Abstract
Background: There has been no comprehensive longitudinal study of pulmonary functions (PFTS) in ALS determining which measure is most sensitive to declines in respiratory muscle strength. Objective: To determine the longitudinal decline of PFTS in ALS and which measure supports Medicare criteria for NIV initiation first. Methods: Serial PFTs (maximum voluntary ventilation (MVV), maximum inspiratory pressure measured by mouth (MIP) or nasal sniff pressure (SNIP), maximum expiratory pressure (MEP), and Forced Vital Capacity (FVC)) were performed over 12 months on 73 ALS subjects to determine which measure showed the sentinel decline in pulmonary function. The rate of decline for each measure was determined as the median slope of the decrease over time. Medicare-based NIV initiation criteria were met if %FVC was ≤ 50% predicted or MIP was ≤ 60 cMH2O. Results: 65 subjects with at least 3 visits were included for analyses. All median slopes were significantly different than zero. MEP and sitting FVC demonstrated the largest rate of decline. Seventy subjects were analyzed for NIV initiation criteria, 69 met MIP criteria first; 11 FVC and MIP criteria simultaneously and none FVC criteria first. Conclusions: MEP demonstrated a steeper decline compared to other measures suggesting expiratory muscle strength declines earliest and faster and the use of airway clearance interventions should be initiated early. When Medicare criteria for NIV initiation are considered, MIP criteria are met earliest. These results suggest that pressure-based measurements are important in assessing the timing of NIV and the use of pulmonary clearance interventions.
Introduction
The use of noninvasive ventilation (NIV) has become a cornerstone treatment for ALS (Citation1). NIV has been shown to prolong survival and improve cognition, sleep, and quality of life in patients living with ALS (Citation2–7). Current guidelines of the American Academy of Neurology (AAN) recommend the use of NIV when respiratory symptoms start or when forced vital capacity (FVC) drops to <50% of predicted value (Citation1). Clinical practitioners initiate NIV based on the AAN and Medicare guidelines. Medicare guidelines for noninvasive respiratory assistance devices in patients are based on restrictive lung disease and require that the patient does not achieve a MIP of at least 60 cmH2O or has a FVC ≤ 50% predicted (Citation1,Citation8). In contrast, the European Federation of Neurological Societies (EFNS) recommend initiating NIV when a patient is symptomatic or has a FVC < 80%, SNIP< −40cmH2O, significant nocturnal deaturation, or a morning pCO2 > 45 (Citation9). A large survey of 186 ALS clinicians across US and European centers underscored the differences in prescribing behaviors with US specialists influenced by Medicare guidelines and insurance reimbursement while European specialists relied most heavily on symptoms (Citation10). This underscores the need for understanding the changes in respiratory function and what changes should be adopted to provide optimal utilization of NIV in ALS. In fact, a recent placebo-controlled study of 54 ALS subjects demonstrated a slower decline in FVC when NIV was implemented at FVC > 80% (Citation11). Furthermore, retrospective studies have demonstrated early NIV initiation can improve survival (Citation12).
While FVC is currently regarded as the standard indicator of disease progression, previous studies have suggested that other pulmonary function measures may be more sensitive indicators of respiratory dysfunction and could lead to earlier initiation of NIV (Citation13). Given the impact of NIV on both quality of life and survival, it is critical that the implementation of this modality is optimized to maximize its benefits. There has not been a systematic study of the full spectrum of pulmonary function tests to determine which measure presages the decline of respiratory muscle strength and which could provide the optimal criterion for NIV implementation.
We performed serial pulmonary function tests over 12 months as a component of a multicenter pilot study to examine nutrition and NIV in ALS patients in order to determine the most sensitive indicator of early decline in respiratory muscle function. In this way, the decline in the different measures can be compared to the “standard of care”. As part of the examination of pulmonary function decline, we also sought to determine if Medicare criteria for initiation of NIV were met first by FVC or if others measures were more sensitive in the ALS population, characterized by progressive loss of muscle power. Our findings should inform how NIV is implemented in clinical practice. Furthermore, by optimizing the start of NIV to coincide with the earliest signs of respiratory muscle weakness, the long-term efficacy of this modality may be enhanced.
Materials and methods
This work was part of a multicenter pilot study of Nutrition and NIV previously described (Citation14). This pilot included 5 nutrition-emphasis sites and 7 NIV-emphasis sites. The NIV emphasis sites employed a stratified design and entered patients into two groups based on the best sitting forced vital capacity (FVC): Group 1, 80–95% of predicted in the NIV Arm and Group 2, 50–79% of predicted. The overall goal of the NIV arm in this pilot study was to determine the rate of NIV acceptance and tolerance with early intervention (FVC at 80%) vs. standard of care (50%) and to identify factors that influence acceptance and tolerance of NIV at each level. In this regard, Group 1 was started on NIV when the FVC fell to 80% and Group 2 was initiated on NIV when the FVC fell to 50%.
An additional goal of this study, reported in this manuscript, was to determine the earliest and most rapid changes of respiratory dysfunction. To this end, subjects in the NIV arm were evaluated at baseline, 8,16,24,32,40 and 48 weeks with pulmonary functions measured with patients in an upright sitting position including: Forced sitting vital capacity (SFVC), sniff nasal pressure (SSNIP), maximal inspiratory pressure (MIP), maximal expiratory pressure (MEP), and maximal voluntary ventilation (MVV). Additionally, because of known effects of position on diaphragm function, SNIP and FVC were also measured in the supine (lying) position (LSNIP and LFVC). FVC and MVV were measured by spirometry (Respironics Renaissance). Pressure measurements were measured using a portable electronic pressure meter (MicroMedical). All measures except for MVV were performed in triplicate and the highest value for each measure was used for analysis. Vital capacities are reported as percent predicted normal; respiratory pressures were measured as cm H2O, and MVV measured in liters/minute. The best value of 3 efforts was used for analysis. For patients with bulbar dysfunction who were unable to keep a mouth seal, face masks with a soft seal to prevent air leaks were used to perform the pulmonary function measures. In examining the slopes of declines we utilized only participants who completed at least three visits so that we had at least three data sets to create the slope. Additionally, we examined for any differences between Group 1 subjects and Group 2 subjects in order to see if there were changes in the rate of progression as pulmonary function deteriorated. We also collected the ALSFRS-R functional rating score in order to determine whether the rate of pulmonary decline was correlated with the rate of functional decline. Finally, we also examined whether MIP or FVC met Medicare criteria earlier (defined as FVC of ≤ 50% of predicted or MIP was ≤ 60 cMH2O).
Statistical methods
Data were expressed as counts and percentages for categorical variables and median (range or interquartile range) for continuous variables overall as well as by disease group. For each measure, we obtained the subject-specific slope by using the best-fitted line of all the longitudinal values for that subject. The rate of decline of each pulmonary function parameter was then estimated using the median of subject-specific slopes for the absolute drops over time. In this way, we could determine if any of the pulmonary functions might serve as an earlier index of respiratory insufficiency when compared to the FVC. Given that most of the pulmonary function measures were not normally distributed, we used non-parametric tests such as the Wilcoxon rank-sum test to compare the respiratory decline measures between groups as well as to test whether each slope was significantly different from 0 with each group or overall. Spearman correlation coefficient was employed for correlation analyses between variables of interest (e.g. among slopes of decline or between slopes of decline and baseline PFTs). A mixed-effects model was also used to determine if the slopes in time were significantly different than zero within each group or from each other between two groups via an interaction term between time and group, after having adjusted for possible confounders or effect modifiers (i.e. age, gender, and household income) while at the same time to account for the correlation among the observations from the same patients over time. Multiple testing adjustments were not made due to the exploratory nature of this study. p-values less than 0.05 were considered statistically significant. SAS version 9.4 (SAS Institute Inc., Cary, NC) was used for all the data analyses.
Results
73 subjects (63%, male (n=46), 37% female (n=27)) enrolled with a median age (range) of 58(36,82) years. Sixty-five subjects (42 in Group 1 and 23 in Group 2) had at least 3 visits and were used for the analysis of pulmonary function decline (median follow-up (range) 336 (73–371) days). The demographic characteristics of this group are presented in . Over the interval measured, all of the longitudinal rates of decline for the 9 PFTs and ALSFRS scores were statistically significantly different than 0 (, p-value not shown). MEP showed the greatest decline among all measures (−2.60%/month) compared to MIP (−1.59%/month), sFVC (−2.01%/month), LFVC (−2.02%/month), MVV (−1.62%/month), SNIP (−1.25%/month) and LSNIP (−1.42%/month) (, ). When comparing the rate of decline between Group 1 and Group 2, there were no statistically significant differences irrespective of NIV use.
Figure 1 Median overall decline in pulmonary functions over time. This figure demonstrates the decline from origin at time 0 and measured in absolute change in % over time. Note the most rapid decline occurs in the MEP followed by the laying FVC and inspiratory pressures (supine SNIP and MIP). This suggests that pressure measurements should be part of clinical assessments of respiratory function and both be used for determining initiation of NIV.
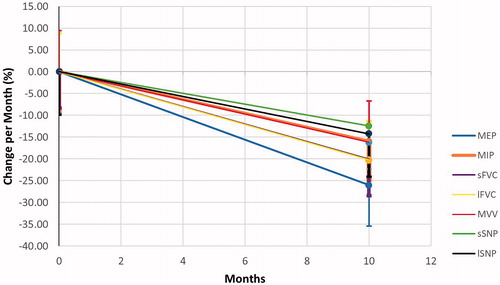
Table 1 Baseline demographics and disease characteristics for patients with ≥3 visits.
Table 2 Rate of decline per month in PFTs and ALS FRS scores overall and by groupa.
Correlations between the baseline values of the pulmonary functions were calculated using Spearman correlation coefficients. We found that all of the baseline values were significantly positively correlated with each other except for MEP and the LFVC, although the strength of association varied from poor to fair (e.g. between LSNIP and SSNIP or LFVC and SVC). (). We also examined the association between the baseline value of each pulmonary function and the rate of decline for all of the variables (). We found that the baseline MIP was significantly negatively associated with the rate of decline in both MIP and MEP. Similarly, the baseline MEP was correlated with the slope of change in MEP and MIP. There was also a significant (mostly fair) negative association between the baseline SNIP and the rate of decline in MIP, MEP and LSNIP as well as between the baseline LSNP and the rate of decline of MIP, MEP, or LSNIP. The baseline ALSFRS-R score was weakly but significantly positively associated with the rate of decline in SFVC and SSNIP. The rate of decline in each of the measured pulmonary functions significantly and positively associated with the rate of decline of all the other parameters except for the rate of decline between MEP and LFVC where p = 0.065 ().
Table 3 Overall correlations among baseline values of pulmonary functions. Spearman Correlation Coefficients Prob > |r| under H0: Rho=0 Number of Observations
Table 4 Overall correlations between baseline values of pulmonary functions and ALS FRS score with rates of their decline over time. Spearman Correlation Coefficients Prob > |r| under H0: Rho=0 Number of Observations
Table 5 Correlations among the rates of decline for PFTS and ALS FRS with each other over time. Spearman Correlation Coefficients Prob > |r| under H0: Rho=0 Number of Observations
All seventy-three subjects were analyzed for current NIV initiation criteria (defined by %FVC and MIP only). Overall, 69 met IP criteria first, 11 met FVC and IP criteria simultaneously, and none met FVC criteria first. Fifty-five subjects met NIV initiation criteria based on IP alone at baseline visit, whereas only 3 met criteria at baseline based on FVC. Thirty-two of the subjects that met NIV criteria by IP never dropped their FVCs low enough to qualify.
Discussion
This pilot study demonstrates that MEP changes fastest from the baseline in patients with ALS compared to the other pulmonary function measures, suggesting a more rapid deterioration of expiratory muscle strength. In fact, MEP changes more rapidly than MIP and FVC which are used to determine eligibility for NIV initiation. Given that the present criteria do not consider MEP and that the criteria to initiate NIV were met earlier using MIP, there is clearly a need to consider MEP in determining both respiratory muscle function and timing of NIV in the clinical setting. Furthermore, it may be useful to integrate MEP and MIP into clinical trials both as part of entry criteria and pulmonary endpoints. Since MEP declines fastest it may be a more sensitive respiratory measure to detect a clinical effect.
Additionally, we have demonstrated that there is a correlation between all the baseline measures of respiratory function and their changes over time as well as with functional changes as measured by the ALSFRS-R score. This supports the similar findings of correlation between ALSFRS-R and FVC that have previously been published (Citation15). However, our study includes longitudinal follow up of not only FVC but measures of muscle strength (MEP and MIP), measures of fatigue, and positional effects on FVC and SNIP. Furthermore, given that expiratory muscle strength declines fastest, attention needs to be given to the implementation of mechanical insufflation/exsufflation (MI-E) devices. Generally, MI-E devices are prescribed based on peak cough flow and while we did not measure peak cough flow, it is likely that reduced expiratory pressures would lead to a decrease in the peak cough flow. This suggests that closer attention should be given to this measure as well.
We also noted no differences in the rates of decline between Group 1 (earlier in disease) and Group 2 irrespective of whether they were on or off NIV. However, this study was not powered to examine the actual effects of NIV on PFT decline.
Beyond using pressure as a criterion to initiate NIV in ALS in addition to lung volumes, the results of this study have pathophysiological importance. As an ALS patient develops weakness of the diaphragm and accessory muscles of inspiration, clearly tidal volume can decrease without the patient experiencing dyspnea. However, it is common that the ALS patient demonstrates bibasilar atelectasis and experiences difficulty with coughing and airway clearance, functions that require expiratory muscle power. The finding that MEP changes are early and more rapid than other objective measures or respiratory decline is supportive of these clinical observations regarding poor pulmonary clearance and suggests that these clearance issues may precede the need for noninvasive ventilation.
The use of measurements of inspiratory respiratory muscle strength to determine a need for ventilation is commonly used in other settings. For instance, when following patients with rapidly-evolving weakness in the ICUs, it is common clinical practice to measure serial MIPs to determine the timing of intubation. The basis for this determination rests on the recognition of the impending critical failure of adequate ventilation, meaning failure to deliver adequate volumes of ventilation. The implication is that weakness precedes failure to deliver tidal volume. This is exactly the same pathophysiological argument for ALS albeit on a different time base. It seems reasonable that optimal clinical practice in ALS would favor the institution of NIV based on serial measurement of MIP and MEP rather than %FVC. MEP may also be useful in predicting the need for airway clearance support (such as training in quad-cough or MIE devices). Similarly, it would follow that serial measures of MIP and MEP could also serve as an informative endpoint in clinical drug trials in ALS.
Importantly, reduction in inspiratory and expiratory pressures may be a modifiable risk factor for respiratory failure. It has been shown that due to small fiber size, abundance of capillaries, and a high aerobic oxidative enzyme activity, diaphragm muscle fibers are resistant to fatigue while intercostal muscles are less resistant due to their fiber type composition. However, the structural and functional characteristics of respiratory muscle fibers are not fixed and can be modified in response to training (Citation16). In fact, expiratory muscle training in ALS subjects demonstrated improved MEP as well as swallowing function over 8 weeks of in-home training (Citation17).
In summary, we would recommend that muscle strength as measured by inspiratory and expiratory pressures should be considered in determining the institution of NIV in clinical settings. We would further advocate for additional studies that examine the impact of muscle training on respiratory function and survival be undertaken and that these measures be considered as respiratory endpoints in clinical trials.
Nutrition/NIPPV Study Group
NIPPV Sites:
Drexel: Terry Heiman-Patterson, MD, Michael Sherman, MD, Melonie Mitchell RPT, Roseanne Sattazahn RN, Sara Feldman, DPT,
Beth Israel: Stephen N. Scelsa, MD, Theresa Imperato RN
SUNY: Jeremy M. Shefner, MD, Ph.D., Mary Lou Watson RN
University of Colorado: Yvonne Rollins, MD, John Cumming
Henry Ford Hospital: Dan Newman, MD, Helen Foley RN
University of Miami: Ashok Verma, MD, Donald Koggan, MD, Julie Steele RN
University of Texas Health Science Center at San Antonio: Carlayne Jackson, MD, Pam Kittrell, RN.
Statistical Support: Temple University Lewis Katz School of Medicine: Daohai Yu, PhD, Xiaoning Lu, MS.
Nutrition Sites
University of Kentucky: -Edward J. Kasarskis, MD, PhD – PI, Stephen Wells, Kathryn Vanderpool, RN, Christie Shrestha, MPH, Deborah Taylor, Marta Mendiondo, Ph.D, Richard Kryscio, Ph.D, Margaret Healey, Marsha Sams, Megan Thompson; Columbia: Hiroshi Mitsumoto, MD, FAAN, Daniel Bell; University of Vermont: Rup Tandan, MD, Chris Potter, Dwight Matthews; University of Utah: Mark Bromberg, MD, Summer Davis; Pennsylvania State University: Zachary Simmons, MD, FAAN, Beth Stephens RN, Ally Brothers.
Disclosures statement
Dr. Heiman Patterson has received payment for serving on the medical advisory board for Mitsubishi Tanabe Pharma America, Biogen, Samus, Cytokinetics, and Orphazyme. She has received an honorarium from IQVA and received a consulting fee from Evidera.
Dr. Khaazal has no relevant disclosures
Dr. Sherman has no relevant disclosures.
Dr. Jackson has served as a consultant for Mitsubishi Tanabe Pharma America, Cytokinetics, ITF Pharma and Brainstorm.
Dr. Daohai Yu has no relevant disclosures.
Dr. Kasarskis has research support from Alexion, AB Science, Neuraltus and collaborative research support from Healey Platform trial, Amylyx, Kansas University, and Columbia University.
Additional information
Funding
References
- Miller RG, Jackson CE, Kasarskis EJ, England JD, Forshew D, Johnston W, et al.; Quality Standards Subcommittee of the American Academy of Neurology. Practice Parameter update: The care of the patient with amyotrophic lateral sclerosis: drug, nutritional, and respiratory therapies (an evidence-based review): report of the quality standards subcommittee of the American Academy of Neurology. Neurology 2009;73:1218–26.
- Lo Coco D, Marchese S, Pesco MC, La Bella V, Piccoli F, Lo Coco A, et al. Non-invasive positive-pressure ventilation in ALS: predictors of tolerance and survival. Neurology 2006;67:761–5.
- Kleopa KA, Sherman M, Neal B, Romano GJ, Heiman-Patterson T. Bipap improves survival and rate of pulmonary function decline in patients with ALS. J Neurol Sci. 1999;164:82–8.
- Aboussouan LS, Khan SU, Banerjee M, Arroliga AC, Mitsumoto H. Objective measures of the efficacy of noninvasive positive-pressure ventilation in ALS. Muscle Nerve. 2001;24:403–9.
- Lyall RA, Donaldson N, Fleming T, Wood C, Newsom-Davis I, Polkey MI, et al. A prospective study of quality of life in ALS patients treated with noninvasive ventilation. Neurology. 2001;57:153–6.
- Newsome-Davis IC, et al. The effect of noninvasive positive pressure ventilation (NIPPV) on cognitive function in ALS: a prospective study. J Neurol Neurosurg Psychiatry. 2001;71:482–7.
- Martin S, Trevor-Jones E, Khan S, Shaw K, Marchment D, Kulka A, et al. The benefit of evolving multidisciplinary care in ALS: a diagnostic cohort survival comparison. Amyotroph Lateral Scler Frontotemporal Degener. 2017;18:569–75.
- Aboussouan LS, Mireles-Cabodevila E. Respiratory support in patients with amyotrophic lateral sclerosis. Respir Care. 2013;58:1555–8.
- Andersen PM, Abrahams S, Borasio GD, de Carvalho M, Chio A, Van Damme P, et al. EFNS guidelines on the clinical management of amyotrophic lateral sclerosis (MALS)-revised report of an EFNS task force. Eur J Neurol. 2012;19:360–75.
- Heiman-Patterson TD, Cudkowicz ME, De Carvalho M, Genge A, Hardiman O, Jackson CE, et al. Understanding the use of NIV in ALS: results of an international ALS specialist survey. Amyotroph Lateral Scler Frontotemporal Degener. 2018; 19:331–41.
- Jacobs TL, Brown DL, Baek J, Migda EM, Funckes T, Gruis KL. Trial of early noninvasive ventilation for ALS: A pilot placebo-controlled study. Neurology 2016;87:1878–83.
- Vitacca M, Montini A, Lunetta C, Banfi P, Bertella E, De Mattia E, et al.; ALS RESPILOM Study Group. Impact of an early respiratory care programme with non-invasive ventilation adaptation in patients with amyotrophic lateral sclerosis. Eur J Neurol. 2018;25:556–e33.
- Noah L, et al. Respiratory measures in amyotrophic lateral sclerosis. Amyotrophic Lateral Sclerosis Frontotemporal Degen 2018;19:321–30.
- Kasarskis EJ, Mendiondo MS, Wells S, Malguizo MS, Thompson M, Healey M, et al.; ALS Nutrition/NIPPV Study Group. The ALS Nutrition/NIPPV Study: design, feasibility, and initial results. Amyotroph Lateral Scler. 2011;12:17–25.
- Jackson C, De Carvalho M, Genge A, Heiman-Patterson T, Shefner JM, Wei J, et al. Relationships between slow vital capacity and measures of respiratory function on the ALSFRS-R. Amyotrophic Lateral Sclerosis Frontotemporal Degen. 2018;19:506–12.
- Polla B, D'Antona G, Bottinelli R, Reggiani C. Respiratory muscle fibres: specialisation and plasticity. Thorax 2004;59:808–17.
- Plowman EK, Tabor-Gray L, Rosado KM, Vasilopoulos T, Robison R, Chapin JL, et al. Impact of expiratory strength training in amyotrophic lateral sclerosis: Results of a randomized, sham-controlled trial. Muscle Nerve. 2019;59:40–6. Epub 2018 Nov 29. PMID: 29981250.