Abstract
Background: Little is known about the effects of verbal instructions on the technique and performance of sports-related tasks. The aim of this study was therefore to investigate the acute effects of knee alignment instructions on knee kinematics and the performance of a single-leg jump in female adolescent soccer players.
Methods: Twenty-eight adolescent female soccer players completed three blocks of 15 single-leg jumps. The participants were randomised to an experimental group receiving verbal instructions focussing on proper knee alignment (the avoidance of knee valgus) or to a control group only receiving instructions on task execution. Outcomes were knee kinematics (flexion and valgus angles) and jump performance (number of incorrect trials and contact time).
Results: The experimental group demonstrated 36% less knee valgus and 11–13% greater knee flexion compared to the control group. While jump performance remained unchanged in the experimental group over the course of the experiment, the control group demonstrated a 20% decrease in the number of incorrect trials and a 25% reduction in contact time over the course of the experiment.
Conclusions: The present results indicate that verbal instructions accentuating proper knee alignment benefit knee kinematics but not jump performance in adolescent female soccer athletes.
Introduction
In the context of rehabilitation, verbal instructions are often given by the therapist to guide the individual toward enhanced performance or a certain technique. The most common approach of using verbal instructions is to direct the attention of the individual internally, i.e. focussing on the movements of the body (e.g. body posture or joint angles) [Citation1]. Such internal instructions are often used in knee injury prevention exercises that aim to sustain proper knee alignment, e.g. by using internal cues (e.g. “keep your knee aligned with your foot”) [Citation2,Citation3].
Injuries to the anterior cruciate ligament (ACL) are receiving a great deal of attention because of the high incidence of injury and increased risk of early onset of osteoarthritis [Citation4]. The majority of the ACL injuries are non-contact in nature and most of these occur during sudden changes in direction and pivoting [Citation5]. The position of the lower extremity while completing these tasks is thought to be a factor contributing to ACL rupture [Citation6]. More specifically, excessive knee valgus during pivoting manoeuvres (e.g. while running and landing) is a risk factor of ACL injury [Citation5] since this position of the knee increases the relative ACL strain [Citation7]. Poor muscular control of the knee and trunk have also been associated with increased knee valgus during cutting movements [Citation6,Citation8]. Exercises focussing on the sustainment of knee alignment in the frontal plane is therefore an important element in knee prevention programmes given the increased risk of ACL injury among individuals with excessive knee valgus [Citation9].
Using internal verbal instructions to guide knee alignment exercises has been shown to be effective for the prevention of knee injury in athletes [Citation10–12]. More specifically, Walden et al. [Citation12] found a 64% decrease in the incidence rate of ACL injuries in adolescent female soccer players participating in knee alignment exercises, together with motor control core exercises, all guided by verbal internal instructions. Female adolescents have received specific attention for proper knee alignment exercises because of their increased risk of ACL injury [Citation13,Citation14], which is partly due to the excessive knee valgus demonstrated in this population [Citation9,Citation15,Citation16]. In soccer specifically, the rate of ACL injury is more than twofold higher in females than in males [Citation13,Citation17].
Although internal instructions are commonly used in rehabilitation, previous findings from motor control research have shown that internal instructions degrade the performance of sports-related tasks (e.g. jumping and running) [Citation2,Citation18–20]. This negative effect of internal instructions is believed to derive from the conscious control of movements while an individual is directing their attention towards how their body is moving [Citation21,Citation22]. Still, little is known about the effect of knee alignment instructions on outcomes related to the technique of sports-related tasks [Citation2].
Altogether, despite the reported disadvantages of internal verbal instructions [Citation2,Citation22], clinical trials support the use of this treatment approach for injury prevention [Citation10–12]. However, it is unclear whether these positive results on injury prevention are the result from neuromuscular training per se or the combination of verbal instructions and neuromuscular training. To address this, an experimental study was conducted to investigate the acute effects of knee alignment instructions on knee kinematics, kinetics and the performance of a single-leg jump in female adolescent soccer players.
Methods
Study design and participants
In this cross-sectional study, participants were randomised either to an experimental group receiving verbal instructions on task execution and on proper knee alignment or to a control group only receiving verbal instructions on task execution. Randomisation was performed in blocks of two, with the use of a web-based software (www.randomisation.com). The power calculation for this study was based on a pilot study on 20 participants (unpublished data) with maximal knee valgus as the primary outcome using the same design as the present study. A power analysis (0.80 power, p < .05) showed that a sample size of 12 participants in each group was required to detect a 4.0° difference between the groups (standard deviation of 4.0°).
Twenty-eight adolescent soccer players were recruited from four different soccer clubs in Stockholm. All participants were aged between 12 and 14 years, were practicing soccer ≥2 sessions per week, and were free of any previous or ongoing injuries to the lower extremities. This study was approved by the Regional Board of Ethics in Stockholm, and all participants, as well as their parents, provided written informed consent prior to their enrolment in the study.
Testing procedures
All assessments were conducted in a movement laboratory setting. The experimental task contained two consecutive single-leg jumps where the participant landed on the dominant leg (i.e. the leg used to kick a ball) after both jumps. The dominant leg was analysed in this study since larger knee valgus angles have been demonstrated for the dominant leg compared to the non-dominant leg in athletes [Citation23]. This difference between the extremities is believed to reflect the specialisation of each leg, i.e. the manipulating role of the dominant kicking-leg compared to the stabilising role adopted by the non-dominant leg. Participants wore tight-fitting clothes and their normal indoor shoes during testing. Prior to the assessment of single-leg jump performance, static knee alignment was assessed during quiet standing (i.e. in a normal upright posture) for 10 s as a reference condition.
The participants were positioned 0.5 m from the nearest edge of two force plates () standing with both legs on the floor with the dominant foot somewhat in front of the non-dominant foot. To standardise the height of the first jump, a balloon was aligned with the front edge of force plate 1 and positioned 0.1 m above the participant’s head. The centre of the two force plates was marked with tape as a reference for the participants. The participants were allowed to look at the landing spot in order to guide the direction of the two jumps and were instructed to keep their arms beside their body while jumping.
Figure 1. (A) Top-view of the experimental setup including two force plates and a balloon (dotted circle) that is positioned 0.1 m above the head of the participant and aligned with the edge of force plate 1. The task included two consecutive single-leg jumps: (1) A forward jump aiming to touch the balloon with the forehead and thereafter land on the dominant leg with the foot entirely on force plate 1; and (2) a sideway cut at a 90° angle in the direction opposite to the leg used for jumping (landing on the left leg required a cut to the right as in this example) and landing entirely on force plate 2. (B) Representative ground reaction force data for the two single-leg jumps. The vertical lines illustrate the landing of jump 1, the take-off for jump 2, and the landing of jump 2, respectively.
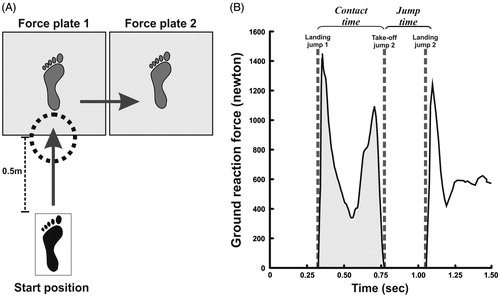
Two consecutive single-leg jumps were performed (). The first jump was a forward jump by the dominant leg aiming to touch the balloon with the forehead and landing on the dominant leg with the foot positioned entirely on force plate 1. The second jump was a sideways cut at a 90° angle in the direction opposite to the dominant leg (landing on the right leg required a cut to the left) aiming to land and stand still on the dominant leg entirely on force plate 2. After the landing of the second jump, the participant was given approximately three seconds to regain stability. The sequence of two jumps including a quick change in medio-lateral direction was used in this study as this type of sport-related movement has been associated with noncontact ACL injuries [Citation4,Citation5].
The participants performed a total of 45 jumps, divided into three blocks of 15 jumps (block A: jump 1–15; block B: jump 16–30; block C: jump 31–45), with 10 min’ rest between each block to avoid fatigue or drop in alertness. Each block was divided into three subsets of five consecutive jumps, with approximately 15 s between each jump. The single-leg jump had not been performed by any participants before.
Verbal instructions
Before the start of data collection, the test leader (a physiotherapist specialised in movement science) demonstrated the single-leg jump and gave all participants the following instructions focussing on the execution of the jump: “Jump forward and touch the balloon with your forehead, land on the first force plate, jump sideways as fast as possible to the second force plate, and then try to stand as still as possible”.
Nine times during the experiment (i.e. before each subset of five jumps), the test leader gave the experimental group instructions focussing on sustaining knee alignment in the frontal plane. Based upon the instructions used in a previous knee injury prevention programme [Citation12], these instructions were phrased: “Keep your knee aligned with your foot and maintain a stable trunk by contracting your core muscles”. The instructions for contracting the trunk muscles were added because excessive movements of these segments have been shown to effect knee alignment [Citation24]. The control group only received task execution instructions (i.e. the same instructions received by both groups prior to testing), and these were given at the same time as the experimental group. No feedback about the technique or performance of the jump were provided to the participants during testing.
Measurements
An eight-camera motion analysis system (Elite 2002, version 2.8.4380; BTS, Milan, Italy) was used to record at 100 Hz the position of six spherical retro-reflective markers located on the greater trochanter, the proximal femur (the midpoint between the spina iliaca anterior superior and the apex patella), the lateral and medial femoral epicondyles, the tibia (the midpoint between the apex patella and the midpoint between the two ankle malleoli), and the lateral malleolus of the dominant leg [Citation24,Citation25]. Simultaneously with the kinematic data collection, ground reaction forces (GRFs) were measured at 100 Hz using two Kistler force plates (0.5 × 0.5 m) (Kistler Group, Winterthur, Switzerland). Two orthogonally placed digital video cameras recorded all of the trials in the sagittal and frontal plane from a distance of 3 m. Three-dimensional trajectories of the markers were reconstructed using a tracking system (Tracklab-BTS, Milan, Italy). Kinematic and kinetic data were processed using MATLAB software (MATLAB, 7.4.0; MathWorks, Natick, MA, USA).
Outcome measurements
The outcomes retained for analysis focussed on jump performance (number of incorrect trials, contact time and jump time), knee kinematics (maximal valgus and flexion angles), and kinetics (GRF and postural sway) of the two single-leg jumps.
The number of incorrect trials was used as an overall measure of jump performance. A jump was classified as incorrect if the individual did not touch the balloon with the head, did not land with the foot entirely on the force plates, or touched the ground with the non-stance leg during the jump sequence. Incorrect trials were detected by the experimenter during data collection, and video recordings were used for reassessment if needed. Incorrect trials were not repeated, i.e. all subjects performed 45 jumps.
The time of landing for the first jump and the take-off and landing of the second jump were determined based on GRF (). A threshold of 10 N was applied to detect these events, i.e. >10 N reflected the onset of landing and <10 N reflected the onset of take-off (). These time events were used to calculate the contact time of the first jump (i.e. the time between the landing of the first jump and the take-off of the second jump) and the jump time of the second jump (i.e. time between the take-off and landing of the second jump). Shorter contact time and jump time were considered to reflect superior jump performance.
Frontal knee alignment (knee valgus and varus) was calculated as the angle between a proximal femur marker, an estimated point at the distal femur (the centre position between the lateral and medial femur epicondyles), and the mid-shank marker [Citation14]. Knee flexion was calculated as the angle between the trochanter major, the lateral femur epicondyle, and the lateral malleolus. Maximum knee valgus, knee flexion, and GRF during the contact phase of the first and second jump were analysed.
The cumulative linear displacement (mm) of the centre of pressure (CoP) was obtained from the contact phase of the second landing. Because all participants stabilised their body quickly after landing, only the CoP displacement during the first 250 ms after landing was analysed.
Statistical analyses
Participant characteristics (age, height, weight, dominant leg), hours of soccer practice per week and static knee alignment are presented as means (standard deviation), medians (interquartile range [IQR]), or proportions (percentage). For the single-leg jump outcomes, the average of 15 consecutive jumps was analysed (i.e. block A, B and C). Because the outcomes were overall not normally distributed, the Mann–Whitney U-test and the Wilcoxon signed rank test were used to test differences between groups and within groups, respectively. Corrections for multiple statistical testing were not used due to the light of criticism of such corrections (e.g. Bonferroni) to minimise the incidence of false negative when examining hypotheses [Citation26]. To obtain information regarding the magnitude of within-group differences between block A and C, non-parametric effect sizes (ES) were calculated based on the z-values obtained from the Wilcoxon signed-rank test (effect size = z/√n). Effect sizes were classified as follows: small effect = 0.1; medium effect = 0.3; and large effect = 0.5 [Citation27]. The significance level was set at p ≤ .05, and the data are presented as median and interquartile ranges.
Results
Participants’ characteristics and static knee alignment in the experimental and the control group are presented in . All participants completed the entire test session of 45 single-leg jumps. There was no significant difference in median static knee alignment between the experimental group and control group (3.3°, IQR: 7.6° vs. 4.7°, IQR: 8.7°, p = .43). Seventeen trials (1%) out of a total of 1260 jumps were excluded due to technical problems (these were randomly distributed across the participants and blocks). The results for jump performance, knee kinematics, GRF and postural stabilisation are presented in and the effects sizes for these outcomes are illustrated in . Typical patterns of jump performance, knee kinematics and postural stabilisation for a subject in the experimental and control group are presented in .
Figure 2. Within-group effect sizes of jump performance, knee kinematics, ground-reaction forces (GRF) and postural stabilisation. The vertical dashed lines illustrate small (0.1), medium (0.3) and large (0.5) changes between block A and B for the experimental group and the control group.
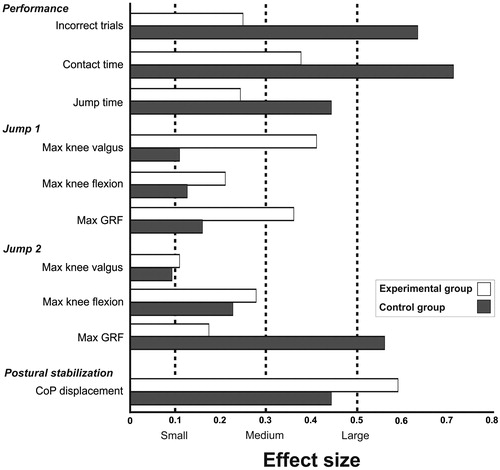
Figure 3. Typical patterns of (A) contact time (s), (B) max knee valgus (°), (C) max knee flexion (°) and (D) centre of pressure (CoP) displacement (mm) plotted for a subject in the experimental group (i.e. verbal instructions focussing on sustaining knee alignment in the frontal plane) and in the control group (i.e. verbal instructions focussing on the execution of the jump). Each data point represents an average of three jumps.
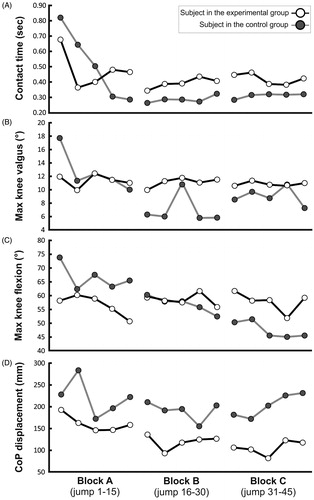
Table 1. Participants’ characteristics.
Table 2. Jump performance, knee kinematics and kinetics in the experimental group and control group throughout the experiment, i.e. block A (jump 1–15), block B (jump 16–30) and block C (jump 31–45).
Jump performance
The number of incorrect trials, contact time, and jump time remained unchanged in the experimental group throughout the experiment (, p > .16). In contrast, the control group demonstrated a 20% reduction in the number of incorrect trials (ES: 0.63, T = 11.0, p = .03) and a 25% decrease in contact time (ES: 0.71, T = 10.0, p < .01, ) between blocks A and C. The experimental group demonstrated a 31% longer contact time in block C compared to the control group (median 0.45 s vs. 0.33 s; U = 53.0, p = .04, ).
Figure 4. (A) Contact time (s), (B) max knee valgus (°), (C) max knee flexion (°) and (D) centre of pressure (CoP) displacement (mm) in the experimental group and control group during block A (jump 1–15), block B (jump 16–30) and block C (jump 31–45). Data are presented as median and interquartile range. *p ≤ .05.
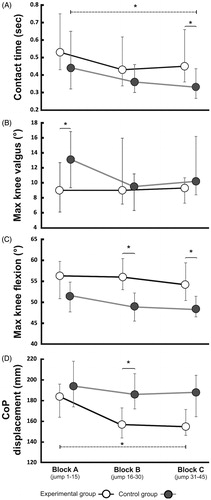
Knee kinematics and ground reaction forces
The experimental group demonstrated 4.6° (36%) less knee valgus during the first landing compared to the control group in block A (U = 51.0, p = .03, ). The experimental group also demonstrated 5.8°–7.0° (11–13%) greater knee flexion for the first landing during block B (U = 38.0, p < .01) and block C compared to the control group (U = 50.0, p = .03, ). The GRF remained unchanged throughout the experiment in the experimental group, whereas the control group showed a 6% increase (71 N, ES: 0.56) in GRF for the second landing between blocks A and C (T = 19.0, p = .04).
Postural stabilisation
As shown in , the experimental group showed a 29 mm decrease (16%, ES: 0.59) in CoP displacement between blocks A and C (T = 15.0, p = .03) and demonstrated 29 mm (17%) lower CoP displacement during block B compared to the control group (U = 48.0, p = .04). The experimental group demonstrated a trend towards lower CoP displacement (33 mm, 19%) during block C compared to the control group (U = 52.0, p = .06).
Discussion
The aim of this experimental study was to investigate the effects of knee alignment instructions on knee kinematics and performance of a single-leg jump in female adolescent soccer players. While this study found acute benefits of knee alignment instructions on knee kinematics, the experimental group demonstrated worse jump performance than the control group. These findings accentuate the importance for physiotherapists to be aware of the diverse effects of knee alignment instructions while tailoring rehabilitation programmes.
In line with recent clinical trials [Citation5,Citation12], the findings of the present study partly support the notion of a combined effect of knee alignment exercises and verbal internal instructions for the improvement of frontal knee alignment in adolescent soccer players. The lower knee valgus, approximately 5°, in the experimental group might hold clinical relevance because excessive knee valgus has been shown to be an independent risk factor for ACL injury [Citation14]. The verbal instructions used in this study partly aimed to direct the participants’ attention toward the adjacent joints because knee valgus has been shown to be affected by displacement of the pelvis [Citation28] and the trunk in the frontal plane [Citation24]. The instructions were also similar to the instructions used in preventive exercises for knee injury [Citation12]. On the other hand, the positive effect of verbal instructions on knee valgus was only observed in the early phase (block A) of the experiment whereas no differences between the groups were found in block B and C. This suggests that female adolescent soccer players directly adopted an explicit learning process in which they were able to use verbal internal instruction to improve frontal knee alignment. However, such explicit learning places high demands on attention might therefore be less durable in performance gains [Citation29] which could explain the lack of benefits of verbal instructions in the later phases of the experiment. It is important to recognise the need to further investigate the underlying mechanism for the clinical effects of knee alignment instructions as well as the to determine the sustainability of these effects.
Furthermore, compared to the experimental group, the control group also adopted a jump strategy characterised by reduced knee flexion during landing. Because knee flexion is crucial for efficient force absorption [Citation30], this strategy could reflect less fine-tuned control of the knee during landing in the control group. Such a knee stiffening strategy has previously been demonstrated during varying jumping tasks in individuals with patellofemoral pain [Citation14,Citation24,Citation31] and during gait in individuals with ACL ruptures [Citation32,Citation33]. Reduced knee flexion during landing is also a risk factor for knee injury [Citation14,Citation34]. Therefore, the combination of greater knee valgus and reduced knee flexion in the control group likely resulted in an increased mechanical load. This is in line with previous findings that training programmes focussing on neuromuscular training and strength training (with the use of external focus) was effective in the prevention of knee injuries in young athletes [Citation5].
While jump performance remained unchanged in the experimental group, the control group showed a 20–25% improvement in jump accuracy and shorter contact time across the experiment. These findings are in line with previous studies showing reduced performance when using verbal instructions that direct the focus internally towards the body [Citation2,Citation22]. Specifically, previous findings have revealed that internal instructions could degrade the performance of vertical and horizontal jumps [Citation2,Citation19–21] and running [Citation18]. The lack of improvements in performance in the experimental group could have derived from increased awareness of the body, which in turn could interfere with movement automaticity and performance [Citation35]. In the context of rehabilitation, the present findings imply that it is important for physiotherapists to be aware of the diverse effects of internal verbal instructions. There is likely a trade-off between the accuracy of movement characteristics (e.g. knee alignment) and task performance. Therefore, shifting between internal verbal instructions and instructions focussing on task execution could be a beneficial approach for rehabilitation.
While the experimental group decreased their postural sway between block A and C, no changes occurred in the control group. This results correspond with previous findings regarding the benefits of frontal knee alignment for body stability (e.g. trunk control) [Citation28]. In contrast, the lack of such learning effect in the control group could have been the result of the decrease in contact time and increase in GRF during the second jump occurring in this group between block A and C. In other words, a faster movement with higher force could be more difficult to stabilise.
The main strengths of this study were the randomised design and the objective assessment of different characteristics of the single-leg jump. However, this study has some limitations requiring consideration. First, we did not control for the maturity status of the participants which could have been relevant as differences in neuromuscular performance have been observed between girls’ soccer players after, compared to before their growth spurt [Citation36]. The study sample was restricted to female soccer players aged between 12 and 14 years which limits the generalisation of this study. On the other hand, this group of athletes is important to investigate due to their increased risk of ACL injury [Citation15]. Second, there was no baseline assessment included in the present study prior to the provision of verbal instructions since this study aimed to investigate novel task performance (i.e. starting when the task was unfamiliar to the participants). As changes in movement characteristics and improved performance of the single-leg jump may occur after only a few trials it was expected that the inclusion of baseline assessment might had interfered with the process of short-term learning. Third, a cross-over design (i.e. each participant act as their own control) could had been a relevant choice of design for the present aim. On the other hand, a cross-over design is prone to carry-over effects between the experiment and control arms and was therefore not used. The lack of baseline assessment and the potential benefits of a cross-over design for the purpose of this study needs to take into consideration while interpreting the results from this study. Finally, although the present study explored acute effects of knee alignment instructions across 45 single-leg jumps, a retention test to assess changes across a longer time span was not included. It is therefore recommended that future studies should investigate the effects of different type of instructions on kinematics and performance by using a longitudinal design to address the sustainability of these effects.
Conclusions
To conclude, the present results indicate that verbal instructions accentuating proper knee alignment benefit knee kinematics but not jump performance in adolescent female soccer athletes. These findings suggest that it is important in rehabilitation to be aware of these varying effects of knee alignment instructions and alternating between verbal instructions focussing on internal feedback (e.g. body posture) and task execution (e.g. performance) could be a beneficial approach for rehabilitation.
Attached_file-_TIDieR-Checklist_Conradsson_et_al.docx
Download MS Word (28.8 KB)Acknowledgements
The authors would like to thank the participants and the physiotherapy students who were involved in this project for their time and energy.
Disclosure statement
No potential conflict of interest was reported by the authors.
References
- Philips N. Motor learning. In: Everett T, Kell, C., editors. Human movement – an introductory text. 6th ed. Edinburgh (UK): Churchill Livingstone; 2010. p. 85–99.
- Benjaminse A, Welling W, Otten B, et al. Novel methods of instruction in ACL injury prevention programs, a systematic review. Phys Ther Sport. 2015;16:176–186. 10.1016/j.ptsp.2014.06.003.
- Michaelidis M, Koumantakis GA. Effects of knee injury primary prevention programs on anterior cruciate ligament injury rates in female athletes in different sports: a systematic review. Phys Ther Sport. 2014;15:200–210. 10.1016/j.ptsp.2013.12.002.
- LaBella CR, Hennrikus W, Hewett TE, et al. Anterior cruciate ligament injuries: diagnosis, treatment, and prevention. Pediatrics 2014;133:e1437–e1450. 10.1542/peds.2014-0623.
- Nessler T, Denney L, Sampley J. ACL injury prevention: what does research tell us? Curr Rev Musculoskelet Med. 2017;10:281–288. 10.1007/s12178-017-9416-5.
- Olsen OE, Myklebust G, Engebretsen L, et al. Injury mechanisms for anterior cruciate ligament injuries in team handball. Am J Sports Med. 2004;32:1002–1012. 10.1177/0363546503261724.
- Withrow TJ, Huston LJ, Wojtys EM, et al. The effect of an impulsive knee valgus moment on in vitro relative ACL strain during a simulated jump landing. Clin Biomech (Bristol, Avon). 2006;21:977–983. 10.1016/j.clinbiomech.2006.05.001.
- Frank B, Bell DR, Norcross MF, et al. Trunk and hip biomechanics influence anterior cruciate loading mechanisms in physically active participants. Am J Sports Med. 2013;41:2676–2683. 10.1177/0363546513496625.
- Hewett TE, Myer GD, Ford KR. Anterior cruciate ligament injuries in female athletes: Part 1, mechanisms and risk factors. Am J Sports Med. 2006;34:299–311. 10.1177/0363546505284183.
- Myklebust G, Engebretsen L, Braekken IH, et al. Prevention of anterior cruciate ligament injuries in female team handball players: a prospective intervention study over three seasons. Clin J Sport Med. 2003;13:71–78.
- Irmischer BS, Harris C, Pfeiffer RP, et al. Effects of a knee ligament injury prevention exercise program on impact forces in women. J Strength Cond Res. 2004;18:703–7. 10.1519/R-13473.1.
- Walden M, Atroshi I, Magnusson H, et al. Prevention of acute knee injuries in adolescent female football players: cluster randomised controlled trial. BMJ 2012;344:e3042.
- Prodromos CC, Han Y, Rogowski J, et al. A meta-analysis of the incidence of anterior cruciate ligament tears as a function of gender, sport, and a knee injury-reduction regimen. Arthroscopy 2007;23:1320–1325 e6. 10.1016/j.arthro.2007.07.003.
- Hewett TE, Myer GD, Ford KR, et al. Biomechanical measures of neuromuscular control and valgus loading of the knee predict anterior cruciate ligament injury risk in female athletes: a prospective study. Am J Sports Med. 2005;33:492–501. 10.1177/0363546504269591.
- Ford K, Myer G, Toms H, et al. Gender differences in the kinematics of unanticipated cutting in young athletes. Med Sci Sports Exerc. 2005;37:124–129.
- Jenkins W, Blaise Williams III DS, Williams K, et al. Sex differences in total frontal plane knee movement and velocity during a functional single-leg landing. Phys Ther Sport 2017;24:1.
- Walden M, Hagglund M, Werner J, et al. The epidemiology of anterior cruciate ligament injury in football (soccer): a review of the literature from a gender-related perspective. Knee Surg Sports Traumatol Arthrosc. 2011; 19:3–10. 10.1007/s00167-010-1172-7.
- Porter JM, Wu WF, Crossley RM, et al. Adopting an external focus of attention improves sprinting performance in low-skilled sprinters. J Strength Cond Res. 2015;29:947–953. 10.1097/jsc.0000000000000229.
- Porter JM, Anton PM, Wu WF. Increasing the distance of an external focus of attention enhances standing long jump performance. J Strength Cond. 2012;26:2389–2393. 10.1519/JSC.0b013e31823f275c.
- Wulf G, Zachry T, Granados C, et al. Increases in jump-and-reach height through an external focus of attention. Int J Sports Sci Coach. 2007;2:275–284.
- Wulf G, Lewthwaite R. Optimizing performance through intrinsic motivation and attention for learning: the OPTIMAL theory of motor learning. Psychon Bull Rev 2016;23:1382–1414. 10.3758/s13423-015-0999-9.
- Wulf G. Attentional focus and motor learning: a review of 15 years. Int Rev Sport Exercise Psychol. 2013;6:77–104. 10.1080/1750984X.2012.723728.
- Ludwig O, Simon S, Piret J, et al. Differences in the dominant and non-dominant knee valgus angle in junior elite and amateur soccer players after unilateral landing. Sports (Basel) 2017; 5(1):14. 10.3390/sports5010014.
- dos Reis AC, Correa JC, Bley AS, et al. Kinematic and kinetic analysis of the single-leg triple hop test in women with and without patellofemoral pain. J Orthop Sports Phys Ther. 2015;45:799–807. 10.2519/jospt.2015.5011.
- Stiffler MR, Pennuto AP, Smith MD, et al. Range of motion, postural alignment, and LESS score differences of those with and without excessive medial knee displacement. Clin J Sport Med. 2015;25:61–66. 10.1097/JSM.0000000000000106.
- Perneger TV. What’s wrong with Bonferroni adjustments. BMJ 1998;316:1236–1238.
- Fritz CO, Morris PE, Richler JJ. Effect size estimates: current use, calculations, and interpretation. J Exp Psychol Gen. 2012;141:2–18. 10.1037/a0024338.
- Kagaya Y, Fujii Y, Nishizono H. Association between hip abductor function, rear-foot dynamic alignment, and dynamic knee valgus during single-leg squats and drop landings. J Sport Health Sci. 2015;4:182–187. 10.1016/j.jshs.2013.08.002.
- Steenbergen B, van der Kamp J, Verneau M, et al. Implicit and explicit learning: applications from basic research to sports for individuals with impaired movement dynamics. Disabil Rehabil. 2010;32:1509–1516. 10.3109/09638288.2010.497035.
- Hoy MG, Zajac FE, Gordon ME. A musculoskeletal model of the human lower extremity: the effect of muscle, tendon, and moment arm on the moment-angle relationship of musculotendon actuators at the hip, knee, and ankle. J Biomech. 1990;23:157–169.
- Rosen AB, Ko J, Simpson KJ, et al. Lower extremity kinematics during a drop jump in individuals with patellar tendinopathy. Orthop J Sports Med. 2015;3:2325967115576100. 10.1177/2325967115576100.
- Beard DJ, Soundarapandian RS, O’Connor JJ, et al. Gait and electromyographic analysis of anterior cruciate ligament deficient subjects. Gait Posture. 1996;4:83–88.
- Rudolph KS, Eastlack ME, Axe MJ, et al. 1998 Basmajian Student Award Paper: movement patterns after anterior cruciate ligament injury: a comparison of patients who compensate well for the injury and those who require operative stabilization. J Electromyogr Kinesiol. 1998;8:349–362.
- Podraza JT, White SC. Effect of knee flexion angle on ground reaction forces, knee moments and muscle co-contraction during an impact-like deceleration landing: implications for the non-contact mechanism of ACL injury. Knee 2010;17:291–295. 10.1016/j.knee.2010.02.013.
- Wulf G, McNevin N, Shea CH. The automaticity of complex motor skill learning as a function of attentional focus. Q J Exp Psychol A. 2001;54:1143–1154. 10.1080/713756012.
- Emmonds S, Morris R, Murray E, et al. The influence of age and maturity status on the maximum and explosive strength characteristics of elite youth female soccer players. Sci Med Football. 2017;1:209–215.