ABSTRACT
Osteogenesis imperfecta (OI) Type VI is characterized by a defect in bone mineralization, which results in multiple fractures early in life. Null mutations in the PEDF gene, Serpinf1, are the cause of OI VI. Whether PEDF restoration in a murine model of OI Type VI could improve bone mass and function was previously unknown. In Belinsky et al, we provided evidence that PEDF delivery enhanced bone mass and improved parameters of bone function in vivo. Further, we demonstrated that PEDF temporally inhibits Wnt signaling to enhance osteoblast differentiation. Here, we demonstrate that generation of induced pluripotent stem cells (iPSCs) from a PEDF null patient provides additional evidence for PEDF's role in regulating extracellular matrix proteins secreted from osteoblasts. PEDF null iPSCs have marked abnormalities in secreted matrix proteins, capturing a key feature of human OI Type VI, which were normalized by exogenous PEDF. Lastly, we place our recent findings within the broader context of PEDF biology and the developmental signaling pathways that are implicated in its actions.
PEDF null mutations result in Osteogenesis imperfecta type VI
Osteogenesis imperfecta (OI) type VI is an autosomal recessive disease characterized by defects in bone mineralization and multiple fractures starting at 6 months of age.Citation1 The single gene defect in OI type VI is Serpinf1, which encodes for the circulating protein pigment epithelium-derived factor (PEDF).Citation2-5 Several truncation mutations in Serpinf1 have been reported, which result in complete absence of circulating PEDF.Citation2-5 The histological hallmark of bones from OI type VI patients is an excess of bone matrix (osteoid) that is inadequately mineralized. These features are recapitulated in the murine model of OI type VI.Citation6
In addition to null mutations, homozygous in-frame deletion or insertion mutations in the Serpinf1 gene cause retention or degradation of PEDF within intracellular compartments and result in markedly reduced PEDF secretion with a clinical presentation resembling the PEDF null state.Citation7 In this form of OI type VI, PEDF levels are more than 10-fold lower than in normal individuals, and clinical presentation may appear at a more advanced age than in patients with complete absence of PEDF. Further, a subtype of OI type V with a S40L mutation in the protein product of the IFITM5 gene results in a histological bone phenotype indistinguishable from the PEDF null state.Citation8 Mutations in the PEDF gene and its involvement in another OI subtype have provided evidence to support a role for PEDF in bone development.
A brief perspective on PEDF biology
PEDF is a 50kDa secreted glycoprotein that was first identified and isolated from the conditioned medium of cultured human fetal retinal pigment epithelium cells.Citation9,10 The PEDF gene, Serpinf1, is located on chromosome 17p13 and expressed in many tissues.Citation10,11 The highest expression levels found in adult humans are in the liver and then adipose tissue.Citation11,12,13 Expression in organs such as the eye, heart, pancreas and others indicate a broad distribution for this protein.Citation11 Circulating levels in human sera are typically ∼100 nM in normal-weight individuals,Citation13 are increased in the obese and in patients with metabolic syndrome to approximately 500 nM,Citation14 and are undetectable in those with Osteogenesis imperfecta type VI.Citation5
Prior to the discovery of the PEDF null state in humans as OI Type VI, functional studies highlighted neurotrophic and anti-angiogenic effects of PEDF.Citation15,16 More recently, numerous studies identified serum PEDF levels as a biomarker of metabolic syndrome, with circulating levels correlating with the degree of adiposity or metabolic dysfunction.Citation14,17,18 Ma and colleagues first provided evidence that offered a unifying explanation for these wide-ranging findings. In retinal epithelial cells, PEDF physically interacted with the Wnt co-receptor low density lipoprotein receptor-related protein 6 (LRP6) and inhibited canonical Wnt ligand-mediated effects.Citation19 Based on these findings, we found that the PEDF protein could direct murine and human mesenchymal stem cells (MSCs) to the osteoblast lineage while impeding adipogenesis in a temporally restricted manner.Citation20 Deletion of LRP6 from progenitor cells abrogated these PEDF-mediated effects.Citation20 The discovery by our group that PEDF-null mice displayed both reduced bone mass and increased adiposity provided in vivo confirmation that PEDF regulates the differentiation of MSCs to the bone cell lineage, at the expense of adipogenesis.Citation20
PEDF enhances osteoblast differentiation in vitro and bone mass in vivo
Our group and others have demonstrated that PEDF can direct MSCs to the osteoblast lineage and regulate genes involved in osteogenesis.Citation20-22 Niyibizi and colleagues reported that exogenous PEDF led to enhanced mineralization and increased expression of osteoblast-related genes, such as alkaline phosphatase in human MSCs.Citation21 PEDF knockdown in hMSCs led to a significant decrease in osteoblast differentiation and mineralization with rescue of differentiation by exogenous PEDF.Citation21 PEDF also enhanced β-catenin levels while suppressing endogenous inhibitors of Wnt signaling that inhibit bone formation, such as sclerostin.Citation20,22 The anatomical localization of increased PEDF by in situ hybridization and staining within proliferative and hypertrophic zones of the epiphyseal growth plate and in osteoblasts lining the bone spicule is consistent with a role in osteoblast differentiation.Citation23,24 In contrast, PEDF expression toward the base of the growth plate and in mature (9 week-old) animals was nearly absent.Citation23 Thus, a PEDF-directed effect on MSC to osteoblast differentiation and its presence on regions of new bone development provide a basis for explaining how the absence of PEDF results in inadequate bone formation. Next, we provide an overview of our recent work.
In our recent workCitation51 we tested the ability of PEDF to increase bone mass in adult wild-type and PEDF-null mice.Citation25 PEDF protein delivery had no effect on bone mass in wild-type mice, but systemic injection of PEDF in fully mature null mice increased trabecular bone mass by 50% at 4 weeks. Subsequently, PEDF delivery in young (19 day-old) PEDF null mice led to a modest increase in bone mass. These trophic effects were accompanied by functional studies that demonstrated improved bone plasticity in the setting of PEDF reconstitution. The relatively modest effect on bone mass in younger compared to fully mature mice provided us additional clues as to the mechanisms of PEDF-directed bone differentiation. As noted, a pathological hallmark of OI type VI and the PEDF-null mice is the presence of excess and unmineralized bone matrix or osteoid. This likely represents a defect in terminal osteoblast differentiation leading us to speculate that accumulation of unmineralized osteoid was more abundant in the older PEDF-null mice. Thus, restoration of PEDF and the greater increase in bone density in older mice might reflect a PEDF effect oN-Terminal differentiation of osteoblasts and mineralization of more abundant osteoid in older mice. This will require further confirmation.
To delineate the mechanisms by which PEDF enhances bone mass, we examined its effects on Wnt signaling and its modulation of the canonical Wnt3a ligand in human MSCs undergoing differentiation to osteoblasts.Citation25 The temporal course of Wnt signaling during differentiation was assessed using a Wnt-β-catenin-GFP reporter. In human MSCs, Wnt-GFP reporter activity was most robust early and was nearly absent at the end of 21 d of MSC to osteoblast differentiation. In contrast, MSCs in non-osteogenic media showed increasing Wnt-GFP activity over time. PEDF secretion was minimal at early time points and gradually increased through osteoblast differentiation. Given this reciprocal regulation between Wnt activity and PEDF secretion, we modeled unopposed Wnt stimulation by providing exogenous Wnt3a ligand throughout the course of differentiation. Continuous Wnt3a exposure led to less well-differentiated MSCs, as determined by diminished mineralization compared to cells in osteoblast differentiation media alone. The combination of continuous Wnt3a ligand and PEDF, provided in the final 8 d of differentiation, normalized mineralization. These results indicate that PEDF antagonizes the effects of Wnt3a ligand and allows for terminal differentiation of hMSCs. We further demonstrated that, like the well-known Wnt signaling inhibitor DKK1, PEDF has an inhibitory effect on the activation status of the Wnt co-receptor LRP6.Citation26 Similar to PEDF, silencing of DKK1 blocked terminal osteoblast differentiation,Citation27 thereby indicating that multiple endogenous mechanisms are required to turn off Wnt signaling to allow for osteoblast differentiation. Determination of the precise temporal regulation of osteoblast differentiation by PEDF, DKK1 and other Wnt modulators will require additional investigation.
Prior to our work, another group published that PEDF restoration had no effect on bone mass in PEDF-null mice.Citation28 Since our work reached the opposite conclusion, differences in these studies may explain the divergent findings. Rajagopal et al used a viral vector delivery system that achieved serum PEDF levels >1000 µg/mL. These levels are far outside of the normal physiological range of PEDF reported in mice and humans. PEDF levels in wild-type mice reported by our group and others ranged from ∼10–120 ng/mL.Citation29,30 In humans, PEDF levels are ∼5–20 µg/mL.Citation13,14 PEDF levels obtained using a viral vector delivery system were, therefore, several magnitudes higher than levels reported in mice or humans. Prior functional studies of PEDF biology identified paradoxical effects with higher concentrations of PEDF.Citation31 The mice used in that study were much older mice (6 months at sacrifice) than those used in our study (<3 weeks of age). Thus, several factors in that study differ from our paper, which found a trophic effect on bone mass with PEDF restoration.
PEDF-null iPSCs display key features of OI type VI
Generation of iPSCs from a PEDF-null patient has allowed us to evaluate the effects of PEDF loss on osteoblast differentiation and determine whether PEDF could correct these defects. The patient was a 2-year old child who presented with multiple fractures. Sequencing of the PEDF gene identified an in-frame duplication of 3 amino acids (p.Ala91_Ser93dup) that had also been described by Al-Jallad et al.Citation7 In that study overexpression of the mutant PEDF sequence into osteoblasts resulted in diminished collagen type I deposition and mineralization.Citation7 The iPSCs generated from our patient were confirmed to express pluripotency markers and had the ability to differentiate toward the mesenchymal lineage (data not shown). Significantly, when these iPSCs were differentiated in osteogenic media, collagen I expression was reduced compared to control iPSCs (). In addition, the expression of the gene for bone sialoprotein, IBSP, was increased 25-fold in 2 separate lines derived from 2 different iPSC clones compared to cells from control iPSCs (). Exogenous PEDF added to differentiated OI VI iPSCs reduced IBSP expression and protein (). This finding is notable because bone sialoprotein is a major constituent of the extracellular matrix secreted by osteoblasts and other cells. Overexpression of IBSP in mice results in decreased bone mass, indicating that regulation of bone matrix proteins can determine bone development and density.Citation32,33 Thus, PEDF regulation of IBSP in iPSCs derived from an OI VI patient identifies PEDF-mediated regulation of another matrix protein that regulates bone development.
Figure 1. Wildtype (WT) or OI6 iPSC-derived MSCs were differentiated for 21 d in osteogenic media. (A) Col1A1 qPCR on WT and 3 OI6 iPSC-derived clones. (B) IBSP qPCR on WT and 3 OI6 iPSC-derived clones. (C) IBSP expression on WT and clone1 with PEDF treatment. (D) Immunoblots for IBSP protein from WT or OI6 clone 1 cells. Where indicated, 300 ng/ml PEDF was added to the culture media on days 14–21. error bars = SEM, 3–6 biological replicates per group.
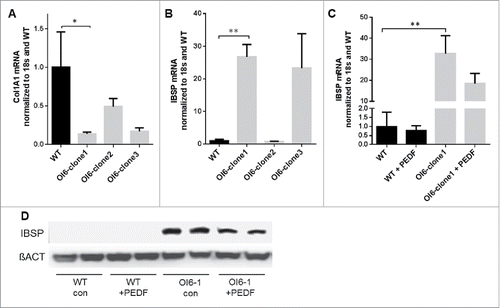
Conclusion
In retrospect, the original studies on PEDF biology strongly suggested its role in modulating a fundamental developmental signaling pathway. PEDF was first identified as a neuronal differentiation factor that induced retinoblastoma (Rb) cells to adopt neuronal markers characteristic of a terminally differentiated state.Citation10,34,35 These differentiating effects were further demonstrated in vivo with the observation that recombinant PEDF stimulated poorly differentiated neuroblastoma cells into a mature cellular phenotype.Citation36 Paradoxically, treatment of Rb cells with PEDF and in vivo transplantation led to massive tumor growth indicating that PEDF has striking context-specific effects.Citation37 This is reflected in the ability of PEDF to promote stem cell renewal in certain stem cell populations,Citation38 while other studies suggest a role for PEDF in their differentiation.Citation19,39-41
Many rare human genetic diseases with gain or loss of bone mass converge on aberrant Wnt signaling.Citation42 Since Wnt signaling plays a fundamental role in development and adult tissue homeostasis, it is not surprising that many regulators of Wnt signaling display broad functional effects outside of bone development.Citation42-45 In a similar manner, OI type VI has provided insights into PEDF's role in bone development and its other well-known functions. A Wnt signaling effect by PEDF has now been described in diverse tissue sites including the retina, skin, liver, and now bone with the common finding that PEDF inhibits Wnt signaling in differentiated and cancer cells.Citation19,22,39,46,47 In recent years, a vital role for Wnt signaling has been attributed to common diseases such as diabetes and the aberrant angiogenesis found in diabetic retinopathy.Citation45,48,49 The strong overlap of PEDF biology with these disorders warrants investigation to determine whether PEDF-directed modulation of Wnt signaling occurs in this context. This would further support the idea that PEDF is a circulating inhibitor of the canonical Wnt signaling pathway.
Methods
Patient material
Informed consent was obtained from the parents of a 2 year-old child with newly diagnosed OI Type VI. The IRB of the Children's Hospital of Eastern Ontario and Yale University School of Medicine approved this study.
Generation of iPSCs
iPSC cells were created from peripheral leukocytes using episomal non-integrating plasmids.Citation50 Expression of SOX2, OCT4 and NANOG pluripotency markers was verified by qPCR. Cells were differentiated toward an MSC lineage by passaging on normal cell culture plastic in 10% FBS, 67% DMEM, 22% F12. Confirmation of MSC markers (cd29, cd73 and nCAD) was confirmed by qPCR. MSC-like cells were differentiated into osteoblast-like cells in standard osteogenic media consisting of 10% FBS, MEM α + 50 µg/ml ascorbic acid 2-phosphate, 10 nM dexamethasone, and 2.5 mM β-glycerol phosphate. After 21 d in osteogenic media, Trizol extracts were made, and RNA and protein isolated.
Disclosure of potential conflicts of interest
No potential conflicts of interest were disclosed.
Acknowledgments
We acknowledge the contributions of the OI VI patient who donated cells for the generation of PEDF null iPSCs and the co-authors of our recent publication.
Funding
This work was supported in part by a VA Merit Grant and grant number 5P30DK034989 from the National Institute of Diabetes and Digestive and Kidney Diseases (NIDDK).
References
- Glorieux FH, Ward LM, Rauch F, Lalic L, Roughley PJ, Travers R. Osteogenesis imperfecta type VI: a form of brittle bone disease with a mineralization defect. J Bone Miner Res 2002; 17:30-8; PMID:11771667; http://dx.doi.org/10.1359/jbmr.2002.17.1.30
- Becker J, Semler O, Gilissen C, Li Y, Bolz HJ, Giunta C, Bergmann C, Rohrbach M, Koerber F, Zimmermann K, et al. Exome sequencing identifies truncating mutations in human SERPINF1 in autosomal-recessive Osteogenesis imperfecta. Am J Hum Genet 2011; 88:362-71; PMID:21353196; http://dx.doi.org/10.1016/j.ajhg.2011.01.015
- Homan EP, Rauch F, Grafe I, Lietman C, Doll JA, Dawson B, Bertin T, Napierala D, Morello R, Gibbs R, et al. Mutations in SERPINF1 cause Osteogenesis imperfecta type VI. J Bone Miner Res 2011; 26:2798-803; PMID:21826736; http://dx.doi.org/10.1002/jbmr.487
- Venturi G, Gandini A, Monti E, Dalle Carbonare L, Corradi M, Vincenzi M, Valenti MT, Valli M, Pelilli E, Boner A, et al. Lack of expression of SERPINF1, the gene coding for pigment epithelium-derived factor, causes progressively deforming Osteogenesis imperfecta with normal type I collagen. J Bone Miner Res 2012; 27:723-8; PMID:22113968; http://dx.doi.org/10.1002/jbmr.1480
- Rauch F, Husseini A, Roughley P, Glorieux FH, Moffatt P. Lack of circulating pigment epithelium-derived factor is a marker of Osteogenesis imperfecta type VI. J Clin Endocrinol Metab 2012; 97:E1550-6; PMID:22669302; http://dx.doi.org/10.1210/jc.2012-1827
- Bogan R, Riddle RC, Li Z, Kumar S, Nandal A, Faugere MC, Boskey A, Crawford SE, Clemens TL. A mouse model for human Osteogenesis imperfecta type VI. J Bone Miner Res 2013; 28:1531-6; PMID:23413146; http://dx.doi.org/10.1002/jbmr.1892
- Al-Jallad H, Palomo T, Roughley P, Glorieux FH, McKee MD, Moffatt P, Rauch F. The effect of SERPINF1 in-frame mutations in Osteogenesis imperfecta type VI. Bone 2015; 76:115-20; PMID:25868797; http://dx.doi.org/10.1016/j.bone.2015.04.008
- Farber CR, Reich A, Barnes AM, Becerra P, Rauch F, Cabral WA, Bae A, Quinlan A, Glorieux FH, Clemens TL, et al. A novel IFITM5 mutation in severe atypical Osteogenesis imperfecta type VI impairs osteoblast production of pigment epithelium-derived factor. J Bone Miner Res 2014; 29:1402-11; PMID:24519609; http://dx.doi.org/10.1002/jbmr.2173
- Tombran-Tink J, Johnson LV. Neuronal differentiation of retinoblastoma cells induced by medium conditioned by human RPE cells. Invest Ophthalmol Vis Sci 1989; 30:1700-7; PMID:2668219
- Steele FR, Chader GJ, Johnson LV, Tombran-Tink J. Pigment epithelium-derived factor: neurotrophic activity and identification as a member of the serine protease inhibitor gene family. Proc Natl Acad Sci U S A 1993; 90:1526-30; PMID:8434014; http://dx.doi.org/10.1073/pnas.90.4.1526
- Tombran-Tink J, Mazuruk K, Rodriguez IR, Chung D, Linker T, Englander E, Chader GJ. Organization, evolutionary conservation, expression and unusual Alu density of the human gene for pigment epithelium-derived factor, a unique neurotrophic serpin. Mol Vis 1996; 2:11; PMID:9238088
- Uhlen M, Fagerberg L, Hallstrom BM, Lindskog C, Oksvold P, Mardinoglu A, Sivertsson Å, Kampf C, Sjöstedt E, Asplund A, et al. Proteomics. Tissue-based map of the human proteome. Science 2015; 347:1260419; PMID:25613900; http://dx.doi.org/10.1126/science.1260419
- Petersen SV, Valnickova Z, Enghild JJ. Pigment-epithelium-derived factor (PEDF) occurs at a physiologically relevant concentration in human blood: purification and characterization. Biochem J 2003; 374:199-206; PMID:12737624; http://dx.doi.org/10.1042/bj20030313
- Yamagishi S, Adachi H, Abe A, Yashiro T, Enomoto M, Furuki K, Hino A, Jinnouchi Y, Takenaka K, Matsui T, et al. Elevated serum levels of pigment epithelium-derived factor in the metabolic syndrome. J Clin Endocrinol Metab 2006; 91:2447-50; PMID:16522692; http://dx.doi.org/10.1210/jc.2005-2654
- Tombran-Tink J, Barnstable CJ. PEDF: a multifaceted neurotrophic factor. Nat Rev Neurosci 2003; 4:628-36; PMID:12894238; http://dx.doi.org/10.1038/nrn1176
- Dawson DW, Volpert OV, Gillis P, Crawford SE, Xu H, Benedict W, Bouck NP. Pigment epithelium-derived factor: a potent inhibitor of angiogenesis. Science 1999; 285:245-8; PMID:10398599; http://dx.doi.org/10.1126/science.285.5425.245
- Chen C, Tso AW, Law LS, et al. Plasma level of pigment epithelium-derived factor is independently associated with the development of the metabolic syndrome in Chinese men: a 10-year prospective study. J Clin Endocrinol Metab 2010; 95:5074-81; PMID:20685859; http://dx.doi.org/10.1210/jc.2010-0727
- Choi KM, Hwang SY, Hong HC, Yang SJ, Choi HY, Yoo HJ, Lee KW, Nam MS, Park YS, Woo JT, et al. C1q/TNF-related protein-3 (CTRP-3) and pigment epithelium-derived factor (PEDF) concentrations in patients with type 2 diabetes and metabolic syndrome. Diabetes 2012; 61:2932-6; PMID:22837306; http://dx.doi.org/10.2337/db12-0217
- Park K, Lee K, Zhang B, Zhou T, He X, Gao G, Murray AR, Ma JX. Identification of a novel inhibitor of the canonical Wnt pathway. Mol Cell Biol 2011; 31:3038-51; PMID:21576363; http://dx.doi.org/10.1128/MCB.01211-10
- Gattu AK, Swenson ES, Iwakiri Y, Samuel VT, Troiano N, Berry R, Church CD, Rodeheffer MS, Carpenter TO, Chung C. Determination of mesenchymal stem cell fate by pigment epithelium-derived factor (PEDF) results in increased adiposity and reduced bone mineral content. Faseb J 2013; 27:4384-94; PMID:23887690; http://dx.doi.org/10.1096/fj.13-232900
- Li F, Song N, Tombran-Tink J, Niyibizi C. Pigment epithelium-derived factor enhances differentiation and mineral deposition of human mesenchymal stem cells. Stem Cells 2013; 31:2714-23; PMID:23939834; http://dx.doi.org/10.1002/stem.1505
- Li F, Song N, Tombran-Tink J, Niyibizi C. Pigment epithelium derived factor suppresses expression of sost/sclerostin by osteocytes: implication for its role in bone matrix mineralization. J Cell Physiol 2015; 230:1243-9; PMID:25363869; http://dx.doi.org/10.1002/jcp.24859
- Quan GM, Ojaimi J, Li Y, Kartsogiannis V, Zhou H, Choong PF. Localization of pigment epithelium-derived factor in growing mouse bone. Calcif Tissue Int 2005; 76:146-53; PMID:15549636; http://dx.doi.org/10.1007/s00223-004-0068-2
- Tombran-Tink J, Barnstable CJ. Osteoblasts and osteoclasts express PEDF, VEGF-A isoforms, and VEGF receptors: possible mediators of angiogenesis and matrix remodeling in the bone. Biochem Biophys Res Commun 2004; 316:573-9; PMID:15020256; http://dx.doi.org/10.1016/j.bbrc.2004.02.076
- Belinsky GS, Sreekumar B, Andrejecsk JW, Saltzman WM, Gong J, Herzog RI, Lin S, Horsley V, Carpenter TO, Chung C. Pigment epithelium-derived factor restoration increases bone mass and improves bone plasticity in a model of Osteogenesis imperfecta type VI via Wnt3a blockade. FASEB J 2016; PMID:27127101
- Cruciat CM, Niehrs C. Secreted and transmembrane wnt inhibitors and activators. Cold Spring Harb Perspect Biol 2013; 5:a015081; PMID:23085770; http://dx.doi.org/10.1101/cshperspect.a015081
- van der Horst G, van der Werf SM, Farih-Sips H, van Bezooijen RL, Lowik CW, Karperien M. Downregulation of Wnt signaling by increased expression of Dickkopf-1 and -2 is a prerequisite for late-stage osteoblast differentiation of KS483 cells. J Bone Miner Res 2005; 20:1867-77; PMID:16160745; http://dx.doi.org/10.1359/JBMR.050614
- Rajagopal A, Homan EP, Joeng KS, Suzuki M, Bertin T, Cela R, Munivez E, Dawson B, Jiang MM, Gannon F, et al. Restoration of the serum level of SERPINF1 does not correct the bone phenotype in Serpinf1 null mice. Mol Genet Metab 2015; 117(3):378-82; PMID:26693895; http://dx.doi.org/10.1016/j.ymgme.2015.11.015
- Crowe S, Wu LE, Economou C, Turpin SM, Matzaris M, Hoehn KL, Hevener AL, James DE, Duh EJ, Watt MJ, et al. Pigment epithelium-derived factor contributes to insulin resistance in obesity. Cell Metab 2009; 10:40-7; PMID:19583952; http://dx.doi.org/10.1016/j.cmet.2009.06.001
- Gattu AK, Birkenfeld AL, Iwakiri Y, Jay S, Saltzman M, Doll J, Protiva P, Samuel VT, Crawford SE, Chung C. Pigment epithelium-derived factor (PEDF) suppresses IL-1beta-mediated c-Jun N-terminal kinase (JNK) activation to improve hepatocyte insulin signaling. Endocrinology 2014; 155:1373-85; PMID:24456163; http://dx.doi.org/10.1210/en.2013-1785
- Apte RS, Barreiro RA, Duh E, Volpert O, Ferguson TA. Stimulation of neovascularization by the anti-angiogenic factor PEDF. Invest Ophthalmol Vis Sci 2004; 45:4491-7; PMID:15557459; http://dx.doi.org/10.1167/iovs.04-0172
- Valverde P, Zhang J, Fix A, et al. Overexpression of bone sialoprotein leads to an uncoupling of bone formation and bone resorption in mice. J Bone Miner Res 2008; 23:1775-88; PMID:18597627; http://dx.doi.org/10.1359/jbmr.080605
- Styrkarsdottir U, Halldorsson BV, Gretarsdottir S, Gudbjartsson DF, Walters GB, Ingvarsson T, Jonsdottir T, Saemundsdottir J, Snorradóttir S, Center JR, et al. New sequence variants associated with bone mineral density. Nat Genet 2009; 41:15-7; PMID:19079262; http://dx.doi.org/10.1038/ng.284
- Becerra SP, Palmer I, Kumar A, Steele F, Shiloach J, Notario V, Chader GJ. Overexpression of fetal human pigment epithelium-derived factor in Escherichia coli. A functionally active neurotrophic factor. J Biol Chem 1993; 268:23148-56; PMID:8226833
- Tombran-Tink J, Chader GG, Johnson LV. PEDF: a pigment epithelium-derived factor with potent neuronal differentiative activity. Exp Eye Res 1991; 53:411-4; PMID:1936177; http://dx.doi.org/10.1016/0014-4835(91)90248-D
- Crawford SE, Stellmach V, Ranalli M, Huang X, Huang L, Volpert O, De Vries GH, Abramson LP, Bouck N. Pigment epithelium-derived factor (PEDF) in neuroblastoma: a multifunctional mediator of Schwann cell antitumor activity. J Cell Sci 2001; 114:4421-8; PMID:11792807
- Seigel GM, Tombran-Tink J, Becerra SP, Chader GJ, Diloreto DA, Jr, del Cerro C, Lazar ES, del Cerro M. Differentiation of Y79 retinoblastoma cells with pigment epithelial-derived factor and interphotoreceptor matrix wash: effects on tumorigenicity. Growth Factors 1994; 10:289-97; PMID:7803045; http://dx.doi.org/10.3109/08977199409010995
- Ramirez-Castillejo C, Sanchez-Sanchez F, Andreu-Agullo C, Ferrón SR, Aroca-Aguilar JD, Sánchez P, Mira H, Escribano J, Fariñas I. Pigment epithelium-derived factor is a niche signal for neural stem cell renewal. Nat Neurosci 2006; 9:331-9; PMID:16491078; http://dx.doi.org/10.1038/nn1657
- Qi W, Yang C, Dai Z, Che D, Feng J, Mao Y, Cheng R, Wang Z, He X, Zhou T, et al. High levels of pigment epithelium-derived factor in diabetes impair wound healing through suppression of wnt signaling. Diabetes 2014; 64(4):1407-19; PMID:25368097; http://dx.doi.org/10.2337/db14-1111
- Shin ES, Sorenson CM, Sheibani N. PEDF expression regulates the proangiogenic and proinflammatory phenotype of the lung endothelium. Am J Physiol Lung Cell Mol Physiol 2014; 306:L620-34; PMID:24318110; http://dx.doi.org/10.1152/ajplung.00188.2013
- Gonzalez R, Jennings LL, Knuth M, Orth AP, Klock HE, Ou W, Feuerhelm J, Hull MV, Koesema E, Wang Y, et al. Screening the mammalian extracellular proteome for regulators of embryonic human stem cell pluripotency. Proc Natl Acad Sci U S A 2010; 107:3552-7; PMID:20133595; http://dx.doi.org/10.1073/pnas.0914019107
- Baron R, Kneissel M. WNT signaling in bone homeostasis and disease: from human mutations to treatments. Nat Med 2013; 19:179-92; PMID:23389618; http://dx.doi.org/10.1038/nm.3074
- Nusse R. Wnt signaling. Cold Spring Harb Perspect Biol 2012; 1-4; http://dx.doi.org/10.1101/cshperspect.a011163
- Laine CM, Joeng KS, Campeau PM, Kiviranta R, Tarkkonen K, Grover M, Lu JT, Pekkinen M, Wessman M, Heino TJ, et al. WNT1 mutations in early-onset osteoporosis and Osteogenesis imperfecta. N Engl J Med 2013; 368:1809-16; PMID:23656646; http://dx.doi.org/10.1056/NEJMoa1215458
- Liu H, Fergusson MM, Wu JJ, Rovira II, Liu J, Gavrilova O, Lu T, Bao J, Han D, Sack MN, Finkel T. Wnt signaling regulates hepatic metabolism. Sci Signal 2011; 4:ra6; PMID:21285411
- Protiva P, Gong J, Sreekumar B, Torres R, Zhang X, Belinsky GS, Cornwell M, Crawford SE, Iwakiri Y, Chung C. Pigment Epithelium-Derived Factor (PEDF) inhibits Wnt/-catenin signaling in the liver. Cell Mol Gastroenterol Hepatol 2015; 1:535-49; PMID:26473164; http://dx.doi.org/10.1016/j.jcmgh.2015.06.006
- Tsai TH, Shih SC, Ho TC, Ma HI, Liu MY, Chen SL, Tsao YP. Pigment epithelium-derived factor 34-mer peptide prevents liver fibrosis and hepatic stellate cell activation through down-regulation of the PDGF receptor. PLoS One 2014; 9:e95443; PMID:24763086; http://dx.doi.org/10.1371/journal.pone.0095443
- Liu W, Singh R, Choi CS, Lee HY, Keramati AR, Samuel VT, Lifton RP, Shulman GI, Mani A. Low density lipoprotein (LDL) receptor-related protein 6 (LRP6) regulates body fat and glucose homeostasis by modulating nutrient sensing pathways and mitochondrial energy expenditure. J Biol Chem 2012; 287:7213-23; PMID:22232553; http://dx.doi.org/10.1074/jbc.M111.286724
- Dejana E. The role of wnt signaling in physiological and pathological angiogenesis. Circ Res 2010; 107:943-52; PMID:20947863; http://dx.doi.org/10.1161/CIRCRESAHA.110.223750
- Okita K, Matsumura Y, Sato Y, Okada A, Morizane A, Okamoto S, Hong H, Nakagawa M, Tanabe K, Tezuka K, et al. A more efficient method to generate integration-free human iPS cells. Nat Methods 2011; 8:409-12; PMID:21460823; http://dx.doi.org/10.1038/nmeth.1591
- Belinsky GS, et al. Pigment epithelium–derived factor restoration increases bone mass and improves bone plasticity in a model of Osteogenesis imperfecta type VI via Wnt3a blockade. FASEB Journal 2016 [epub ahead of print]; http://dx.doi.org/10.1096/fj.201500027R