Abstract
The inferior creep ductility of Inconel 718 (IN718) fabricated by selective laser melting (SLM), which is unable to meet aerospace material specification (≥4%), limits its engineering application. Despite persistent optimization efforts in the SLM process and post-heat treatment, this issue remains unresolved. This study involved SLM fabrication of the modified IN718 with trace amounts of LaB6 and/or P, followed by homogenization and double aging heat treatment. The addition of trace LaB6 notably influenced the recrystallized grain structure and carbide precipitation at grain boundaries, while trace P addition had minimal impact. All samples exhibited similar γ′ and γ″ strengthening precipitates. Creep tests conducted at 650°C/690 MPa revealed that the individual modification of LaB6 showed limited improvement in creep performance and P showed no improvement, but the combined addition of LaB6 and P led to a substantial enhancement in creep performance, especially reaching as high as 8.6% creep strain. This remarkable improvement in creep ductility is unlikely to arise from the altered grain structure and grain boundary precipitates induced by LaB6 but predominantly arises from the synergistic effect of P and B in enhancing resistance to crack propagation during the third stage of creep.
1. Introduction
IN718 is a nickel-based superalloy known for its exceptional high-temperature strength, excellent corrosion resistance and oxidation resistance at temperatures below 650°C [Citation1,Citation2]. It has found widespread applications in many fields such as aviation, aerospace, nuclear energy and others [Citation3,Citation4]. Recently, many efforts have been made to investigate IN718 fabricated by SLM, a mainstream additive manufacturing technique based on laser powder bed fusion, which offers a powerful capability for constructing complex structures to release the potential of free design [Citation5]. Previous research studies have shown that IN718 has good suitability for SLM and the tensile properties of SLM IN718 are comparable to those of the forged IN718, but the creep performance of SLM IN718, especially the creep ductility, is markedly lower than that of the forging standard [Citation6–10]. This is probably ascribed to the unique microstructure of SLM IN718 distinct from the forged specimen, including the grain size inhomogeneity and continuous fine precipitates at the grain boundaries leading to severe stress/strain concentration and weak crack propagation resistance [Citation11]. The very low creep ductility of IN718 fabricated by the SLM technique seriously hinders its engineering application, considering the risk of sudden failure.
In the past several years, researchers have made efforts to enhance the creep performance of SLM IN718 by modifying SLM process parameters [Citation4,Citation10,Citation12] and post-heat treatment regimes [Citation13–15]. The outcomes of these researches bring the obvious improvement in the creep life, but the creep ductility remains very low, mostly no more than 2%, which is unable to meet the aerospace material specification for IN718 required in AMS 5662M (≥4%) [Citation16]. Previous research has shown that the grain boundary failure is responsible for the low creep ductility in SLM IN718, indicating that the rapid propagation of grain boundary cracks in the third creep stage is particularly critical [Citation17].
Microalloying of grain boundary-strengthening elements is a common way to enhance the creep properties in superalloys. Boron is a commonly used trace element in superalloys to strengthen grain boundaries by increasing atom bonding strength at grain boundaries and decreasing grain boundary energy. Furthermore, the interaction of boron with phosphorus has shown an even more remarkable improvement in creep performance in forged IN718 [Citation18–21]. Although the effect of microalloying with grain boundary strengthening elements on the performance of traditional manufacturing superalloys is well known, we are still intrigued to explore whether boron and/or phosphorus microalloying can solve the issue of poor creep ductility of SLM IN718 due to its distinct microstructure and creep behavior. Additionally, powder as the feedstock of SLM has an order of magnitude higher oxygen content than that of bulk material. Rare earth elements exhibiting a strong affinity for oxygen can purify grain boundaries by stabilizing oxygen to improve the ductility of SLM IN718 [Citation22]. Therefore, this study modifies SLM IN718 alloy by adding trace LaB6 and/or P with the expectation of achieving the required creep ductility.
2. Experimental details
Two batches of IN718 powder with particle size ranging from 15 to 53 µm produced by plasma rotating electrode process were purchased from Sino-Euro Materials Technologies of Xi’an Co. Their chemical compositions were measured, as shown in Table . One batch of IN718 powder (named M1) was mixed with variable contents of nanoscale LaB6 particles (200 nm) using a resonance-assisted mixing method on an ES-35WLS3-340 vibration system. This approach achieved a uniform distribution of LaB6 on the surface of IN718 powder, maintaining the original spherical morphology of the powder. Two kinds of mixed powder with low and high LaB6 contents were named M1-B1 and M1-B2, respectively. Another batch of IN718 powder (named M2) was mechanically mixed with BNi89P powder (38µm) containing 10.97 wt.% P and the balanced Ni using a VH-5L mixer. The mixed powder with 0.023 wt.% P was named M2-P. Then, the M2 powder was mixed with varying LaB6 and BNi89P contents, which were named M2-BP1 and M2-BP2. The SEM images of four kinds of powders are shown in Figure S1.
Table 1. Chemical composition of two batches of IN718 powder (wt. %).
A BLT-S200 SLM machine was used to fabricate specimens with dimensions of 82 × 15 × 15 mm3. The optimization of SLM process parameters using M1 powder is illustrated in Figure S2, and the final parameters are chosen as follows: a laser power of 275 W, a layer thickness of 40 µm, a scanning speed of 880 mm/s and a scanning spacing of 100 µm. The adjacent layer rotation angle was set at 67°. Oxygen content during the SLM process was maintained below 200 ppm. Bulk specimens with L × W × H dimensions of 80 mm × 15 mm × 15 mm were built for creep testing.
The post-heat treatment applied to the as-built SLM specimens included homogenization treatment at 1180°C for 2 h under vacuum, and two-step aging treatment at 720°C for 8 h with furnace cooling and 620°C for 8 h in air cooling. Our previous research has confirmed that this heat treatment regimen is optimal for the stress rupture performance of SLM IN718 [Citation23]. Compared to the standard heat treatment for forged or cast IN718 specified in AMS 5663 [Citation24]and 5383 [Citation25], the solution treatment at 980°C for 1 h was removed for SLM IN718 heat treatment because fine δ phase precipitates at grain boundary during the solution treatment, which would damage the stress rupture performance of SLM IN718.
The actual content of B, La and P elements in the heat-treated specimens was measured using Inductively Coupled Plasma Mass Spectrometry (ICP-MS), and the results are presented in Table .
Table 2. Measured trace element contents and creep performance of heat-treated samples.
The machining drawing of the creep test specimen is shown in Figure S3. The creep tests were conducted under 690 MPa at 650°C according to ISO 204:2009. The creep elongation of the specimen was measured with a high-temperature extensometer.
Microstructure characterization was carried out using optical microscopy and a Scanning Electron Microscope (SEM) equipped with Electron Backscatter Diffraction (EBSD) and Energy-Dispersive X-ray Spectroscopy (EDS) detectors.
3. Results and discussion
Figure (a,b) displays EBSD orientation maps of the as-built M2 and M2-BP2 samples, respectively. The as-built samples exhibit columnar-oriented microstructures, with <001> crystallographic texture along the SLM deposition direction. Figure (c) presents the SEM microstructure of the as-built M2-BP2 sample, revealing dendritic growth microstructure formed during solidification. Due to the extremely rapid cooling, the primary dendrite arm spacing is very fine, measuring about 1µm, and the secondary dendrite arms are not well-developed. Figure (d,e) displays EBSD orientation maps of the heat-treated M2 and M2-BP2 samples, respectively. It shows that recrystallization occurs during the heat treatment process, and the grain structure transforms from columnar to equiaxed and the grain size is coarsened. Other samples in this study exhibit similar as-built microstructure characteristics and recrystallization during heat treatment, as shown in Figure S4 and Figure S5. Due to recrystallization, the texture intensity was decreased significantly, so the influence of sample texture on creep performance did not become the focus of this study.
Figure 1. Longitudinal EBSD-IPF and SEM images of the SLM IN718 (a) as-built M2, (b) as-built M2-BP2, (c) as-built M2-BP2, (d) heat-treated M2, (e) heat-treated M2-BP2, (f) the porosity of as-built samples, and the grain size and the twin boundary percentage of heat-treated samples.
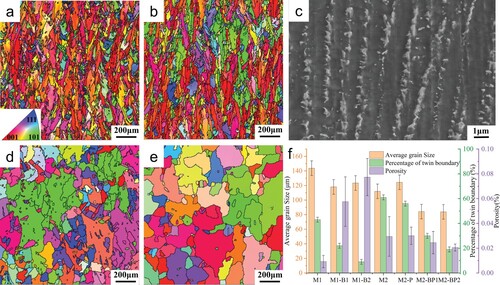
Figure (f) provides a statistical result of the average grain size and the twin boundary percentage of all heated-treated samples. It shows that the addition of LaB6 can inhibit twin boundary formation significantly and retard grain coarsening during heat treatment. Conversely, the addition of trace P element shows little influence on the recrystallization process.
Additionally, the porosity results measured by the metallographic method show an increasing amount of pores with higher addition of LaB6 while the addition of P element does not have such a detrimental effect. Nevertheless, the porosity of all samples is below 0.1%, indicating excellent SLM manufacturing quality, and it suggests that the influence of trace elements addition on SLM manufacturability of IN718 alloy is limited.
Figure shows that the solidified dendritic structure with micro-segregation of elements was homogenized in the heat-treated samples, and many bright particles precipitated both at grain boundaries and inside grains. EDS analysis shows that these precipitates are enriched in Nb and Mo elements, and identified as Nb/Mo-rich carbides. The grain boundary precipitates are mostly less than 2 µm while those inside grains are even smaller, measuring less than 0.5 µm. Table presents the statistical size analysis of grain boundary precipitates in all heat-treated samples. Compared to the original M1 sample, the grain boundary precipitates in the M1-B1 sample were refined significantly, probably due to the refinement effect of La element on carbide [Citation26]. However, as the amount of added LaB6 further increased in the M1-B2 sample, the grain boundary precipitates became much larger because the boride-carbide compound precipitated instead of carbides. A similar phenomenon also occurred in the M2-BP1 and M2-BP2 samples compared with the M2 sample. In contrast, the addition of P element shows little effect on grain boundary precipitates. Moreover, measurements reveal that there is no obvious size variation in strengthening precipitates of spherical γ′ phase and disc-shaped γ″ phase among the studied samples (Figure S6).
Figure 2. Precipitates in heat-treated samples, (a, a1) M1, (b) M1-B1, (c, c1) M1-B2, (d, d1) M2, (e, e1) M2-BP2.
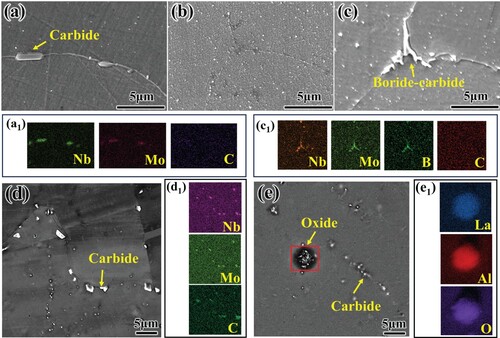
Table 3. Line number density of grain boundary precipitates (mm−1).
Additionally, after the addition of LaB6, gray oxide particles were found in the M2-BP2 samples. The number density of oxides was extremely lower, but their size can reach several micrometers. As shown in Figure (e1), the small oxides were identified as La-Al-O oxide by EDS-mapping analysis. The mass ratio of La to O is approximately 5.8:1 for La2O3 oxide. According to the element content listed in Table , the oxygen content is significantly excessive for lanthanum. Therefore, although La has a greater affinity for oxygen compared to Al, La-Al-O oxide forms due to the insufficient content of La. This also means that the rare earth element is beneficial for fixing the oxygen in superalloy.
Figure shows the creep strain—time curves of all heat-treated specimens under 650°C/690 MPa, and the corresponding creep performance is listed in Table . The creep results reveal that the individual addition of trace LaB6 can enhance both the creep life and ductility of IN718 alloy, but the creep ductility is still lower than the forging standard. The individual addition of trace P element shows no improvement in creep performance. Remarkably, the combined addition of LaB6 and P significantly enhances creep performance, especially creep ductility. As shown in the enlarged inset, the creep rate and the onset of the third creep stage of all samples have no significant difference. The prolongation of the third creep stage in the heat-treated M2-BP1 and M2-BP2 samples leads to superior creep strain, achieving as high as 6.5% and 8.6%, which fully meet the AMS 5662M requirement (≥4%).
Figure shows the creep fracture of the heat-treated samples, respectively. The creep fracture surface can be divided into two distinct regions: the crack initiation and propagation zone (I) with a smooth surface and the exposed precipitates on it, and the instantaneous fracture zone (II) with a dimpled surface. As the stress concentrated at the grain boundaries gradually during loading, grain boundary cracks first initiated in some local zones to weaken its strength, which in turn promoted the crack propagation around there. Then the crack zone developed gradually to further weaken the load-bearing capacity until instantaneous fracture occurred at a certain moment.
Figure 4. Comparison of the M2 and M2-BP2 samples after creep, (a–d) M2, (e–h) M2-BP2, (b,f) zone I, (c,g)zone II, (d,h) KAM maps of longitudinal cross-section beneath creep fracture.
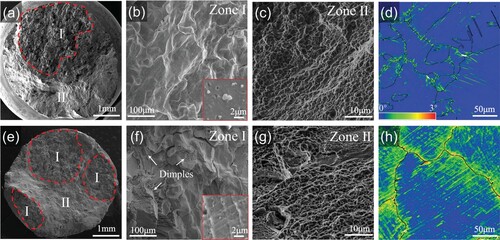
Compared to the M2 sample, fewer precipitates were observed on the creep fracture of the M2-BP2 sample. Meanwhile, it can be observed in Figure (b,f) that zone I of the M2 sample exhibits entirely smooth intergranular fracture characteristics while zone I of the M2-BP2 sample shows smooth fractures with many slip lines and partial dimple fracture, indicating a mixed fracture mode of intergranular and transgranular. Zone II in Figure (c,g) shows no visible difference. Figure (d,h) displays KAM maps of longitudinal cross-section beneath the creep fracture in the heat-treated M2 and M2-BP2 samples, respectively. The maps reveal notable dislocation pile-ups around grain boundaries, suggesting significant stress concentration at these sites. A comparison between them indicates a more pronounced strain around grain boundaries in the M2-BP2 sample after creep, agreeing with its better creep ductility. The above comparative results mean that the M2-BP2 sample exhibits larger resistance to grain boundary damage, suppressing the weakening of load-bearing capacity to facilitate greater plastic deformation [Citation27]. This ultimately results in the significant enhancement of creep ductility in the M2-BP2 sample.
Although the individual addition of LaB6 leads to the reduction of grain size and twin boundaries and the variation of grain boundary precipitates in the M1-B1 and M1-B2 samples (as exposed on the creep fracture surface in Figure S7), the enhancement of the creep strain is limited. For the combined addition of LaB6 and P in the M2-BP1 and M2-BP2 samples, the creep ductility exhibits remarkable improvement. This means that the superior creep ductility of SLM IN718 is mainly attributed to the interaction of P and B.
In the forged IN718, the combined addition of P and B at their optimum levels (P: 0.022 wt.%, B: 0.012 wt.%) can generate an increase in the stress rupture lifetime, or a decrease in the steady-state creep rate of up to tenfold, but the stress rupture ductility is unchanged [Citation18–20]. Previous studies have confirmed that no preferred P and B segregation exists in γ′/γ″ precipitates and the interfaces of γ-γ′ and γ-γ″, but both P and B strongly segregate to the grain boundaries [Citation28]. This means that the stability of γ′ and γ″ precipitates does not change with P and B additions, and thus the improvement of creep performance doesn't result from the effect of P and B on γ′ and γ″ precipitates. One proposed possible mechanism involves the effect of P and B on dislocation pinning, resulting in higher resistance to creep deformation and improving stress rupture life.
In SLM IN718, the combined addition of P and B does not induce notable changes during the steady-state creep stage but mainly prolongs the third stage of creep, especially enhancing creep ductility. SLM IN718 exhibits an extremely inhomogeneous grain structure with inferior coordination capability of creep deformation. Fine-grained regions are prone to localized stress and strain concentration, which can lead to the early initiation of cracks and their subsequent propagation along grain boundaries, ultimately resulting in fracture [Citation11]. Consequently, the creep ductility of SLM IN718 is significantly reduced compared to its forged counterpart. The synergistic effect of P and B on the creep ductility improvement of SLM IN718 would be the increased grain boundary cohesion by P and B atoms, effectively impeding crack propagation during the third stage of creep. This is also supported by the fractographic analysis, revealing that the transition from intergranular fracture to a mixture of intergranular and transgranular fracture was induced by the combined addition of P and B, which is most likely controlled by grain boundary cohesion.
In other words, the combined addition of P and B in SLM IN718 enhances the resistance to crack propagation, significantly improving its creep ductility, unlike their effect in forged IN718, which primarily enhances resistance to creep deformation and substantially prolongs creep life.
4. Conclusion
The unique microstructure characteristics of SLM IN718 lead to the rapid initiation and propagation of grain boundary damage during creep, resulting in inferior creep ductility that does not meet aerospace material specifications. This study shows that the individual addition of LaB6 leads to the variation of grain structure and grain boundary precipitates, which shows limited enhancement of creep performance. The individual addition of P does not have any beneficial effect on creep performance. The combined addition of LaB6 and P exhibits superior creep performance, especially the remarkable improvement of creep ductility which is mainly attributed to the prolongation of the third stage of creep. It is suggested that the cohesion of grain boundaries is likely to be enhanced by the synergistic effect of P and B, thereby improving the resistance to crack propagation during the third stage of creep. The solution of insufficient creep ductility by microalloying of LaB6 and P is expected to promote the engineering application of SLM IN718.
Supplemental Material
Download MS Word (10.1 MB)Disclosure statement
No potential conflict of interest was reported by the author(s).
Additional information
Funding
References
- Liu Z, Kim H, Liu W, et al. Influence of energy density on macro/micro structures and mechanical properties of as-deposited Inconel 718 parts fabricated by laser engineered net shaping. J Manuf Process. 2019;42:96–105. doi:10.1016/j.jmapro.2019.04.020
- Hosseini E, Popovich VA. A review of mechanical properties of additively manufactured Inconel 718. Addit Manuf. 2019;30:100877.
- Zhang D, Feng Z, Wang C, et al. Comparison of microstructures and mechanical properties of Inconel 718 alloy processed by selective laser melting and casting. Mater Sci Eng A. 2018;724:357–367. doi:10.1016/j.msea.2018.03.073
- Sanchez S, Hyde CJ, Ashcroft IA, et al. Multi-laser scan strategies for enhancing creep performance in LPBF. Addit Manuf. 2021;41:101948.
- Mostafaei A, Ghiaasiaan R, Ho I-T, et al. Additive manufacturing of nickel-based superalloys: a state-of-the-art review on process-structure-defect-property relationship. Prog Mater Sci. 2023;136:101108, doi:10.1016/j.pmatsci.2023.101108.
- Sanchez S, Smith P, Xu Z, et al. Powder bed Fusion of nickel-based superalloys: a review. Int J Mach Tools Manuf. 2021;165:103729, doi:10.1016/j.ijmachtools.2021.103729
- Trosch T, Strößner J, Völkl R, et al. Microstructure and mechanical properties of selective laser melted Inconel 718 compared to forging and casting. Mater Lett. 2016;164:428–431, doi:10.1016/j.matlet.2015.10.136.
- Wang LY, Zhou ZJ, Li CP, et al. Comparative investigation of small punch creep resistance of Inconel 718 fabricated by selective laser melting. Mater Sci Eng A. 2019;745:31–38. doi:10.1016/j.msea.2018.12.083
- Xu Z, Murray JW, Hyde CJ, et al. Effect of post processing on the creep performance of laser powder bed fused Inconel 718. Addit Manuf. 2018;24:486–497, doi:10.1016/j.addma.2018.10.027.
- Kuo Y-L, Horikawa S, Kakehi K. Effects of build direction and heat treatment on creep properties of Ni-base superalloy built up by additive manufacturing. Scr Mater. 2017;129:74–78, doi:10.1016/j.scriptamat.2016.10.035.
- Zhang S, Lin X, Wang L, et al. Influence of grain inhomogeneity and precipitates on the stress rupture properties of Inconel 718 superalloy fabricated by selective laser melting. Mater Sci Eng A. 2021;803:140702, doi:10.1016/j.msea.2020.140702
- Nagahari T, Nagoya T, Kakehi K, et al. Microstructure and creep properties of Ni-base superalloy IN718 built up by selective laser melting in a vacuum environment. Metals. 2020;10:362, doi:10.3390/met10030362
- Ma T, Zhang G-P, Tan P, et al. Effects of homogenization temperature on creep performance of laser powder bed fusion-fabricated Inconel 718 at 650°C. Mater Sci Eng A. 2022;853:143794, doi:10.1016/j.msea.2022.143794
- Wu S, Peng HZ, Gao X, et al. Improving creep property of additively manufactured Inconel 718 through specifically-designed post heat treatments. Mater Sci Eng A. 2022;857:144047, doi:10.1016/j.msea.2022.144047
- Zhang S, Wang L, Lin X, et al. Precipitation behavior of δ phase and its effect on stress rupture properties of selective laser-melted Inconel 718 superalloy. Compos Part B Eng. 2021;224:109202, doi:10.1016/j.compositesb.2021.109202
- AMS5662M. Nickel alloy, corrosion and heat resistant, bars, forgings, and rings 52.5Ni 19Cr 3.0Mo 5.1Cb 0.90Ti 0.50Al 18Fe, consumable electrode or vacuum induction melted 1775°F (968°C) solution heat treated, precipitation hardenable - SAE international, 2009.
- Deng D, Peng RL, Moverare J. High temperature mechanical integrity of selective laser melted alloy 718 evaluated by slow strain rate tests. Int J Plast. 2021;140:102974, doi:10.1016/j.ijplas.2021.102974
- McKamey CG, Carmichael CA, Cao WD, et al. Creep properties of phosphorus + boron-modified alloy 718. Scr Mater. 1998;38:485–491, doi:10.1016/s1359-6462(97)00476-4.
- Li N, Sun W, Xu Y, et al. Effect of P and B on the creep behavior of alloy 718. Mater Lett. 2006;60:2232–2235, doi:10.1016/j.matlet.2005.12.117.
- Horton JA, McKamey CG, Miller MK, et al. Microstructural characterization of superalloy 718 with boron and phosphorus additions. Oak Ridge (TN): Oak Ridge National Lab. (ORNL); 1997.
- Li Z, Ma C, Tian S, et al. Microstructure and creep property of isothermal forging GH4169G superalloy, high temp. Mater Process. 2014;33:447–451, doi:10.1515/htmp-2013-0063.
- Palleda TN, Banoth S, Tanaka M, et al. The role of yttrium micro-alloying on microstructure evolution and high-temperature mechanical properties of additively manufactured Inconel 718. Mater Des. 2023;225:111567, doi:10.1016/j.matdes.2022.111567.
- Zhang S, Wang L, Lin X, et al. Precipitation behavior of δ phase and its effect on stress rupture properties of selective laser-melted Inconel 718 superalloy. Compos B Eng. 2021;224:109202, doi:10.1016/j.compositesb.2021.109202
- AMS5663. Nickel alloy, corrosion and heat resistant, bars, forgings, and rings 52.5Ni 19Cr 3.0Mo 5.1Cb 0.90Ti 0.50Al 19Fe, consumable electrode or vacuum induction melted 1775°F (968°C) solution and precipitation heat treated - SAE international, 1965.
- AMS5383. Nickel alloy, corrosion and heat-resistant, investment castings, 52.5Ni 19Cr 3.0Mo 5.1Cb(Nb) 0.90Ti 0.60Al 18Fe, vacuum melted homogenization and solution heat treated - SAE international, 1966.
- Palleda TN, Chowdhury HT, Banoth S, et al. Effects of yttrium content on solidification, microstructure, and mechanical properties of laser powder bed fused IN718 superalloy. J Alloys Compd. 2024;978:173404, doi:10.1016/j.jallcom.2023.173404
- Zhao X, Zhang L, Song Y, et al. Creep deformation and damage characteristics of nickel-based superalloy GH4169. Mater Sci Eng A. 2023;885:145589, doi:10.1016/j.msea.2023.145589
- Miller MK, Horton JA, Cao WD, et al. Characterization of the effects of boron and phosphorus additions to the nickel-based superalloy 718. J Phys IV France. 1996;6:C5–246. doi:10.1051/jp1:1996129