulose/SP films are poised to play significant roles in a wide variety of photoelectronic applications that include reversibly tunable color switching as well as heating, sensing, and heat visualization.
Supplemental Material
Download MS Word (4.1 MB)Acknowledgments
The authors acknowledge financial support from the Korea Institute of Science and Technology (Grant No. 2E30470) and support from the Basic Science Research Program through the National Research Foundation of Korea (NRF) funded by the Ministry of Science, ICT & Future Planning (2021R1A2C1094308).
Disclosure statement
No potential conflict of interest was reported by the author(s).
References
- Klajn R. Spiropyran-based dynamic materials. Chem Soc Rev. 2014;43:148–184.
- Kortekaas L, Browne WR. The evolution of spiropyran: fundamentals and progress of an extraordinarily versatile photochrome. Chem Soc Rev. 2019;48:3406–3424.
- Kishimoto Y, Abe J. A Fast photochromic Molecule that colors only under UV light. J Am Chem Soc. 2009;131:4227–4229.
- Tomasulo M, Sortino S, Raymo FM. A Fast and stable photochromic switch based on the opening and closing of an oxazine ring. Org Lett. 2005;7:1109–1112.
- Lukyanov BS, Lukyanova MB. Spiropyrans: synthesis, properties, and application. (review). Chem Heterocycl Compd. 2005;41:281–311.
- Irie M, Fukaminato T, Matsuda K, et al. Photochromism of diarylethene molecules and crystals: memories, switches, and actuators. Chem Rev. 2014;114:12174–12277.
- Ali AA, Kharbash R, Kim Y. Chemo- and biosensing applications of spiropyran and its derivatives - A review. Anal Chim Acta. 2020;1110:199–223.
- Park IS, Jung Y-S, Lee K-J, et al. Photoswitching and sensor applications of a spiropyran–polythiophene conjugate. Chem Commun. 2010;46:2859–2861.
- Nam Y-S, Yoo I, Yarimaga O, et al. Photochromic spiropyran-embedded PDMS for highly sensitive and tunable optochemical gas sensing. Chem Commun. 2014;50:4251–4254.
- Andersson J, Li S, Lincoln P, et al. Photoswitched DNA-binding of a photochromic spiropyran. J Am Chem Soc. 2008;130:11836–11837.
- Frolova LA, Rezvanova AA, Lukyanov BS, et al. Design of rewritable and read-only non-volatile optical memory elements using photochromic spiropyran-based salts as light-sensitive materials. J Mater Chem C. 2015;3:11675–11680.
- Abdollahi A, Roghani-Mamaqani H, Razavi B. Stimuli-chromism of photoswitches in smart polymers: Recent advances and applications as chemosensors. Prog Polym Sci. 2019;98:101149.
- Abdollahi A, Roghani-Mamaqani H, Razavi B, et al. Photoluminescent and chromic nanomaterials for anticounterfeiting technologies: Recent advances and future challenges. ACS Nano. 2020;14:14417–14492.
- Li M, Zhang Q, Zhou Y-N, et al. Let spiropyran help polymers feel force!. Prog Polym Sci. 2018;79:26–39.
- Zhao D, Zhu Y, Cheng W, et al. Cellulose-Based flexible functional materials for emerging intelligent electronics. Adv Mater. 2020;2000619:1–18.
- Tu H, Zhu M, Duan B, et al. Recent progress in high-strength and robust regenerated cellulose materials. Adv Mater. 2021;33:2000682.
- Kamel S, Khattab TA. Recent advances in cellulose supported metal nanoparticles as Green and sustainable catalysis for organic synthesis. Cellulose. 2021;28:4545–4574.
- Ray U, Zhu S, Pang Z, et al. Mechanics design in cellulose-enabled high-performance functional materials. Adv Mater. 2021;33:2002504.
- Clarkson CM. El awad azrak SM, forti ES. et al. Recent Developments in Cellulose Nanomaterial Composites. Adv Mater. 2021;33:2000718.
- Tian W, Tian J. Synergy of different fluorescent enhancement effects on spiropyran appended onto cellulose. Langmuir. 2014;30:3223–3227.
- Shuiping L, Lianjiang T, Weili H, et al. Cellulose acetate nanofibers with photochromic property: Fabrication and characterization. Mater Lett. 2010;64:2427–2430.
- Khattab TA, El-Naggar ME, Abdelrahman MS, et al. Facile development of photochromic cellulose acetate transparent nanocomposite film immobilized with lanthanide-doped pigment: ultraviolet blocking, superhydrophobic, and antimicrobial activity. Luminescence. 2021;36:543–555.
- Shi M, Li X, Zhu Y, et al. Preparation and luminescence performance of thermochromic luminescent fiber based on reversible thermochromic red pigment. J Mater Sci Mater Electron. 2021;32:9074–9086.
- Mancipe JMA, Nista SVG, Caballero GER, et al. Thermochromic and/or photochromic properties of electrospun cellulose acetate microfibers for application as sensors in smart packing. J Appl Polym Sci. 2021;138:50039.
- Sylvia GM, Heng S, Bachhuka A, et al. A spiropyran with enhanced fluorescence: A bright, photostable and red-emitting calcium sensor. Tetrahedron. 2018;74:1240–1244.
- Shiraishi Y, Itoh M, Hirai T. Thermal isomerization of spiropyran to merocyanine in aqueous media and its application to colorimetric temperature indication. Phys Chem Chem Phys. 2010;12:13737–13745.
- Abdollahi A, Mouraki A, Sharifian MH, et al. Photochromic properties of stimuli-responsive cellulosic papers modified by spiropyran-acrylic copolymer in reusable pH-sensors. Carbohydr Polym. 2018;200:583–594.
- Abdollahi A, Mahdavian AR, Salehi-Mobarakeh H. Preparation of stimuli-responsive functionalized latex nanoparticles: The effect of spiropyran concentration on size and photochromic properties. Langmuir. 2015;31:10672–10682.
- Abdollahi A, Rad JK, Mahdavian AR. Stimuli-responsive cellulose modified by epoxy-functionalized polymer nanoparticles with photochromic and solvatochromic properties. Carbohydr Polym. 2016;150:131–138.
- Qiu W, Gurr PA, da Silva G, et al. Insights into the mechanochromism of spiropyran elastomers. Polym Chem. 2019;10:1650–1659.
- Meng X, Qi G, Li X, et al. Spiropyran-based multi-colored switching tuned by pressure and mechanical grinding. J Mater Chem C. 2016;4:7584–7588.